Introduction
China is the largest cotton producer in the world. Due to its large population and limited arable land area, China has widely adopted the use of double- or multi-cropping systems to increase crop productivity per unit land area with extended growing seasons in regions with sufficient heat and light resources (Mao et al., Reference Mao, Zhang, Zhang, Jochem, Wopke, Wang, Sun, Su and Huub2015; Zhang et al., Reference Zhang, van der Werf, Zhang, Li and Spiertz2008). However, the yield of single crops in a double- or multi-cropping system is generally decreased due to competition for resources between crops (Szumigalski et al., Reference Szumigalski and Van Acker2006). Significant differences between actual crop yield and potential yield can be attributed to low resource productivity, while increased resource use efficiency can reduce environmental impacts (Shabu, Reference Shabu2013). Therefore, improving resource use efficiency might be an effective measure to improve crop yield and then maximize the productivity of the whole system in a double- or multi-cropping system.
Resource use efficiency is defined as the ability to derive maximum output per unit of resource and is the key indicator of production/ecological sustainability of crop production (De Vries et al., Reference De Vries, Van de Ven, Van Ittersum and Giller2010; Van Ittersum and Rabbinge, Reference Van Ittersum and Rabbinge1997). Among several ecological factors that reduce productivity, the lack of light and heat is most crucial in determining crop success and more efficient use of heat and light resources can further improve crop productivity (Caviglia et al., Reference Caviglia, Sadras and Andrade2004; Caviglia and Andrade, Reference Caviglia and Andrade2010; Zhang et al., Reference Zhang, Zhang, Yang, Song, Zhao, Ji and Zhu2011). Previous studies have indicated that increased interception of solar radiation, greater light use efficiency, or their combination can effectively improve crop biomass production and yield (Keating and Carberry Reference Keating and Carberry1993; Midmore, Reference Midmore1993; Willey, Reference Willey1990). In addition to light, heat is another key factor in determining crop yield and is also a major input to crop production. Heat determines the length of the growing season and is strongly related to crop yield and quality (Burke et al., Reference Burke, Mahan and Hatfield1988; Keerthi et al., Reference Keerthi, Pannu, Singh and Dhaka2016; Liakatas et al., Reference Liakatas, Roussopoulos and Whittington1998; Reddy et al., Reference Reddy, Davidonis, Johnson and Vinyard1999; Waddle, Reference Waddle, Kohel and Lewis1984). Crop growth and development rates are directly related to temperature during the growing season and can be expressed in terms of either accumulated heat units or growing degree days (GDDs) (McMahon and Low, Reference McMahon and Low1972; Peng et al., Reference Peng, Krieg and Hicks1989; Roussopoulos et al., Reference Roussopoulos, Liakatas and Whittington1998; Reddy et al., Reference Reddy, Baker and Hodges1991, Reference Reddy, Reddy and Hodges1992). Due to the critical importance of heat and light resources, it is imperative to improve the use efficiency of these resources for sustainable development of crop production.
A solo crop can use only a small portion of available resources. In a double- or multi-cropping system, resource capture and use efficiency may dramatically increase in both time and space (Fukai and Trenbath Reference Fukai and Trenbath1993; Hook and Gascho, Reference Hook, Gascho and Hargrove1988; Trenbath, Reference Trenbath and Francis1986). Double- or multi-cropping systems can alter canopy architecture and canopy microclimate due to differences in planting date, growth period, and crop composition, and these differences among cropping systems result in varied distribution, capture, and use of heat and light resources (Allen et al., Reference Allen, Sinclair and Lemon1976). The Yellow River Valley is one of the three cotton production regions in China, and the cotton-wheat double-cropping system is currently one of the leading cropping systems in this region (CRI, 2013). The cotton-wheat double-cropping system not only guarantees food and nutritional security for the increasing population but also effectively stabilizes cotton acreage, improves farmers’ income, and has been recognized as a sustainable practice in the Yellow River Valley. In cotton-wheat double-cropping systems, cotton can be intercropped with wheat or transplanted or directly seeded into bare fields following wheat harvest (CRI, 2013). Double-cropping systems extend the period of soil coverage on an annual basis, which can lead to higher capture and use efficiency of solar radiation as well as more accumulated heat units than monocropping (Awal et al., Reference Awal, Koshi and Ikeda2006; Fukai and Trenbath Reference Fukai and Trenbath1993; Keating and Carberry, Reference Keating and Carberry1993; Tsubo et al., Reference Tsubo, Walker and Mukhala2001). Greater land use efficiency and radiation use efficiency (RUE) were previously achieved in a wheat-cotton intercropping system (Zhang et al., Reference Zhang, van der Werf, Zhang, Li and Spiertz2007, Reference Zhang, van der Werf, Zhang, Li and Spiertz2008 and Mao et al., Reference Mao, Zhang, Zhao, Liu, Van der, Zhang, Spiertz and Li2014). However, few studies have examined the light use efficiency of wheat-cotton sequential systems. In addition, the use efficiency of heat resources has not been well studied in wheat-cotton double-cropping systems.
Soil fertility is another important factor that controls crop productivity, especially under double- or multi-cropping systems (Kintchéa et al., Reference Kintchéa, Guibertc, Sogbedji, Levêque, Bonfoh and Tittonell2015). Soil fertility can mainly be indicated by the content of organic matter, available nitrogen, phosphorus, and potassium (Dong et al., Reference Dong, Kong, Li, Tang and Zhang2010b). The variability of soil fertility poses a major challenge for the efficient use of resources to increase crop productivity (Tittonell et al., Reference Tittonell, Vanlauwe, De Ridder and Giller2007, Wopereis et al., Reference Wopereis, Tamélokpo, Ezui, Gnakpénou, Fofana and Breman2006; Zingore et al., Reference Zingore, Murwira, Delve and Giller2007). However, how soil fertility impacts resource use efficiency in wheat-cotton double-cropping systems is still unclear.
In our previous publication (Feng et al., Reference Feng, Wang, Han, Li, Zhu, Zhou and Cao2017), we clarified why the yield difference occurred in different wheat-cotton cropping systems under different soil fertility regimes, and we pointed out that differences in growth periods of cotton contributed to yield variation in the different cropping systems. These differences in growth periods of cotton can lead to differences in light capture and heat resources, further affecting the heat and light use efficiency of cotton and the whole cropping system. Therefore, the specific objective of this study was to analyze the effects of cropping systems on the heat and light capture and use efficiency of cotton as well as the overall resource use efficiency of wheat-cotton double-cropping systems in fields differing in soil fertility. Such information would provide useful information to help cotton production achieve higher productivity and high resource use efficiency and would provide insight into yield differences among different cropping systems.
Materials and Methods
Experimental years and sites
A 2-year field study was carried out during 2012/2013 and 2013/2014 in a farmer’s field and at the research station of the Institute of Cotton Research of Chinese Academy of Agricultural Sciences. Both fields were located in Anyang, Henan, China (36°07′N, 116°22′E), and were 1 km away from each other. The soil in both fields was clay loam. To determine the soil nutrient status, soil samples were collected from each treatment plot and averaged across all plots in each field. For each plot, five sampling points were selected according to a zig-zag pattern. At each sampling point, two soil samples were taken: one from a cotton row and the other from a wheat/bare soil row. To test soil fertility, soil samples were taken at depths of 0–20 cm. A soil test was conducted each year prior to sowing and after harvesting the cotton. The testing results were reported in our previous publication (Feng et al., Reference Feng, Wang, Han, Li, Zhu, Zhou and Cao2017) and revealed higher soil organic matter, total N, and available P in the farmer’s field than in the research station field, although no difference in the available K content was observed between the two fields. For convenience, the farmer’s field was named ‘high fertility’ and the research station field was named ‘low fertility’ in this study. Daily meteorological data, including mean temperature, precipitation, and solar radiation, were recorded at 30-min intervals by using the weather station (AG1000; Campbell Scientific, Logan, UT, USA) located near the experimental field. A summary of the meteorological data is presented in Figure 1.

