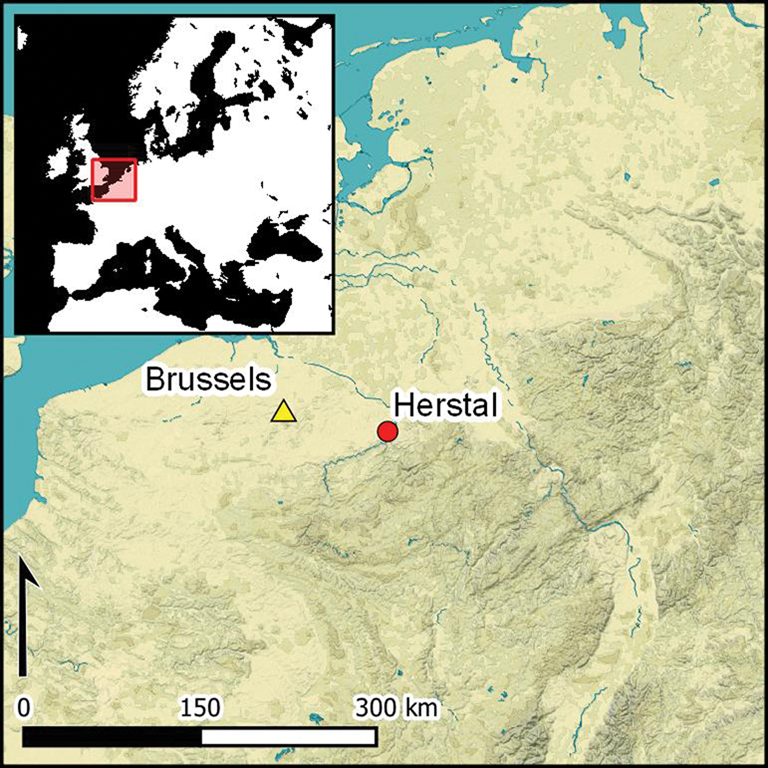
Introduction
During the Roman period, many new animal species were imported into North-western Europe as a result of socio-economic, cultural, administrative and military needs. These species included camels, donkeys, mules, fallow deer, monkeys and peacocks (Lepetz & Yvinec Reference Lepetz, Yvinec and Gardeisen2002; Sykes Reference Sykes2004; Pigière & Henrotay Reference Pigière and Henrotay2012). The flow of exotic animals from south to north should be considered as a useful proxy in the study of the acculturation process that resulted in the incorporation of vast territories under Roman political authority. Each individual species' introduction, however, involved its own specific dynamics and processes.
Fallow deer (Dama dama) are native to the western Iranian plateau, the Eastern Mediterranean (Chapman & Chapman Reference Chapman and Chapman1975, Reference Chapman and Chapman1980; Uerpmann Reference Uerpmann1987; Masseti Reference Masseti1996; Vigne et al. Reference Vigne, Daujat and Monchot2016), Turkey and probably the Balkan Peninsula (Figure 1). Small numbers of fallow deer bones, however, have been recovered from north-west Roman sites in Switzerland, France, the Netherlands and Britain (Sykes et al. Reference Sykes, Baker, Carden, Higham, Hoelzel and Stevens2011; Davis & MacKinnon Reference Davis and MacKinnon2013). Dominated by shed antlers and foot bones, these scattered elements have been suggested to represent the trade in raw materials for craft production or for medicinal properties (Sykes Reference Sykes2004; Miller & Sykes Reference Miller and Sykes2016). As such, these sporadic finds do not necessarily indicate the presence of live fallow deer in Roman North-western Europe. Indeed, previous articulated remains from the apparently secure Roman villa contexts at Binchester and Sparsholt have been radiocarbon-dated to the medieval period (Sykes et al. Reference Sykes2016).
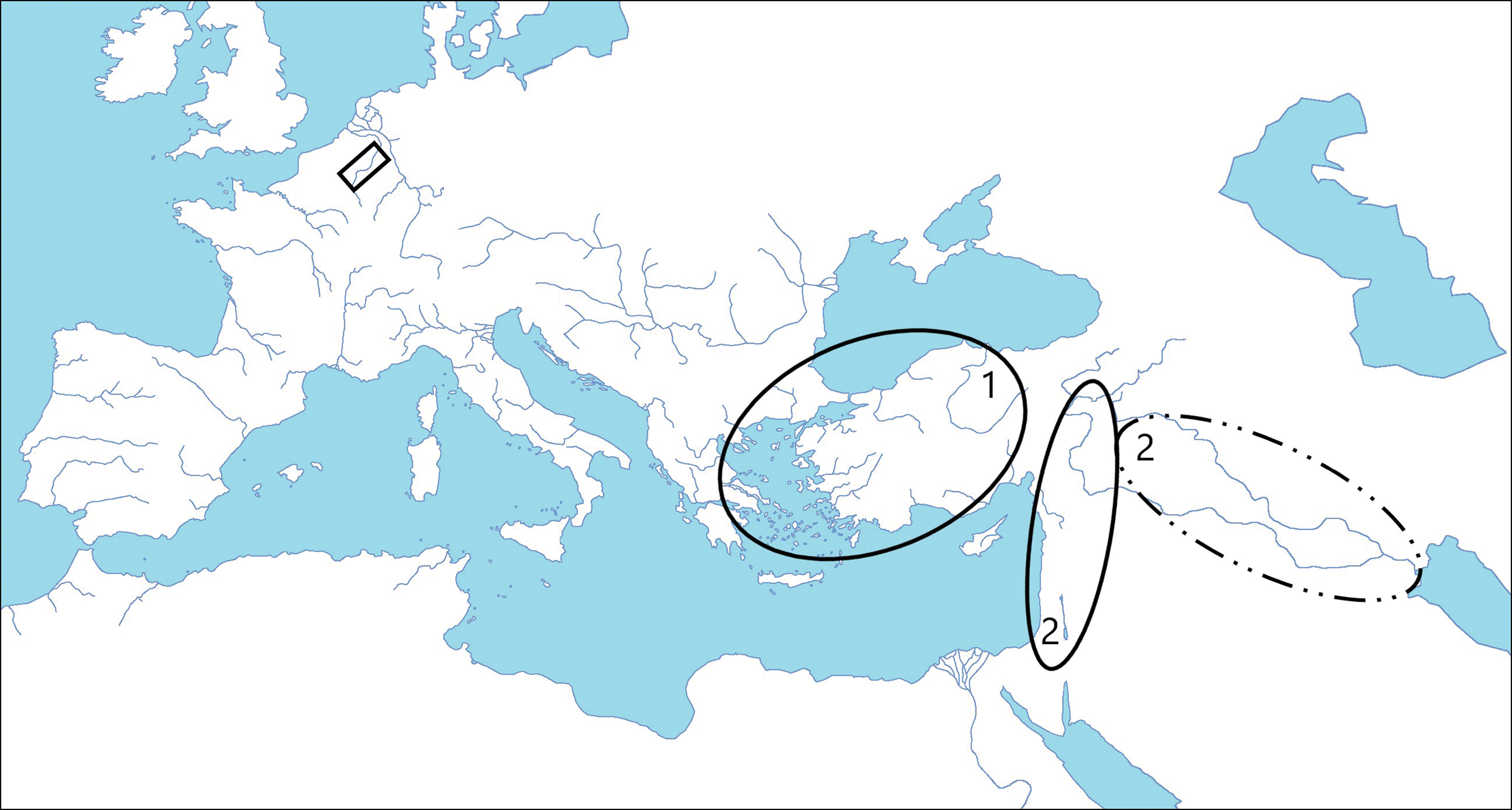
Figure 1. Map showing the location area of the Herstal site in Belgium (black rectangle) and the Early Holocene distribution of: 1) European fallow deer (Dama dama dama); 2) Persian fallow deer (Dama dama mesopotamica), according to Chapman and Chapman (Reference Chapman and Chapman1980); Uerpmann (Reference Uerpmann1987); Vigne et al. (Reference Vigne, Daujat and Monchot2016) (© creative commons, modified by F. Pigière).
