INTRODUCTION
Recent research has demonstrated the utility of noninvasive brain stimulation techniques, including transcranial magnetic stimulation (TMS) and transcranial direct current stimulation (tDCS), as neuroscientific tools to modulate cognitive functions (Coffman, Clark, & Parasuraman, Reference Coffman, Clark and Parasuraman2014; McKinley, Bridges, Walters, & Nelson, Reference McKinley, Bridges, Walters and Nelson2012; Parasuraman & McKinley, Reference Parasuraman and McKinley2014). Commonly used protocols for tDCS (i.e., anodal tDCS over a targeted region) and TMS (i.e., repetitive high-frequency TMS) have shown mixed results (Dedoncker, Brunoni, Baeken, & Vanderhasselt, Reference Dedoncker, Brunoni, Baeken and Vanderhasselt2016; McKinley et al., Reference McKinley, Bridges, Walters and Nelson2012; Oberman, Edwards, Eldaief, & Pascual-Leone, Reference Oberman, Edwards, Eldaief and Pascual-Leone2011), including facilitatory effects (Hoy et al., Reference Hoy, Emonson, Arnold, Thomson, Daskalakis and Fitzgerald2013, Reference Hoy, Bailey, Michael, Fitzgibbon, Rogasch, Saeki and Fitzgerald2016; Luber & Lisanby, Reference Luber and Lisanby2014), inhibitory effects (Berryhill, Wencil, Coslett & Olson, Reference Berryhill, Wencil, Coslett and Olson2010; Morgan, Jackson, van Koningsbruggen, Shapiro, & Linden, Reference Morgan, Jackson, van Koningsbruggen, Shapiro and Linden2013) and null findings (Horvath, Forte, & Carter, Reference Horvath, Forte and Carter2015; Meier & Sauter, Reference Meier and Sauter2018; van Wessel et al., Reference van Wessel, Claire Verhage, Holland, Frens and van der Geest2016). These findings indicate a need to further investigate and optimize the use of these methods. Newer specialized forms of these types of stimulation, such as high-definition tDCS (HD-tDCS) and theta burst stimulation (TBS), may have enhanced neuromodulatory effectiveness due to increased focality (i.e., HD-tDCS: Datta et al., Reference Datta, Bansal, Diaz, Patel, Reato and Bikson2009; Kuo et al., Reference Kuo, Bikson, Datta, Minhas, Paulus, Kuo and Nitsche2013) or may be more efficient due to physiological effects (i.e., TBS: Di Lazzaro et al., Reference Di Lazzaro, Pilato, Dileone, Profice, Oliviero, Mazzone, Insola, Ranieri, Meglio, Tonali and Rothwell2008; Huang, Edwards, Rounis, Bhatia, & Rothwell, Reference Huang, Edwards, Rounis, Bhatia and Rothwell2005; Oberman et al., Reference Oberman, Edwards, Eldaief and Pascual-Leone2011; Suppa et al., Reference Suppa, Huang, Funke, Ridding, Cheeran, Di Lazzaro, Ziemann and Rothwell2016). However, these new stimulation techniques have not been directly compared in previous studies and the question of which technique may be optimal for modulating cognitive functioning remains unclear. Given the expanding literature exploring the use of noninvasive brain stimulation to enhance cognition in healthy populations (Gbadeyan, McMahon, Steinhauser, & Meinzer, Reference Gbadeyan, McMahon, Steinhauser and Meinzer2016; Gozenman & Berryhill, Reference Gozenman and Berryhill2016; Hill, Rogasch, Fitzgerald, & Hoy, Reference Hill, Rogasch, Fitzgerald and Hoy2017; Hogeveen et al., Reference Hogeveen, Grafman, Aboseria, David, Bikson and Hauner2016; Lowe, Manocchio, Safati, & Hall, Reference Lowe, Manocchio, Safati and Hall2018; Nikolin, Loo, Bai, Dokos, & Martin, Reference Nikolin, Loo, Bai, Dokos and Martin2015) and in clinical disorders (Martin, McClintock, Forster, Lo, & Loo, Reference Martin, McClintock, Forster, Lo and Loo2017; Richardson, Datta, Dmochowski, Parra, & Fridriksson, Reference Richardson, Datta, Dmochowski, Parra and Fridriksson2015; Shekhawat & Vanneste, Reference Shekhawat and Vanneste2018; Suppa et al., Reference Suppa, Huang, Funke, Ridding, Cheeran, Di Lazzaro, Ziemann and Rothwell2016), this study aimed to investigate the relative efficacy of these newer forms of stimulation as tools for modulating cognition.
tDCS involves the application of a sub-threshold electrical stimulus to the brain via electrodes placed upon the scalp to modulate neuronal activity. HD-tDCS is a newly developed form of tDCS that utilizes multiple smaller-sized electrodes and electrode montages to limit the spread of current flow outside of the targeted stimulated region (Datta et al., Reference Datta, Bansal, Diaz, Patel, Reato and Bikson2009; Kuo et al., Reference Kuo, Bikson, Datta, Minhas, Paulus, Kuo and Nitsche2013). Previous studies showed that HD-tDCS was useful for probing cognitive functions of particular targeted regions (Nikolin et al., Reference Nikolin, Loo, Bai, Dokos and Martin2015) and could induce robust neuromodulatory changes (Hill et al., Reference Hill, Rogasch, Fitzgerald and Hoy2017). When used for cognitive enhancement, however, results have been mixed (Gbadeyan et al., Reference Gbadeyan, McMahon, Steinhauser and Meinzer2016; Hill et al., Reference Hill, Rogasch, Fitzgerald and Hoy2017; Hill, Rogasch, Fitzgerald, & Hoy, Reference Hill, Rogasch, Fitzgerald and Hoy2018; Nikolin, Lauf, Loo, & Martin., Reference Nikolin, Lauf, Loo and Martin2019; Nikolin et al., Reference Nikolin, Loo, Bai, Dokos and Martin2015). This raises the need for further investigation into the efficacy of this approach.