Figure 1. Weather conditions during both experimental years.
Experimental design and cropping systems
According to Ma (Reference Ma1990), several different factors can be combined and considered a single treatment when attempting to assess the comprehensive effects of different factors. In this study, four cropping patterns [cotton intercropped with wheat (CIW), cotton directly seeded after wheat (CDW), cotton transplanted after wheat (CTW), and cotton monoculture (CM)] and their respective cultivation practices were considered as four treatments and were applied to fields in a completely randomized block design with three replications in 2012/2013 and 2013/2014. Wheat in the double-cropping system was sown in October (the 26th in 2012 and the 27th in 2013) and harvested in June (the 10th in 2013 and the 12th in 2014). The winter wheat (Triticum aestivum L.) cultivar ZY-36 was used in this study. Based on the varied planting dates of the different cropping systems, the long-season cotton variety CRI-79 and the short-season cotton variety CRI-74, both developed by the Institute of Cotton Research of the Chinese Academy of Agricultural Sciences, were used in this study. CRI-79 was sown in CM and CIW in April (the 18th in 2013 and the 23rd in 2014). CRI-74 was transplanted in CTW (sown on May 10th in the greenhouse) and directly sown in the field in CDW on June 14th, 2013 and June 17th, 2014. The cotton growth stages in both years are shown in Table 1. The cotton was hand harvested and finished before wheat was sown (October 21st, 2013 and October 24th, 2014). The field experiment was managed according to local practices. In CM, cotton was sown in 70-cm-spaced rows. In CIW, two rows of cotton (70 cm row width for cotton) were relay intercropped with six rows of wheat (17.5 cm row width for wheat) with 70 cm between the wheat and cotton rows and a total strip width (wheat + cotton) of 210 cm. In CTW and CDW, winter wheat was sown with a row width of 17.5 cm, and cotton was planted after wheat with a row width of 70 cm. For a full description of the cropping system, see the study by Feng et al. (Reference Feng, Wang, Han, Li, Zhu, Zhou and Cao2017).
Table 1. Cotton growth periods in two fields differing in soil fertility in 2013 and 2014 under varying cropping system: cotton monoculture (CM), cotton intercropped with wheat (CIW), cotton transplanted after wheat (CTW), and cotton directly seeded after wheat (CDW)