A multi-proxy approach on well-dated Roman faunal assemblages from Fishbourne Palace in Sussex and the Monkton settlement in Kent has demonstrated that fallow deer were introduced to and established in southern Britain during the early Roman period, from the first century AD onwards (Sykes et al. Reference Sykes, White, Hayes and Palmer2006, Reference Sykes, Baker, Carden, Higham, Hoelzel and Stevens2011; Miller et al. Reference Miller, Carden, Evans, Lamb, Madgwick, Osborne, Symmons and Sykes2016). No genetic connection, however, has been demonstrated between this Roman-period deer population, and later deer populations in North-western Europe (Sykes et al. Reference Sykes, Baker, Carden, Higham, Hoelzel and Stevens2011; Madgwick et al. Reference Madgwick, Sykes, Miller, Symmons, Morris and Lamb2013), which were reintroduced during the Middle Ages (Chapman & Chapman Reference Chapman and Chapman1980; Uerpmann Reference Uerpmann1987; Masseti Reference Masseti1996; Sykes Reference Sykes2004; Sykes et al. Reference Sykes, Baker, Carden, Higham, Hoelzel and Stevens2011; Madgwick et al. Reference Madgwick, Sykes, Miller, Symmons, Morris and Lamb2013). The Roman introductions appear to be small in scale, and beyond southern Britain there is little evidence that fallow deer were established in Northern Europe during the Roman period. In this article, we present the first known, articulated, Roman-period European fallow deer skeleton, recovered from Herstal (Liège) in Belgium. By adopting a multi-proxy approach enabling us to address crucial issues related to species identification, chronology, origin and diet, we aim to provide important insights into the process of the introduction, management and exploitation of this exotic species.
The finds from Herstal
During March and April 2015, the archaeological heritage division of the ‘Service Public de Wallonie’ conducted archaeological investigations prior to the construction of an apartment building in the ‘rue Jean Lamoureux’, at a place called ‘Sous-la-Chapelle’, in the centre of Herstal, Province of Liège, Belgium (Figure 2). The excavation revealed five Merovingian graves (end of the sixth to the seventh centuries AD), a dump of early Roman domestic rubbish (probably second century AD) and a single pit (F09) containing the partial, in situ skeleton of a deer (Henrard et al. Reference Henrard, Gilet and Pigière2016) (Figure 3).

Figure 2. Location of Herstal town, the 2015 excavation at the Sous-la-Chapelle site (in black) and surrounding archaeological finds: 1) early Roman La Barrière grave; 2) late antiquity inhumation graves; 3) Merovingian graves discovered at the beginning of the twentieth century; 4) Sint-Lambert church (figure by F. Giraldo Martin, Agence wallonne du Patrimoine).

Figure 3. Plan of the 2015 Sous-la-Chapelle excavation: 1) modern pits; 2) early Roman pit; 3) Merovingian graves; 4) early Roman animal skeleton in situ (figure by F. Giraldo Martin, Agence wallonne du Patrimoine).
