Introduction
The extinct subfamily Leptarctinae includes Miocene mustelids that are sister taxon to the clade including crown mustelids (i.e., neomustelids) and their close relatives (Plesiogale and Paragale) (Wang et al., Reference Wang, Qiu. and Wang2004; Bever and Zakrzewski, Reference Bever and Zakrzewski2009). They are found from the early Hemingfordian to early Hemphillian North American Land Mammal Ages (18.8–6.7 Ma; Baskin, Reference Baskin1998; Lim et al., Reference Lim, Martin and Wilson2001; Tedford et al., Reference Tedford, Albright, Barnosky, Ferrusquia-Villafranca, Hunt, Storer, Swisher, Voorhies, Webb and Whistler2004) and known primarily from cranial and dental material. Most of the diversity of leptarctines is found in North America, but the Asian fossil record of this subfamily has grown through recent finds (e.g., Wang et al., Reference Wang, Qiu and Opdyke2003; Wang et al., Reference Wang, Qiu. and Wang2004). Leptarctines are also found in European faunas from the early to mid-Miocene (17–15 Ma) of France, Germany, Luxembourg, and Spain (e.g., Robles et al., Reference Robles, Alba, Moyà-Solà, Casanovas-Vilar, Galindo, Rotgers, Almécija and Carmona2010). The subfamily Leptarctinae includes at least five, possibly six, genera (Trocharion, Kinometaxia, Schultzogale, Craterogale, Leptarctus, and possibly Hypsoparia). All leptarctines share prominent features of the skull, including a short face, parasagittal crests, roughened parietals, robust zygomatic arches, grooves on the lingual side of the lower canines, and unusual projections on the tympanic bullae (Baskin, Reference Baskin1998; Lim and Martin, Reference Lim and Martin2002; Wang et al., Reference Wang, Qiu. and Wang2004).
Leptarctus was erected by Leidy (Reference Leidy1856) on the basis of an isolated upper fourth premolar (P4), but subsequent finds have produced enough fossil material to characterize the genus by a number of distinct features of the skull and dentition. These diagnostic dental characters include: a large P4 with a prominent parastyle, an internal cingulum, a large hypocone, a quadrate upper first molar (M1) with widely separated paracone and metacone and a posterointernal hypocone, and numerous features of the lower dentition including m1 with a strong metaconid, a long talonid, and a notch between the metaconid and the cingulum of the talonid (Baskin, Reference Baskin1998; Wang et al., Reference Wang, Qiu. and Wang2004; Bever and Zakrzewski, Reference Bever and Zakrzewski2009). The holotype of Leptarctus oregonensis (LACM CIT 206) was collected in the Mascall Formation (Barstovian: 15.77–14.80 Ma; Tedford et al., Reference Tedford, Albright, Barnosky, Ferrusquia-Villafranca, Hunt, Storer, Swisher, Voorhies, Webb and Whistler2004) of Oregon and described by Stock (Reference Stock1930). It consists of a partial maxilla with the left P4–M1, the right P4, a partial zygomatic arch, and a cranial fragment including part of the lateral temporal crest. Leptarctus oregonensis was distinguished from the previously identified species of Leptarctus (L. primus, L. wortmani, Matthew, Reference Matthew1924 and L. progressus Simpson, Reference Simpson1930) by size and details of tooth morphology. Additional material attributed to L. oregonensis was found in the Mascall Formation (a single upper P4, UCMP 39102; Downs, Reference Downs1956). Baskin (Reference Baskin1998, Reference Baskin2005) interpreted the Leptarctus material from the Olcott Formation of Nebraska (Snake Creek Beds, 17–15 Ma; Tedford et al., Reference Tedford, Albright, Barnosky, Ferrusquia-Villafranca, Hunt, Storer, Swisher, Voorhies, Webb and Whistler2004) that Matthew (Reference Matthew1924) had described as L. primus as belonging in fact to L. oregonensis, thus adding a lower jaw (AMNH 18270) and a nearly complete skull (AMNH 18241) to the material known for L. oregonensis and extending the geographic distribution of the species outside of Oregon. Baskin, (Reference Baskin1998) argued that the “smaller P4 with a less prominent hypocone and [the] relatively smaller M1” (Baskin, Reference Baskin1998, p. 158) of AMNH 18241 compared to the holotype of L. primus, which supported its assignment to Leptarctus oregonensis. Lim and Martin (Reference Lim and Martin2001a, Reference Lim and Martin2002) and Lim et al. (Reference Lim, Martin and Wilson2001) did not recognize the AMNH skull and the lower jaw as L. oregonensis and, instead, identified these specimens as L. primus. Wang et al. (Reference Wang, Qiu. and Wang2004) also identified AMNH 18241 as L. primus. The sizes of the P4 and M1 of AMNH 18241 are intermediate between those of the type specimen of L. primus and the L. oregonensis material from Oregon, and therefore do not allow this debate to be settled (Table 1). Although figured (Matthew, Reference Matthew1924, fig. 37), AMNH 18241 was only briefly described by Matthew (Reference Matthew1924), as well as by Korth and Baskin (Reference Korth and Baskin2009) who described parts of its zygomatic arch and tympanic projections. The lack of substantial cranial material from Oregon (beyond LACM CIT 206) has prevented an assessment of the differences in cranial morphology between specimens from Nebraska and those from Oregon. We are now able to describe an additional specimen of Leptarctus oregonensis from the Mascall Formation of eastern Oregon, the type formation for this taxon. This new, almost complete skull (UOMNH F-35458) is the first specimen to preserve the morphology of the auditory bulla and its processes. It also includes details of the basicranium, previously undescribed for L. oregonensis, which are frequently important in carnivore systematics and should be important in future phylogenetic analyses of the leptarctines. UOMNH F-35458 provides the opportunity for a comprehensive description of the cranial morphology of this taxon, a revision of the diagnosis of the species, and additions to the long-standing debate concerning intraspecific variation in species of Leptarctus (see Korth and Baskin, Reference Korth and Baskin2009). Indeed, despite some morphological differences between the specimens from Oregon and those from Nebraska, we argue here based on UOMNH F-35458 that the morphological similarities between AMNH 18241 and the L. oregonensis material from Oregon support the presence of L. oregonensis in Nebraska.