TMS involves the generation of a magnetic field, which is used to induce neuronal depolarization in the brain (Fitzgerald, Fountain, & Daskalakis, Reference Fitzgerald, Fountain and Daskalakis2006). When high-frequency repetitive TMS (rTMS) is administered immediately prior to a cognitive task, it can modulate task performance (Luber & Lisanby, Reference Luber and Lisanby2014). However, these effects are modest in magnitude and short-lasting. An alternative approach to the delivery of rTMS, namely TBS, may have greater neuromodulatory potential than the standard approach (Hoy et al., Reference Hoy, Bailey, Michael, Fitzgibbon, Rogasch, Saeki and Fitzgerald2016). TBS involves the delivery of rTMS pulses in very short bursts at a repetitive high frequency (e.g., 50 Hz), and these short bursts are applied at 5 Hz. TBS is thought to more robustly alter cortical excitability by mimicking the brain’s natural firing patterns (Hoy et al., Reference Hoy, Bailey, Michael, Fitzgibbon, Rogasch, Saeki and Fitzgerald2016; Oberman et al., Reference Oberman, Edwards, Eldaief and Pascual-Leone2011). In the motor cortex, intermittent TBS (iTBS) has been shown to induce greater and longer-lasting effects on cortical excitability than high-frequency rTMS (Di Lazzaro et al., Reference Di Lazzaro, Pilato, Dileone, Profice, Oliviero, Mazzone, Insola, Ranieri, Meglio, Tonali and Rothwell2008; Huang et al., Reference Huang, Edwards, Rounis, Bhatia and Rothwell2005). Preliminary evidence further suggests that prolonged continuous TBS (ProcTBS) could induce similar lasting and potentially greater excitability-enhancing effects as iTBS (Gamboa, Antal, Moliadze, & Paulus, Reference Gamboa, Antal, Moliadze and Paulus2010). In terms of cognitive effects, previous studies have found that iTBS delivered to the left dorsolateral prefrontal cortex (DLPFC) significantly improved healthy participants’ performance on a working memory task with low, but not high, load (Hoy et al., Reference Hoy, Bailey, Michael, Fitzgibbon, Rogasch, Saeki and Fitzgerald2016), and that when applied over the left posterior parietal cortex (PPC), iTBS showed a trend toward decreased response time in attention (Anderkova et al., Reference Anderkova, Pizem, Klobusiakova, Gajdos, Koritakova and Rektorova2018). Similar to HD-tDCS, these mixed and limited effects raise the need for further investigation into the efficacy of iTBS. Further, to the best of our knowledge, the cognitive effects of ProcTBS have not been examined to date.
The PPC, in particular, the intraparietal sulcus (IPS), has been implicated as an important node in the dorsal attention network (DAN) (Vossel, Geng, & Fink, Reference Vossel, Geng and Fink2014) and a functionally important region for multiple cognitive functions, including working memory (Luber et al., Reference Luber, Kinnunen, Rakitin, Ellsasser, Stern and Lisanby2007), episodic memory (Innocenti et al., Reference Innocenti, Cappa, Feurra, Giovannelli, Santarnecchi, Bianco, Cincotta and Rossi2013; Jacobson, Goren, Lavidor, & Levy, Reference Jacobson, Goren, Lavidor and Levy2012), long-term memory (Jones, Gozenman, & Berryhill, Reference Jones, Gozenman and Berryhill2014), associative memory (Wang et al., Reference Wang, Rogers, Gross, Ryals, Dokucu, Brandstatt, Hermiller and Voss2014), visuospatial attention (Capotosto et al., Reference Capotosto, Baldassarre, Sestieri, Spadone, Romani and Corbetta2017) and visual divided attention (Santangelo & Macaluso, Reference Santangelo and Macaluso2013). Of interest, several studies have demonstrated a functional role of the left PPC in subserving working memory by employing conventional rTMS (Albouy, Weiss, Baillet, & Zatorre, Reference Albouy, Weiss, Baillet and Zatorre2017; Hamidi, Slagter, Tononi, & Postle, Reference Hamidi, Slagter, Tononi and Postle2009). Further, a recent study found moderate-sized improvements in working memory maintenance and divided attention following HD-tDCS administered to the left IPS (P3), although these effects failed to reach statistical significance (Nikolin et al., Reference Nikolin, Lauf, Loo and Martin2019). Compared to TMS, which focally stimulates the cortical regions directly beneath the coil, the relative diffusivity of HD-tDCS stimulates a greater proportion of the brain (Hill et al., Reference Hill, Rogasch, Fitzgerald and Hoy2017). This approach then could also have utility for modulating functions of the PPC. Functional neuroimaging studies have further implicated both the left and right IPS as important for maintenance functioning during working memory (Cabeza & Nyberg, Reference Cabeza and Nyberg2000; Jha & McCarthy, Reference Jha and McCarthy2000). In addition, the left and right IPS were recently implicated in supporting divided attention functioning (Fagioli & Macaluso, Reference Fagioli and Macaluso2009; Santangelo & Macaluso, Reference Santangelo and Macaluso2013). Taken together, these results may suggest a specific functional role of the IPS in supporting the storage of processing resources for both abilities. However, fMRI can only indicate the correlations between brain and behavior (Sliwinska, Vitello & Devlin, Reference Sliwinska, Vitello and Devlin2014). The causal relationship between the left PPC and maintenance in working memory and divided attention has yet to be demonstrated, for example, by using brain stimulation. Given the relative focality of both TBS and HD-tDCS, a secondary aim was to explore the potential of these neurostimulation approaches for modulating these specialized functions of the PPC. The left PPC, specifically, was targeted, due to the language-processing requirements of the working memory maintenance and divided attention tasks used in our prior HD-tDCS study (Nikolin et al., Reference Nikolin, Lauf, Loo and Martin2019) and the dominance of the left hemisphere for such processing in the majority of individuals (Kreisler et al., Reference Kreisler, Godefroy, Delmaire, Debachy, Leclercq, Pruvo and Leys2000).
This current study thus had two aims: (1) to investigate the cognitive enhancing effects of HD-tDCS and TBS (iTBS and ProcTBS) and (2) to explore the effects on specialized functions of the left PPC, namely, working memory maintenance and divided attention. We hypothesized that, compared to sham, all three approaches would cause significant performance improvement on the assessed outcome measures. Further, given the suspected specialized role of the left PPC in subserving working memory maintenance and divided attention functions, we hypothesized that effects would be greater on these outcomes compared to that of control task which assessed generalized attentional processing.
METHODS
Trial Design and Study Protocol
A single-blind, cross-over, sham-controlled experimental design was used. In line with our first aim, a power analysis suggested that a sample size of N = 22 was required to detect a medium-sized effect of Cohen’s d ≥ .55 between active and sham stimulation conditions (alpha = 5%, power = 80%) (Hoy et al., Reference Hoy, Bailey, Michael, Fitzgibbon, Rogasch, Saeki and Fitzgerald2016). Participants were informed that they would receive TBS and HD-tDCS and would be required to complete some cognitive task, without any further detail of expected effects. Participants were blinded to each stimulation condition. The order of stimulation was randomized and counterbalanced across all participants. After participants signed informed consent, working memory (Digit Span Test; Wechsler, Coalson, & Raiford, Reference Wechsler, Coalson and Raiford2008) and attention/processing speed (Ruff 2 & 7 Selective Attention Test; Ruff & Allen, Reference Ruff and Allen1996) were assessed to determine baseline cognitive functioning.