During the Roman period, this settlement (of unknown nature) was located at the junction of two main roads. Here, the road from the Caput Civitatis of Tongeren crossed the River Meuse to reach, on the opposite bank, the small town of Jupille (Henrard Reference Henrard2013). Human occupation developed at this important crossroads from antiquity onwards. Indeed, Roman building remains have been discovered at several locations on the Rue Hoyoux (Figure 2; Collart-Sacré Reference Collart-Sacré1930). At the nearby site of La Barrière, a burial beneath a tumulus featured wealthy grave goods, including bronze objects imported from Italy during the second half of the second century AD. This indicates the presence of wealthy individuals in the area (Figure 2) (Renard Reference Renard1901; Amand & Mariën Reference Amand and Mariën1976).
This article focuses on the partial deer skeleton found in pit F09. The southern side of this context was heavily disturbed (Figure 4), although no clear divide between the intact and disturbed sediments could be discerned during excavation. Within the disturbed context, some Roman building materials were found, including fragments of tile (tegulae), which probably indicate the presence of a nearby building. The animal was placed in a quadrangular pit on its right side, with the posterior limbs strongly flexed. The positioning of the animal's body within the pit suggests that it may have been constrained at the time of burial (Figure 4). No archaeological materials were found in association with the skeleton.

Figure 4. Context F09 at Sous-la-Chapelle, with the partial European fallow deer skeleton (photograph by D. Henrard Martin, Agence wallonne du Patrimoine).
Methods
Identifying fallow deer: morphological criteria, metrical and DNA analyses
To distinguish red deer from fallow deer, identification of the Herstal cervid employed the osteological criteria published by Lister (Reference Lister1996) and comparison with modern reference skeletons from the Royal Belgian Institute of Natural Sciences. As this research concerns the fallow deer—the presence of which in Roman North-western Europe is rare—ancient DNA (aDNA) analysis was also used to confirm the species identification (see the online supplementary material (OSM) for details on the aDNA methodology).
Radiocarbon dating and stable isotope analyses
In order to establish the date of the in situ cervid skeletal remains, a complete rib and a sample of a left tibia were submitted to the Royal Institute for Cultural Heritage (KIRK-IRPA) in Brussels for radiocarbon dating. Analyses of carbon (δ13C) and nitrogen (δ15N) isotopes in the bone collagen, undertaken as part of this radiocarbon-dating programme, were used to reconstruct the animal's diet, and to provide insight into the management of early breeding populations of fallow deer in North-western Europe (for details on the radiocarbon dating and isotope analyses, see the OSM). Diet was also investigated to ascertain whether the resulting data were representative of the local environment during the Roman period. The growing corpus of carbon and nitrogen stable isotope data on modern and archaeological fallow deer provide a more refined understanding of the factors responsible for variations in isotope values; previous recorded differences in carbon isotope values of fallow deer from archaeological contexts reflect differences in the local environmental conditions in which they lived (Madgwick et al. Reference Madgwick, Sykes, Miller, Symmons, Morris and Lamb2013; Miller et al. Reference Miller, Carden, Evans, Lamb, Madgwick, Osborne, Symmons and Sykes2016; Sykes et al. Reference Sykes2016; Osborne Reference Osborne2017; Beglane et al. Reference Beglane, Baker, Carden, Hoelzel, Lamb, Fhionnghaile, Miller and Sykes2018). Various factors influence the nitrogen isotope values of mammalian herbivore bone collagen, although the soils at the base of the food chain are viewed as the most important (Cormie & Schwarcz Reference Cormie and Schwarcz1996; Sponheimer et al. Reference Sponheimer2003; Codron et al. Reference Codron, Brink, Rossouw and Clauss2008). Other factors considered to be responsible for variations are dietary protein quality and environmental conditions, such as salinity or ecophysiological stress effects.
We compared the results of our isotope analysis of the Herstal deer to the mean values of a dataset for fallow deer from Roman to post-medieval times in Ireland, south-east Britain, Northern Europe and the Mediterranean area, as well as from the Neolithic to the medieval period in Turkey and Central Europe (means of data presented in Madgwick et al. Reference Madgwick, Sykes, Miller, Symmons, Morris and Lamb2013; Miller et al. Reference Miller, Carden, Evans, Lamb, Madgwick, Osborne, Symmons and Sykes2016; Sykes et al. Reference Sykes2016; Beglane et al. Reference Beglane, Baker, Carden, Hoelzel, Lamb, Fhionnghaile, Miller and Sykes2018). Fallow deer from Fishbourne Palace and the rural Monkton site in south-east Britain provide the main Roman samples so far available for comparison (Madgwick et al. Reference Madgwick, Sykes, Miller, Symmons, Morris and Lamb2013).
Identification of the Dama dama dama skeleton at Herstal
Analysis of the bones from pit F09 at Herstal resulted in the identification of a partial cervid skeleton. The animal was probably buried as a complete, articulated individual, but disturbed by later digging activity. Only the posterior vertebrae and ribcage, along with the pelvic girdle and the upper parts of posterior limbs, were still in articulation (Figure 4). Several other bones, which were scattered within the burial context, can be attributed to the same animal (Table 1). These comprise skull fragments, one of which has parts of an antler attached, vertebrae, ribs and elements from the anterior limbs. Several elements were completely absent, and were probably removed by the later disturbance.
Table 1. Skeletal elements of the European fallow deer from Herstal, Belgium. MNE: minimum number of elements; F: fused; NF: non-fused; Fg: fusing; prox: proximal; dist: distal.

Morphological identification
The red deer is the only medium-sized cervid indigenous to North-western Europe (Frechkop Reference Frechkop1958). Native to the Eastern Mediterranean, the fallow deer was long known to have been introduced to North-western Europe during the Middle Ages (Chapman & Chapman Reference Chapman and Chapman1980; Uerpmann Reference Uerpmann1987; Masseti Reference Masseti1996; Sykes Reference Sykes2004). As described above, however, recent evidence now indicates that fallow deer were present in southern Britain during the Roman period. Given the osteological similarity between the red and fallow deer, and the overlap in size between them, both species were considered as possible identifications for the Herstal individual. Roe deer can be excluded due to their smaller size (Frechkop Reference Frechkop1958): the bone measurements for the Herstal skeleton do not plot within the size variation of roe deer (Sykes Reference Sykes2004).