* DAP means days after planting.
Land use efficiency
To assess the land use efficiency of different treatments, the land equivalent ratio (LER) was calculated according to the equation of Willey (Reference Willey1985):

where Y w,i, Y w,s, Y c,i, and Y c,s are the grain yields of intercropped wheat and wheat alone and the yields of intercropped cotton and cotton alone, respectively. Y w,s was estimated by using the wheat yield in CDW.
Leaf area index and aboveground biomass
One meter section of a row of wheat plants was sampled in each plot at 15-day intervals from March 20th, 2013 to June 3rd, 2013, and from April 1st, 2014 to June 6th, 2014. The leaves, stems, and spikes of the sampled plants were subsequently separated. Two randomly selected cotton plants from the central two rows of each plot were sampled at 15-day intervals from May 24th to September 24th in both years; the vegetative parts (roots, main stems and fruiting branches, leaves) and reproductive parts (squares, flowers, and bolls) of these plants were subsequently separated. Green leaves were immediately scanned by using a Phantom 9800xl scanner (Microtek, Shanghai, China), and the images were analyzed using Image-Pro Plus 7.0 (Media Cybernetics, Rockville, MD, USA) for leaf area, after which the leaf area index (LAI) was calculated. The dry weight of each part of the wheat and cotton was recorded as biomass after drying in an oven at 75 °C until constant weight.
Yield determination
An area of 4.2 m2 (2 × 2.1 m) of wheat was harvested from each plot for yield determination. The grain yields were determined assuming a water content of 12% in the sun-dried grains. At the beginning of October, cotton bolls from an area of 7 m2 (0.7 × 10 m) in the central rows of each plot were hand harvested to estimate cotton yield. Plant density, boll number per plant, and average boll weight were recorded for the calculation of cotton yield. The yield of the whole system was determined by summarizing the cotton yield and wheat yield.
Light interception
Photosynthetically active radiation (PAR) intercepted by the cotton canopy was collected from 10:00 to 11:00 a.m. on cloudless days using a quantum sensor (LI-191SA; LI-COR, Lincoln, NE, USA) and a light sensor data logger (LI-1400; LI-COR). For accurate measurements, the incident transmitted PAR (PARt) and reflected PAR (PARr) were measured horizontally and vertically according to Zhi et al. (Reference Zhi, Han, Mao, Wang, Feng, Yang, Fan, Du, Lu and Li2014) in all plots on the same dates on which the LAI was measured. The crop canopy was divided into several layers spaced 20 cm apart from the bottom to the top of the canopy. For each layer, PARt and PARr were measured every 17.5 cm from the western row toward the adjacent eastern row. Thus, we collected five measurement points at 0, 17.5, 35, 52.5, and 70 cm for each layer for the CM, CTW, and CDW. In the CIW, because the strip width was 210 cm, thirteen measurement points were established for each layer at 17.5 cm intervals. The incident PAR (PARI) at 20 cm above the canopy was measured simultaneously. During the wheat growing season, the measurements were taken from March 20th to June 3rd, 2013 and from April 1st, 2014 to June 6th, 2014. The transmitted PAR rate (T r), reflected PAR rate (R r), and intercepted PAR rate (I r) were then calculated according to the following equations:



where PARI is the incident PAR above the canopy (μmol m−2 s−1) and PARt and PARr are the incident transmitted PAR (μmol m−2 s−1) and reflected PAR (μmol m−2 s−1), respectively, at each grid position in the canopy. Using spatial interpolation and Kriging methods, the T r, R r, and I r for the other positions were estimated. The light interception (LI) of the canopy was computed according to the Simpson 3/8 rules of integration as follows:


where Ai refers to the light volume of a certain cross-sectional area; the coefficient vector is {1, 3, 3, 2, 3, 3, 2, …, 3, 3, 2, 1}; Δx and Δy are the vertical and horizontal distances of the grid, respectively; (i, j) are grid node numbers; G(i, j) is the Kriging interpolation point; and volume stands for the total LI of the canopy.
The daily fraction of LI (fPAR) was calculated using the methods of Charles-Edwards and Lawn (Reference Charles-Edwards and Lawn1984) as follows:

The LI of each day was determined by fitting polynomial functions between the measured fPAR interception and days after sowing of each crop (Caviglia et al., Reference Caviglia, Sadras and Andrade2004; Van Opstal et al., Reference Van Opstal, Caviglia and Melchiori2011). The cumulative intercepted PAR (IPAR) of the canopy during the crop growth period was the product of the daily fPAR and the incoming daily PAR (μmol m−2 s−1). The incoming daily PAR (μmol m−2 s−1) was calculated by multiplying the daily incoming solar radiation (μmol m−2 s−1) by 0.5 (Monteith and Unsworth, Reference Monteith and Unsworth1990). The IPAR of the whole system was the summation of the IPAR of both cotton and wheat.
Radiation use efficiency
The RUE (g MJ−1) represents the aboveground biomass production (ΔBiomass, g m−2) per unit light intercepted by the crop canopy (ΔIPAR, MJ m−2) for a certain period; it can be calculated with the following equation (George-Jaeggli et al., Reference George-Jaeggli, Jordan, Van Oosterom, Broad and Hammer2013):

To determine the RUE of the whole system, the ΔBiomass, which reflects the summation of the biomass of both cotton and wheat, and the ΔIPAR, which reflects the summation of the IPAR of both cotton and wheat, were calculated and used.
GDDs and thermal product efficiency
GDD (ºC) was calculated by taking the accumulated heat above the base temperature. The base temperatures were set as 12 and 0 °C for cotton and wheat, respectively. Thermal product efficiency (TPE, kg hm−2 °C−1) was determined by dividing the total aboveground biomass (ΔBiomass, kg hm−2) by the GDDs (ΔGDD, °C) during the crop growth period:

For the whole system on an annual basis, the TPE was calculated according to the following equation:

where i =1, 2, …n; n represents the total number of crops planted in the field on an annual basis and k is a constant equal to the number of days with crops grown in the field divided by the total days on an annual basis.
Solar energy use efficiency
Solar energy use efficiency (E u) was calculated by dividing the thermal energy stored in the biomass of the crop in a certain period by the total solar radiation received during the same period:

where H is the calorific power of 1 kg of biomass, which is 17.8 MJ kg−1 for cotton and wheat (Jiang et al., Reference Jiang, Tang and Ge1987; Xu et al., Reference Xu, Niu and Bian2007), ΔW (kg m−2) is the biomass increase during a certain period, and ΔQ (MJ m−2) is the solar radiation received during this period.
For the whole system on an annual basis, the Eu was calculated according to the following equation:

where i =1, 2, …n; n represents the total number of crops planted in the field on an annual basis and k is a constant equal to the number of days with crops grown in the field divided by the total days on an annual basis.
Product of thermal effectiveness and PAR
Product of thermal effectiveness and photosynthetically active radiation (PTP) was calculated as the product of the values of relative thermal effectiveness (RTE) and PAR according to Zhao et al. (Reference Zhao, Meng, Li, Chen, Xu, Wang and Zhou2012):

where RTE is the daily relative thermal effectiveness (Li et al., Reference Li, Chen, Zhang, Fu, Xing, Li, Qian, Li, Wang, Fan, Yan, Wang and Yang2015) and PAR is the daily photosynthetically active radiation. RTEi and the relative temperature effect (RTE(T)) were calculated using equations (14) and (15), respectively, as follows:


where T avg, T max, and T min refer to the mean daily average and the maximum and minimum air temperatures, respectively. T b, T o, and T c are the base, optimum, and ceiling temperatures for cotton development, which were set as 15, 30, and 35 °C, respectively, and for wheat development, which were 0, 20, and 30 °C, respectively.
The use efficiency of PTP (PTPU) was calculated by dividing the accumulated biomass (ΔBiomass) (g m−2) by the cumulative PTP (ΔPTP) for a defined period of time:

For the whole system on an annual basis, the PTPUwhole was calculated according to the following equation:

where i represents the number of crops planted in the field on an annual basis, n represents the total crops planted in the field on an annual basis, and k is a constant equal to the number of the days with crops in the field divided by the total days on an annual basis.
The RUE, GDD, TPE, Eu, and PTP were estimated from March 20 to June 3, 2013 and from April 1 to June 6, 2014 for the wheat, from April 18 (May 10 in CTW; June 14 in CDW) to the cotton boll opening stage in 2013 and from April 23 (May 10 in CTW; June 17 in CDW) to the cotton boll opening stage in 2014 for cotton, and from March 20 to the cotton boll opening stage in 2013 and from April 1 to the cotton boll opening stage in 2014 for the whole cropping systems.
Experimental design and statistical analysis
The four treatments, that is, CM, CIW, CTW, and CDW, were arranged in a randomized complete block design with three replications in both fields during 2012/2013 and 2013/2014. Weather data for both years of the experiment are given in Figure 1. Replications (rep) and rep × treatments (cropping systems) were considered random effects. Years and years × treatments (cropping systems) were also considered random effects. Cropping systems were considered fixed effects. Since no significant interactions were observed between year and treatment, the two years’ data were then pooled. The collected data were analyzed statistically by using the Proc MIXED procedure in accordance with Satterthwaite’s degrees of freedom in SAS 9.2 ( SAS Institute, Cary, NC, USA). The means were separated using the protected least significant difference test at the significance level of 0.05.
Results
Productivity and land use efficiency
Cropping systems significantly affected the yield and biomass of cotton, wheat, and the whole system across both years (Table 2). The lint yields and biomass in all double-cropping systems were substantially lower than those in CM. Compared to that of CM and averaged across both years, the lint yield of CIW, CTW, and CDW decreased by 16.2, 30.0, and 38.8% in the low-fertility field, respectively, and by 7.5, 22.4, and 22.7% in the high-fertility field, respectively. CTW and CDW had greater wheat yields and biomass than did CIW, with no significant difference observed between them. For the whole cropping system, cotton-wheat double cropping considerably increased crop productivity compared with CM. Among all double-cropping systems, CTW produced the highest yield and biomass across all treatments, followed by CDW and then CIW, with no significant difference observed between CTW and CDW. In addition, yield and biomass differences with respect to cotton and the whole system between CTW and CDW diminished in the high-fertility field.
Table 2. Yield, biomass, and land equivalent ratio (LER) in two fields differing in soil fertility under varying cropping system: cotton monoculture (CM), cotton intercropped with wheat (CIW), cotton transplanted after wheat (CTW), and cotton directly seeded after wheat (CDW)
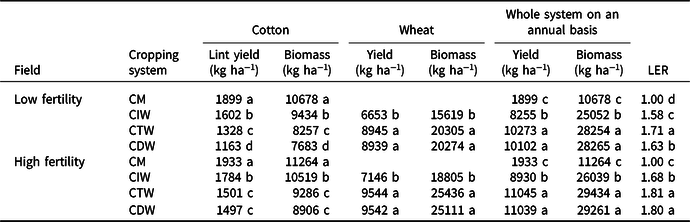
Data were pooled (2013 and 2014). The means followed by the same letter are not significantly different at p = 0.05.
Treatment affected land use efficiency (measured by the LER) in both fields (Table 2). The LER varied from 1.00 to 1.78, with double-cropping systems presenting markedly greater LER than that of CM in both fields. The LER of CIW was the lowest in all double-cropping systems in both fields. The LER of CTW was the highest in the low-fertility field, while no significant difference in LER was observed between CTW and CDW in the high-fertility field, which was due in part to the increased crop yield in CDW.
GDD and TPE
Cropping systems significantly affected the GDD by cotton and the whole system in both fields (Table 3). Among all cropping systems and averaged across 2 years, cotton in CIW and CTW accumulated the most GDD in both fields, followed by CM and then CDW. Double-cropping systems received more GDD compared with CM on an annual basis, especially in CIW and CTW, which received 71.8 and 70.9% more GDD, respectively, than did CM in the low-fertility field, and 72.4 and 70.7% more GDD, respectively, in the high-fertility field, when averaged across both years. There was no significant difference between CIW and CTW in GDD for cotton and on an annual basis.
Table 3. Growing degree days (GDD) and thermal production efficiency (TPE) of both cotton and whole system in two fields differing in soil fertility under varying cropping system: cotton monoculture (CM), cotton intercropped with wheat (CIW), cotton transplanted after wheat (CTW), and cotton directly seeded after wheat (CDW)

Data were pooled (2013 and 2014). The means followed by the same letter are not significantly different at p = 0.05.
Treatments also significantly impacted the TPE of cotton and the whole system (Table 3). Across 2 years, CTW had the lowest TPE with respect to cotton across all cropping systems, while CM had the highest in both fields. CIW and CDW did not differ in the TPE of cotton in the low-fertility field, whereas CDW had distinctly higher TPE than did CIW in the high-fertility field. Regarding the whole cropping system, CM had much lower TPE than did double-cropping systems; the highest TPE was observed in CDW, followed by CTW and CIW. These findings indicated that CDW produced more biomass per unit of GDD than did other cropping systems in both fields during both years. Moreover, the TPE values of crops and cropping systems in the high-fertility field were substantially greater than those in the low-fertility field.
LAI and fPAR of cotton
Cropping systems significantly influenced the LAI of cotton in both fields in both years (Figure 2). The LAI of cotton in the double-cropping systems was lower than that in CM. The peak values of the LAI of cotton occurred earlier (approximately 2 weeks) in CM and CIW than in CTW and CDW due to earlier planting dates. The differences in the LAI of cotton between treatments were greater at the earlier stage but then diminished. Across both years, the LAI peak values increased by 8.6, 12.5, 15.6, and 21.3% for CM, CIW, CTW, and CDW in the high-fertility field as compared with those in the low-fertility field, respectively. In addition, the initiation of LAI reduction was delayed in the high-fertility field in both years.