Each participant completed four sessions, with each session separated by an interval of at least 1 week. In each session, all participants received a single type of stimulation (HD-tDCS, iTBS, ProcTBS or sham HD-tDCS) and completed three cognitive tasks which assessed attention, working memory maintenance and divided attention, with the order of tests randomized and counterbalanced for each participant. For active and sham HD-tDCS sessions, after receiving 5 min of active/sham HD-tDCS, participants completed cognitive tasks while receiving the remaining 15 min of active or sham stimulation. In each TBS session, participants first received iTBS/ProcTBS and then waited for 10 min before commencing the cognitive tasks (Figure 1). This duration was chosen based on a previous study which reported that the effects of iTBS and ProcTBS on cortical excitability reached their maximum after 10 min of stimulation (Gamboa et al., Reference Gamboa, Antal, Moliadze and Paulus2010).

Fig. 1. Experimental procedure. iTBS, intermittent theta burst stimulation; ProcTBS, prolonged continuous TBS; HD-tDCS, high-definition transcranial direct current stimulation. Cognitive tasks included maintenance task, divided attention and emotional Stroop task. The total time of these tasks was 15 min. The order of tasks was randomized and counterbalanced for each participant.
Participants
Twenty-two healthy participants (14 females) recruited from the University of New South Wales took part in this study. Inclusion criteria were: aged between 18 and 40, right-handed as assessed by the Edinburgh Handedness Inventory (Oldfield, Reference Oldfield1971), fluent in English, normal color vision and hearing, not taking any concurrent medications which may affect cognitive performance, free from any neurological or psychiatric disorder, no recent head injury, no history of seizure or stroke and no current history of drug or alcohol abuse or dependence. The study was approved by the human research ethics committee of the University of New South Wales, and performed in accordance with the principles outlined in the Australian National Statement of Ethical Conduct in Human Research.
High-Definition Transcranial Direct Current Stimulation
Active HD-tDCS (Soterix Medical, New York, NY) was delivered continuously for 20 min at a current intensity of 2 mA. The current was gradually ramped up over 30 s, and back down over 30 s at the cessation of stimulation. Each participant received anodal stimulation of the left PPC (P3 according to the international 10–20 EEG system) using a 4 × 1 ring electrode configuration whereby cathodes were placed on adjacent electrode sites (P7, Pz, C3, O1). Left PPC was chosen as the targeted region based on the results of our previous study (Nikolin et al., Reference Nikolin, Lauf, Loo and Martin2019) and due to its importance in auditory verbal working memory (Albouy et al., Reference Albouy, Weiss, Baillet and Zatorre2017; Warrington & Shallice, Reference Warrington and Shallice1969), visual divided attention (Fagioli & Macaluso, Reference Fagioli and Macaluso2009; Santangelo & Macaluso, Reference Santangelo and Macaluso2013) and the DAN (Anderkova et al., Reference Anderkova, Pizem, Klobusiakova, Gajdos, Koritakova and Rektorova2018; Paulraj, Schendel, Curran, Dronkers, & Baldo, Reference Paulraj, Schendel, Curran, Dronkers and Baldo2018). The electrode positions are shown in Figure 2 (left panel). For the sham condition, the same electrode positions were used, though the stimulation was gradually ramped up over 30 s to 1 mA, then ramped down over 30 s and the machine completely switched off. This procedure has been shown to elicit minimal discomfort and to be indistinguishable from active stimulation by study participants (Gandiga, Hummel & Cohen, Reference Gandiga, Hummel and Cohen2006).
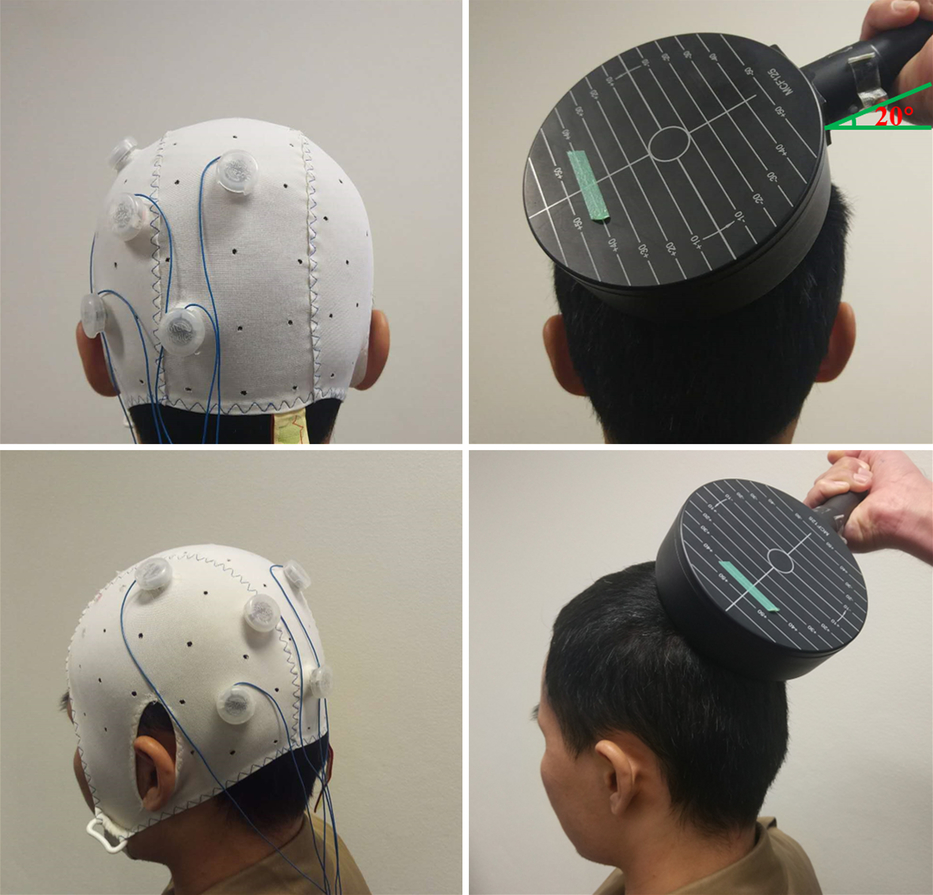
Fig. 2. Montages and coil position used for HD-tDCS (left) and TBS (right). Left panel: Sample 4 × 1 HD-tDCS setup: the anode electrode over the left PPC (P3) and the cathode electrodes over adjacent sites (P7, Pz, C3, O1). Right panel: Coil position in TBS sessions: the circular coil was placed on P3 with the handle pointing laterally and slightly anteriorly (20° from the horizontal line).