Table 1 Cranial measurements for Leptarctus oregonensis and other mustelids.

UOMNH F-35458, L. oregonensis; AMNH 18241, L. oregonensis (from Wang et al., Reference Wang, Qiu. and Wang2004 and based on UCMP 27295); LACM (CIT 206), L. oregonensis; UCMP 39102, L. oregonensis; AMNH 25258, L. primus (from Lim and Martin, Reference Lim and Martin2002); UF 5706, L. ancipidens (from Lim and Martin, 2001; Lim and Martin, Reference Lim and Martin2002); CM 81783, L. mummorum (from Korth and Baskin, Reference Korth and Baskin2009); UNSM 20843, L. martini (from Lim and Miao, Reference Lim and Miao2000; Lim and Martin, 2001); UWBM 28348, Mustela putorius; UWBM 6418, Bassariscus astutus; UWBM 41393, Taxidea taxus; UWBM 41022, Gulo gulo; UWBM 59961, Procyon lotor; UWBM 41336, Mephitis mephitis; UWBM 51603, Spilogale putorius; UWBM 32973, Meles meles. Abbreviations: LR, Length of rostrum from posterior to the postorbital process of the jugal anteriorly; RW, width of rostrum at canines; WZA, width of skull across zygomatic arches; HZA, total height of zygomatic arches; SL, skull length; POC, width of skull at postorbital constriction; PW, width of palate between M1s; OW, maximum width of orbits; LP2, length of P2; WP2, width of P2; LP3, length of P3; WP3, width of P3; LP4, length of P4; WP4, width of P4 across protocone; LM1, length of M1; WM1, width of M1. Measurements of the P4 and M1 were taken following the guidelines of Popowics (Reference Popowics2003). Measurements in mm.
* =Broken feature, measurement approximate;
1 one of the two measurements given in the original publication;
† specimen’s cheek teeth are too worn to be accurately measured.
Because mustelids are known for their ecological diversity and resource partitioning (e.g., Bonesi et al., Reference Bonesi, Chanin and MacDonald2004; Harrington and McDonald, Reference Harrington and Macdonald2008; Dumont et al., Reference Dumont, Wall, Botton-Divet, Goswami, Peigné and Fabre2016; Valenciano et al., Reference Valenciano, Baskin, Abella, Pérez-Ramos, Álvarez-Sierra, Morales and Hartstone-Rose2016), the ecology of the Leptarctinae cannot be easily understood through extant phylogenetic bracketing. Inferences from skeletal anatomy are therefore critical to determining the ecology of the leptarctines and informing future studies of community structure in the Miocene of eastern Oregon and more broadly across North America and Asia. Leptarctine ecology and diet have been the topic of much debate and many modern analogs for Leptarctus have been proposed. Starting with the publication of L. primus by Leidy (Reference Leidy1856), many similarities between Leptarctus and procyonid carnivorans, such as Nasua, Potos, and Procyon, were recognized, leading to debates as to the taxonomic affinities of Leptarctus (see Korth and Baskin, Reference Korth and Baskin2009). Although Gazin (Reference Gazin1936) established that leptarctines belong to Mustelidae, the morphological similarities with procyonids have been interpreted as evidence for similarities in ecology. Olsen (Reference Olsen1957) proposed that Leptarctus was similar to the extant mustelid Taxidea taxus Schreber, Reference Schreber1777, the North American badger, a carnivore (Long, Reference Long1973), but some have since then disputed this analogy (Lim and Martin, Reference Lim and Martin2001b). Lim and Miao (Reference Lim and Miao2000) noted the molariform shape of the upper fourth premolar of Leptarctus and, together with its even wear, interpreted it as indicative of an omnivorous diet similar to that of the raccoon (Procyon lotor Storr, Reference Storr1780; Lotze and Anderson, Reference Lotze and Anderson1979). Lim and Martin (Reference Lim and Martin2002) noted similarities in the wear of the canine of the leptarctine Hypsoparia with the coati (Nasua nasua Linnaeus, Reference Linnaeus1766, an omnivore; Gompper and Decker, Reference Gompper and Decker1998), interpreting the subfamily as more herbivorous than most carnivorans. The enlarged zygomatic arches and large tympanic projections ventral to the auditory bullae that are superficially similar to those found in the extant koala (Phascolarctos cinereus Goldfuss, Reference Goldfuss1817, an arboreal herbivore; Cork et al., Reference Cork, Hume and Dawson1983) were seen as additional evidence for Leptarctus incorporating foliage in its diet and possibly being arboreal (Lim and Martin, Reference Lim and Martin2001a). Lim and Martin (Reference Lim and Martin2001b) interpreted a suite of craniodental characters of Leptarctus as evidence for “an omnivorous diet, with a strong plant component” (Lim and Martin, Reference Lim and Martin2001b, p. 317). They compared the peculiar morphology of the upper third premolar of L. desuii to that of the fruit-eating bat Pteropus rodricensis Dobson, Reference Dobson1878 (Lim and Martin, Reference Lim and Martin2001b). They also noted several similarities in the morphology of the cheek teeth (including the enlarged molariform morphology of the upper fourth premolar and the relatively long talonid of the lower first molar) and the skull (notably an enlarged surface for the attachment of the masseter muscle) with Procyon lotor or even Ursus americanus Pallas, Reference Pallas1780 (the American black bear) and the koala (Lim and Martin, Reference Lim and Martin2001b). Korth and Baskin (Reference Korth and Baskin2009) refuted the interpretation of leptarctines as herbivorous koala-like animals, pointing out the lack of specialized incisors, the presence of a prominent canine, the absence of a diastema, and the cuspate shape of the teeth as opposed to the shearing crests found in herbivorous taxa (including the koala). The new material of L. oregonensis described herein also allows a functional and paleoecological study of the craniodental morphology of one of the oldest species of Leptarctus and thus helps shed light on the dietary habits of the Leptarctinae.
