1. Introduction
The Central Asian Orogenic Belt (CAOB) is one of the world’s largest orogens (Jahn et al. Reference Jahn, Windley, Natal’in and Dobretsov2004; Windley et al. Reference Windley, Alexeiev, Xiao, Kröner and Badarch2007; Xiao et al. Reference Xiao, Windley, Sun, Li, Huang, Han, Yuan, Sun and Chen2015). The CAOB was formed by the amalgamation of continental blocks, arcs and accretionary complexes during Palaeozoic time (Allen et al. Reference Allen, Windley and Zhang1993; Xiao et al. Reference Xiao, Zhang, Qin, Sun and Li2004; Charvet et al. Reference Charvet, Shu, Laurent-Charvet, Wang, Faure, Cluzel, Chen and De Jong2011). After final assembly, multi-stage tectonic movements (e.g. the convergence and closure of the Palaeotethys, Mesotethys, Neotethys and Mongol–Okhotsk oceans, and India–Eurasia collision) impacted the region during the Mesozoic and Cenozoic eras (Hendrix et al. Reference Hendrix, Dumitru and Graham1994; Bullen et al. Reference Bullen, Burbank, Garver and Abdrakhmatov2001; De Grave et al. Reference De Grave, Buslov and Van den Haute2007, Reference De Grave, Glorie, Ryabinin, Zhimulev, Buslov, Izmer, Elburg, Vanhaecke and Van den Haute2012; Jolivet et al. Reference Jolivet, Dominguez, Charreau, Chen, Li and Wang2010; Glorie et al. Reference Glorie, De Grave, Buslov, Zhimulev, Stockli, Batalev, Izmer, Haute, Vanhaecke and Elburg2011). This resulted in Mesozoic tectonic uplift, mainly in the Late Triassic and Late Jurassic to Cretaceous periods (Hendrix et al. Reference Hendrix, Graham, Carroll, Sobel, McKnight, Schulein and Wang1992; Dumitru et al. Reference Dumitru, Zhou, Chang, Graham, Hendrix, Sobel, Carroll, Hendrix and Davis2001; Glorie et al. Reference Glorie, De Grave, Buslov, Elburg, Stockli, Gerdes and Van den Haute2010; De Grave et al. Reference De Grave, Glorie, Ryabinin, Zhimulev, Buslov, Izmer, Elburg, Vanhaecke and Van den Haute2012; Glorie & De Grave, Reference Glorie and De Grave2016). Cenozoic tectonic activity was mainly induced by India–Eurasia collision during Oligocene and early Miocene times (Molnar & Tapponnier, Reference Molnar and Tapponnier1975; Windley et al. Reference Windley, Allen, Zhang, Zhao and Wang1990; Hendrix et al. Reference Hendrix, Dumitru and Graham1994; Sobel & Dumitru, Reference Sobel and Dumitru1997; Yin et al. Reference Yin, Nie, Craig, Harrison, Ryerson, Qian and Geng1998; Dumitru et al. Reference Dumitru, Zhou, Chang, Graham, Hendrix, Sobel, Carroll, Hendrix and Davis2001; Sobel et al. Reference Sobel, Chen and Heermance2006; Glorie et al. Reference Glorie, De Grave, Buslov, Zhimulev, Stockli, Batalev, Izmer, Haute, Vanhaecke and Elburg2011; De Grave et al. Reference De Grave, Glorie, Buslov, Stockli, McWilliamsn, Batalev and Van den Haute2013; Macaulay et al. Reference Macaulay, Sobel, Mikolaichuk, Landgraf, Kohn and Stuart2013, Reference Macaulay, Sobel, Mikolaichuk, Kohn and Stuart2014). The India–Eurasia collision reactivated pre-existing structures in the Tianshan, which is recorded by young (<50 Ma) low-temperature thermochronology ages along major structures (i.e. the South and North Tianshan Suture zone, Talas–Fergana fault; Jolivet et al. Reference Jolivet, Dominguez, Charreau, Chen, Li and Wang2010; Glorie et al. Reference Glorie, De Grave, Buslov, Elburg, Stockli, Gerdes and Van den Haute2010, Reference Glorie, De Grave, Buslov, Zhimulev, Stockli, Batalev, Izmer, Haute, Vanhaecke and Elburg2011, Reference Glorie, De Grave, Buslov, Zhimulev, Elburg and Van den Haute2012; De Grave et al. Reference De Grave, Glorie, Ryabinin, Zhimulev, Buslov, Izmer, Elburg, Vanhaecke and Van den Haute2012, Reference De Grave, Glorie, Buslov, Stockli, McWilliamsn, Batalev and Van den Haute2013; Bande et al. Reference Bande, Radjabov, Sobel, Sim, Brunet, McCann and Sobel2017; Jepson et al. Reference Jepson, Glorie, Konopelko, Mirkamalov, Danišík and Collins2018 b).
The Tianshan Mountains extend E–W along the southwestern margin of the CAOB. The Tianshan is usually divided into the North, Central and South Tianshan by the Main Tianshan Shear Zone (Laurent-Charvet et al. Reference Laurent-Charvet, Charvet, Monié and Shu2003; locally named the Aqikkuduk Fault in this study; Shu et al. Reference Shu, Charvet, Lu and Ma1998, Reference Shu, Jacques, Guo, Lu and Sebastien1999) and Atbashy–Inychek–South Nalati–Qawabulak Fault (Xiao et al. Reference Xiao, Zhang, Qin, Sun and Li2004, Reference Xiao, Windley, Allen and Han2013). Geographically, the Tianshan in China is divided into the Eastern Tianshan and Western Tianshan along the 88° E longitude line (Li et al. Reference Li, Wang, Li, Sun, Chu, Li and Zhu2006). The Jueluotage area, which is a southern branch of the Eastern Tianshan, is located south of the Tuha Basin and northeast of the Tarim Basin. As the Jueluotage area comprises multiple accreted arcs and major faults, and is located at the southern margin of the CAOB, it retains a record of the intracontinental reactivation induced by the tectonic events at the Eurasian plate margin after amalgamation (e.g. Molnar & Tapponnier, Reference Molnar and Tapponnier1975; De Grave et al. Reference De Grave, Buslov and Van den Haute2007; Jolivet et al. Reference Jolivet, Dominguez, Charreau, Chen, Li and Wang2010; Glorie et al. Reference Glorie, De Grave, Buslov, Zhimulev, Stockli, Batalev, Izmer, Haute, Vanhaecke and Elburg2011, Reference Glorie, Otasevic, Gillespie, Jepson, Danišík, Zhimulev, Gurevich, Zhang, Song and Xiao2019; Gillespie et al. Reference Gillespie, Glorie, Jepson, Zhang, Xiao, Danišík and Collins2017, Reference Gillespie, Glorie, Jepson, Xiao and Collins2020; Jepson et al. Reference Jepson, Glorie, Konopelko, Gillespie, Danišík, Evans, Mamadjanov and Collins2018 a). Previous thermochronology studies in the Jueluotage area were mainly concentrated in the Aqishan–Yamansu belt (Guo et al. Reference Guo, Zhang, Liao and Fang2002; Gao et al. Reference Gao, Liu, He, Tian and Che2014; Liu et al. Reference Liu, Yan, He, Chen, Gao, Suo, Wang, Zhang, Cao, Wang and Zhao2014; Sun et al. Reference Sun, Sun, Chen, Yu, Yin, Li, Zhang and Liu2015). However, a systematic thermochronological study of different tectonic belts, and particularly of major fault zones (the Kanggurtag ductile shear zone (KDSZ) and the Aqikkuduk Fault), have not previously been undertaken.
