Introduction
Human activities in Antarctica can cause both immediate and cumulative impacts on the environment (Naveen Reference Naveen1996, Convey Reference Convey2020). Coastal areas in particular have suffered the impacts of the establishment of national scientific research stations since the mid-twentieth century, on both the Antarctic Peninsula and Continental Antarctic (Vodopivez et al. Reference Vodopivez, Curtosi, Villaamil, Smichowski, Pelletier and Mac Cormack2015), as well as from the earlier marine exploitation industries targeting seals and whales in the Scotia Arc archipelagos and the sub-Antarctic islands (Convey & Lebouvier Reference Convey and Lebouvier2009). Increasing research activities in Antarctica, especially after the International Geophysical Year (1958–1959), have led to a range of impacts, including anthropogenic contamination in the environment, particularly in the limited available ice-free areas (Santos et al. Reference Santos, Silva-Filho, Schaefer, Albuquerque-Filho and Campos2005, Brooks et al. Reference Brooks, Jabour, Van Den Hoff and Bergstrom2019). More recently, Antarctic tourism, predominantly based on cruise ships, has shown exponential growth since the late 1970s, when there were only a few hundred visitors each year (Naveen Reference Naveen1996), to a total of 56 168 tourists in the 2018/2019 summer (https://iaato.org/wp-content/uploads/2020/03/IP140-IAATO-Overview-of-Antarctic-Tourism-2018-19-Season-and-Preliminary-Estimates-for-2019-20-Season.pdf). Although there is currently a hiatus in both national research activity and tourism in Antarctica resulting from the COVID-19 pandemic, leading to a temporary reduction in pressure on the Antarctic environment, both are expected to recover in future years (Hughes & Convey Reference Hughes and Convey2020).
Human activities can contaminate soils with heavy metals. However, as heavy metals are also natural components of the Earth's crust, it can be difficult to distinguish between their natural and anthropogenic sources. The main natural sources of such metals in Antarctica are rock and soil dust, volcanic emissions, sea spray, marine biogenic activity and atmospheric aerosols (Bockheim Reference Bockheim2015). Anthropogenic chemical contamination is typically associated with historic and currently occupied research stations, field camps, former industrial sites and other regularly used locations. Elevated levels of heavy metals including lead (Pb), chromium (Cr), arsenic (As), zinc (Zn), cadmium (Cd), copper (Cu), mercury (Hg) and nickel (Ni) are commonly found at contaminated sites (Cabrita et al. Reference Cabrita, Padeiro, Amaro, dos Santos, Leppe and Verkulich2017). Soils are the major sink for heavy metals released by human activity into the environment. For instance, they may be contaminated by solid waste, fuel residues and domestic wastewater (de Lima Neto et al. Reference de Lima Neto, Guerra, Thomazini, Daher, de Andrade and Schaefer2017). Batteries, rubbish and buildings are point sources of metals (Pb, Zn and Cu) in soils, while burning fuel can lead to widespread contamination by Pb, as documented in the McMurdo Sound region of Victoria Land (Claridge et al. Reference Claridge, Campbell, Powell, Amin and Balks1995). Metals do not undergo microbial or chemical degradation and, in the absence of leaching, they can persist for very long periods after their introduction (Wuana & Okieimen Reference Wuana and Okieimen2011). However, changes in their bioavailability and chemical forms can occur (Wuana & Okieimen Reference Wuana and Okieimen2011). Heavy metal contamination may, therefore, threaten the soil ecosystem. Zhao & Xu (Reference Zhao and Xu2000) reported that the contents of Cr, Cu, Mn, Ni, Pb and Zn in lichens close to a power generation station and fuel storage depot in Fildes Peninsula, King George Island (South Shetland Islands), were considerably higher than in lichens from a more distant control site, with Pb content being ~30 times greater.
Heavy metals include both biologically essential and non-essential elements. Exposure to all heavy metals at high concentrations is toxic to microalgae, although the extent of toxicity and the level at which an element shows toxic effects differ between essential and non-essential metals. Low amounts of essential metals are required for the normal growth of microalgae, but at higher levels these metals can become toxic, with the boundary between deficiency and toxicity being extremely narrow (Clemens Reference Clemens2006). For instance, Afkar et al. (Reference Afkar, Ababna and Fathi2010) reported that a lower concentration of three tested metals (1 × 10−9 M Cu, Co or Zn) had a stimulatory effect on the biomass yield of Chlorella vulgaris cultured at 22°C for 7 days, while higher concentrations exerted inhibitory effects (1 × 10−8 to 1 × 10−6 M). Total protein and carbohydrate contents and the total free amino acids in C. vulgaris gradually decreased with increasing metal concentration in the medium. The adverse impacts of heavy metals on algae may cascade through food webs to affect organisms at higher trophic levels, consequently disrupting food chains. Biomagnification through accumulation may also occur (Chu et al. Reference Chu, Dang, Kok, Yap, Phang and Convey2019a).
Antarctic ecosystems are particularly sensitive to anthropogenic modifications (Santos et al. Reference Santos, Silva-Filho, Schaefer, Albuquerque-Filho and Campos2005). Ice-free land occupies ~0.2–0.4% of the continent's area, and most human activities are concentrated in these areas, leading inevitably to conflict or competition with the native terrestrial ecosystems as well as with the concentrations of marine vertebrates that often breed there (Santos et al. Reference Santos, Silva-Filho, Schaefer, Albuquerque-Filho and Campos2005, Tin et al. Reference Tin, Fleming, Hughes, Ainley, Convey and Moreno2009). A local decline in the lichen community has been documented in a permanent monitoring plot close to Svea Station in Dronning Maud Land, Continental Antarctica, over a 10 year period (Johansson & Thor Reference Johansson and Thor2008). Increased growth of the native nitrophilous alga Prasiola crispa around Syowa Station, East Antarctica, due to soil eutrophication influenced by human activities has also been reported (Ohtani et al. Reference Ohtani, Suyama, Yamamoto, Aridomi, Itohm and Fukuoka2000). The diversity and importance of microalgae in Antarctic terrestrial and freshwater ecosystems have long been appreciated.