Materials and methods
We describe UOMNH F-35458, a mostly complete skull of Leptarctus oregonensis, including the right P4 and M1, the left third premolar (P3), P4, and M1, and lacking only the rostrum. We compare this new material to UCMP 39102 and LACM (CIT 206), all previously known specimens of L. oregonensis, a cast of AMNH 18241 (UCMP 27295), photos of F:AM 25385, the skulls of several extant mustelids, mephitids, and procyonids (Table 1), and published specimens of the subfamily Leptarctinae. F-35458 was collected at the early Barstovian locality UO 2993 in 1997 during a road construction project west of Dayville, Oregon, along Highway 26 near the Mascall type area. We use nomenclature following recent publications of other species of Leptarctus (in particular Korth and Baskin, Reference Korth and Baskin2009) for skull terminology and cranial foramina. We use the terminology of Olsen (Reference Olsen1957) for the description of the dentition. Our measurements (Table 1) are either drawn from the literature or were taken directly on the specimens using Mitutoyo Absolute Digimatic CD-6”C and Mitutoyo Absolute Digimatic CD-8”CX calipers.
In the absence of the rostrum, which prevents the measurement of skull length, we measured the occiput to orbit length of UOMNH F-35458 to estimate the body mass of Leptarctus oregonensis; we used the regression formula for mustelids of Van Valkenburgh (Reference Van Valkenburgh1990). This new body mass estimate for L. oregonensis allowed us to calculate the maximum body size of its prey using the approach of Meers (Reference Meers2002). We used the “all predators” equation of Meers (Reference Meers2002, table 4) and corrected the estimate for logarithmic transformation bias using the ratio estimator correction factor calculated by Wilson et al. (Reference Wilson, Ekdale, Hoganson, Calede and Vander Linden2016).
We used the dry skull method of Thomason (Reference Thomason1991) to estimate the bite force of Leptarctus oregonensis. This approach has been successfully applied to both mustelids and fossil taxa by Wroe et al. (Reference Wroe, McHenry and Thomason2005) and Wilson et al. (Reference Wilson, Ekdale, Hoganson, Calede and Vander Linden2016). We used photos of the dorsal, ventral, and lateral views of UOMNH F-35458 (see Figs. 1, 2) to calculate the physiological surface area of the temporal and masseter muscles, the moments of the temporal and of the masseter, and the lengths of the carnassial out-lever and the M1 out-lever. All measurements were made on the better-preserved right side of the specimen. The surface area and position of the centroid of the muscles were estimated in ImageJ 1.51 (Schneider et al., Reference Schneider, Rasband and Eliceiri2012). We used a standard muscle stress value of 300kPa following Wroe et al. (Reference Wroe, McHenry and Thomason2005) and Wilson et al. (Reference Wilson, Ekdale, Hoganson, Calede and Vander Linden2016) to calculate the force of the masseter and temporal muscles. Because bite force scales with body mass (Meers, Reference Meers2002; Wroe et al., Reference Wroe, McHenry and Thomason2005; Wilson et al., Reference Wilson, Ekdale, Hoganson, Calede and Vander Linden2016), we estimated a bite force quotient (BFQ) at the carnassial for L. oregonensis and 30 other extant mammals using data and methodology from Wroe et al. (Reference Wroe, McHenry and Thomason2005). BFQs allow the comparisons of bite force across species of different body masses by using the residuals of a dataset-specific regression of the log of bite forces and log of body masses (Wroe et al., Reference Wroe, McHenry and Thomason2005). Our regression (R2=0.89, p=2.10−16) led us to estimate BFQ at the carnassial for our dataset using the following formula: BFQ=(bite force at the carnassial/10^(0.5906*Log (body mass)+1.9842))*100. The regression was run in R 3.3.1 (R Development Core Team, 2015) using RStudio 1.0.143 (RStudio, 2015). Although our formulas differ (they are dataset dependent), the BFQs we calculated are very similar to those calculated by Wroe et al. (Reference Wroe, McHenry and Thomason2005).