In the northern part of the Eastern Tianshan (Tuha Basin and Karlik Shan), Cenozoic structures, which are attributed to deformation in response to the India–Asia collision, were observed (Hendrix et al. Reference Hendrix, Graham, Carroll, Sobel, McKnight, Schulein and Wang1992; Shao et al. Reference Shao, Stattegger, Li and Haupt1999; Zhu et al. Reference Zhu, Ma, Hu and Xu2001; Cunningham et al. Reference Cunningham, Owen, Snee and Li2003). However, disagreement on whether Cenozoic reactivation of the Eastern Tianshan can be constrained by thermochronological ages persists (Wang et al. Reference Wang, Li, Zhang, Liu and Ma2008; Gillespie et al. Reference Gillespie, Glorie, Jepson, Zhang, Xiao, Danišík and Collins2017). As the major fault zones are more likely to record Cenozoic activity in the Tianshan Mountains, a thermochronological study of major fault zones (i.e. the Aqikkuduk Fault) in the Jueluotage area can shed light on the nature and timing of Cenozoic reactivation in the Eastern Tianshan.
This study aims to reveal the low-temperature cooling history of the Jueluotage area and help in the construction of a more complete Mesozoic tectonic evolution of the Eastern Tianshan. Zircon (U–Th)/He (ZHe), apatite (U–Th)/He (AHe) and apatite fission-track (AFT) dating and associated thermal history models were obtained from 13 samples in order to constrain the 180–40 °C thermal history (Wolf et al. Reference Wolf, Farley and Kass1998; Farley, Reference Farley2000; Reiners et al. Reference Reiners, Spell, Nicolescu and Zanetti2004).
2. Geological setting
The research area is located in the Jueluotage area, south of the Turfan–Hami Basin and northeast of the Tarim Basin (Fig. 1). From north to south, the study area comprises the Dananhu arc, KDSZ, Aqishan–Yamansu belt and Central Tianshan arc (Fig. 2). These tectonic belts are separated by three major faults: the Kanggur Fault, Yamansu Fault and Aqikkuduk Fault (Fig. 2).

Fig. 1. (a) Map of Eastern Asia showing the location of Figure 1b in NW China. (b) Regional topography of NW China showing the main structures in the Eastern Tianshan (modified after Cai et al. Reference Cai, Xu, He and Wang2012) and the altitude difference between the Eastern Tianshan and other parts of the Tianshan. AFT – apatite fission-track; AHe – apatite (U–Th)/He. Published AFT ages from the Tianshan and surrounding areas are indicated by coloured dots. Stars are AFT ages from this study. Squares are AHe ages from this study. Data are from Dumitru et al. (Reference Dumitru, Zhou, Chang, Graham, Hendrix, Sobel, Carroll, Hendrix and Davis2001), Guo et al. (Reference Guo, Zhang, Liao and Fang2002), Zhu et al. (Reference Zhu, Wan, Shu, Sun and Wang2004, Reference Zhu, Shu, Wan, Sun, Wang and Zhao2006), Shen et al. (Reference Shen, Mei, Zhang, Liu, Tang, Zhou, Yan and Luo2008), Wang et al. (Reference Wang, Li, Zhang, Liu and Ma2008), Zhang et al. (Reference Zhang, Zhu, Shu, Wan, Yang, Su and Zheng2009, Reference Zhang, Zhu, Shu, Wan, Yang, Zheng and Su2011), Jolivet et al. (Reference Jolivet, Dominguez, Charreau, Chen, Li and Wang2010), Lü et al. (Reference Lü, Chang, Wang and Zhou2013), Gao et al. (Reference Gao, Liu, He, Tian and Che2014), Liu et al. (Reference Liu, Yan, He, Chen, Gao, Suo, Wang, Zhang, Cao, Wang and Zhao2014), Tang et al. (Reference Tang, Zhang, Li, Li, Luo and Chen2015), Gillespie et al. (Reference Gillespie, Glorie, Jepson, Zhang, Xiao, Danišík and Collins2016, Reference Gillespie, Glorie, Jepson, Zhang, Xiao, Danišík and Collins2017, Reference Gillespie, Glorie, Jepson, Xiao and Collins2020) Yin et al. (Reference Yin, Chen, Hodges, Xiao, Cai, Yuan, Sun, Liu and van Soest2017), Wang et al. (Reference Wang, Cai, Sun, Xiao, De Grave, Wan and Bao2018), Glorie et al. (Reference Glorie, Otasevic, Gillespie, Jepson, Danišík, Zhimulev, Gurevich, Zhang, Song and Xiao2019) and Zhang et al. (Reference Zhang, Chen, Liu, Yin and Sun2020). The study area is shown in the red rectangle and is expanded in Figure 2. STSF – South Tianshan Fault; STS – South Tianshan; CTSF – Central Tianshan Fault; CTS – Central Tianshan; Nalati F – Nalati Fault; NTSF – North Tianshan Fault.

Fig. 2. Geological map of the study area (modified after Wang et al. Reference Wang, Wang and He2006). Samples from this study are indicated with the corresponding ZHe, AHe and AFT ages. ZHe – zircon (U–Th)/He; AHe – apatite (U–Th)/He; AFT – apatite fission-track. Ages of granites are labelled in blue. Ages of granites are from Wu et al. (Reference Wu, Zhang, Zaw, Della-Pasque, Tang, Zheng, Wang and San2006) and Zhou et al. (Reference Zhou, Yuan, Zhang, Fan, Liu, Peng and Zhang2010). See Tables 2 and 3 for detailed data.
The Dananhu arc is mainly made up of Ordovician to Devonian–Carboniferous intermediate–acid volcanic, pyroclastic and intrusive rocks (Song et al. Reference Song, Li, Li, Wang and Wang2002; Qin et al. Reference Qin, Sun, Li, Fang, Wang and Liu2010). It is thought to have been formed by N-dipping subduction of the North Tianshan Ocean, which was a branch of the Palaeo-Asian Ocean and located between the Junggar–Tuha block and Central Tianshan block (Xiao et al. Reference Xiao, Zhang, Qin, Sun and Li2004; Wang et al. Reference Wang, Zhang, Feng, Liao, Zhang, Kang, Guo, Xuan and Han2019). The collision between the Dananhu arc and the Yamansu–Central Tianshan arc occurred in latest Carboniferous to early Permian time (Xiao et al. Reference Xiao, Zhang, Qin, Sun and Li2004; Zhang et al. Reference Zhang, Wei, Li, Du and Chai2010). However, recent research constrained the closure of the North Tianshan Ocean to the latest Permian – Early Triassic (Chen, Z et al. Reference Chen, Xiao, Windley, Schulmann, Mao, Zhang, Zhang, Li and Song2020), or even later in the Middle–Late Triassic (Ao et al. Reference Ao, Qigui, Windley, Song, Zhang, Zhang, Wan, Han and Xiao2021).