Theta Burst Stimulation
TBS was applied using a MagPro×100 (MagVenture Company, Lucernemarken, Denmark) stimulator and a 114-mm-diameter circular coil. The circular coil was chosen because it stimulates a larger area than the figure-of-8 coil (Hallett, Reference Hallett2007; Rossini et al., Reference Rossini, Burke, Chen, Cohen, Daskalakis, DiIorio and Ziemann2015). Previous studies have similarly used a circular coil to stimulate the PPC (Chang & Ro, Reference Chang and Ro2007; Ro, Wallace, Hagedorn, Farne & Pienkos, Reference Ro, Wallace, Hagedorn, Farne and Pienkos2004). The stimulation intensity used for TBS conditions was determined as a percentage of each participant’s resting motor threshold (RMT), latter defined by the method of limits, i.e., as the intensity required to elicit motor-evoked potentials with a peak-to-peak amplitude of at least 50 μV, in three out of six trials (Conforto, Z’Graggen, Kohl, Rösler, & Kaelin-Lang, Reference Conforto, Z’Graggen, Kohl, Rösler and Kaelin-Lang2004; Mello et al., Reference Mello, Cohen, Monteiro dos Anjos, Conti, Andrade, Tovar Moll, Fernandes, Rodrigues and Conforto2015). We first used a 65-mm-diameter figure-of-8 coil to locate the representation area of the contralateral right first dorsal interosseous muscle. Then RMT was determined using the circular coil.
Similar to the HD-tDCS, the circular coil was placed over P3 (Herwig, Satrapi & Schönfeldt-Lecuona, Reference Herwig, Satrapi and Schönfeldt-Lecuona2003), with the position marked on each participant’s head with a pen (Figure 2, right panel). The coil orientation was lateral-medial with the handle pointing laterally and slightly anteriorly (20° from the horizontal line), to induce a current in the underlying cortical tissue approximately perpendicular (Mazzoni, Jacobs, Venuti, Silvanto, & Cattaneo, Reference Mazzoni, Jacobs, Venuti, Silvanto and Cattaneo2017). The TBS pattern consisted of bursts containing three pulses at 50 Hz repeated at 5 Hz. For iTBS, TBS was applied using an intermittent protocol at 80% of RMT, and a 2-s train of TBS was repeated every 10 s for a total of 190 s (600 pulses) (Hoy et al., Reference Hoy, Bailey, Michael, Fitzgibbon, Rogasch, Saeki and Fitzgerald2016). For ProcTBS, an 80-s train of uninterrupted TBS was given (1200 pulses) over P3 at 70% of RMT (Gamboa et al., Reference Gamboa, Antal, Moliadze and Paulus2010).
Cognitive Tasks
Cognitive tasks were all administered via a computer using custom-made programs, scripted through software package Inquisit (version 4; Millisecond Software LLC, Seattle, Washington). The total duration of these three tasks was approximately 15 min.
Working memory maintenance task
The working memory maintenance task was an operation span task (Turner & Engle, Reference Turner and Engle1989). This was the same task as that used in a prior study which investigated the effects of HD-tDCS stimulation over P3 (Nikolin et al., Reference Nikolin, Lauf, Loo and Martin2019). It has been previously shown to have a high test–re-test reliability (r > .70) (Klein & Fiss, Reference Klein and Fiss1999; Unsworth, Heitz, Schrock & Engle, Reference Unsworth, Heitz, Schrock and Engle2005). Participants saw a series of letters presented, during which they were required to perform simple mathematic calculations in between each letter presentation shown on the screen. At the end of each trial, participants were asked to recall as many letters as possible from the beginning of the series, in the order of presentation (Figure 3a). Letters were presented in series lengths of 4, 6, 8 and 10 letters in a random order, with two trials of each length. The outcome measure of this task was the total correct score, which is the total number of letters recalled across all the series lengths (maximum score = 56), representing verbal maintenance capacity.
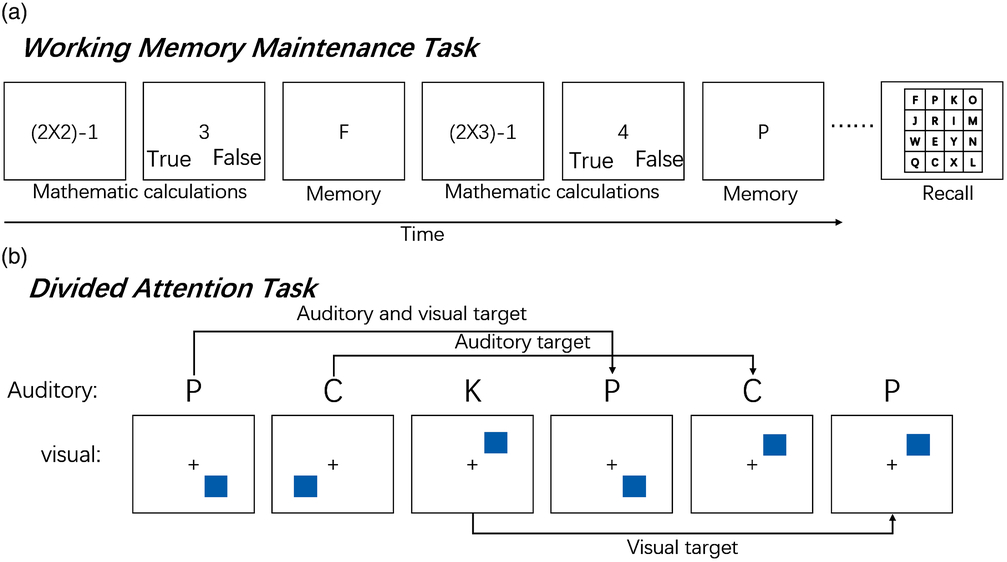
Fig. 3. Experimental paradigms of maintenance task and divided attention task. (a) Schematic of the working memory maintenance task. Participants saw a series of letters presented, during which they were required to perform simple mathematical calculations (in between each letter presentation, shown on the screen). At the end of the trial, participants were asked to recall as many letters as possible from the beginning of the series, in the order of presentation. (b) Participants heard a series of eight different letters presented one at a time, each paired simultaneously with a visual stimulus. The visual stimulus consisted of a box presented in one of eight locations around the periphery of the screen. Participants were asked to respond by pressing different keys whenever the current location of the box/letter matches the location/letter three trials back (press “A” for a matching location; press “L” for a matching letter; press “A” and “L” when the location and the letter match).