Figure 1 Skull of Leptarctus oregonensis, UOMNH F-35458: (1) dorsal view; (2) ventral view. Abbreviations: aud, auditory bulla; bac, basioccipital; euc, Eustachian canal; fr, frontal; fov, foramen ovale; glsq, glenoid fossa of the squamosal; iof, infraorbital foramen; ju, jugal; lam, lambdoidal crest; mas, mastoid process; max, maxilla; occ, occipital condyles; pal, palatine; par, parietals; poc, paroccipital process; pop, postorbital process; psag, parasagittal crest; pte, pterygoid flange; sq, squamosal; tym, tympanic projections. Scale bar is 1 cm.
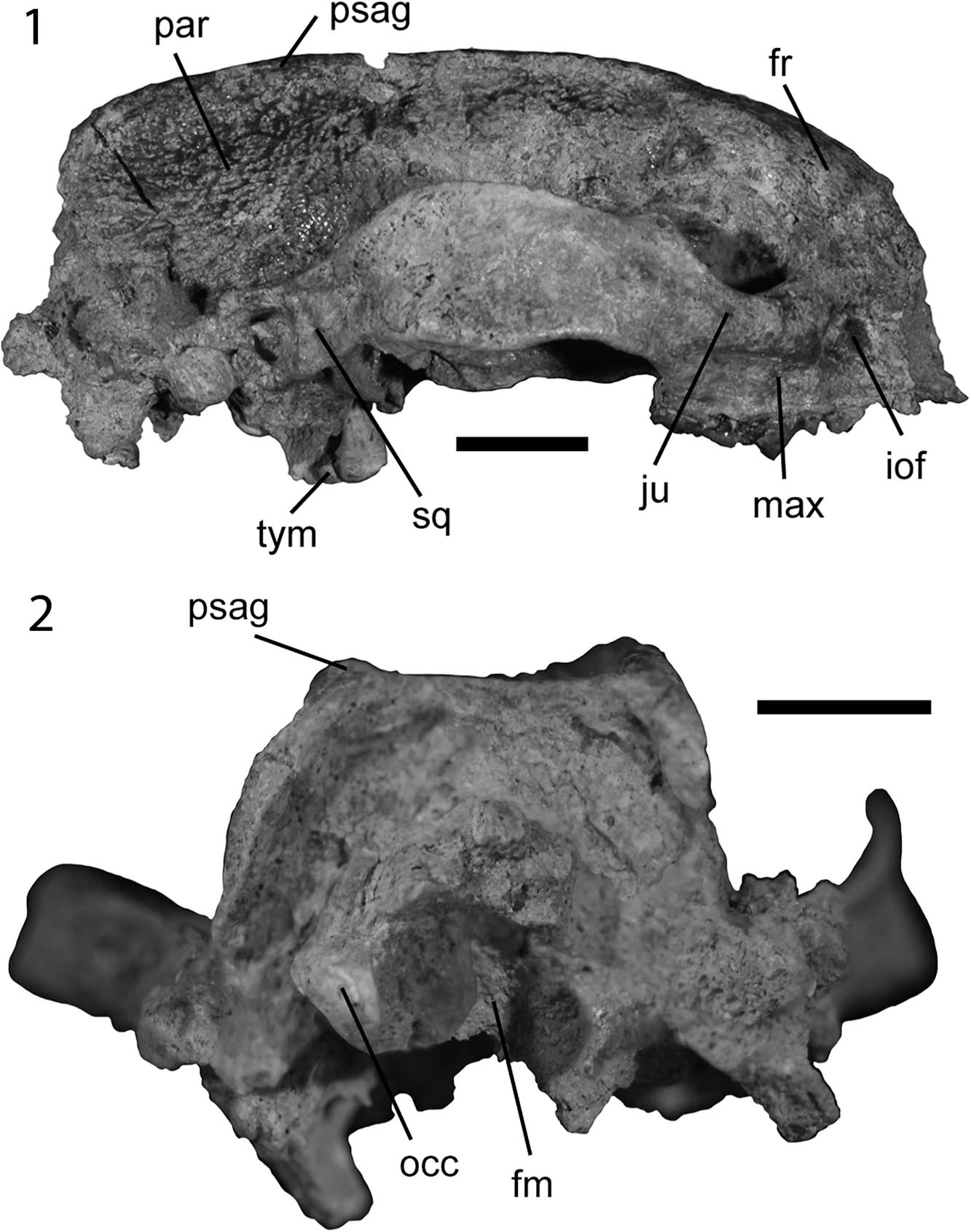
Figure 2 Skull of Leptarctus oregonensis, UOMNH F-35458: (1) right lateral view; (2) posterior view. Abbreviations: fm, foramen magnum; fr, frontal; iof, infraorbital foramen; ju, jugal; max, maxilla; occ, occipital condyles; par, parietals; psag, parasagittal crest; sq, squamosal; tym, tympanic projections. Scale bars are 1 cm.
We also investigated the proportions of the teeth of Leptarctus oregonensis and their possible link with diet by expanding upon a previous analysis by Popowics (Reference Popowics2003). We undertook a principal component analysis (PCA) and a canonical variate analysis (CVA) of five ratios of tooth measurements that describe the shape of the P4 and M1 of small carnivorans. We used the data from Popowics (Reference Popowics2003) and newly collected data for extant small carnivores (Table 2) measuring the P4 and M1 following the guidelines of Popowics (Reference Popowics2003). The PCA and CVA were run in R 3.3.1 (R Development Core Team, 2015) using RStudio 1.0.143 (RStudio, 2015), the package vegan 2.4-3 (Oksanen et al., Reference Oksanen, Blanchet, Kindt, Legendre, Minchin, O’Hara, Simpson, Solymos, Stevens and Wagner2015), and biostats (McGarigal, Reference McGarigal2015). We used a broken-stick distribution to determine the PCA axes whose eigenvalues were larger than expected by chance and only displayed those.