The KDSZ, separated from the Dananhu arc by the Kanggur Fault in the north (Fig. 2), is composed of Devonian–Carboniferous volcano-sedimentary rocks, as well as greywacke and turbidite. Ophiolitic slices were also found in this belt (Li et al. Reference Li, Dong and Zhou2000). The Kanggurtag tectonic belt comprises accretionary complexes that developed during bipolar subduction of the North Tianshan Ocean beneath the Dananhu arc in the north (Xiao et al. Reference Xiao, Zhang, Qin, Sun and Li2004) and the Central Tianshan arc in the south (Chen et al. Reference Chen, Xiao, Windley, Schulmann, Mao, Zhang, Zhang, Deng and Song2019). This belt contains an E–W-trending and N-dipping ductile shear zone, which was characterized by strike-slip shear deformation during late Permian to Triassic times (Chen et al. Reference Chen, Sun, Zhang, Xiao, Wang, Wang, Jiang and Yang2005, Reference Chen, Zhang, Qin, Wang, Wang and Liu2007).
The Aqishan–Yamansu belt, separated from the KDSZ by the Yamansu Fault (Fig. 2), is characterized by Carboniferous volcanic and plutonic rocks (Wu et al. Reference Wu, Zhang, Zaw, Della-Pasque, Tang, Zheng, Wang and San2006; Zhou et al. Reference Zhou, Yuan, Zhang, Fan, Liu, Peng and Zhang2010). Sporadic Lower–Middle Jurassic sandstones unconformably overlie upper Carboniferous pyroclastic rocks and Carboniferous granites in the south of the Aqishan–Yamansu belt (BGMRXUAR, 1966), indicating that the granites were already exposed at the surface by Jurassic time. Various tectonic settings for the Aqishan–Yamansu belt have been proposed: (1) it was formed on the fore-arc accretionary prism of the Dananhu arc during the northward subduction of the North Tianshan Ocean (Xiao et al. Reference Xiao, Zhang, Qin, Sun and Li2004); (2) it was formed by the southward subduction of the North Tianshan Ocean beneath the Central Tianshan arc (Wu et al. Reference Wu, Zhang, Zaw, Della-Pasque, Tang, Zheng, Wang and San2006; Luo et al. Reference Luo, Liao, Zhang, Chen, Wang and Huang2016; Han & Zhao, Reference Han and Zhao2018; Zhang et al. Reference Zhang, Sun, Yuan, Long, Jiang, Li, Huang and Du2018; Zhao et al. Reference Zhao, Chen, Zhang, Zhang, Yang and Yan2018); (3) a rift formed during early Carboniferous time resulting in the Aqishan–Yamansu belt formation (Qin et al. Reference Qin, Fang, Wang, Zhu, Feng, Yu and Xiu2002; Wang et al. Reference Wang, Wang and He2006). Regardless of the precise mechanism, widespread late Carboniferous granitoids are observed.
The Central Tianshan arc is located south of the Aqikkuduk Fault and is separated from the South Tianshan by the Kawabulak Fault to the south (Fig. 2). It is composed of calc-alkaline-type volcanic rocks, I-type granitoids and Precambrian basement rocks (Hu et al. Reference Hu, Jahn, Zhang, Chen and Zhang2000; Xiao et al. Reference Xiao, Zhang, Qin, Sun and Li2004). The Central Tianshan arc was regarded as having formed by northward subduction of the South Tianshan oceanic plate at the southern margin (Allen et al. Reference Allen, Windley and Zhang1993; Gao et al. Reference Gao, Li, Xiao, Tang and He1998; Xiao et al. Reference Xiao, Zhang, Qin, Sun and Li2004); however, other researchers have hypothesized formation via southward subduction of the North Tianshan oceanic plate (Chen, Z et al. Reference Chen, Xiao, Windley, Schulmann, Mao, Zhang, Zhang, Li and Song2020; Zhang et al. Reference Zhang, Zhao, Eizenhöfer, Sun, Han, Hou, Liu, Wang, Liu and Xu2015 a,b, Reference Zhang, Zhao, Eizenhöfer, Sun, Han, Hou, Liu, Wang, Liu, Xu and Zhu2016).
The Aqikkuduk Fault, located in the north of the Central Tianshan arc (Fig. 2), was regarded as the demarcation line of the Central Tianshan and North Tianshan (Shu et al. Reference Shu, Jacques, Guo, Lu and Sebastien1999; Laurent-Charvet et al. Reference Laurent-Charvet, Charvet, Monié and Shu2003). This fault was formed during late Silurian time (Shu et al. Reference Shu, Charvet, Lu and Ma1998, Reference Shu, Jacques, Guo, Lu and Sebastien1999), and reactivated as dextral strike-slip in the Triassic period owing to the collision between the Junggar–Tuha and Central Tianshan blocks (Cai et al. Reference Cai, Xu, He and Wang2012). The Aqikkuduk Fault was also reactivated after Pliocene time as evidenced by cross-cutting Pliocene sedimentary rocks (Gao et al. Reference Gao, He, Li and Huang2010).
In addition to the record of activity along the Aqikkuduk Fault, Cenozoic fault activity has also been found within or near the study area. Between the Jueluotage Mountain and the Tuha Basin, Jurassic strata were folded and thrust above Cenozoic strata in the Tuokexun area (Zhu et al. Reference Zhu, Ma, Hu and Xu2001). The Kanggur Fault was formed in early Carboniferous time and cuts Cenozoic strata, indicating that it was active during Cenozoic time (BGMRXUAR, 1988).
Several studies have used low-temperature thermochronology to explore the exhumation processes in or near the study area. Previous work mainly targeted the Aqishan–Yamansu belt, and yielded AFT and AHe ages ranging from Late Cretaceous to early Cenozoic (Guo et al. Reference Guo, Zhang, Liao and Fang2002; Liu et al. Reference Liu, Yan, He, Chen, Gao, Suo, Wang, Zhang, Cao, Wang and Zhao2014; Sun et al. Reference Sun, Sun, Chen, Yu, Yin, Li, Zhang and Liu2015). AFT and (U–Th)/He dating in the Yandong porphyry Cu deposit, located in the Dananhu arc (Fig. 2), revealed rapid early Carboniferous cooling (Yin et al. Reference Yin, Chen, Thomson, Sun, Wang, Xiao, Yuan, Sun and Long2019), with additional cooling in the late Permian to Triassic and Middle Jurassic to Early Cretaceous periods (Gong et al. Reference Gong, Kohn, Zhang, Xiao, Wu and Chen2021). In the Tuha Basin, located in the north of the study area, AFT ages obtained from Jurassic and Cretaceous sandstones ranged from 190 Ma to 79 Ma, and revealed a Cretaceous fast cooling stage (Zhu et al. Reference Zhu, Wan, Shu, Sun and Wang2004). AFT dating yielded Oligocene–Miocene ages in the western part of Bogda–Harlik Mountain (Shen et al. Reference Shen, Mei, Liu, Tang and Zhou2006, Reference Shen, Mei, Zhang, Liu, Tang, Zhou, Yan and Luo2008; Wang et al. Reference Wang, Li, Zhang, Liu and Ma2008) and Miocene ages in the east (Wang et al. Reference Wang, Li, Zhang, Liu and Ma2008). However, in the eastern segment of Bogda–Harlik Mountain, Gillespie et al. (Reference Gillespie, Glorie, Jepson, Zhang, Xiao, Danišík and Collins2017) found two stages of Mesozoic cooling (Early to Middle Triassic and Late Cretaceous), and no Cenozoic low-temperature thermochronological ages were found owing to the insufficient exhumation.