The criteria provided by Lister (Reference Lister1996) to distinguish between red and fallow deer encompass morphological variations in the antlers, permanent dentition and most of the post-cranial elements. For the Herstal individual, species identification was based on five skeletal elements: antler, metacarpal, tibia, and first and second phalanges. Although the antler is fragmented, analysis of the basal element shows that the brow tine makes a clear, oblique angle with the beam (Figure 5). This is characteristic of fallow deer antler. For the anterior limb elements, species-identification criteria can only be applied to the metacarpal (Lister Reference Lister1996). The tuberosity on the anterior, proximal part of the shaft—just below the epiphysis—is rather smooth and is bordered by a neat ridge, which is characteristic of fallow deer. For the posterior limbs, proximal and distal parts of the tibia were examined (Figure 6). The internal and external peaks of the proximal epiphysis project to an approximately equal extent, and the distal epiphysis shows a small, discrete area of flat bone, roughly perpendicular to the bone's transverse axis. Furthermore, its external edge is more U-shaped. All of these features on the tibia suggest an identification consistent with fallow deer.
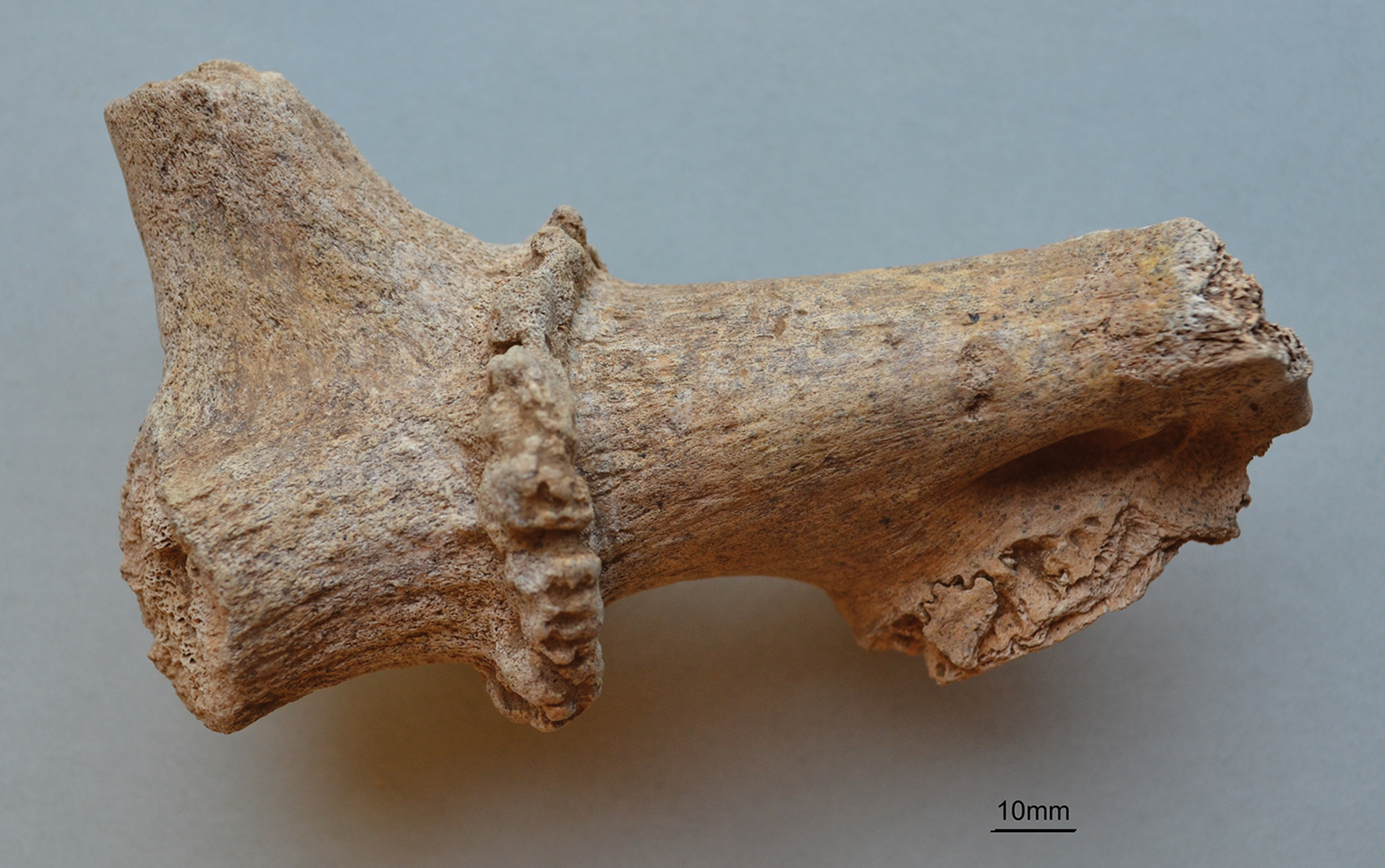
Figure 5. European fallow deer skull fragment with part of an antler attached (photograph by E. Dewamme, Royal Belgian Institute of Natural Sciences).