Table 2 Ratios of dental measurements used in assessing likely diet of Leptarctus oregonensis.

Abbreviations: P4LB, length of P4; P4W, width of P4; P4PM, length of basin of P4; PRBL, length of shearing blade of P4; M1BL, length of shearing surface of M1; M1LL, length of M1; M1W, width of M1. Sources: 1: Species average from Popowics (Reference Popowics2003), 2: UWBM 6418, 3: UWBM 41022, 4: UOMNH F-35458, 5:UWBM 41336, 6: UWBM28348, 7: UWBM 59961, 8: UWBM 51603, 9: UWBM 41393. New measurements were taken following the guidelines of Popowics (Reference Popowics2003). All measurements are in mm. Diet data are taken from Long (Reference Long1973), Popowics (Reference Popowics2003), Nowak (Reference Nowak2005), Figueirido et al. (Reference Figueirido, MacLeod, Krieger, De Renzi, Pérez-Claros and Palmqvist2011), and Myslajek et al. (Reference Myslajek, Nowak, Rożen and Jędrzejewska2013).
Repositories and institutional abbreviations
Types, figured, and other specimens examined in this study are deposited in the following institutions: American Museum of Natural History (AMNH), New York City, USA; Frick Collection of the American Museum of Natural History (F:AM), New York City, USA; Natural History Museum of Los Angeles County (LACM), Los Angeles, USA; University of California Museum of Paleontology (UCMP), Berkeley, USA; University of Florida (UF), Gainesville, USA; University of Oregon Museum of Natural and Cultural History (UOMNH), Eugene, USA; University of Washington Burke Museum of Natural History and Culture (UWBM), Seattle, USA.
Systematic paleontology
Nomenclature abbreviations
P4LB, length of P4; P4W, width of P4; P4PM, length of basin of P4; PRBL, length of shearing blade of P4; M1BL, length of shearing surface of M1; M1LL, length of M1; M1W, width of M1.
Class Mammalia Linnaeus, Reference Linnaeus1758
Order Carnivora Bowdich, Reference Bowdich1821
Family Mustelidae Fischer, Reference Fischer1817
Genus Leptarctus Leidy, Reference Leidy1856
Type species
Leptarctus primus Leidy, Reference Leidy1856, by original designation
Leptarctus oregonensis Stock, Reference Stock1930
1924 Leptarctus primus Reference MatthewMatthew, p. 139, figs. 37, 38.
1930 Leptarctus oregonensis Reference StockStock, Pl. I, fig. 1a, b, text-figures 1, 2.
1957 Leptarctus oregonensis; Reference OlsenOlsen, p. 2, fig. 2A.
1998 Leptarctus oregonensis; Reference BaskinBaskin, p. 158.
2001a Leptarctus primus (part); Reference Lim and MartinLim and Martin, p. 636.
2001 Leptarctus primus (part); Reference Lim, Martin and WilsonLim, Martin, and Wilson, p. 1044, fig. 1.
2002 Leptarctus primus (part); Reference Lim and MartinLim and Martin, p. 271, fig. 2B.
2005 Leptarctus oregonensis; Reference BaskinBaskin, p. 431.
2009 Leptarctus oregonensis; Reference Korth and BaskinKorth and Baskin, p. 29.
Holotype
Partial maxilla with the left P4–M1, the right P4, a partial zygomatic arch, and a cranial fragment including part of the lateral temporal crest (LACM (CIT) 206) from LACM CIT 113: exposures of Mascall deposits north of the east fork of the John Day River, ~2.4 km NW of Dayville, Oregon (Stock, Reference Stock1930).
Diagnosis
Smaller than L. ancipidens, L. mummorum Korth and Baskin, Reference Korth and Baskin2009, L. neimenguensis Zhai, Reference Zhai1964, L. primus, L. webbi Baskin, Reference Baskin2005, L. wortmani, and L. kansaensis Lim and Martin, Reference Lim and Martin2001a; rostrum proportionately shorter than in L. wortmani and L. mummorum; skull proportionately narrower, more rectangular in posterior view than that of L. mummorum; postorbital processes not as strong as those of L. mummorum; infraorbital foramen proportionately larger than in L. wortmani; optic foramen directed anterodorsally to the sphenorbital fissure unlike L. mummorum; parasagittal crests do not converge, unlike L. mummorum; post-glenoid foramen larger and located more laterally than in L. mummorum; parietals not as inflated as in L. primus; projections of the tympanic projection do not overlap, unlike in L. primus; tympanic projections present, unlike in L. webbi, and aligned with external auditory meatus, unlike in L. primus; hypocone of P4 smaller than in L. primus and L. ancipidens; metacone blade of P4 longer than in L. primus; tympanic projections do not extend posteriorly to edge of paroccipital process, unlike L. kansaensis; parastyle of P4 not as strong as in L. ancipidens; M1 roughly square, unlike L. ancipidens; no distinct metastyle adjoining the posterior border of the metacone on M1 unlike in L. neimenguensis; tympanic projection double (with a central foramen) unlike L. mummorum, L. wortmani, and L. supremus; paracone of P4 smaller and more acute than in L. desuii and L. martini; metacone of P4 longer and more blade-like than in L. desuii and L. martini; parastyle of M1 longer and more-blade like than in L. martini; P4 not wider than long, unlike in L. progressus.