Divided attention task
The divided attention task consisted of both auditory and visual components of the dual three-back task (adapted from Jaeggi, Buschkuehl, Jonides, & Perrig, Reference Jaeggi, Buschkuehl, Jonides and Perrig2008). This task was identical to that used in a prior study which examined the stimulation effects of HD-tDCS over P3 (Nikolin et al., Reference Nikolin, Lauf, Loo and Martin2019). Participants heard a series of eight different letters presented one at a time, each paired simultaneously with a visual stimulus. The visual stimulus consisted of a box presented in one of eight locations around the periphery of the screen. Each paired stimulus presentation lasted for 500 ms, and a following inter-stimulus interval lasted for 2500 ms, during which participants could respond. Participants were asked to respond by pressing different keys whenever the current location of the box/letter matched the location/letter three trials back (Figure 3b). This task consisted of 16 auditory targets, 16 visual targets, 8 combined targets and 40 non-targets. D-prime (Z (hit rate) – Z (false alarm rate)) was calculated to provide a measure of divided attention accuracy. The reliability of the n-back task with high task levels (two- and three-back) was >.8 (Jaeggi, Buschkuehl, Perrig, & Meier, Reference Jaeggi, Buschkuehl, Perrig and Meier2010).
Emotional Stroop task
The emotional Stroop task was chosen as a control task to assess generalized attentional processing (Peterson et al., Reference Peterson, Skudlarski, Gatenby, Zhang, Anderson and Gore1999). It has been shown to have a high test–re-test reliability (i.e., r’s > .70) (Strauss, Allen, Jorgensen, & Cramer, Reference Strauss, Allen, Jorgensen and Cramer2005). One hundred twenty-five words from five different categories (aggression, neutral, positive, negative, color word) in four different colors (blue, red, yellow, green) were presented on the center of the screen. Participants were asked to press one of four response keys to indicate the color of the words regardless of their meaning as soon as possible (Smith & Waterman, Reference Smith and Waterman2003). The outcome measure of this task was the reaction time (RT) for correct responses.
Assessment of Safety and Subjective Impression of Improvement
At the end of each session, patients were asked to report any adverse events that were associated with stimulation, using a standardized questionnaire developed by the authors (Martin et al., Reference Martin, Alonzo, Ho, Player, Mitchell, Sachdev and Loo2013). At the completion of the final session, each participant was asked: “In your opinion, was there a type of brain stimulation that improved your performance?”
Statistical Analysis
Statistical analyses were conducted using the Statistical Package for the Social Sciences (SPSS) for Windows (version 25.0; IBM Corp., Armonk, NY, USA). RT data <200 ms, or outside the range of three standard deviations away from the study mean for that task, were removed prior to analysis. Separate mixed-effects models for repeated measures (MMRM) were conducted for each cognitive outcome measure using stimulation condition and emotion type (for the emotional Stroop task only) as fixed factors, subject as a random factor, and session number (visit) as a covariate. MMRM analysis was chosen as these models can better account for individual subjects’ variability in repeated measurements over time through the inclusion of subject as a random factor (Gueorguieva & Krystal, Reference Gueorguieva and Krystal2004). For the main effect of stimulation condition on the respective outcome measures, adjustments for multiple comparisons were made using a Bonferroni correction. Post hoc pairwise comparisons were conducted to interpret significant main effects. A p-value < .05 was considered statistically significant. Paired t-tests and Cohen’s d effect size were used to examine performance differences between active and sham stimulation conditions if the main effect of stimulation was significant.
Secondly, we tested the hypotheses further using Bayesian repeated-measures ANOVA through the software package Jeffreys’s Amazing Statistics Program (JASP, version 0.8.6; Wagenmakers, Love, et al., 2018; Wagenmakers, Marsman, et al., Reference Wagenmakers, Marsman, Jamil, Ly, Verhagen, Love and Morey2018). Whereas a typical analysis would not allow for the interpretation of null effects, this Bayesian technique allowed us to evaluate the strength of both the null and alternative hypothesis based on the current data (Wagenmakers, Love, et al., Reference Wagenmakers, Love, Marsman, Jamil, Ly, Verhagen, Selker, Gronau, Dropmann, Boutin, Meerhoff, Knight, Raj, van Kesteren, van Doorn, Šmíra, Epskamp, Etz, Matzke, de Jong, van den Bergh, Sarafoglou, Steingroever, Derks, Rouder and Morey2018; Wagenmakers, Marsman, et al., Reference Wagenmakers, Marsman, Jamil, Ly, Verhagen, Love and Morey2018). Bayer factors (BF10) were used to assess the strength of evidence for H1 relative to H0. A BF10 >3 indicates evidence for the alternative hypothesis and <1/3 indicates evidence for the null hypothesis. BFs between 1/3 and 3 indicate data insensitivity in distinguishing H0 and H1 (Wagenmakers, Love, et al., Reference Wagenmakers, Love, Marsman, Jamil, Ly, Verhagen, Selker, Gronau, Dropmann, Boutin, Meerhoff, Knight, Raj, van Kesteren, van Doorn, Šmíra, Epskamp, Etz, Matzke, de Jong, van den Bergh, Sarafoglou, Steingroever, Derks, Rouder and Morey2018; Wagenmakers, Marsman, et al., Reference Wagenmakers, Marsman, Jamil, Ly, Verhagen, Love and Morey2018).
RESULTS
Demographics and RMT
Table 1 shows the details of demographics, baseline cognitive functioning and RMT of the participants.
Table 1. Demographic data and resting motor threshold

RMT, resting motor threshold.
Working Memory Maintenance Task
MMRM analyses showed that the main effect of stimulation condition was not statistically significant for the total correct score (Table 2).
Table 2. Results for the working memory maintenance task

SEM, standard error of the mean; iTBS, intermittent theta burst stimulation; ProcTBS, prolonged continuous TBS; HD-tDCS, high-definition transcranial direct current stimulation.