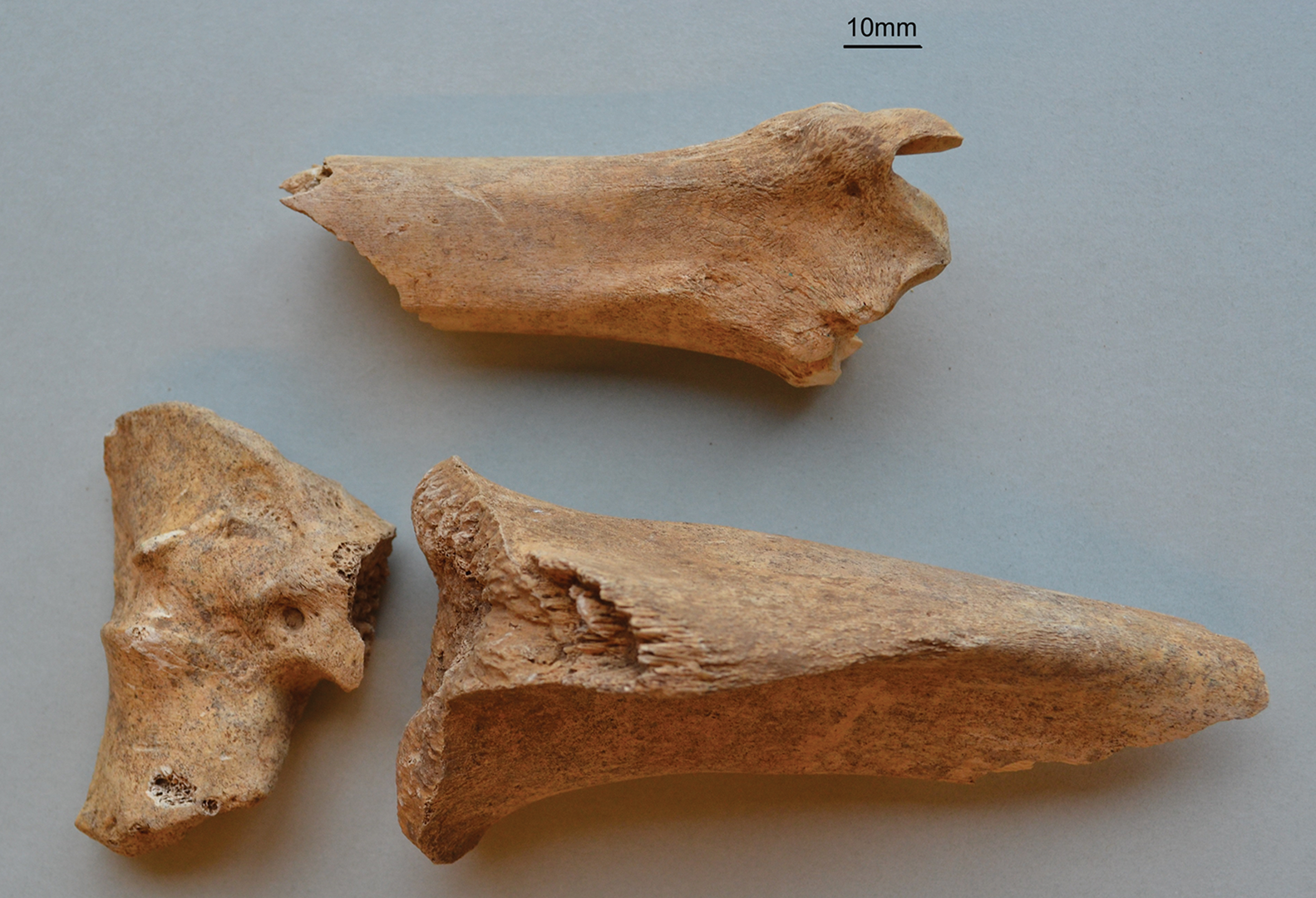
Figure 6. Proximal and distal parts of the European fallow deer right tibia (photograph by E. Dewamme, Royal Belgian Institute of Natural Sciences).
Analysis of the first phalanx reveals that directly posterior to the articular facet, the bone drops away distally. The second phalanx displays an emargination of the proximal articular facet, which is also characteristic of fallow deer. To conclude, morphological evidence from the five skeletal elements from Herstal is entirely consistent with an identification of a fallow deer.
Ancient DNA identification
Four samples of bone powder were collected from three bone fragments: 50mg from the distal end of the left tibia, 50mg from the proximal end of the right tibia, and two samples of 50mg from the proximal end of a metacarpal (right anterior leg). DNA sequences of the 12S ribosomal DNA gene (mitochondrial DNA) were obtained for three of the four sampled bones, as no DNA could be amplified or sequenced from the sample extracted from the proximal end of the tibia (for more information about DNA analysis protocols, see the OSM). The sequences comprised 126 base pairs (after the trimming of the primers), and matched for the three bone samples. Comparison with the public repository of nucleotide sequences GenBank shows that all of the closest matches are with the European fallow deer (Dama dama dama), with 100 per cent coverage and 100 per cent identity. In this region of 126 base pairs, the number of substitutions observed between two sequences (that is, the number of nucleotides that are different between two sequences) provides an idea of the similarity between these two sequences. Compared to the sequences obtained by Fajardo et al. (Reference Fajardo, González, López-Calleja, Martín, Rojas, Hernández, García and Martín2007), we observed zero to one substitution compared to European fallow deer sequences; four substitutions compared to Persian fallow deer (Dama dama mesopotamica) sequences; three to five substitutions compared to red deer (Cervus elaphus) sequences; two to four substitutions compared to sika deer (Cervus nippon) sequences; and three substitutions compared to the chital deer (Axis axis) sequence (Figure 7). The sequence that was retrieved here is therefore identical (zero substitution) or slightly different (one substitution) to the sequences of European fallow deer, and more different from the sequences of the other Cervidae (>2 substitutions). Substitutions among sequences of the same species are possible and represent intraspecific variability. A total of 113 Cervidae sequences (65 haplotypes) of the 12S ribosomal DNA fragment studied here were retrieved from GenBank. The phylogenetic tree, constructed with the maximum likelihood method and based on this 12S ribosomal DNA fragment, confirms that the Herstal specimen belongs to the clade comprising all Dama dama dama representatives (Figure 8), and does not belong to Dama dama mesopotamica nor any species of the genera Cervus or Axis (Figure 8). Thus, the aDNA analysis confirms the species identification as a European fallow deer and proves the reliability of Lister's (Reference Lister1996) morphological criteria.