Figure 2. Leaf area index of cotton as affected by the cropping system and soil fertility in an experiment conducted in Anyang, Henan, in 2013 and 2014.
The course of cumulative fPAR for cotton in the different treatments in both years is shown in Figure 3. The course of fPAR of cotton showed an initial delay in all double-cropping systems, especially in CTW and CDW, compared with that of CM. The fPAR in cotton peaked first in CM, followed by CIW, CTW, and CDW, in both fields.

Figure 3. Fraction of intercepted photosynthetically active radiation by cotton as affected by cropping system and soil fertility in an experiment conducted in Anyang, Henan, in 2013 and 2014.
Accumulated PAR and Eu
The accumulated solar radiation of cotton and the whole cropping system was significantly influenced by treatments (Table 4). Across both years, cotton in CIW received the most accumulated PAR across all cropping systems, followed by CTW, and then CM in both fields. Cotton in CDW had the lowest accumulated PAR in all cropping systems. On an annual basis, double-cropping systems had much more accumulated PAR than CM. Across both years, CIW, CTW, and CDW received 123.3, 121.2, and 100.7% more accumulated PAR than CM in the low-fertility field and 125.1, 121.0, and 100.6% more accumulated PAR than CM in the high-fertility field, respectively.
Table 4. Accumulated solar radiation and solar energy use efficiency (Eu) in both cotton and whole system in two fields differing in soil fertility under varying cropping system: cotton monoculture (CM), cotton intercropped with wheat (CIW), cotton transplanted after wheat (CTW), and cotton directly seeded after wheat (CDW)

Data were pooled (2013 and 2014). The means followed by the same letter are not significantly different at p = 0.05.
Cropping systems significantly influenced the E u of crops and the whole system (Table 4). Across both years, CM exhibited higher E u of cotton compared with that of the double-cropping treatments in both fields. The E u of cotton was higher in CIW than in CTW but lower than in CDW in both fields. Regarding the whole cropping system, double-cropping systems had higher E u than did monoculture systems. Among the double-cropping systems, CDW showed the highest E u in both fields across both years. The E u in CIW was lower than that in CTW in the high-fertility field, and no significant difference in Eu between CIW and CTW was observed in the low-fertility field. The E u relative to cotton as well as the whole cropping system was greater in the high-fertility field than in the low-fertility field.
IPAR and RUE
Cotton in CM intercepted more PAR than did CIW when averaged across both years, followed by CTW (Table 5). The smallest IPAR was observed in CDW in both fields. Regarding the whole cropping system, the double-cropping systems received higher IPAR than did the monoculture system, with no obvious differences observed among CIW, CTW, and CDW.
Table 5. Intercepted photosynthetically active radiation (IPAR) and radiation use efficiency (RUE) of both cotton and whole system in two fields differing in soil fertility under varying cropping system: cotton monoculture (CM), cotton intercropped with wheat (CIW), cotton transplanted after wheat (CTW), and cotton directly seeded after wheat (CDW)

Data were pooled (2013 and 2014). The means followed by the same letter are not significantly different at p = 0.05.
Cropping system significantly influenced the RUE of cotton and cropping systems in both years (Table 5). The RUE of cotton in wheat-cotton double-cropping systems increased by 2.3–25.9% relative to that of CM across both fields. In addition, the RUE of cotton in CDW was distinctively higher than that in CTW, followed by that in CIW, in both fields. No obvious differences were observed between CM and CIW in the RUE of cotton in both fields. Likewise, the RUE of the whole system exhibited a similar trend across most treatments; however, an obvious difference was observed between CIW and CM in the RUE on an annual basis.
PTP and PTPU
Cumulative PTP is a comprehensive indicator of the acquisition of light and temperature and treatments significantly affected the PTP of cotton and whole system (Table 6). Cotton in CDW had the lowest PTP in both fields when averaged across both years. The PTP of cotton in CM was greater than that in CDW but lower than that in CIW and CTW in both fields across both years. The PTP of cotton in CIW was comparable to that of cotton in CTW in both fields. For the whole cropping system, the wheat-cotton double-cropping system had greater PTP than did CM. In all double-cropping treatments, CTW had the highest PTP (1180 in the low-fertility field and 1188 in the high-fertility field). There was no significant difference in PTP between CIW and CTW on an annual basis.
Table 6. Photothermal product (PTP) and PTP use efficiency (PTPU) of both cotton and whole system in two fields differing in soil fertility under varying cropping system: cotton monoculture (CM), cotton intercropped with wheat (CIW), cotton transplanted after wheat (CTW), and cotton directly seeded after wheat (CDW)