Signy Island (60°43'S, 45°38'W), a small island in the South Orkney Islands, lies in the South Atlantic sector of the Southern Ocean 600 km north-east of the northern extremity of the Antarctic Peninsula (Broady Reference Broady1979). It is composed of regionally metamorphosed sediments, mainly quartz-mica schists, with subordinate amphibolites and marbles. Some local soils are derived from in situ weathering of schist and marble, but most of the island's mineral soil is of local glacial origin. Solifluction processes play a major part in soil formation (Holdgate et al. Reference Holdgate, Allen and Chambers1967).
While several earlier studies reported aspects of the distribution of algae on Signy Island (Broady Reference Broady1979, Reference Broady1996, Hawes Reference Hawes1983, Davey Reference Davey1991), there have been no recent studies on the taxonomy of the island's algae or on the influence of trace elements on microalgal occurrence. The influences of moisture, nutrient availability, pH and salinity on algal community composition and distribution in the island's terrestrial habitats have been recognized (Arnold et al. Reference Arnold, Convey, Hughes and Wynn-Williams2003). Broady (Reference Broady1979) differentiated between subaerial and aerial habitats in his assessment of factors influencing algal distribution on the island. Davey (Reference Davey1991) investigated the influence of seasonal variation in irradiance, soil temperature, snow/ice cover and soil water content on algal communities. The present study set out to examine the relationship between heavy metal presence and algal occurrence across a range of human-impacted and natural environmental locations on Signy Island.
Materials and methods
Study area
Signy Island has a total surface area of ~20 km2, with a longest axis (from north to south) of 8 km, a maximum width of 5 km and a highest elevation of 280 m. The northern point of Signy Island is separated by 1.5 km from the much larger and more heavily glaciated Coronation Island, the largest island of the South Orkney group. Soil samples were collected between January and April 2015 from a range of differently impacted sites. Soil surface samples (0 to 5–8 cm depth) of ~50 g were collected using sterile 50 ml Falcon tubes and immediately sealed with Parafilm before being transported to the laboratory at the British Antarctic Survey (BAS) research station on Signy Island. A total of 175 soil samples were obtained from five sampling locations (35 samples from each location), namely Berntsen Point, Gourlay Peninsula, Jane Col, North Point and Pumphouse Lake (Fig. 1).

Fig. 1. Sampling locations on Signy Island. The five sampling locations, namely Berntsen Point, Gourlay Peninsula, Jane Col, North Point and Pumphouse Lake, are represented by yellow circles and the location of Signy research station on Berntsen Point is represented by a red triangle.
A general description of the five sampling locations is given in Table I. Berntsen Point is a low-altitude vegetated fellfield area where the BAS and predecessor organizations have maintained a research station since the mid-1940s, on a site previously occupied by a small whaling station constructed in the 1920s. Gourlay Peninsula and North Point host large penguin rookeries, with the former also hosting elephant and fur seals and the latter other breeding seabird species. Jane Col is a higher-altitude location typified by largely bare ground. A fuel store (coal), pumphouse and pipeline constructed to supply water for the whaling station were located close to the shoreline of Pumphouse Lake, and corroded metal engine parts and traces of coal remain visible around the lake. These sites represent human impact (past and ongoing for Pumphouse Lake and Berntsen Point, respectively), intense animal impact (Gourlay Peninsula and North Point) and minimal impact (Jane Col). Soil surface temperatures recorded at the time of collection ranged between 2°C and 5°C, as is typical of summer soil temperatures across the Maritime Antarctic (Convey et al. Reference Convey, Coulson, Worland and Sjöblom2018).
Table I. General description of the sampling locations on Signy Island.

Culturing of microalgae from Signy Island
The soil samples collected on Signy Island were stored frozen (-80°C) on station and during transport from Signy Island to the BAS in Cambridge, UK (~2 months). The samples were then transported by air to Malaysia under dry ice.
In the laboratory, ~0.5 g of each soil sample was transferred into one well of a 24 well plate, using a total of eight plates to cover all of the soil samples. These were grown in Bold's Basal Medium (BBM) at 4 ± 2°C illuminated with fluorescent light at 42 μmol/m2/s in a 12:12 h light-dark cycle for 3–4 weeks. Microalgae that grew in the wells were examined using light microscopy and identified based on morphological features as described by Broady (Reference Broady1976), Broady et al. (Reference Broady, Garrick and Anderson1984), Broady & Ingerfeld (Reference Broady and Ingerfeld1993) and Ohtani et al. (Reference Ohtani, Suyama, Yamamoto, Aridomi, Itohm and Fukuoka2000). The diacritical characteristics used for algal identification are given in Supplemental Table S1.
Metal analyses
The labile or bioavailable forms of metals from the soil samples were quantified using inductively coupled plasma mass spectrometry (ICP-MS). A total of 174 soil samples from the five sampling locations were examined, with one being unsuitable for analysis as it contained a single piece of rock. All plasticware used was acid washed in 20% (v/v) analytical reagent (AR)-grade HNO3 (65% w/w; EMSURE®) and then soaked for > 24 h and triple rinsed in deionized water (611 DI Sartorius Arium, Millipore Molsheim, France) before use. The 1 M HCl solution was prepared from AR-grade concentrated HCl (37% w/w; EMSURE®). Deionized water with a resistivity of ≥ 18 MΩ cm-1 was used to prepare all standard and sample solutions (Snape et al. Reference Snape, Scouller, Stark, Stark, Riddle and Gore2004).