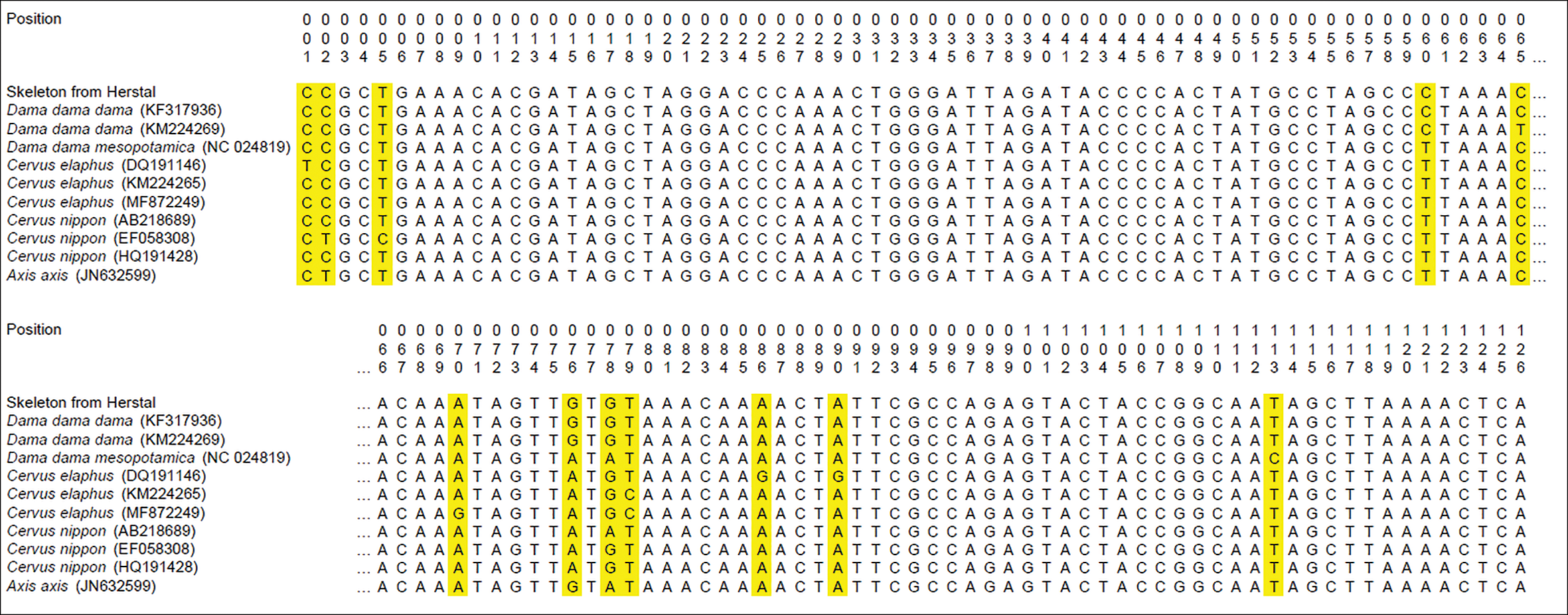
Figure 7. Ancient DNA analysis: character-based comparison of 12S DNA fragments. One representative sequence record for each unique haplotype is provided (accession numbers in GenBank are given in parentheses). Variable sites are highlighted and character positions are given using three digits (hundreds, tens and ones are given from top to bottom) (figure by G. Sonet).

Figure 8. Ancient DNA analysis: phylogenetic tree constructed with the maximum likelihood method and based on 12S ribosomal DNA. Only unique haplotypes were used (coded with Roman numbers). Bootstrap values (%) above 70 per cent are given at nodes. This tree was rooted with tufted deer (Elaphodus cephalophus) (figure by G. Sonet).
Description and radiocarbon dating of the individual
The age-at-death of the Herstal European fallow deer can be estimated on the basis of the epiphyseal fusion of the extant elements (Table 1). According to fusion ages provided by Habermehl (Reference Habermehl1985) and Carden and Hayden (Reference Carden, Hayden and Ruscillo2002), the animal died aged between two and three years old. This estimation is based on the fusion state of the tibia, for which we observed that the proximal epiphysis is unfused and the distal epiphysis is fused. Moreover, the proximal femur epiphysis was partially fused. The presence of the antler indicates that the animal was a male (stag). There is no evidence for cut marks on bones that would indicate the removal of body parts. Although antlers and feet, in particular, appear to have held special value during the Roman period, and to have been traded (Miller & Sykes Reference Miller and Sykes2016), the evidence from Herstal suggests that the entire corpse of a stag was buried in pit F09, without the removal of any of these valuable elements.
The rib and a sample from the left tibia submitted for radiocarbon dating yielded dates of 1849±28 BP (RICH 22910; cal AD 80–240 at 95.4% confidence) and 1813±30 BP (RICH 24095.2.1; cal AD 120–260 at 88.1% confidence) for the latter (calibrations were performed using OxCal v.3 and the IntCal13 calibration curve data; Bronk Ramsey Reference Bronk Ramsey1995, Reference Bronk Ramsey2001; Reimer et al. Reference Reimer2013; for the method, see the OSM). These results date the animal firmly within the early Roman period.