Data were pooled (2013 and 2014). The means followed by the same letter are not significantly different at p = 0.05.
The PTPU of cotton in CM was higher than that in the double-cropping systems in both fields across both years, while CTW showed the lowest PTPU in both fields (Table 6). CDW had distinctively higher PTPU of cotton than did CIW in both fields across both years. There was no significant difference between CM and CDW in the PTPU of cotton in the high-fertility field. However, the situation was somewhat reversed for the whole cropping system. Double-cropping systems showed significant advantages with respect to PTPU compared with monoculture systems. CDW resulted in the greatest PTPU across all treatments, followed by CIW and CTW. No significant differences in PTPU of the cropping system were observed between CTW and CIW.
The relationship between cotton yield and resource use efficiency
Relationships between seed cotton yield, biomass, capture, and the efficient use of resources were investigated in all treatments (Table 7). Lint yield and biomass were significantly and positively correlated with the TPE, IPAR, accumulated solar radiation, E u, PTP, and PTPU among the cropping systems (Table 7). Cotton yield, biomass, and boll number were not significantly correlated with GDD, but boll weight was positively correlated with GDD. RUE was not significantly related to any parameters investigated (seed cotton yield, biomass, and both yield components), indicating that RUE is not a limiting factor for cotton production.
Table 7. Correlation coefficients among seed cotton yield, biomass, growing degree days (GDD), accumulated solar radiation, intercepted photosynthetically active radiation (IPAR), photothermal product (PTP), thermal production efficiency (TPE), solar energy use efficiency (E u), radiation use efficiency (RUE), and PTP use efficiency (PTPU) across 2 years