Table 3 further shows the results of Bayesian repeated-measures ANOVA for these measures. “Models” lists the models under consideration. The “Null model” contains the grand mean, and the “stimulation” model adds an effect of stimulation. The most relevant is the “BF10” column; this column shows the Bayes factor for each row model against the null model. For working memory maintenance, there was moderate evidence for H0.
Table 3. Model comparison of the Bayesian repeated-measures ANOVA of D-prime on the working memory maintenance task

“Models” lists the models under consideration. The “Null model” contains the grand mean, and the “stimulation” model adds an effect of stimulation. The “P(M)” column shows the uniform distribution of prior model probabilities across all candidate models. The “P(M | data)” column shows the posterior model probabilities. The “BFM” column shows the change from prior model odds to posterior model odds. The “BF10” column shows the Bayes factor for each row model against the null model. The “error %” indicates the size of the error in the integration routine relative to the Bayes factor.
Divided Attention Task
Table 4 shows that the main effect of stimulation condition was not statistically significant for D-prime. The results of Bayesian repeated-measures ANOVA show that there was strong evidence for H0 (Table 5).
Table 4. Results for the divided attention task

SEM, standard error of the mean; iTBS, intermittent theta burst stimulation; ProcTBS, prolonged continuous TBS; HD-tDCS, high-definition transcranial direct current stimulation.
Table 5. Model comparison of the Bayesian repeated-measures ANOVA of D-prime on the divided attention task

Emotional Stroop Task
Errors (4% of trials) and outliers (1% of trials) were excluded from the analysis. Table 6 shows the means and standard deviations of RT for all conditions. MMRM analysis found a significant main effect of stimulation condition (F (3, 398) = 6.638, p < .001), but not for emotion type (F (4, 398) = 1.936, p = .104) or the interaction between stimulation and emotional type (F (12, 398) = .505, p = .911). Pairwise comparisons between each active and sham condition show that the RTs of all three active stimulation conditions were significantly faster compared to the sham condition. The effect size for iTBS was the largest (d = .32, p < .001), followed by ProcTBS (d = .21, p = .01), and the smallest for HD-tDCS (d = .15, p = .044) (see Figure 4).
Table 6. Mean reaction times and standard deviations (ms) on the emotional Stroop task across all experimental conditions

iTBS, intermittent theta burst stimulation; ProcTBS, prolonged continuous TBS; HD-tDCS, high-definition transcranial direct current stimulation.

Fig. 4. Box-and-whisker plots, with individual participant values overlaid (colored circles), showing the effect of mean reaction time on emotional Stroop task (y axis) for each experimental condition (x axis). iTBS, intermittent theta burst stimulation; ProcTBS, prolonged continuous TBS; HD-tDCS, high-definition transcranial direct current stimulation. Error bars indicate standard error of means (SEM). *significant at p < .05; **significant at p < .01; ***significant at p < .001.
Table 7 and 8 show the results of Bayesian repeated-measures ANOVA of RT on the emotional Stroop task. The results show that compared to the Null model, the Stimulation-only model received overwhelming support from the data (BF10 = 59.812, very strong evidence for H1). In contrast, both the main effect of emotion type and the interaction between stimulation and emotion type indicated substantial evidence in favor of H0.
Table 7. Model comparison of Bayesian repeated-measures ANOVA of reaction time on the emotional Stroop task

All models include subject. “Models” lists the models under consideration. The “Null model” contains the grand mean, and the “stimulation” model adds an effect of stimulation. The “P(M)” column shows the uniform distribution of prior model probabilities across all candidate models. The “P(M | data)” column shows the posterior model probabilities. The “BFM” column shows the change from prior model odds to posterior model odds. The “BF10” column shows the Bayes factor for each row model against the null model. The “error %” indicates the size of the error in the integration routine relative to the Bayes factor.
Table 8. Analysis of the effects of Bayesian repeated-measures ANOVA of reaction time on the emotional Stroop task

The “P(incl)” column shows the prior inclusion probability. The “P(incl | data)” column shows the posterior inclusion probability. The “BFInclusion” column shows the change from prior to posterior inclusion odds.
Assessment of Safety and Subjective Impression of Improvement
One participant reported severe tingling during HD-tDCS stimulation, and one participant reported moderate headache after ProcTBS. All other reported side effects were mild or moderate, consisting of tingling, itching, mild burning, pain and headache, which typically lasted between 2 and 10 min (see Supplementary material). No participant reported any nausea, light-headedness, dizziness or fatigue following the stimulation. Sixteen participants reported mild tingling, itching, burning, pain and headache during sham stimulation, and one participant reported moderate headache after sham stimulation.
In terms of subjective impression of whether one type of brain stimulation led to more improvement, 12 participants answered “No”. Of the 10 participants who responded “Yes”, 4 thought they performed best in the sham condition, 6 nominated active stimulation (iTBS, n = 3; ProcTBS, n = 3).
DISCUSSION
This study investigated the use of three newer forms of noninvasive brain stimulation (HD-tDCS, iTBS and ProcTBS) for modulating and exploring functioning of the left PPC. To the best of our knowledge, this is the first study to directly test the cognitive effects of both tDCS and TMS in a single study. Results showed that all active stimulation conditions induced faster response times compared to sham on the emotional Stroop task, thus indicating the capacity of all approaches to enhance generalized attentional processing. Contrary to our hypotheses, however, no approach was found to significantly modulate specialized cognitive processes thought to be subserved by the left PPC, namely, working memory maintenance or divided attention.