3. Samples and methods
Thirteen samples were collected from different tectonic belts. Four samples were collected inside the KDSZ. Three are intensely deformed (DTS1327 and DTS1359, mylonite; DTS1371, mylonitized granite) and the other (DTS1326) is undeformed granite porphyry. Five samples were taken in the Aqishan–Yamansu belt. All are undeformed (DTS1159 and DTS1337, granite; DTS1336 and DTS1341, biotite granite; J52, biotite hornblendite) and intruded during Carboniferous time (Zhou et al. Reference Zhou, Yuan, Zhang, Fan, Liu, Peng and Zhang2010). Two samples (DTS1308 and DTS1378, gneiss) were collected inside the Aqikkuduk Fault and one (DTS1306, granite) on the north edge of the fault. Another sample (J58, granite) was collected in the Central Tianshan arc. Sample locations are marked in Figure 2 and detailed information is provided in Table 1.
Table 1. Information for samples in this study

KDSZ – Kanggurtag ductile shear zone.
Apatite and zircon (U–Th)/He analyses were carried out at the Institute of Geology, Chinese Academy of Geological Sciences (IGCAGS). AFT dating was performed at the Beijing Quick-Thermo Science & Technology Co., Ltd. Thermal history modelling was conducted using HeFTy (Ketcham, Reference Ketcham2005). The detailed analytical methods are described in the online Supplementary Material.
4. Results and interpretation
ZHe and AHe dating results are shown in Table 2. Criteria for determining ZHe and AHe age outliers are described in the online Supplementary Material. AFT dating results are shown in Table 3.
Table 2. Zircon and apatite (U–Th)/He results for collected samples in the Jueluotage area

re – re-analysed apatite by applying zircon dissolution procedure; ** anomalously old (U–Th)/He ages ((U–Th)/He age older than the emplacement age or AHe reversely older than ZHe) and treated as outliers; # outliers having abnormally large concentration of U or Th relative to other grains of the same sample; * datum identified as an outlier using the Hampel identifier methods with an outer limit of four.
Table 3. Apatite fission-track ages summary

Ns – number of spontaneous tracks counted; ρs – spontaneous track density; 238U – average uranium concentration by laser ablation inductively coupled plasma mass spectrometry (Hasebe et al. Reference Hasebe, Barbarand, Jarvis, Carter and Hurford2004); P(χ2) – chi-square probability that all single-crystal ages represent a single population of ages where degrees of freedom = Nc − 1 (Galbraith, Reference Galbraith1981); NL – number of confined track lengths (TINTs) measured; MTL – mean (confined) track length; SE – standard error; SD – standard deviation of the track length distribution; Dpar – mean track etch pit diameter parallel to the crystallographic c-axis (Donelick, Reference Donelick1993).
4.a. Zircon (U–Th)/He ages
Ten samples were analysed by zircon (U–Th)/He dating (Fig. 2; Table 2). Two samples (DTS1326 and DTS1371) in the KDSZ yielded average ages of 253.7 ± 16.4 Ma and 233.5 ± 15.0 Ma, respectively. Samples DTS1159, DTS1336, DTS1337 and J52 were collected in the Aqishan–Yamansu belt. DTS1159 and DTS1337 yielded average ages of 210.8 ± 14.7 Ma and 241.9 ± 15.4 Ma after excluding one outlier for each sample. The average ages of DTS1336 and J52 are 230.8 ± 14.8 Ma and 244.2 ± 14.5 Ma, respectively. DTS1306 was collected on the north edge of the Aqikkuduk Fault and yielded an average age of 218.0 ± 13.0 Ma from grains DTS1306-1 and DTS1306-3. Two gneiss samples (DTS1308 and DTS1378) collected from the Aqikkuduk Fault yielded average ages of 211.9 ± 13.9 Ma and 237.5 ± 15.5 Ma, respectively. One sample (J58) was collected in the Central Tianshan arc and yielded an average age of 217.1 ± 13.1 Ma.
4.b. Apatite (U–Th)/He ages
Twelve samples were analysed by the AHe method. As the AHe ages of DTS1326 and DTS1378 were older than their corresponding ZHe ages (Table 2), they were completely dissolved using zircon dissolution methods in order to fully release parent isotopes held in refractory undetected inclusions (Vermeesch et al. Reference Vermeesch, Seward, Latkoczy, Wipf, Günther and Baur2007).
Three samples (DTS1326, DTS1327 and DTS1359) taken from the KDSZ were AHe dated. After excluding outliers, three grains from DTS1326 and DTS1327 yielded average ages of 145.9 ± 9.2 Ma and 149.5 ± 9.3 Ma, respectively. No outliers were excluded from DTS1359, yielding an average age of 135.1 ± 9.0 Ma.
Five samples collected from the Aqishan–Yamansu belt were analysed by AHe dating. DTS1159 yielded an average age of 84.3 ± 5.6 Ma after excluding an outliner (DTS1159-3). Five grains from DTS1336 yielded an average age of 75.0 ± 4.4 Ma. Only two grains of DTS1337 were left after excluding outliers, and gave an average age of 82.1 ± 5.6 Ma. One grain (DTS1341-4) of DTS1341 was identified as an outlier, and the rest of the grains gave an average age of 87.8 ± 5.8 Ma. After excluding an outlier (J52-3), J52 yielded an average age of 76.2 ± 5.0 Ma.
DTS1306 was collected on the north edge of the Aqikkuduk Fault and yielded an average age of 134.8 ± 8.8 Ma. DTS1308 and DTS1378 were collected in the Aqikkuduk Fault. Three grains were analysed for both, and the average ages of 135.9 ± 8.7 Ma for DTS1308 and 143.0 ± 8.7 Ma for DTS1378 were obtained.
Sample J58 was taken from the Central Tianshan arc and yielded an average age of 146.9 ± 8.7 Ma.
4.c. Apatite fission-track data
The sample DTS1336 yielded a central AFT age of 188.6 ± 6.8 Ma. The average track length with standard deviation is 12.3 ± 0.2 μm (n = 113). Sample DTS1341 yielded a central AFT age of 164.0 ± 11.0 Ma. However, track length data were not acquired owing to the low concentration of 238U (2.93 ppm to 10.8 ppm in 20 grains, with a mean value of 7.10 ppm). Both samples passed the χ2 test (P (χ2) >5 %) suggesting the single grain ages form a single population. Detailed AFT results are presented in Table 3 and Figure 3.