*,†indicate significance of correlation at 0.05 and 0.01 levels, respectively.
Overall use efficiency of resources
The radar diagrams illustrate the overall use efficiency of resources (Figure 3), indicating the sustainability of the cropping system for the regions of the Yellow River Valley. The overall use efficiency of resources provided a way to compare the relative performance of cropping systems with respect to their use of resources. Regions with high resource use efficiencies are characterized by larger areas on the diagrams, and vice versa. In these diagrams, it is assumed that all resources are of equal importance to overall sustainability, without considering their specific contribution to sustainability (De Vries et al., Reference De Vries, Van de, Gerrie, Van Ittersum and Giller2011). Regarding the treatments, double-cropping systems showed obvious advantages compared to CM. CDW performed well in most of the efficiency criteria and was the best overall-performing cropping system in terms of efficiency in both fields. In addition, the overall use efficiency of resource was greater in the high-fertility field for all treatments than in the low-fertility field.
Discussion
Low resource use efficiency has become a major limiting factor for crop yield improvement, especially in China, which has a large population and limited arable land (Fan et al., Reference Fan, Shen, Yuan, Jiang, Chen, Davies and Zhang2011). Therefore, the development of farming systems that not only increase both crop productivity and economic benefits but also improve the ability to capture and use resources is critical. Light and heat are the most two important factors that influence crop yield. Therefore, improving the efficient use of light and heat is effective for increasing crop yield and land productivity. Herein, we found a substantially high LER in cotton-wheat double-cropping systems, although the solo crop yield was lower than that in CM (Table 2), which is consistent with previous studies (Zhang et al., Reference Zhang, van der Werf, Zhang, Li and Spiertz2008). Therefore, the wheat-cotton double-cropping system considerably increased land use efficiency, which is important for countries that have a large population, such as China.
Due to different growth environments (e.g., different planting dates) and canopy structure (e.g., LAI) resulting from different cropping systems, the use of light and heat varied among treatments. Among cropping systems, CDW had the smallest GDD and IPAR during the cotton growing period due to the short growth period. The IPAR of double-cropped cotton was smaller than that of CM, especially in CDW (Tables 3 and 5). This effect on the IPAR is due to the inadequate canopy closure (low LAI) and low fPAR during the growing season in double cropping (Figures 2, 3). The reasons for the differences in IPAR among the three double-cropping systems are different. These differences may be related to reduced leaf expansion due to the initial shading in intercropped cotton (Zhang et al., Reference Zhang, van der Werf, Zhang, Li and Spiertz2008), slow development of the canopy for late planting in transplanted cotton, and reduced incoming PAR due to delayed sowing in directly seeded cotton (Caviglia et al., Reference Caviglia, Sadras and Andrade2004). However, on an annual basis, double-cropping systems exhibited significantly higher capture of GDD and IPAR relative to that in CM. In addition, CIW and CTW showed relatively more GDD and IPAR than did CDW (Tables 3 and 5).
Resource capture is a component of resource use efficiency. In accordance with previous findings (Du et al., Reference Du, Chen, Shen, Zhang and Zhou2015), we found that wheat-cotton double cropping distinctly increased the use of both heat and radiation resources on an annual basis by increasing TPE and RUE. Among crop systems, CDW had the highest TPE and RUE, while CIW and CTW showed significantly higher GDD and IPAR values (Tables 3 and 5). There was an intergrowth period of cotton and wheat lasting more than 1 month in CIW that resulted in competition between cotton and wheat for natural resources. This competition delayed cotton seedling growth during the early growth stage, which resulted in a delayed increase of LAI; this delay was not compensated for in the end (Figure 2). The peak LAI of CIW decreased by 14.6% compared with that in CM (Figure 2). Similarly, there was a slow seedling growth period in CTW for seedlings recovering from transplanting. Therefore, the mutual competition between cotton and wheat in CIW and the slow seedling growth period caused by transplanting may have contributed to the relatively low resource use efficiency compared with that of CDW.
Moreover, cropping systems significantly affected cotton TPE and RUE, as double cropping had a higher RUE value than did CM. The RUE values reported for various cotton genotypes range from 1.18 to 2.00 g of dry matter (DM) MJ−1 (Gonias et al., Reference Gonias, Oosterhuis and Bibi2006; Milroy and Bange, Reference Milroy and Bange2003; Zhang et al., Reference Zhang, van der Werf, Zhang, Li and Spiertz2008; Yeates et al., Reference Yeates, Constable and McCumstie2010). These values are consistent with those of the present study, in which the RUE of cotton varied from 1.30 to 1.65 g DM MJ−1 among cropping systems (Table 5). In addition, because of better soil aeration and nutrient supply under high soil fertility, the light and heat use efficiency parameters were generally improved for both cotton and the whole system in the high-fertility field relative to those in the low-fertility field.
Cotton biomass and yield are strongly related to resource capture (Van der, Reference Van der, Zam-ski and Schaffer1996; Willey, Reference Willey1990). The correlation analysis also demonstrated that cotton yield and biomass productivity among cropping systems were positively related to IPAR, TPE, E u, and PTP but not to RUE (Table 6), which indicates that the key factor limiting double-cropped cotton production is less radiation capture rather than the RUE, which is consistent with previous reports (Du et al., Reference Du, Chen, Shen, Zhang and Zhou2015). In addition, based on the integrative evaluation, the double-cropping system showed great advantages in overall resource use efficiency compared with CM, especially CDW (Figure 4). Resource use efficiency was even greater in the high-fertility field than in the low-fertility field. These findings indicate that the use of the CDW cropping system should be expanded in the Yellow River Valley. Since the IPAR of cotton in CDW was the lowest among all treatments, which could be attributed to low LAI and fPAR, there is potential for further improvements in cotton yield through the use of agricultural practices and new varieties (Figures 2, 3). Agricultural practices including reasonably close planting, optimized irrigation and nutrient supply, and improved soil fertility may be effective in optimizing canopy structure and increasing IPAR. Because delayed planting dates resulted in immature cotton bolls late in the season, new cultivars with shorter growing seasons for either cotton or wheat could improve resource use efficiency. Therefore, the differences in resource capture and resource use efficiency might explain the differences in crop yield and productivity among the treatments.

Figure 4. Overall resource use efficiency (averaged across 2 years) of different cropping systems in fields with differing soil fertility.
Conclusion
Cropping systems significantly influenced the capture and use efficiency of resources of the studied crops. On an annual basis, wheat-cotton double cropping showed great advantages in heat and light use efficiency as a result of increased heat and light capture. The key factor limiting double-cropped cotton production was less radiation capture rather than the RUE. Further increases in cotton yield in wheat-cotton double cropping should be based on the improved capture of radiation by cotton. Due to its high heat and light resource use efficiency and its overall resource use efficiency combined with its high productivity and feasibility for mechanization, the cropping system of CDW should be expanded in the Yellow River Valley as the development of high-efficiency agriculture is needed for sustainable agricultural production. High soil fertility also plays an important role in improving light and heat use efficiency. As the IPAR of cotton in the cropping system of CDW was the lowest among all treatments, which may be attributed to low LAI and daily fraction of LI (fPAR), there is potential for improvement of LAI and daily fraction of LI (fPAR) through the use of agronomic practices including reasonably close planting density, optimized irrigation, and improved soil fertility as well as the application of new short-season varieties of either cotton or wheat.
Acknowledgements
This work was funded by the National Key Research and Development Program of China (2018YFD1000900).
Conflict of interest
The authors declare that they do not have any commercial or associative interest that represents a conflict of interest in connection with the work submitted.
Financial support
National Key Research and Development Program of China (2018YFD1000900).