The significant cognitive-enhancing effects of all conditions on the control task as indicated by faster response times suggests that all approaches, when administered to the left PPC, can be used to cause generalized upregulation of the DAN. Although the exact neural mechanisms underpinning the effects for the different approaches remains unclear, a recent fMRI study suggested that better attentional processing after iTBS could be due to increased functional connectivity within this network (Anderkova et al., Reference Anderkova, Pizem, Klobusiakova, Gajdos, Koritakova and Rektorova2018). Of the approaches tested, the effect size for iTBS was the largest, followed by ProcTBS. The neuromodulatory effects of iTBS have been demonstrated in other brain regions such as the motor cortex (Di Lazzaro et al., Reference Di Lazzaro, Pilato, Dileone, Profice, Oliviero, Mazzone, Insola, Ranieri, Meglio, Tonali and Rothwell2008; Gamboa et al., Reference Gamboa, Antal, Moliadze and Paulus2010; Huang et al., Reference Huang, Edwards, Rounis, Bhatia and Rothwell2005) and DLPFC (Hoy et al., Reference Hoy, Bailey, Michael, Fitzgibbon, Rogasch, Saeki and Fitzgerald2016). A previous study showed that, similar to iTBS, ProcTBS administered to the motor cortex could significantly increase the amplitudes of motor-evoked potentials following the stimulation period (Gamboa et al., Reference Gamboa, Antal, Moliadze and Paulus2010). Consistent with our findings, Anderkova et al. (Reference Anderkova, Pizem, Klobusiakova, Gajdos, Koritakova and Rektorova2018) observed a trend toward decreased RT on a Stroop task following iTBS administered to the left PPC. Using an intensity of 70% active motor threshold, they found a marginal pre-post RT effect. Compared to that study, the present study used a higher stimulation intensity (80% of RMT) and included a sham control. It is possible that the stimulation intensity used in this study was more optimal for inducing modulatory effects. Indeed, a recent study which examined the intensity effects of iTBS when administered to the DLPFC suggested that the largest neurophysiological effects were obtained using 75% RMT. In that study, relatively smaller effects were found for both 50% and 100% RMT, suggesting an inverted U-shaped intensity–response association (Chung et al., Reference Chung, Rogasch, Hoy, Sullivan, Cash and Fitzgerald2018).
Compared to TMS, one limitation of conventional tDCS is its lower spatial resolution (Priori, Hallett, & Rothwell, Reference Priori, Hallett and Rothwell2009; Sellaro, Nitsche, & Colzato, Reference Sellaro, Nitsche and Colzato2016). HD-tDCS has been shown to better localize the effects of stimulation (Alam, Truong, Khadka, & Bikson, Reference Alam, Truong, Khadka and Bikson2016), which may narrow the gap of spatial resolution between tDCS and TMS. The present results showed that active HD-tDCS over the left PPC could induce a faster response compared to sham tDCS. However, the effect size of HD-tDCS was smaller than that of both iTBS and ProcTBS. This small effect size is consistent with a recent meta-analysis which similarly found faster response times on cognitive tasks using anodal tDCS in healthy participants (Dedoncker et al., Reference Dedoncker, Brunoni, Baeken and Vanderhasselt2016). By inducing an electric current inside the brain, TMS directly caused neuronal depolarization (Anderkova et al., Reference Anderkova, Pizem, Klobusiakova, Gajdos, Koritakova and Rektorova2018; Ziemann, Reference Ziemann2017). tDCS, in comparison, only changed the threshold for neuronal firing, causing increases or decreases in the rate of spontaneous neural firing (Antal et al., Reference Antal, Alekseichuk, Bikson, Brockmoller, Brunoni, Chen, Cohen, Dowthwaite, Ellrich, Flöel, Fregni, George, Hamilton, Haueisen, Herrmann, Hummel, Lefaucheur, Liebetanz, Loo, McCaig, Miniussi, Miranda, Moliadze, Nitsche, Nowak, Padberg, Pascual-Leone, Poppendieck, Priori, Rossi, Rossini, Rothwell, Rueger, Ruffini, Schellhorn, Siebner, Ugawa, Wexler, Ziemann, Hallett and Paulus2017; Nikolin et al., Reference Nikolin, Loo, Bai, Dokos and Martin2015). These underlying physiological differences may suggest that TMS is of advantage in experiments that probe the neurophysiologic effects on specific brain circuits (Priori et al., Reference Priori, Hallett and Rothwell2009). Our findings further provide empirical evidence for the larger efficacy of TBS compared to HD-tDCS. However, HD-tDCS, as compared to rTMS, has advantages in terms of adverse effect profiles, as well as the cost, availability and portability of equipment. While these advantages may position HD-tDCS as having greater translational appeal (Coffman et al., Reference Coffman, Clark and Parasuraman2014; Priori et al., Reference Priori, Hallett and Rothwell2009; Sellaro et al., Reference Sellaro, Nitsche and Colzato2016), due to the limited cognitive effects as shown in this study, further research is required to understand its mechanisms of action and optimize efficacy (Berryhill & Martin, Reference Berryhill and Martin2018).
The secondary aim of this study was to explore the use of all three stimulation methods for modulating the hypothesized specialized functions of the left PPC of maintenance, specifically working memory and divided attention. Prior neuroimaging work has reported significant IPS activity in divided attention and maintenance tasks (Santangelo & Macaluso, Reference Santangelo and Macaluso2013; Xu & Chun, Reference Xu and Chun2006). However, in our study, as all three forms of stimulation did not induce significantly enhanced performance, neither on divided attention nor on a maintenance task, we concluded that HD-tDCS, iTBS and ProcTBS over the left PPC are not suitable to modulate working memory maintenance performance or divided attention with the current study protocol. Consistent with our findings, several studies also have not found significant behavioral changes on memory tasks after PPC brain stimulation (Hamidi, Tononi, & Postle, Reference Hamidi, Tononi and Postle2008; Meier & Sauter, Reference Meier and Sauter2018; Rossi et al., Reference Rossi, Pasqualetti, Zito, Vecchio, Cappa, Miniussi, Babiloni and Rossini2006). However, using inhibitory continuous TBS to disrupt the activity of right PPC, Morgan et al. (Reference Morgan, Jackson, van Koningsbruggen, Shapiro and Linden2013) found an impaired working memory effect. Similarly, Albouy et al. (Reference Albouy, Weiss, Baillet and Zatorre2017) reported that applying theta-rhythmic rTMS over left IPS could boost the accuracy of an auditory working memory task. There are several possible explanations for these conflicting findings. Firstly, the PPC may play a generalized role in attention processing but not a critical role in either divided attention or working memory maintenance. Rossi et al. (Reference Rossi, Pasqualetti, Zito, Vecchio, Cappa, Miniussi, Babiloni and Rossini2006) found that the interference of rTMS over the left and right PPC only influenced RTs in a purely attentive visuospatial task but not encoding or retrieval performance. It is well established that the PPC is a major node of the DAN (Capotosto et al., Reference Capotosto, Baldassarre, Sestieri, Spadone, Romani and Corbetta2017; Corbetta & Shulman, Reference Corbetta and Shulman2002), which is proposed to mediate the processing of visual information and the top-down guided allocation of attention to location or features (Vossel et al., Reference Vossel, Geng and Fink2014). In line with this, significant faster response times on the emotional Stroop task were found in the present study. A recent study also observed a similar behavioral effect after iTBS over the left PPC, which was associated with a significant increase of the resting-state connectivity of the DAN (Anderkova et al., Reference Anderkova, Pizem, Klobusiakova, Gajdos, Koritakova and Rektorova2018). Taken together, these results thus suggest network rather than regional effects following the stimulation of the left PPC.