Fig. 3. (a, c) Radial plot of AFT ages for sample DTS1336 and DTS1341 constructed by the RadialPlotter software (Vermeesch, Reference Vermeesch2009). The Dpar values are indicated by a red/yellow colour scale. (b) Frequency plot depicting distribution of confined fission tracks for sample DTS1336. In the track length histograms, MTL is the mean track length, SD is the standard deviation, and n is the number of spontaneous tracks counted.
4.d. Thermal history modelling
The modelling results are shown in Figures 4 and 5. The model for sample DTS1326 shows cooling between ∼250 Ma and 200 Ma through 180 °C to 60 °C, yielding a rate of 2.4 °C Ma−1. An AHe thermal model was performed for sample DTS1327 and DTS1359. Both show cooling from 80 °C to ∼50 °C between 190 Ma and 170 Ma. The models for samples DTS1306, DTS1378 and J58 were based on ZHe and AHe ages, and revealed cooling from 180 °C to 60 °C between ∼240 Ma and 180 Ma, with a rate of 2 °C Ma−1. The models for DTS1308 and DTS1371 were set to start from the muscovite Ar–Ar closure temperature. Based on ZHe and AHe ages, DTS1308 shows a cooling phase from 180 °C to 80 °C between 230 Ma and ∼180 Ma, with a rate of 2 °C Ma−1. DTS1371 was modelled based on ZHe ages, and shows cooling from 180 °C to 80 °C between ∼240 Ma and 200 Ma, with a rate of 2.5 °C Ma−1. After the fast cooling, almost all these samples show relatively slow cooling to ∼30 °C from Early Jurassic time to ∼120 Ma and a much slower cooling to surface temperature from ∼120 Ma to the present day.
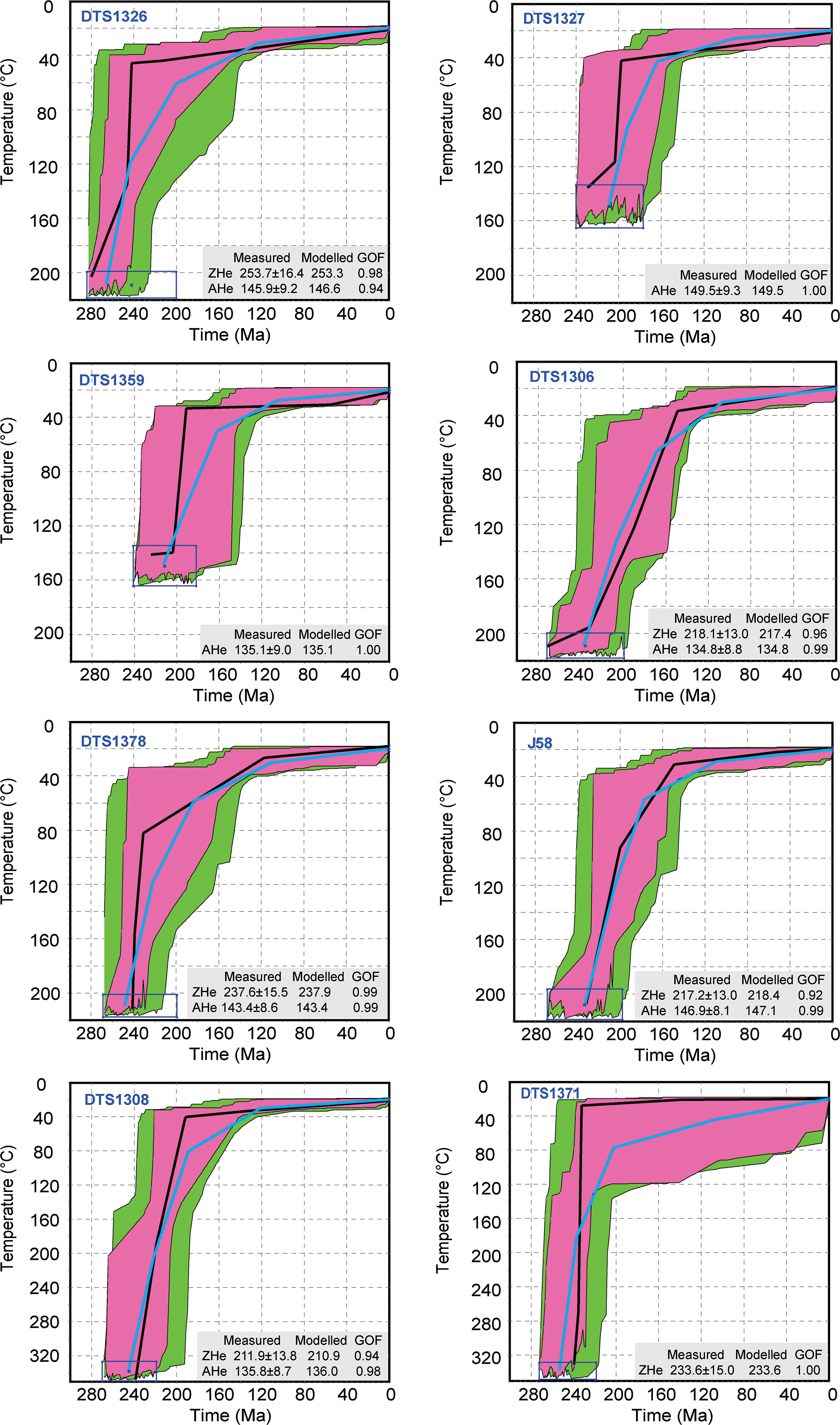
Fig. 4. The modelled thermal histories of samples from the KDSZ, Aqikkuduk Fault and CTS. The best path and weighted average path of all good and acceptable paths are shown as black and light blue curves, respectively.