Metal ions were extracted using HCl (Santos et al. Reference Santos, Silva-Filho, Schaefer, Albuquerque-Filho and Campos2005). Each soil sample (1 g) was extracted using 20 ml of 1 M HCl (i.e. 1:20 w/v) for 4 h on an orbital platform shaker. The sample was then centrifuged (3000 × g, 10 min) and the supernatant was filtered through a 0.45 μm nylon syringe filter (Sartorius) and stored in a 50 ml plastic centrifuge tube until analysis. The samples were analysed using ICP-MS (Perkin-Elmer-SCIEX, Thornhill, ON, Canada) for a suite of 14 elements, namely Al, As, Ca, Cd, Co, Cr, Cu, Fe, Hg, Mg, Mn, Ni, Pb and Zn (Snape et al. Reference Snape, Scouller, Stark, Stark, Riddle and Gore2004).
A multi-elemental calibration standard stock solution (Pure Plus, PerkinElmer) with the elements to be analysed was prepared with the following concentrations: 0, 5, 10, 50, 100 and 200 ppb. A test solution containing 200 ppb of yttrium (Y) and lanthanum (La) was used as an internal standard to correct for instrumental drift and possible matrix effects. All of the solutions were dissolved in 2% (v/v) HNO3 (Ródenas de la Rocha et al. Reference de la Rocha, Sánchez-Muniz, Gómez-Juaristi and & Marín2009). High-purity argon gas (> 99.996%) was used as a plasma source. The data obtained were analysed using ELAN® version 3.4 software (PerkinElmer).
Statistical analyses
Differences between the microalgal communities obtained from the five sampling locations were determined using permutational multivariate analysis of variance (PERMANOVA). Before calculating the similarity matrix, the microalgal occurrence data were expressed as presence or absence. A non-metric multidimensional scaling (nMDS) ordination of a zero-adjusted Bray-Curtis similarity matrix (dummy variable with value 1 was added) was performed to identify groups of samples with similar taxonomic compositions (Clarke & Warwick Reference Clarke and Warwick2001). Similarity percentages (SIMPER) analysis was used to calculate the contribution of the individual microalgae to site similarity or dissimilarity, based on the Bray-Curtis dissimilarity metric, with the cut-off set at 70% for low contributions (Lone et al. Reference Lone, Hamid and Bhat2021).
Differences in soil metal contents between the five sampling locations were analysed using one-way analysis of variance (ANOVA). Where significant, the analysis was followed with post hoc multiple comparisons using the Student-Newman-Keuls (S-N-K) test. The Levene test was used to check for homogeneity of variance before ANOVA was performed. Where the data violated the requirement for homogeneity of variance, a non-parametric Kruskal-Wallis test was performed instead of ANOVA. P < 0.05 was considered statistically significant.
A principle component analysis (PCA) was performed on the heavy metal data to identify any patterns in the occurrence of the targeted metals in the 174 soil samples. The raw concentration data were normalized, except for the Cd and Hg data, which were log(x + 1)-transformed to generate a normal distribution, before performing the PCA (Sun et al. Reference Sun, Skidmore, Wang and Zhang2019). Relationships between the occurrence of the different metals in the soil samples were analysed using Pearson's correlation coefficient (de Lima Neto et al. Reference de Lima Neto, Guerra, Thomazini, Daher, de Andrade and Schaefer2017).
The contamination factor (CF) and pollution load index (PLI) are widely used to evaluate the degree of heavy metal pollution in soils (Bhuiyan et al. Reference Bhuiyan, Parvez, Islam, Dampare and Suzuki2010). The CF is the ratio estimated by dividing the concentration of each metal in the soil by the baseline or background value. For the purpose of this study, the metal concentrations in the soil samples from Jane Col (the site least impacted from any source) was taken as the background value. The extent of contamination was categorized as follows: CF < 1 = low contamination, 1 ≥ CF ≥ 3 = moderate contamination, 3 ≥ CF ≥ 6 = considerable contamination and CF > 6 = very high contamination (Harikumar & Jisha Reference Harikumar and Jisha2010).
The PLI is an empirical index that provides a simple comparative means for assessing the level of heavy metal pollution. The PLI is expressed as:

Where n is the number of metals studied and CF is the contamination factor calculated for each metal as described above. PLI < 1 indicates no pollution, PLI = 1 indicates baseline levels of pollutants are present and PLI > 1 indicates significant pollution (Tomlinson et al. Reference Tomlinson, Wilson, Harris and Jeffrey1980).
The relationship between microalgal communities and heavy metal presence was investigated using distance-based linear modelling (DistLM) (Anderson et al. Reference Anderson, Gorley and Clarke2008).
The ANOVA, post hoc (S-N-K), Levene and Kruskal-Wallis tests and Pearson's correlation coefficient assessments were performed using SPSS v.25 (IBM Corporation). All PERMANOVA, nMDS, SIMPER, PCA and DistLM analyses (Clarke & Gorley Reference Clarke and Gorley2015) were performed using PRIMER v.7 (PRIMER-e Ltd, Plymouth, UK).
Results
Microalgal community composition and diversity
A total of 29 taxa of microalgae were identified in cultures derived from the 175 soil samples collected from Signy Island (Table II). The light micrographs of microalgae are presented in Supplemental Figs S1–S4. The majority of these were Chlorophyta, followed by Cyanobacteria. The greatest algal diversity was recorded from North Point (20 taxa), while Pumphouse Lake had the lowest (11 taxa). Representatives of Bacillariophyta, Chlorophyta, Cyanobacteria and Tribophyta were cultured from soil samples from Berntsen Point, Gourlay Peninsula and North Point. Bacillariophyta were not obtained from Jane Col and Tribophyta were not obtained from Pumphouse Lake. The highest diversity of Chlorophyta was present in samples from Jane Col and North Point (10 taxa), while the highest diversity of cyanobacteria was obtained from Gourlay Peninsula and North Point (5 taxa). Generally, there was a low diversity of Bacillariophyta at most sampling locations except North Point (4 taxa).