Osteometrics
Metric analysis of the Herstal individual permits comparisons with fallow deer populations resident in different geographic areas. Osteometric studies on modern and archaeological fallow deer indicate that native populations in Turkey and Greece are far larger in body size than historically translocated herds in Southern and Northern Europe (Sykes et al. Reference Sykes, Baker, Carden, Higham, Hoelzel and Stevens2011, Reference Sykes, Carden and Harris2013). The reasons for this size difference are not yet fully understood, but they likely relate to the so-called ‘insular dwarfism’: namely the transition from large free-roaming populations to restricted and more restrained groups, which caused a decline in body size due to genetic, environmental and/or nutritional factors (Lomolino Reference Lomolino1985, Reference Lomolino2005). Study of this phenomenon in a wide variety of species demonstrated that in 175 years, larger species, among other cervids, undergo dwarfism (Schmid & Jensen Reference Schmidt and Jensen2003). Peterson et al. (Reference Peterson, Vucetich, Beyer, Schrage and Räik-Könen2011) argue that reduced body size developed in Isle Royale moose within 50 years of their establishment. Our osteometric study was therefore intended to establish whether the Herstal individual derived from a population of larger animals from the Eastern Mediterranean, or from the smaller animals of translocated herds.
Following standard measurements defined by von den Driesch (Reference von den Driesch1976), the greatest breadth of the distal tibia from the Herstal specimen was plotted against measurements of native, Neolithic to Iron Age populations from Turkey and Greece, Roman-period individuals from North-western Europe, Roman to post-medieval specimens from Italy and Iberia, as well as modern male and female fallow deer from Phoenix Park in Dublin (Sykes et al. Reference Sykes, Baker, Carden, Higham, Hoelzel and Stevens2011, Reference Sykes, Carden and Harris2013; Carden et al. Reference Carden, Sykes and Hayden2013; data from the deer bone database are available online at https://www.nottingham.ac.uk/zooarchaeology/deer_bone/search.php?12May2019) (Figure 9). The data measurements from modern female and male red deer from Ireland are also plotted on the graph, showing that these individuals have far larger body sizes than the fallow deer. A small amount of overlap is recorded between the smallest female red deer and the largest male fallow deer from Greece. The high degree of sexual dimorphism in the fallow deer is clear in the distribution data of modern male and female individuals.

Figure 9. Distal breadth (Bd) measurements of the fallow deer tibia from Herstal, shown against the distribution for modern Phoenix Park (Dublin) individuals and archaeological specimens from Neolithic–Iron Age Turkey and Greece, the Roman individuals from North-western Europe, the Roman to post-medieval specimens from Italy and the Iberian peninsula, as well as modern Irish red deer. F, modern female; M, modern male (figure by F. Pigière).
The samples from Turkey and Greece of Neolithic to Iron Age date are distinguished from the other populations by their larger size. Although caution is required in interpreting the data due to limited sample sizes, the smallest measurements are recorded amongst the North-west European and the Iberian samples. The Italian individuals display an intermediate size between the larger Turkish and Greek populations and the smaller Iberian and North-west European specimens. The Herstal stag plots within the size range of the Italian and Iberian males. As the Herstal individual is a juvenile, its distal tibia measurement is less than that of its potential adult size. Thus, the individual probably ranges either among the largest translocated males (particularly the Italian ones), or among the smallest native Eastern Mediterranean males. The small size of most of the translocated North-western European Roman-period individuals suggests that they derived from translocated herds, rather than from native Mediterranean populations. Further insight into the Herstal individual's local environmental conditions and geographic area can be obtained from stable isotope analyses.
Isotope analyses
The following isotopic values were obtained from the bone collagen of two skeletal elements:
• Left tibia: δ15N = +7.1‰, δ13C = −22.4‰, C/N = 3.3.
• Rib: δ15N = +8.0‰, δ13C = −22.5‰, C/N = 3.3.
While the δ13C values of the two bones are similar, the δ15N values vary between the two elements—a variation that can be explained by the different rates of tissue turnover between the two bones. Indeed, isotopes accumulate over a longer period in the tibia than rib bones (Tieszen et al. Reference Tieszen, Boutton, Tesdahl and Slade1983). Figure 10 shows that the Herstal values fall within the range of historical, North-western European data, with a depletion in δ13C and higher δ15N values compared with the Turkish fallow deer, as well as those from the Mediterranean and Central Europe. The Herstal individual has intermediate values that fall between the Irish and the Northern European samples, the latter being slightly more enriched in carbon and nitrogen than the former. The sample from Britain has equivalent carbon values to the specimens from Northern Europe, but a significantly lower nitrogen value. For the Roman-period fallow deer, a comparison with the Fishbourne Palace and the Monkton samples shows that the values of the Herstal individual are closer to the former sample, while the Monkton individuals are distinguished from the other two by less depleted carbon values (Figure 10).

Figure 10. Carbon and nitrogen isotope results for Herstal, Fishbourne Palace and Monkton fallow deer specimens, summary of comparative data from Roman to post-medieval south-east Britain, Northern Europe, Ireland and the Mediterranean, and from Neolithic–medieval Turkey and Central Europe. Site means and error bars indicating the standard deviation (68 per cent) are mentioned (figure by F. Pigière).