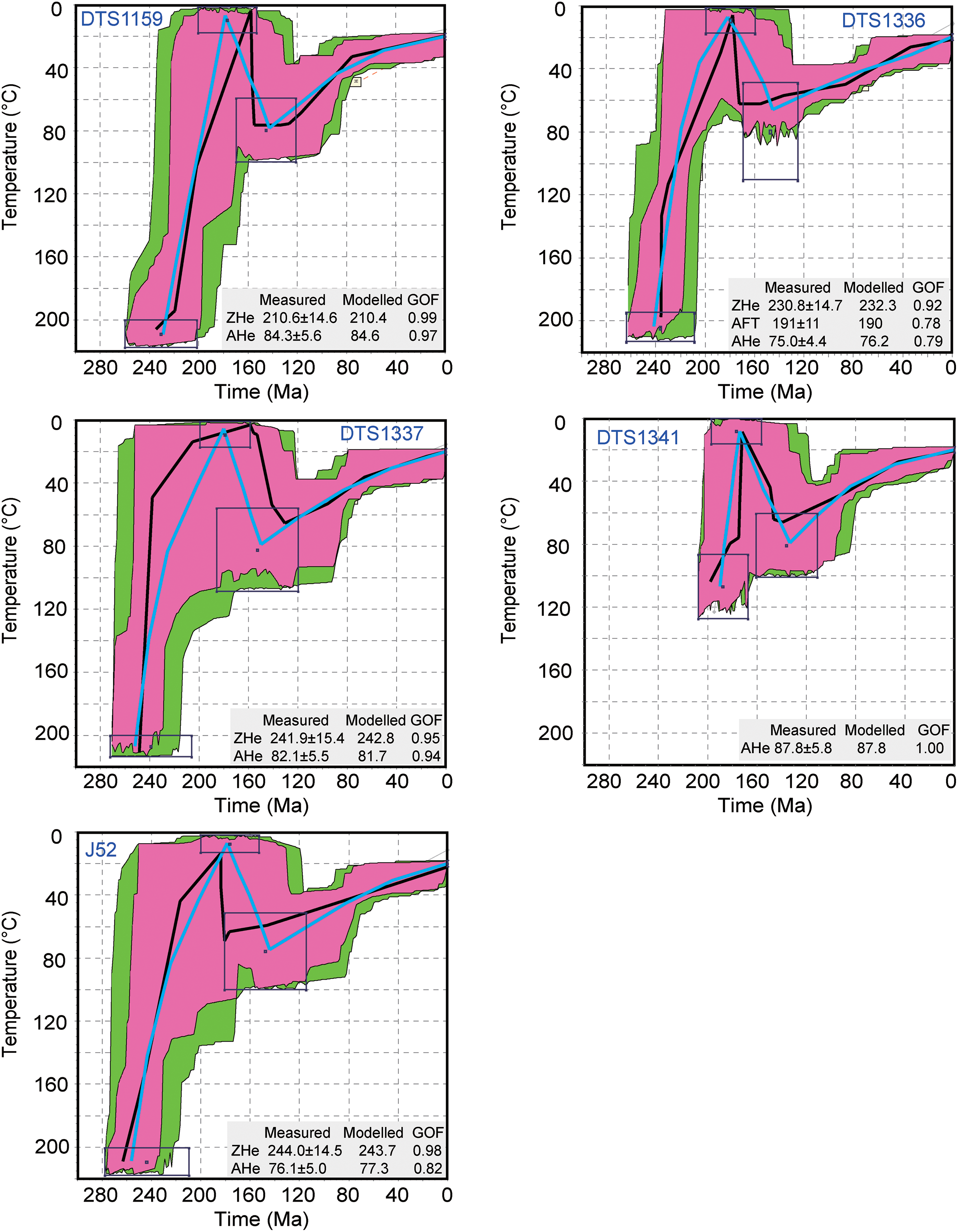
Fig. 5. The modelled thermal histories of samples from the Aqishan–Yamansu belt. The best path and weighted average path of all good and acceptable paths are shown as black and light blue curves, respectively.
The samples in the Aqishan–Yamansu belt were exposed to the surface during Early–Middle Jurassic times, which was indicated by the existence of an unconformity between Lower–Middle Jurassic sandstones and Carboniferous granites (BGMRXUAR, 1966); the models for DTS1159, DTS1336, DTS1337 and J52 show Triassic to Early Jurassic cooling (Fig. 5). Without ZHe ages, the model for DTS1341 shows cooling during Middle Jurassic time. All five samples in the Aqishan–Yamansu belt reached a maximum burial temperature of ∼80 °C at ∼140 Ma and show subsequent slow cooling from ∼140 Ma to present-day surface temperatures with a cooling rate of 0.57 °C Ma−1 (Fig. 5).
5. Discussion
5.a. Triassic–Early Jurassic cooling
Ten samples from different tectonic units in the Jueluotage area preserve Triassic cooling ages (Table 2). Thermal history models, based on ZHe, AHe and AFT ages, constrain a period of cooling from Triassic to Early Jurassic times (Figs 4, 5).
Within the study area, the KDSZ and the Aqikkuduk Fault were active as dextral strike-slip faults from late Permian to Middle Triassic times (256–243 Ma) (Chen et al. Reference Chen, Sun, Zhang, Xiao, Wang, Wang, Jiang and Yang2005; Cai et al. Reference Cai, Xu, He and Wang2012). These ages were constrained by Ar–Ar dating and represent the time of mylonite formation at temperatures between 350 °C and 400 °C (e.g. Maluski et al. Reference Maluski, Rajlich and Matte1993; Wang et al. Reference Wang, Zhang, Wang, Zhang, Li and Sun2005). Together with the ZHe ages (closure temperature of ∼180 °C; Reiners et al. Reference Reiners, Spell, Nicolescu and Zanetti2004), this suggests that the major fault zones in the Jueluotage area underwent cooling (from over 350 °C to <180 °C) during the Triassic period. This cooling episode is consistent with the modelling results (Figs 4, 5). Not only is the Triassic cooling associated with mylonitization, in addition, there is widespread regional magmatism in the Triassic period (Zhang, Z. Z. et al. Reference Zhang, Gu, Wu, Li, Xi and Wang2005; Zhou et al. Reference Zhou, Yuan, Zhang, Fan, Liu, Peng and Zhang2010; Zhang et al. Reference Zhang, Zhao, Sun, Han and Liu2017). The widespread Triassic metallogenetic events also indicate intense magmatic activity at this time (Li et al. Reference Li, Chen, Lu, Yang, Guo and Mei2005; Zhang, L. C. et al. Reference Zhang, Xiao, Qin, Qu and Du2005; Chen et al. Reference Chen, Zhang, Zhao, Han and Wang2006; Deng et al. Reference Deng, Chen, Santosh, Wang, Li, Yue, Zheng, Chen, Tang, Dong and Qu2017; Sun et al. Reference Sun, Li, Danišík, Xia, Jiang, Wu, Yang, Fan and Zhu2017; Wu et al. Reference Wu, Zhou, Li and Chen2017). These magmatic events suggest that the Jueluotage area experienced strong tectonic activity during the Triassic period. The maximum depositional ages of sandstone from the mélange matrix in the KDSZ and a marked change of provenance of the Dananhu arc and Yamansu–Central Tianshan arc constrained the closure of the North Tianshan Ocean to Permian–Early Triassic (Chen, Z et al. Reference Chen, Xiao, Windley, Schulmann, Mao, Zhang, Zhang, Li and Song2020) and Middle–Late Triassic (Ao et al. Reference Ao, Qigui, Windley, Song, Zhang, Zhang, Wan, Han and Xiao2021) times. In late Permian and Early Triassic times, thermal subsidence in the Tuha Basin took place and reflects uplift and magmatic activity in the Jueluotage area (Shao et al. Reference Shao, Stattegger, Li and Haupt1999; Greene et al. Reference Greene, Carroll, Hendrix, Graham, Wartes, Abbink, Hendrix and Davis2001). Considering the temporal coincidence between the cooling and the collision event, Triassic cooling in the Jueluotage area was likely induced by the closure of the North Tianshan Ocean (Chen, Z et al. Reference Chen, Xiao, Windley, Schulmann, Mao, Zhang, Zhang, Li and Song2020; Ao et al. Reference Ao, Qigui, Windley, Song, Zhang, Zhang, Wan, Han and Xiao2021; Fig. 6a, b).