Table II. The number (n) and frequency (F) of observations of each algal taxon identified in cultures from each of the five sampling locations on Signy Island.

The most widespread species was Desmococcus olivaceus, which was obtained from 68 samples. A maximum of 11 taxa were obtained from a single sample. Pseudococcomyxa sp. was only recorded from Berntsen Point, Gloeocapsa sp. only from Gourlay Peninsula and Nostoc commune only from Pumphouse Lake. Four taxa were obtained only from Jane Col (Tetracystis sp., Chlorella reisiglii, Koliella sp. and Gloeobotrys sp.), and four were only obtained from North Point (Navicula sp., Pinnularia notata, Chlorella sp. 1 and Stichococcus sp. 2). Botrydiopsis constricta was the dominant species at Berntsen Point, while Oscillatoria boryana was dominant in both animal-impacted sites (Gourlay Peninsula and North Point). Desmococcus olivaceus was dominant at Pumphouse Lake.
PERMANOVA confirmed that the microalgal community composition differed significantly (F = 8.110, P = 0.001) between the five sampling locations (Supplemental Table S2). The nMDS ordination presented in Fig. 2 illustrates the differences in microalgal community composition across the five sampling locations. The ordination indicates differences between the sites, although distinct groups were not formed, and some sampling locations clustered together.

Fig. 2. Non-metric multidimensional scaling ordination (nMDS) for microalgal occurrence associated with the five sampling locations on Signy Island: Berntsen Point (BP), Gourlay Peninsula (GP), Jane Col (JC), North Point (NP) and Pumphouse Lake (PL).
SIMPER was applied to compare the information obtained from nMDS and to help identify the algal groups underlying differences between the sampling locations. The SIMPER results (Supplemental Table S3) indicated that the maximum average dissimilarity (96.00%) was found between Gourlay Peninsula and Jane Col, with the top three algal contributors being O. boryana (17.63%), D. olivaceus (15.80%) and Coccomyxa gloeobotrydiformis (8.44%). Jane Col and North Point exhibited the next greatest average dissimilarity (94.65%), with the top three contributors being O. boryana (14.01%), D. olivaceus (13.85%) and Oscillatoria raciborskii (9.10%). Within each sampling location (Table III), the highest average similarity of algae community was observed at Pumphouse Lake (SIMPER average similarity = 38.01%), with the top contributor being D. olivaceus (75.44%). This species was also a major contributor to the within-group similarity at all five sampling locations, contributing 20.25–75.44%.
Table III. Average abundance and similarity values of algae within each sampling site on Signy Island.

Soil heavy metal contents
The bioavailable concentrations of 14 heavy metals (Al, As, Ca, Cd, Co, Cr, Cu, Fe, Hg, Mg, Mn, Ni, Pb and Zn) in the Signy Island soils sampled are presented in Table IV. The metal concentrations varied spatially and differed significantly (ANOVA, all P < 0.05) between sampling sites, although there was little evidence of consistent patterns across the different metals between the sites. The most abundant bioavailable metals were Al, Ca and Fe. The Al, Fe and Mn levels were significantly higher at Pumphouse Lake compared to the other sites. High levels of As, Ca, Cd, Cu and Zn were detected at the strongly animal-impacted locations of Gourlay Peninsula and North Point, with those at Gourlay Peninsula being significantly higher than at the other sampling sites. The highest Cr and Ni levels were found in samples from Jane Col. Co levels were lower at Berntsen Point and Gourlay Peninsula compared to the other sampling sites, while Hg and Pb levels were higher at Berntsen Point than elsewhere. Thirteen of the 35 samples obtained from Berntsen Point contained high levels of Pb (12.270–127.643 μg/g), with these specific samples obtained from areas near to the storage hut of the research station. The Pb content of samples from Pumphouse Lake was generally low, with the exception of a single sample that contained 211.054 μg/g. This sample had no visible association with any evidence of human contamination, but its Pb content was the highest recorded in any sample. The highest levels of Mg were detected at Gourlay Peninsula and the lowest at Berntsen Point, while the highest Mn level was obtained from Pumphouse Lake.
Table IV. Metal contents (μg g-1 dry mass) of soil samples from the five sampling locations on Signy Island. Both full range and mean ± SD are presented for each location.

a,b,c,d Significant differences between mean values. Sites with different letters are significantly different (P < 0.05).
Table V. Pearson correlation matrix for heavy metal concentrations in soils from Signy Island, Antarctica.

*P < 0.05, **P < 0.01.
PCA was used to analyse the patterns in the metal ion concentration data across the 174 soil samples (Fig. 3). The first two principal components (PCs) explained 34.4% and 17.2% of the total variance. Three main groups of metal ions could be identified: 1) Al and Fe, 2) As, Ca, Cd, Cu, Mg and Zn and 3) Hg and Pb. Most of the Gourlay Peninsula samples and some North Point samples had a strongly negative value on PC1, while Berntsen Point, Jane Col and Pumphouse Lake samples had positive values on PC1. Pumphouse Lake and Jane Col samples had strongly negative values on PC2. PERMANOVA indicated that the differences in metal contents between sampling locations were statistically significant (F = 17.333, P = 0.001) (Supplemental Table S4).