Occurrence
Early Barstovian of the Mascall Formation of Oregon; early Barstovian of the Olcott Formation of Nebraska.
Description
UOMNH F-35458 is a nearly complete skull. The rostrum—the only cranial material represented by the type specimen—is missing, broken anteriorly to P3. The upper canines and incisors are also absent. The P4 and M1 are preserved. The skull is medio-laterally broad and anteroposteriorly short (Figs. 1, 2). It exhibits the strong parasagittal crests and zygomatic arches characteristic of Leptarctus (Korth and Baskin, Reference Korth and Baskin2009). It is smaller in both length and width (SL and WZA, Table 1) than L. ancipidens, L. mummorum, L. supremus, L. primus, L. martini, L. neimenguensis, L. webbi, and L. wortmani (see Olsen, Reference Olsen1958; Zhai, Reference Zhai1964; Lim and Miao, Reference Lim and Miao2000; Lim et al., Reference Lim, Martin and Wilson2001; Baskin, Reference Baskin2005; Korth and Baskin, Reference Korth and Baskin2009). Based on an occiput to orbit length of 60.9 mm, we calculated a mass of 2.2 kg for L. oregonensis, which is comparable to the mass of Mephitis mephitis Schreber, Reference Schreber1776, the striped skunk, which weighs up to 2.5 kg (Nowak, Reference Nowak2005), or the mass of a medium-sized Martes (martens and sable; Nowak, Reference Nowak2005). At its widest point the skull is 60–65% as wide as it is long, although the skull is broken anteriorly and the total length of the skull is therefore unknown. The bones of the skull are overall well fused such that the sutures can hardly be observed. The cranial morphology of UOMNH F-35458 is broadly similar to AMNH 18241, a skull of L. oregonensis from the Olcott Formation, although the UOMNH specimen is not as deformed taphonomically.
The frontals are smooth and convex dorsally (Figs. 1.1, 3). They are slightly flatter in UOMNH F-35458 than in AMNH 18241. They are fused medially in a groove extending anteroposteriorly. The frontals are damaged in UOMNH F-35458 but can be observed in AMNH 18241 in which they display a mid-sagittal ridge originating in the groove between the paired frontals and extending posteriorly to the fusion between the lambdoidal crests. The fossae on either side of this mid-sagittal ridge can be observed in UOMNH F-35458. Anteriorly, the infraorbital foramen is round in UOMNH F-35458 (Fig. 3) and more teardrop-shaped in AMNH 18241, with the broad end lateral and the acute angle medial. The jugal is very strong and thickened dorsally and posteriorly to the infraorbital foramen (Fig. 2.1). The roots of the cheek teeth extend dorsally, into the upper jaw, up to the ventral margin of this thickening. The orbit is small relative to skull size and round (Table 1, Fig. 3). The postorbital processes of the frontal and jugal are triangular with those of the frontal longer and more acute than those of the jugal (Fig. 1.1). They are not as strong as the postorbital process of L. mummorum (Korth and Baskin, Reference Korth and Baskin2009). The suture between the jugal and the squamosal cannot be easily distinguished in UOMNH F-35458 but is apparent in AMNH 18241 posteriorly to the postorbital process of the jugal. The dorso-ventrally tallest point of the zygomatic arch is part of the squamosal. Both specimens display a concavity in the squamosal ventral to the tallest point of the zygomatic arch and a convexity (bump) ventral to the postorbital process of the jugal (Fig. 3).

Figure 3 Oblique view of the skull of Leptarctus oregonensis, UOMNH F-35458. Scale bar is 1 cm.
The zygomatic arch is dorsally curved. It is also medio-laterally thickened ventrally (Fig. 1.2). This strong zygomatic arch is the origin for an enlarged masseter muscle, a jaw-closing muscle (Hall, Reference Hall1926; Davis, Reference Davis1964; Riley, Reference Riley1985). The glenoid fossa of the squamosal is medio-laterally broad, extending from the lateral end of the squamosal medially to the middle of the auditory bulla and aligned with the buccal cusps of M1. The robust pre- and post-glenoid processes support a strong articulation with the dentary (Peigné et al., Reference Peigné, Salesa, Antóm and Morales2005). The post-glenoid process is stronger than the pre-glenoid and the glenoid fossa is frontally oriented. The posterior end of the zygomatic arch contacts the anterior edge of the bulla as well as the anterolateral end of the lambdoidal crest, which ends at a ball-like structure: the mastoid process. The mastoid process is connected by a ridge of bone extending posteriorly to a process located posteriorly to the auditory bulla. The external opening of the auditory meatus is located just anterior to the mastoid process and surrounded ventrally by a thickened lip of bone. The mastoid process is larger in UOMNH F-35458 than in AMNH 18241.