Fig. 6. Schematic diagrams showing the tectonic evolution of the south branch of the Eastern Tianshan. (a) Before the Triassic, the North Tianshan Ocean, a branch of the Palaeo-Asian Ocean, remained open. (b) During the Triassic–Early Jurassic (˜250–180 Ma), the North Tianshan Ocean closed by doubly dipping subductions, and the exhumation lasted to Early Jurassic time. The Aqishan–Yamansu belt was exposed to the surface at the end of the Early Jurassic. (c) During the Early Jurassic – Early Cretaceous (180– >140 Ma), the Aqishan–Yamansu belt was buried. The south branch of the Eastern Tianshan was experiencing deplanation. (d) In the Early Cretaceous (˜140 Ma), differential uplifting occurred, the Aqishan–Yamansu belt uplifted ˜1.3 km more than other tectonic belts. AY – Aqishan–Yamansu belt; CTS – Central Tianshan; STS – South Tianshan; KDSZ – Kanggurtag ductile shear zone.
As a branch of the Palaeo-Asian Ocean, the youngest closure time of the North Tianshan Ocean indicates that final amalgamation of the Palaeo-Asian Ocean occurred in Middle–Late Triassic times (Xiao et al. Reference Xiao, Windley, Sun, Li, Huang, Han, Yuan, Sun and Chen2015, Reference Xiao, Windley, Han, Liu, Wan, Zhang, Ao, Zhang and Song2018; Ao et al. Reference Ao, Qigui, Windley, Song, Zhang, Zhang, Wan, Han and Xiao2021; Mao et al. Reference Mao, Ao, Windley, Wang, Li and Xiao2021). Several stratigraphic and low-temperature thermochronological studies have recorded closure of the Palaeo-Asian Ocean. In the Bogeda Shan (a northern branch of the Eastern Tianshan), a sub-horizontal Lower Jurassic layer lies on intensively folded upper Permian strata, indicating a Triassic tectonic event (Chen et al. Reference Chen, Li and Wan2015). In the Kuche basin, located in the north of Kuche city at the south edge of the Chinese Western Tianshan, a significant switch of sedimentary provenance and a contemporaneous change in palaeocurrent directions indicates Triassic uplift and denudation of the Tianshan Mountains (Han et al. Reference Han, Zhao, Sun, Eizenhöfer, Hou, Zhang, Liu and Wang2016). In the north of the Tuha Basin, Triassic cooling was recorded by AFT in the Harlik Shan (Gillespie et al. Reference Gillespie, Glorie, Jepson, Zhang, Xiao, Danišík and Collins2017; Chen, Y et al. Reference Chen, Wang, Kapp, Shen, Zhang, Zhu and Cao2020), and was ascribed to being related to the closure of the Palaeo-Asian Ocean (Gillespie et al. Reference Gillespie, Glorie, Jepson, Zhang, Xiao, Danišík and Collins2017). Triassic cooling was also identified by low-temperature thermochronology in the Dananhu arc (Gong et al. Reference Gong, Kohn, Zhang, Xiao, Wu and Chen2021), Du-Ku Highway section, Kepingtage in the northwestern Tarim (Dumitru et al. Reference Dumitru, Zhou, Chang, Graham, Hendrix, Sobel, Carroll, Hendrix and Davis2001; Jolivet et al. Reference Jolivet, Dominguez, Charreau, Chen, Li and Wang2010), Central Chinese Tianshan (Yin et al. Reference Yin, Chen, Hodges, Xiao, Cai, Yuan, Sun, Liu and van Soest2017; Zhang et al. Reference Zhang, Chen, Liu, Yin and Sun2020), West Junggar (Li et al. Reference Li, Cao, Qin, Evans, McInnes and Liu2014; Yin et al. Reference Yin, Chen, Xiao, Long, Tao, Liu, Yuan and Sun2018; Glorie et al. Reference Glorie, Otasevic, Gillespie, Jepson, Danišík, Zhimulev, Gurevich, Zhang, Song and Xiao2019; Gillespie et al. Reference Gillespie, Glorie, Jepson, Xiao and Collins2020), Kyrgyz and Uzbekistan–Tajikistan Tianshan (De Grave et al. Reference De Grave, Glorie, Buslov, Izmer, Fournier-Carrie, Batalev, Vanhaecke, Elburg and Van den Haute2011, Reference De Grave, Glorie, Buslov, Stockli, McWilliamsn, Batalev and Van den Haute2013; Jepson et al. Reference Jepson, Glorie, Konopelko, Gillespie, Danišík, Evans, Mamadjanov and Collins2018 a,b) and Siberian Altai–Sayan (Glorie & De Grave, Reference Glorie and De Grave2016).
The models in this study reveal that cooling continued until Early Jurassic time (Figs 4, 5, 6b). This is consistent with the Late Triassic – Early Jurassic accelerated basin subsidence in the Tuha Basin (Hendrix et al. Reference Hendrix, Graham, Carroll, Sobel, McKnight, Schulein and Wang1992; Shao et al. Reference Shao, Stattegger, Li and Haupt1999). In the eastern Junggar Basin, Lower Jurassic conglomerate and a base Jurassic angular unconformity were observed (Vincent & Allen, Reference Vincent, Allen, Hendrix and Davis2001). Upper Triassic strata consisting of alluvial conglomerate and associated braided-fluvial sandstone were observed in the Tarim Basin (Hendrix et al. Reference Hendrix, Graham, Carroll, Sobel, McKnight, Schulein and Wang1992). Late Triassic – Early Jurassic basement cooling was also recorded by low-temperature thermochronology in Beishan (Gillespie et al. Reference Gillespie, Glorie, Jepson, Zhang, Xiao, Danišík and Collins2016), Chinese Tianshan (Dumitru et al. Reference Dumitru, Zhou, Chang, Graham, Hendrix, Sobel, Carroll, Hendrix and Davis2001; Jolivet et al. Reference Jolivet, Dominguez, Charreau, Chen, Li and Wang2010; Glorie et al. Reference Glorie, Otasevic, Gillespie, Jepson, Danišík, Zhimulev, Gurevich, Zhang, Song and Xiao2019; Zhang et al. Reference Zhang, Chen, Liu, Yin and Sun2020) and Kyrgyz and Uzbekistan Tianshan (De Grave et al. Reference De Grave, Glorie, Buslov, Izmer, Fournier-Carrie, Batalev, Vanhaecke, Elburg and Van den Haute2011; Glorie & De Grave, Reference Glorie and De Grave2016; Jepson et al. Reference Jepson, Glorie, Konopelko, Mirkamalov, Danišík and Collins2018 b; Nachtergaele et al. Reference Nachtergaele, De Pelsmaeker, Glorie, Zhimulev, Jolivet, Danišík, Buslov and De Grave2018), as well as the Altyn Tagh range (Sobel et al. Reference Sobel, Arnaud, Jolivet, Ritts, Brunel, Hendrix and Davis2001). This event was contemporaneous with collision/accretion of the Qiangtang Block and Kunlun terrane (Schwab et al. Reference Schwab, Ratschbacher, Siebel, McWilliams, Minaev, Lutkov, Chen, Stanek, Nelson and Frisch2004; Xu et al. Reference Xu, Dilek, Cao, Yang, Robinson, Ma, Li, Jolivet, Roger and Chen2015; Kapp & DeCelles, Reference Kapp and DeCelles2019), suggesting that the Late Triassic – Early Jurassic uplift of the Tianshan Mountains resulted from the far-field effects of this collision (Hendrix et al. Reference Hendrix, Graham, Carroll, Sobel, McKnight, Schulein and Wang1992; Glorie & De Grave, Reference Glorie and De Grave2016).