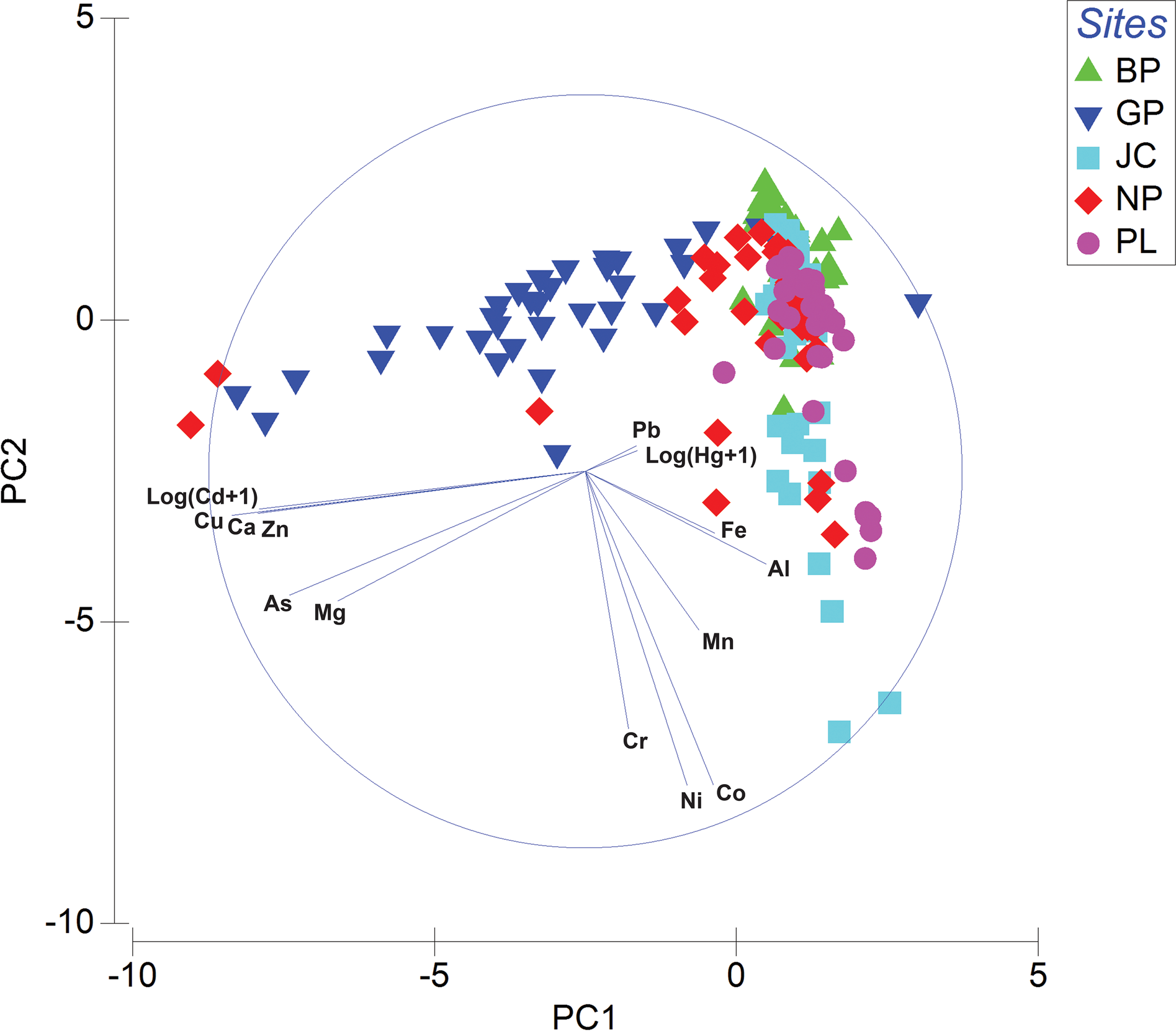
Fig. 3. Biplot of the first two principal components (PC1 and PC2) in the principal component analysis of metal ion concentration data in 174 soil samples collected from five sampling locations on Signy Island: Berntsen Point (BP), Gourlay Peninsula (GP), Jane Col (JC), North Point (NP) and Pumphouse Lake (PL). The vector length and direction reflect the importance of the contribution of each variable to each of the two axes. The Pearson correlation matrix was calculated for the 14 metal ion concentrations from the 174 soil samples (Table V). Thirty-eight of the 91 correlations were statistically significant at P < 0.01 and 10 of the correlations were statistically significant at P < 0.05. There were strong positive correlations of: Al with Fe; Cu with As, Ca, Cd and Zn; As with Cd; Ca with Zn; and Co with Ni. Negative correlations were statistically significant in 14 of 91 instances at P < 0.01 and 10 of 91 instances at P < 0.05.
The average CF values calculated from the 35 samples obtained at each of the four more impacted sampling locations and the baseline location at Jane Col are listed in Table VI. The CF values for Pb were relatively high at Berntsen Point and Pumphouse Lake, while Cd, Cu and Zn values were high at Gourlay Peninsula and North Point. The mean CF values for the metal ions at the human-impacted Berntsen Point decreased in the order Pb > Hg > Cd > Zn > As > Fe > Al > Cu > Ca > Ni > Co > Mg > Cr > Mn. At the animal-impacted Gourlay Peninsula the order was Cd > Zn > Cu > Ca > As > Mg > Fe > Al > Cr > Pb > Hg > Mn > Ni > Co.
Table VI. Mean (and SD) contamination factors of the studied metal ions in soil samples from the four human-impacted or animal-impacted sites on Signy Island, calculated relative to the baseline minimally impacted location at Jane Col.

a,b,c,d Significant differences between mean values. Sites with different letters are significantly different (P < 0.05).