The foramina of the orbital region could only be observed on UOMNH F-35458 and cannot be described in AMNH 18241. The lacrimal bears two foramina dorsal to the infraorbital foramen. The nasolacrimal foramen is round and located dorsally to the infraorbital foramen. The canal extends ventrally from the foramen. The vascular foramen of the lacrimal is located immediately dorso-medially to the infraorbital foramen. It is as large as the nasolacrimal foramen. This foramen is present in most species of Leptarctus, including L. mummorum (Korth and Baskin, Reference Korth and Baskin2009). The minute foramen dorsal to the nasolacrimal foramen described by Korth and Baskin (Reference Korth and Baskin2009) in L. mummorum and purportedly not present in any other Leptarctus can be observed in UOMNH F-35458. The round sphenopalatine foramen is posterodorsal to M1, medial to the posterolateral edge of the palate. No ethmoid foramen could be distinguished. The optic foramen is small and round, and unlike in L. mummorum, is not directly anterior to the sphenorbital fissure, but rather anterodorsal to it. The sphenorbital fissure itself is several times broader at its anterior opening than at its posterior one. The foramen rotundum, located posteriorly to the sphenorbital fissure, is broader at its posterior end than at its anterior one.
The skull of Leptarctus oregonensis from Oregon, UOMNH F-35458, bears two prominent parasagittal crests extending anteroposteriorly from just anterior to the postorbital processes of the frontal to the lambdoidal crests (Fig. 1.1). They only extend posterior to the postorbital processes of the frontals in AMNH 18241 from the Olcott Formation (Lim et al., Reference Lim, Martin and Wilson2001, fig. 1). The parasagittal crests are parallel to one another in UOMNH F-35458, do not converge (unlike in L. mummorum; Korth and Baskin, Reference Korth and Baskin2009), and are taller posteriorly. The postorbital pinching of the frontals is stronger in AMNH 18241 than in UOMNH F-35458. In AMNH 18241, the parasagittal crests diverge posteriorly.
The left parietal of UOMNH F-35458 is missing but the right one can be observed. Both are preserved in AMNH 18241. The parietals are laterally inflated, although not as much as in Leptarctus primus (Fig. 1.1). The dorsal surface of the parietals is roughened (Fig. 2.1). This roughening is more accentuated posteriorly, closer to the lambdoidal crests. The bone there is porous and textured alike an irregular lattice. Lim et al. (Reference Lim, Martin and Wilson2001) also described roughening of the parietal and occipital bones in Leptarctus supremus. The intensity of the roughening is similar in both L. oregonensis specimens and between sides of a single specimen, but the pattern of the lattice differs both within (i.e., between left and right sides) and across specimens. This roughening of the parietals is evidence of the attachment of a large jaw-closing temporalis muscle (Riley, Reference Riley1985; Vizcaíno et al., Reference Vizcaíno, De Iuliis and Bargo1998). The presence of parasagittal and lambdoidal crests increases the surface area for the origin of this temporalis muscle, as in the giant panda (Davis, Reference Davis1964).
In posterior view (Fig. 2.2), the skull is rectangular, much more so than Leptarctus mummorum. The lambdoidal crests meet dorsally and extend ventrally along the sides of the skull thinning ventrally until the mastoid process connected to the basicranium. With the exception of the occipital condyles, the occipital region is concave. The occipital condyles are enlarged compared to other similarly sized mustelines. The dorsal side of the foramen magnum is surrounded by a bony lip connecting posteroventrally to the skull. The two occipital condyles project posteriorly as in other species of Leptarctus (Olsen, Reference Olsen1958; Lim et al., Reference Lim, Martin and Wilson2001; Korth and Baskin, Reference Korth and Baskin2009), but also display a smooth articular surface medially.
The ventral surface of the skull, particularly the basicranium, is better preserved in the new skull from the Mascall Formation of Oregon (UOMNH F-35458) than in the specimen from the Olcott Formation of Nebraska (AMNH 18241). The following description is based on the specimen from Oregon except when specifically referencing the specimen from Nebraska. In ventral view (Fig. 1.2), both the maxilla and the palatine are slightly concave in UOMNH F-35458. The maxilla of AMNH 18241 also displays a concavity. The anterior border of the suture between the maxilla and palatine is at the mesial end of P4. The lateral branches of the maxilla and palatine suture extend just medial to M1. The posterior palatine foramen cannot be distinguished. The palatine is much narrower posterior to the tooth row and ends with the pterygoid flanges. The pterygoid flanges are curved and extend posteriorly; they are tall (Table 1) and thickened anteriorly (Fig. 1.2). There is a ridge of bone lateral to this thickening, parallel to the pterygoid flange. The size and thickening of the pterygoid and the presence of this bony ridge may indicate an enlarged pterygoideus muscle, a jaw-closing muscle (Hall, Reference Hall1926; Davis, Reference Davis1964; Endo et al., Reference Endo, Taru, Yamamoto, Arishima and Sasaki2003).