The carbon and nitrogen signals from the Fishbourne Palace and Herstal samples are those typically to be expected from herbivore species feeding in temperate environments (Sykes et al. Reference Sykes, White, Hayes and Palmer2006; Miller et al. Reference Miller, Carden, Evans, Lamb, Madgwick, Osborne, Symmons and Sykes2016). Furthermore, strontium (Sr) isotope analyses of tooth enamel have confirmed that the Fishbourne specimens were from an established breeding population in the Palace area (Sykes et al. Reference Sykes, White, Hayes and Palmer2006). An animal of first-generation introduction dated to AD 60±40 (Beta-201535; cal BP 1990–1820 at 94.6% confidence) was identified using this approach. While the strontium signature for the first molar demonstrated a non-local origin, those from the second and the third molars from the same individual are compatible with the Fishbourne area, and suggest that the translocation occurred at an early stage of life (Miller et al. Reference Miller, Carden, Evans, Lamb, Madgwick, Osborne, Symmons and Sykes2016). Strontium analyses on a second individual dated to c. AD 90±40 (Beta-201534; cal BP 1930–1740 at 94.6% confidence) and a third individual's first molar indicate that both probably represent fallow deer that were born and raised in the Fishbourne Palace area.
It has been demonstrated that the fallow deer bones from Monkton were also from an established population, based on radiocarbon dating, skeletal representation, isotope analyses and osteometric analysis (Madgwick et al. Reference Madgwick, Sykes, Miller, Symmons, Morris and Lamb2013). Furthermore, the higher carbon values compared to other locations in southern Britain are related to the site's coastal environment, as the multi-proxy approach refuted first-generation introduction of animals fed on C4 plants, such as millet (Madgwick et al. Reference Madgwick, Sykes, Miller, Symmons, Morris and Lamb2013).
Discussion and conclusion
Morphological, ancient DNA and osteometric analyses have identified the Herstal animal as a juvenile, two- to three year-old, male European fallow deer. This skeleton therefore represents the first articulated individual from Roman North-western Europe, confirming without any doubt the importation of a living animal into the northern provinces. Isotope analyses indicate that the animal had a diet typical of a temperate environment. This suggests that the animal was not a first generation import from Southern Europe or Turkey, but rather that it was bred and raised in temperate North-western Europe.
The animal was probably connected to a local early Roman settlement. Pit F04 is itself evidence for the presence of Roman-period activity in the immediate vicinity. The deposition of an unbutchered European fallow deer at Herstal suggests that this animal had symbolic, rather than economic, significance, and was kept for prestigious purposes by the elite classes. Although the stag was apparently constrained at deposition, no feature in the pit provides further evidence to support ritual significance.
The role of the animal as a high-status symbol has been shown for the fallow deer kept in a special enclosure at the Fishbourne Roman Palace, the purported residence of the client king Togidubnus (Sykes et al. Reference Sykes, White, Hayes and Palmer2006, Reference Sykes, Baker, Carden, Higham, Hoelzel and Stevens2011). There, the animal appears as an icon of high social position through a complex symbolism that references the deer's exotic origin and the colonial power of the Roman Empire as well as human dominance over nature via the animal's position within a manmade enclosure. The Herstal animal's exceptional size amongst translocated populations reinforces its symbolic role. The presence of Romanised elites that could keep such a stag is evidenced by several archaeological sites around Herstal, including the nearby richly furnished burial at La Barrière (Renard Reference Renard1901; Amand & Mariën Reference Amand and Mariën1976). Furthermore, excavations at the nearby site of Pré Wigier have revealed a large late first- to late third-century AD settlement site, and the wealthy domus at Place Saint-Lambert in Liège demonstrates contemporaneous high-status occupation within this region (Otte Reference Otte1984; Henrard et al. Reference Henrard, van der Sloot and Léotard2008).
Although the presence of the European fallow deer at Herstal can be related to the elite, who kept the prestigious animal in their rural domain, remains of this species are also attested at Roman urban sites of North-western Europe (Sykes et al. Reference Sykes, Baker, Carden, Higham, Hoelzel and Stevens2011). The finds on urban sites never exceed two bones and there is a clear over-representation of antlers, metapodia, scapulae and radii (Sykes et al. Reference Sykes, Baker, Carden, Higham, Hoelzel and Stevens2011). The highly selective nature of fallow deer skeletal elements at these urban centres suggests that particular body parts were traded. While body-part importation from southern areas—as opposed to local breeding—has long been assumed, to date, no isotopic analyses have confirmed the trade of skeletal elements or cured meat between North-western Europe and the Mediterranean (Miller et al. Reference Miller, Carden, Evans, Lamb, Madgwick, Osborne, Symmons and Sykes2016). The findings from Herstal confirm definitively the presence of live animals in the northern provinces and support the long-term presence of the fallow deer in these areas. Although there is earlier evidence from Fishbourne Palace, dated to the mid first century AD, the Herstal skeleton suggests that fallow deer were still being raised in temperate environments during the mid second or early third century AD. Consequently, North-western European breeding appears to be a more likely source for products traded to towns.
Uncovering the origins of introduced fallow deer in North-western Europe is critical for wider understanding of the dynamics of exchange between the different regions of the Roman Empire. Isotope data from the Herstal individual and the metric analyses of several north-western fallow deer now suggest that these animals originated from translocated herds, rather than from native Eastern Mediterranean populations. Refining the origin and timing of the established population of fallow deer in North-western Europe requires further investigation through a combined analysis of zooarchaeological, DNA, geometric morphometrics and isotopes analyses.
Acknowledgements
The authors would like to thank Karis Baker for sharing data and providing recommendations on aDNA analysis. Many thanks to Holly Miller for her thoughts on this article and the two anonymous referees for their comments.
Funding statement
The DNA analysis performed for this research was financially supported by the Belgian Science Policy (BELSPO) through the Joint Experimental Molecular Unit.
Supplementary material
To view supplementary material for this article, please visit https://doi.org/10.15184/aqy.2020.207