The PLI values from both the animal-impacted locations of Gourlay Peninsula (1.67) and North Point (1.31) confirmed that the sites are polluted (Fig. 4). The relatively low PLI values of Berntsen Point (0.66) and Pumphouse Lake (0.93) suggest that these locations are less polluted overall or unpolluted.

Fig. 4. Pollution load index of heavy metals in soil samples from Signy Island. Error bars indicate standard deviation.
Relationship between microalgal communities and soil heavy metal contents
DistLM was used to calculate correlations and the explanatory contribution between each heavy metal and variation in microalgal communities across the five sampling locations (Table VII). Nine of the 14 metals had a significant influence on microalgal community variation, in the decreasing order Mn > Ca > Mg > Fe > Zn > Cd > Co > Cr > Cu. However, while significant, each of the ions individually only contributed 1.32–2.46% of the microalgal community variation, and the total variation explained by all metal ions was 16.11%.
Table VII. Correlations between heavy metal levels and variation in microalgal community composition across the five sampling locations on Signy Island. Data were assessed by marginal tests using distance-based linear modelling, and the significance of the relationship (P) and the proportion of the variability in the multivariate dataset explained by each metal are presented.

*P < 0.05, **P < 0.01, ***P < 0.001.
Discussion
This is the first study to report heavy metal contents and their relationship with microalgal community composition in soils from locations with different levels of human or marine vertebrate impact on Signy Island in the Maritime Antarctic. A total of 29 microalgal taxa, representing Bacillariophyta, Chlorophyta, Cyanobacteria and Tribophyta, were recorded in cultures derived from a total of 175 soil samples collected from five distinct locations on Signy Island. The most common microalgae found were D. olivaceus, Chlamydomonas sp. and Chloromonas sp., with D. olivaceus being the most abundant species. Desmococcus olivaceus is a common Antarctic soil alga that is present in both the Maritime Antarctic and Continental Antarctic (Broady & Ingerfeld Reference Broady and Ingerfeld1993, Broady Reference Broady2005). The species is distributed globally and is commonly found on various surfaces such as rocks, soil and tree trunks, including polluted habitats (Laundon Reference Laundon1985), and it has been described as ‘the commonest green alga in the world’.
In this study, only one type of culture medium was used, and we recognize the potential role of the selectivity of the culture medium for particular taxa in influencing the diversity found. The abundance of green microalgae in this study could indicate the optimal growth conditions provided by BBM media for these species. Broady (Reference Broady1979) used three techniques to examine the distribution of microalgae from Signy Island (direct microscopic examination, moist plate enrichment culture and culture in mineral salts medium) and suggested that, to obtain the most complete assessment of diversity, all three methods should ideally be used. We also recognize that algae that are difficult or impossible to grow in any given culture medium will not be identified.
Diatoms (Bacillariophyta) are one of the most abundant algal groups in Antarctic and sub-Antarctic regions. In the present study, the diatoms recorded were members of the genera Navicula and Pinnularia, with two representatives of each genus. These genera are commonly reported in soils, freshwaters and saline lakes in the sub-Antarctic and Antarctic (Sabbe et al. Reference Sabbe, Verleyen, Hodgson, Vanhoutte and Vyverman2003, Van de Vijver Reference Van de Vijver2008, Zidarova et al. Reference Zidarova, Kopalová and Van de Vijver2016). The most abundant diatom genus found in the present study was Navicula, which was particularly prominent in the animal-impacted locations of Gourlay Peninsula and North Point. In comparison with some other studies from Signy Island, this study reported a low number of diatom taxa. For instance, Broady (Reference Broady1979) reported 30 terrestrial diatom taxa, of which 28 were identified using direct microscopic examination, a more appropriate technique for the identification of diatoms as compared to culturing in BBM. The 12 soil diatom taxa reported by Mataloni et al. (Reference Mataloni, Tell and Wynn-Williams2000) were similarly mainly detected via direct microscopic examination. The BBM growth medium used in this study does not contain silicon (Si), which is an essential inorganic nutrient required for diatom growth (Gilpin et al. Reference Gilpin, Davidson and Roberts2004); hence, the low number of diatoms could also be attributed to this limiting factor.
Cyanobacteria are present in most soil habitats globally. Oscillatoria sp., Phormidium autumnale and Pseudanabaena sp. identified in this study have been recorded previously from Signy Island (Davey & Rothery Reference Davey and Rothery1993, Chong et al. Reference Chong, Pearce, Convey, Tan, Wong and Tan2010). Nostoc commune and Phormidium sp. also occur across many different climate zones globally (Wynn-Williams Reference Wynn-Williams1996, Rahmonov et al. Reference Rahmonov, Cabala, Bednarek, Rozek and Florkiewicz2015). Davey & Clarke (Reference Davey and Clarke1991) reported that representatives of Nostoc and Phormidium dominated frost-sorted soil polygons at Jane Col, but these taxa were not identified from the samples from Jane Col in the current study. Davey & Clarke (Reference Davey and Clarke1991) used direct microscopic examination for the identification of algae and reported that incubation of soil samples in growth media led to little change in the diversity of soil flora detected, but that the coverage of most taxa declined during the period of incubation. Fifteen species of cyanobacteria were reported by Mataloni et al. (Reference Mataloni, Tell and Wynn-Williams2000), also via direct microscopic examination. It is recognized that many cyanobacteria are difficult to culture (Broady Reference Broady1996), while culture-based approaches are also limited by the high degree of phenotypic difference between cyanobacteria in the cultured and free-living states (Velichko et al. Reference Velichko, Smirnova, Averina and Pinevich2021). The only cyanobacteria detected at Jane Col was Pseudanabaena sp., again differing from the study of Davey & Rothery (Reference Davey and Rothery1993), which reported five cyanobacterial taxa from this location.