In summary, the results suggest that Triassic–Early Jurassic cooling in the Jueluotage area was related to closure of the North Tianshan Ocean and subsequent far-field effects of collision/accretion of the Qiangtang Block and Kunlun terrane.
5.b. Differential exhumation process and late Mesozoic–Cenozoic slow cooling
As Lower–Middle Jurassic sandstones unconformably overlie granites in the Aqishan–Yamansu belt (BGMRXUAR, 1966), the granites must have been exposed at the surface before Middle Jurassic time and then experienced burial during deposition of Jurassic strata (Fig. 6b, c). The AHe ages of samples in the Aqishan–Yamansu belt (DTS1159, DTS1336, DTS1337, DTS1341 and J52) were constrained to the Late Cretaceous (ranging from 75.0 ± 4.4 Ma to 87.8 ± 5.8 Ma). However, samples in major fault zones (DTS1326, DTS1327, DTS1359, DTS1371, DTS1306, DTS1308 and DTS1378) and the Central Tianshan arc (J58) yielded Late Jurassic and Early Cretaceous AHe ages (ranging from 134.8 ± 8.8 Ma to 149.5 ± 9.3 Ma). The AHe ages in the Aqishan–Yamansu belt differ from other tectonic belts, which suggests that this belt experienced a different denudation history from other tectonic belts. The AFT ages in the Aqishan–Yamansu belt are older than the depositional ages, indicating that the subsequent deposition upon the granites was insufficient to reset their AFT ages, and the reheating temperature was <110 °C and the burial depth was <3.3 km (assuming a palaeogeothermal gradient of 30 °C km−1). According to the thermal history pathways, reheating stopped at around 140 Ma and was followed by monotonic cooling to surficial temperatures (Fig. 5).
During the Jurassic period, the Tianshan Mountain region was experiencing a progressive planation of a late Palaeozoic to early Mesozoic topographic relief (Jolivet et al. Reference Jolivet, Dominguez, Charreau, Chen, Li and Wang2010; Liu et al. Reference Liu, Jolivet, Yang, Zhang, Cheng, Zhu and Guo2013; Morin et al. Reference Morin, Jolivet, Robin, Heilbronn, Barrier, Bourquin and Jia2018). The sediments on both sides of the Tianshan changed from Lower Jurassic proximal coarse-grained sediments to distal finer-grained deposits (Hendrix et al. Reference Hendrix, Graham, Carroll, Sobel, McKnight, Schulein and Wang1992; Morin et al. Reference Morin, Jolivet, Robin, Heilbronn, Barrier, Bourquin and Jia2018), which supports the decrease in relief. Several coal seams were observed in Jurassic sediments inside the Aqishan–Yamansu belt (BGMRXUAR, 1966), also indicating the presence of low relief. Thermal modelling of samples from major fault zones and the Central Tianshan arc shows that Triassic–Early Jurassic cooling was followed by a slower cooling stage in Middle Jurassic – Early Cretaceous times (Figs 4, 6c). This indicates that the tectonic belts on both side of the Aqishan–Yamansu belt were experiencing slow denudation during Jurassic time.
Since Early Cretaceous time, burial in the Aqishan–Yamansu belt stopped and exhumation began. This change coincides with the latest Jurassic – earliest Cretaceous tectonic activity in the Tuha and Junggar basins (Hendrix et al. Reference Hendrix, Graham, Carroll, Sobel, McKnight, Schulein and Wang1992; Shao et al. Reference Shao, Stattegger, Li and Haupt1999; Jolivet et al. Reference Jolivet, Heilbronn, Robin, Barrier, Bourquin, Guo, Jia, Guerit, Yang and Fu2013) and Kyrgyz and Chinese Tianshan (Dumitru et al. Reference Dumitru, Zhou, Chang, Graham, Hendrix, Sobel, Carroll, Hendrix and Davis2001; Glorie, et al. Reference Glorie, De Grave, Buslov, Elburg, Stockli, Gerdes and Van den Haute2010; Jolivet et al. Reference Jolivet, Dominguez, Charreau, Chen, Li and Wang2010). Low-temperature thermochronology ages for the Yandong deposit (a porphyry copper deposit in the Dananhu arc) also revealed accelerated cooling in Early Cretaceous time (Gong et al. Reference Gong, Kohn, Zhang, Xiao, Wu and Chen2021). As shown by the thermal modelling results, there was a difference of ∼40 °C between samples in the Aqishan–Yamansu belt and other tectonic belts at ∼140 Ma (Figs 4, 5, 6c). This indicates that the burial depth in the Aqishan–Yamansu belt was 1.3 km deeper than in other tectonic belts. Considering the samples in different belts are at the same altitude now, we speculate that differential uplift occurred between tectonic belts in the Jueluotage area during Early Cretaceous time, culminating in ∼1.3 km more uplift in the Aqishan–Yamansu belt relative to other belts (Fig. 6d). In late Mesozoic and Cenozoic times, all the tectonic belts in the Jueluotage area remained stable, and the major faults (i.e. Aqikkuduk Fault) experienced little to no reactivation. The present topography of the Jueluotage area reflects the topography of the late Mesozoic period (Guo et al. Reference Guo, Zhang, Liao and Fang2002).
6. Conclusion
New zircon and apatite (U–Th)/He and AFT ages further constrain episodes of exhumation in the Jueluotage area. A Triassic–Early Jurassic (∼240–180 Ma) cooling stage is revealed throughout the whole Jueluotage region. This cooling stage was driven by exhumation induced by the closure of the North Tianshan Ocean and subsequent far-field effects of collision/accretion of the Qiangtang Block and Kunlun terrane. After the Triassic–Early Jurassic cooling, the major fault zones (KDSZ and Aqikkuduk Fault) and the Central Tianshan arc have remained tectonically stable since Early Jurassic time. Meanwhile, the Aqishan–Yamansu belt experienced burial until Late Jurassic to Early Cretaceous times. At the beginning of Early Cretaceous time, differential uplift may have occurred between the Aqishan–Yamansu belt and other tectonic belts.
Acknowledgements
This study was supported by the National Natural Science Foundation of China (Grant No. 41503057, 41473053, 41830430); the National Key Research and Development Project of China (2017YFC0601301); and the China Geological Survey (Grant No. DD20190001, DD20190004). We thank Hongwei Ji and Li Yang for their help with field trips, and engineer Xinyu Liu and Qiaoda Zhang for their help with experimental analysis. We also thank Olivier Lacombe for handling this manuscript, and Stijn Glorie and Gilby Jepson for their valuable comments that improved the manuscript.
Supplementary material
To view supplementary material for this article, please visit https://doi.org/10.1017/S0016756821000522