The second reason for the inconsistent findings may be that the IPS activity involved in maintenance and divided attention functioning is bilateral (Santangelo & Macaluso, Reference Santangelo and Macaluso2013; Xu & Chun, Reference Xu and Chun2006). Therefore, focalized stimulation over one hemisphere (i.e., left) may not be sufficient to modulate performance. Using bilateral tDCS, Jacobson et al. (Reference Jacobson, Goren, Lavidor and Levy2012) found a significant improvement in the ability to discriminate studied from unstudied words. Further, there is some evidence to suggest that, compared to the left PPC, anodal tDCS over the right PPC may induce stronger effects on attentional performance (Filmer et al., Reference Filmer, Dux and Mattingley2015; Roy, Sparing, Fink, & Hesse, Reference Roy, Sparing, Fink and Hesse2015; Sarasso et al., Reference Sarasso, Ninghetto, Salatino, Ronga, Bongiardina, Iarrobino, Neppi-Modona and Ricci2019; Scheldrup et al., Reference Scheldrup, Greenwood, McKendrick, Strohl, Bikson, Alam, McKinley and Parasuraman2014). Some rTMS studies have also shown that the right PPC is important for visual attention functions (Fierro et al., Reference Fierro, Brighina, Oliveri, Piazza, La Bua, Buffa and Bisiach2000; Sack et al., Reference Sack, Kohler, Bestmann, Linden, Dechent, Goebel and Baudewig2007; Salatino, Poncini, George, & Ricci, Reference Salatino, Poncini, George and Ricci2014). This could be the reason why stimulating the left PPC did not induce significant behavioral change on the divided attention task, which contained a visual attentional component. Further work is required to determine whether either bilateral or right-sided stimulation may be more effective.
Lastly, compared to the delayed recognition and the discrimination task modulated in previous studies (Albouy et al., Reference Albouy, Weiss, Baillet and Zatorre2017; Hamidi et al., Reference Hamidi, Slagter, Tononi and Postle2009; Hamidi et al., Reference Hamidi, Tononi and Postle2008), the dual three-back and maintenance tasks used in this study were more demanding (i.e., in relation to working memory load). The potential for noninvasive brain stimulation to improve performance accuracy could be limited for such complex abilities in healthy participants using current approaches. In line with this, a previous study found that tDCS induced improvement only in the two-back working memory task, but not in the three-back task for RT only (i.e., not for performance accuracy) (Hoy et al., Reference Hoy, Emonson, Arnold, Thomson, Daskalakis and Fitzgerald2013). In addition, a meta-analysis of tDCS cognitive effects in healthy participants found only an overall small-sized effect for RT, though no effect for performance accuracy (Dedoncker et al., Reference Dedoncker, Brunoni, Baeken and Vanderhasselt2016). Alternatively, multifocal stimulation or different stimulation parameters may be required. A recent study revealed that network-targeted tDCS could double motor cortex excitability compared to traditional tDCS (Fischer et al., Reference Fischer, Fried, Ruffini, Ripolles, Salvador, Banus, Ketchabaw, Santarnecchi, Pascual-Leone and Fox2017). It would be interesting to explore the efficacy of using such multifocal stimulation approaches for modulating cognition in future studies. Further, as the present study only examined the effects from a single session of stimulation, the potential for effects from multiple repeated stimulation sessions cannot be ruled out.
Several limitations to this study need to be acknowledged. First, the timing of stimulation relative to task presentation was different between the HD-tDCS and TBS protocols. For tDCS, the timing of brain stimulation delivery in relation to task execution has been identified as an important factor for cognitive effects, with stimulation given during performance associated with better efficacy (Martin, Liu, Alonzo, Green, & Loo, Reference Martin, Liu, Alonzo, Green and Loo2014). In the current study, cognitive testing was commenced 5 min after HD-tDCS stimulation onset. Given the evidence that tDCS cognitive effects may take longer to take effect (e.g., Giglia et al., Reference Giglia, Mattaliano, Puma, Rizzo, Fierro and Brighina2011; Teo, Hoy, Daskalakis, & Fitzgerald, Reference Teo, Hoy, Daskalakis and Fitzgerald2011), this timing may have been suboptimal. These differences might, in part, explain the discrepancy in effect sizes between brain stimulation modalities. Secondly, the present study only administered a HD-tDCS sham condition. There was no TBS sham equivalent. Compared to tDCS, which utilizes a smaller and soundless stimulator, rTMS may have been perceived by participants as more impressive (i.e., the coil, stimulator, sound produced by the TBS trains and possible muscle movement during stimulation), which could have influenced their performance. It is important to note, however, that significant performance improvement was found only on one task, and 4 of 10 participants who thought brain stimulation improved their performance were in the sham HD-tDCS condition. Nevertheless, to minimize this potential bias in future studies, the use of sham rTMS should be considered. Third, because it is unclear which specific region within the left PPC might contribute to divided attention and maintenance, the present study used a circular coil, which could deliver stimulation to the broader cortical region of the left PPC. However, a limitation to the use of this coil is that other frontoparietal sensorimotor regions may have also been stimulated. Hence, the current observed effects with TBS may not be specific to the activation of left PPC but to other more widespread frontoparietal networks as well. Future research could examine the contribution of specific subregions within the PPC by using a figure-of-8 coil and neuronavigation equipment. Finally, the present study did not investigate neurophysiological changes induced by different forms of stimulation. Compared to behavioral change, markers of brain function such as TMS-evoked potentials might be more sensitive to show differences in the outcomes of brain stimulation and should be considered in future studies.
In conclusion, this study revealed that iTBS, ProcTBS and HD-tDCS, when administered to the left PPC, could induce faster response times compared to sham stimulation on the emotional Stroop task, suggesting improved generalized attentional processing. As the first study to compare tDCS and rTMS in a single study, the findings further suggest that rTMS may have greater efficacy for modulating cognition. None of the forms of stimulation were found to significantly modulate working memory maintenance or divided attention. Future research is thus required to confirm whether the left PPC plays a specialized role in subserving these abilities.
ACKNOWLEDGMENTS
We thank all the participants for their contributions to this study. This work was conducted while Tian Gan was visiting the School of Psychiatry at the University of New South Wales supported by the China Scholarship Council (No. 201708330020).
CONFLICT OF INTEREST
The authors have nothing to disclose
SUPPLEMENTARY MATERIAL
To view supplementary material for this article, please visit https://doi.org/10.1017/S1355617719000766