PERMANOVA and nMDS analysis indicated that the microalgal communities across all five sampling locations on Signy Island were significantly different. The SIMPER results indicated that the highest average dissimilarity was found between the animal-impacted sites (Gourlay Peninsula and North Point) and the minimally impacted Jane Col, with the main contributors being O. boryana and D. olivaceus. Jane Col consists mostly of bare soil and rock surfaces and is minimally impacted compared to the other sampling locations. The microalgal community at Jane Col may consist of taxa that can grow well under typical fellfield conditions and are able to survive desiccation (Davey & Clarke Reference Davey and Clarke1991). Despite this, many other members of the microalgal community at this site were shared with the other sampling locations.
Analysis of the soil metal contents showed that high levels of As, Ca, Cd, Cu and Zn were detected in both of the animal-impacted areas at Gourlay Peninsula and North Point. The levels detected at Gourlay Peninsula were the highest amongst the sampling sites. Most of the samples from Gourlay Peninsula contained high levels of Cu (88.57%), with a maximum concentration of 286.63 μg/g. A high Cu content was also detected in several samples from North Point (31.43%). The CF values for Cu at Gourlay Peninsula and North Point were high, with CF values of 28.02 and 5.44, respectively, indicating polluting levels of Cu. Chong et al. (Reference Chong, Dunn, Convey, Tan, Wong and Tan2009) reported similar levels of Cu in Gourlay Peninsula soil samples (188 μg/g). High contents of metals have been reported elsewhere in studies of animal-impacted sites in Antarctica. For instance, Espejo et al. (Reference Espejo, Celis, González-Acuña, Jara and Barra2014) found high Cu, Zn and Pb levels (up to 287, 380 and 2.9 μg/g, respectively) in the guano of two species of penguin from different locations on the Antarctic Peninsula. Similarly, on Deception Island (South Shetland Islands), soils from penguin rookeries contained higher levels of Cu, Cd and Zn than their non-ornithogenic counterparts (Santamans et al. Reference Santamans, Boluda, Picazo, Gil, Ramos-Miras and Tejedo2017). Higher levels of Cu, Cd and Zn in the animal-impacted sites and significant positive Pearson correlations between Cu and Zn (r = 0.884), Cd and Cu (r = 0.733) and Cd and Zn (r = 0.602) can be attributed to their common ornithogenic source (Chu et al. Reference Chu, Yang, Wang, Sun, Yang, Yang and Gao2019b). However, it is also appropriate to note that the geology of Signy Island includes copper-bearing minerals, whose presence is apparent through copper staining of rock outcrops. Areas near the coast in Antarctica have also been reported to contain high levels of Cu. For instance, in Potter Cove, King George Island, this has been associated with transfer from surface marine sediments, which are known to contain high levels of Cu (Andrade et al. Reference Andrade, Poblet, Scagliola, Vodopivez, Curtosi, Pucci and Marcovecchio2001).
High Pb levels were detected in the long-term human-impacted location of Berntsen Point. Thirteen of the 35 samples from this location contained notably high levels of Pb (12.3–127.6 μg/g), in particular the soils sampled close to the storage hut of the research station. The CF value for Pb in Berntsen Point was also relatively high (14.0). Potential anthropogenic sources of Pb include fuel and paint contamination (Santos et al. Reference Santos, Silva-Filho, Schaefer, Albuquerque-Filho and Campos2005). Although the Pumphouse Lake sampling location also has an older history of human impact, Pb levels here were generally low, with the exception of a single sample, which gave the highest level of all samples (211.1 μg/g). This individual instance of locally enriched levels in Pumphouse Lake soil could derive from previous burning of coal and the low mobility of these metal ions and their persistence in the Antarctic terrestrial environment (Claridge et al. Reference Claridge, Campbell, Powell, Amin and Balks1995, Tin et al. Reference Tin, Fleming, Hughes, Ainley, Convey and Moreno2009).
In the PCA, the first two PCs explained a total of 51.6% of the variation in the metal data from 174 soil samples collected from five sampling locations (PC1 = 34.4%, PC2 = 17.2%). Three main groups of variables were identified: 1) Al and Fe, probably of geogenic origin, 2) As, Ca, Cd, Cu, Mg and Zn, likely to have mixed marine-geogenic and marine vertebrate origins, and 3) Hg and Pb, probably from anthropogenic sources. Most of the Gourlay Peninsula samples and some from North Point were located at highly negative values on PC1, indicating that the soils at these sites were strongly influenced by the second group of metal ions, probably associated with marine vertebrates. The human-impacted Berntsen Point samples were mainly influenced by Pb and Hg, which is consistent with an interpretation of probable anthropogenic input.
Correlation analysis revealed that levels of 17 pairs of metals showed strong positive correlation, perhaps indicating a common source, mutual dependence or identical behaviour (Li et al. Reference Li, Huang, Zeng, Yuan, Li and Liang2013). The correlations between metals were consistent with the results obtained from PCA. According to the Pearson correlation matrix, Al and Fe, as well as As, Ca, Cd, Cu, Mg and Zn, showed significant strong positive correlations with each other. This supports the associations between metals, indicating their common origin (Bhuiyan et al. Reference Bhuiyan, Parvez, Islam, Dampare and Suzuki2010). In particular, levels of As, Cd, Cu and Zn were generally highly correlated with each other. Santamans et al. (Reference Santamans, Boluda, Picazo, Gil, Ramos-Miras and Tejedo2017) reported similar findings in soil samples linked to penguin rookeries in the South Shetland Islands and Antarctic Peninsula, again consistent with these metal ions having a marine biogenic origin.
Metal ion levels measured in this study were generally consistent with the data reported by Chong et al. (Reference Chong, Pearce, Convey, Tan, Wong and Tan2010), who also found the highest Cu content in the animal-impacted sites of Gourlay Peninsula and North Point and the highest Pb content at Berntsen Point. Chong et al. (Reference Chong, Pearce, Convey, Tan, Wong and Tan2010) also reported that the most abundant trace element detected in Signy Island soil was Fe, consistent with the geology of the island. The PLI confirmed that the sites most affected by metal ion pollution were the animal-impacted sites of Gourlay Peninsula and North Point, with the human-impacted sites at Berntsen Point and Pumphouse Lake showing lower levels of pollution. This is perhaps unsurprising given the size of the vertebrate populations and the physical extent of their influence on Signy Island relative to the small size and extent of the research and former whaling industry presence on the island and their relatively short duration.
Direct relationships between microalgae and in situ metal contaminants have rarely been investigated. In contrast, multiple studies have addressed relationships between metal ions and bacterial or fungal variables such as total biomass or total biodiversity (Gillan et al. Reference Gillan, Danis, Pernet, Joly and Dubois2005, Chen et al. Reference Chen, He, Zhang, Sun, Zheng and Zheng2014). The DistLM analysis performed in the current study showed that levels of Mn, Ca, Mg, Fe, Zn, Cd, Co, Cr and Cu were significantly correlated with variation in microalgal community composition on Signy Island, although the metals individually contributed a very small proportion (1.32–2.46%) of this variation. Gourmelon et al. (Reference Gourmelon, Maggia, Powell, Gigante, Hortal and Gueunier2016), also using DistLM analysis, reported that soil bacterial and fungal communities in New Caledonia were significantly influenced by Zn, Fe, Mn and Mg. Cd, Pb, Mg and Zn were negatively and significantly correlated with soil bacterial communities in the abandoned mining town of Picher, Oklahoma (Beattie et al. Reference Beattie, Henke, Campa, Hazen, McAliley and Campbell2018), while heavy metals (As, Cd, Cr, Co and Pb) also showed a negative correlation with microalgal diversity in sewage wastewater in a study in India (Renuka et al. Reference Renuka, Sood, Prasanna and Ahluwalia2014). In a non-microbial study, the benthic marine faunal community composition close to Casey Station, East Antarctica, was found to have differences correlated with the levels of Cd, Cu, Pb, Sn and Zn (Stark et al. Reference Stark, Riddle, Snape and Scouller2003).
Overall, a significant if relatively small fraction (16.11%) of the variation in microalgal community composition on Signy Island was explained by the combined metal ion concentrations, with Mn, Ca, Mg, Fe and Zn having the strongest influence. These metals are all essential metals known to be involved in key cellular processes in microalgae. For instance, Mn is involved in the thylakoidal water-splitting oxygen-evolving complex, Ca acts as an intracellular messenger, Mg is required in chlorophyll production, Zn is required in carboxysomal carbonic anhydrase and Fe is required as a redox cofactor in biological processes (Cavet et al. Reference Cavet, Borrelly and Robinson2003).
Multiple environmental factors other than metals are also likely to contribute to the observed variations in microalgal communities, such as nutrient conditions, pH and temperature. Wynn-Williams (Reference Wynn-Williams1990) suggested carbon as the limiting factor for algal colonization of frost-sorted soil at Jane Col. Moisture availability was suggested to be the primary factor limiting Antarctic terrestrial life by Kennedy (Reference Kennedy1993), and more generally the primary controlling factor of Antarctic terrestrial biodiversity by Convey et al. (Reference Convey, Chown, Clarke, Barnes, Bokhorst and Cummings2014). Ecophysiological strategies can also play a key role. For instance, dehydration resistance is possibly more important that cold resistance to survival in Antarctica (Kennedy Reference Kennedy1993). Chong et al. (Reference Chong, Dunn, Convey, Tan, Wong and Tan2009) previously argued that pH, conductivity and Cu and Pb contents had strong influences on soil prokaryote biodiversity on Signy Island.
Conclusions
A total of 29 microalgal taxa, representing Cyanobacteria, Bacillariophyta, Chlorophyta and Tribophyta, were recorded in cultures derived from 175 soil samples collected on Signy Island, South Orkney Islands. High levels of As, Ca, Cd, Cu and Zn were detected in animal-impacted areas at both Gourlay Peninsula and North Point, while high Pb levels were detected in the human-impacted location of Berntsen Point. A significant though relatively low fraction (16.11%) of the variation in microalgal community composition was explained by the combined metal concentrations. Mn, Ca, Mg, Fe and Zn had the strongest influence on microalgal communities on Signy Island.
Acknowledgements
We are grateful to Laura Gerrish of the BAS Mapping and Geographic Information Centre (MAGIC) for producing Fig. 1. We thank two anonymous reviewers for their constructive comments.
Financial support
The funding support from the Ministry of Science, Technology and Innovation (MOSTI), Malaysia, under the Antarctica Flagship Programme (Sub-Project 4: FP1213E036), is gratefully acknowledged. Peter Convey is supported by NERC core funding to the BAS ‘Biodiversity, Evolution and Adaptation’ Team. Nguk-Ling Dang is a scholarship recipient of the Public Service Department (JPA) of Malaysia.
Dedication
This paper is dedicated to Prof Wan-Loy Chu (deceased) for his devotion to algal research and his selflessness, which will always be remembered.
Author contributions
N-LD, W-LC, K-SIY, Y-YK, S-MP and PC conceived the study. N-LD performed the algal culturing and chemical measurements, analysed the data and wrote the first draft of the manuscript. W-LC identified microalgae based on light micrographs. K-KC collected samples, measured parameters on site and wrote part of the ‘Materials and methods’ section. All authors edited the final version of the manuscript.
Supplemental material
Four supplemental figures and four supplemental tables will be found at https://doi.org/10.1017/S0954102021000390.