A ridge also extends medially to the pterygoid flanges, starting at the posterior end of the palatine and ending at the posterior end of the basioccipital. It is tall and medio-laterally compressed anteriorly but is low and broadened posteriorly. The suture on the right flange of the pterygoid indicates that both the palatine and the basisphenoid contribute to the pterygoid flange. The foramen ovale of the basisphenoid is within the size range of that of L mummorum (Korth and Baskin, Reference Korth and Baskin2009). The bone ridge lateral to the pterygoid flange extends lateral to the foramen ovale and contacts the auditory bulla at the posterior end of the foramen. The foramen is teardrop shaped with the broader end located posteriorly; the opposite end extends between the pterygoid flange and the ridge of bone. Laterally to the foramen ovale and medially to the glenoid fossa of the squamosal is a narrow concave bone surface (Fig. 1.2). The squamosal wraps around the fossa farther anteriorly and ventrally in UOMNH F-35458 than in AMNH 18241, which is evidence for a tighter dentary-squamosal articulation in the Mascall specimen. The eustachian canal is located anteriorly to the bulla and posterior to the foramen ovale. It is small, but slightly larger in UOMNH F-35458 than in AMNH 18241. As mentioned by Korth and Baskin (Reference Korth and Baskin2009) for other specimens, there is no evidence for a foramen anterior to the paroccipital process and posterior to the auditory bulla in either one of the adult skulls (UOMNH F-35458 or AMNH 18241). This foramen would be the entrance of the auricular branch of the vagus nerve, purportedly lost during ontogeny in Leptarctus (Korth and Baskin, Reference Korth and Baskin2009). The paroccipital process of L. oregonensis, visible in UOMNH F-35458 (Fig. 1.2), is likely the site of the origin of a powerful digastric muscle, as in other members of Mustelidae, Mephitis, the giant panda, and some felids (Hall, Reference Hall1926; Davis, Reference Davis1964; Riley, Reference Riley1985; Antón et al., Reference Antón, Salesa, Pastor, Sánchez, Fraile and Morales2004). The strong mastoid process of UOMNH F-35458 would have provided additional surface for the origin of a powerful digastricus muscle as it does in Mephitis, Spilogale, and Martes (Hall, Reference Hall1926). The digastricus muscle depresses the mandible, opening the mouth (Antón et al., Reference Antón, Salesa, Pastor, Sánchez, Fraile and Morales2004). In Enhydra, the sea otter, the mastoid also provides surface attachment for the temporalis and the horizontal portion of the masseter muscles (Riley, Reference Riley1985), giving the animal a greater bite force at the canines and/or cheek teeth than in relatives without this expanded muscle attachment area (e.g., Lontra), although body mass may also play a role in this increased bite force (Riley, Reference Riley1985).
Posterior to the glenoid fossa of the squamosal is an oval, anterolaterally to medio-laterally oriented post-glenoid foramen (Fig. 1.2). It is larger and located more laterally than in L. mummorum (Korth and Baskin, Reference Korth and Baskin2009). The auditory bulla is fully fused to the base of the skull, as in Leptarctus mummorum. The auditory bulla is narrow medio-laterally. It bears a peculiar process extending anteroventrally (Figs. 1.2, 2.1, 4). This is the bullar process of Korth and Baskin (Reference Korth and Baskin2009), also called tympanic projection by Lim and Martin (Reference Lim and Martin2001a). This is a common feature of the genus Leptarctus, with the exception of L. webbi, which lacks processes (Baskin, Reference Baskin2005). Overall, the tympanic projection of L. oregonensis is most similar to that of L. ancipidens (see Olsen, Reference Olsen1958). It is very tall and its ventral edge is the ventral-most feature of the skull (Fig. 2.1). The posterior side of the bulla bears two parallel ridges extending dorso-laterally to ventro-medially. These two ridges are thicker on their dorsal-most end and thinner towards their attachment to the bulla. The anterior process of the bulla (Fig. 4) consists of a series (unlike the simple processes of L. mummorum and L. supremus; Lim et al., Reference Lim, Martin and Wilson2001; Korth and Baskin, Reference Korth and Baskin2009) of non-overlapping (unlike L. primus, but as in L. ancipidens; Olsen, Reference Olsen1958; Lim and Martin, Reference Lim and Martin2001a) anteroventral projections, the two anterior-most of which are separated by a canal, as in L. ancipidens (see Olsen, Reference Olsen1958) and L. primus (Lim and Martin, Reference Lim and Martin2001a). The tympanic projection is broken posterior to a third damaged projection. Additional projections can be distinguished at the dorsal end of the posterior surface of the tympanic projection, forming lobes ventral to the paroccipital process (Fig. 4; jugular process of Lim and Martin, Reference Lim and Martin2001a). These lobes and the third projection (posterior to the ones than form the canal) are not present in other species of Leptarctus. The contact between the two projections and the canal are aligned with the external auditory meatus, located dorso-posteriorly to the tympanic projection, as in L. ancipidens. This state is unlike L. primus, where the contact and canal are aligned with the postglenoid foramen, located anterodorsally to the tympanic projection farther from the mastoid process than the external auditory meatus. As in L. primus, but unlike L. kansaensis, the tympanic projections of L. oregonensis do not extend posteriorly to the edge of the paroccipital process (Lim and Martin, Reference Lim and Martin2001a). The ventral end of the tympanic projections is smooth and superficially resembles a condyle. The tympanic projections bear a number of round to oval holes, although those seem to be taphonomic in origin, as interpreted from their location and structure (Fig. 4). The medial edge of the bulla is not preserved and cannot be described.

Figure 4 Tympanic projections of the auditory bullae of Leptarctus oregonensis (UOMNH F-35458): (1) medial view; (2) lateral view. White * denote the taphonomic damage mentioned in the text. Scale bar is 5 mm.
The lobate shape of the tympanic projections ventral to the paroccipital process, combined with the nearby, prominent mastoid process, may have provided L. oregonensis additional surface attachment for jaw muscles involved in crushing and chewing. Olsen (Reference Olsen1958) suggested that they may have served as surface attachment for the digastric muscles. In some felids, the auditory bulla is an area of insertion for the digastric muscle (Antón et al., Reference Antón, Salesa, Pastor, Sánchez, Fraile and Morales2004). Korth and Baskin (Reference Korth and Baskin2009) pointed out that a raphe from the masseter attaches just lateral to the auditory bulla in Canis, and proposed that the tympanic projections of Leptarctus provided surface area for the attachment of the masseter. Whether the masseter, or the digastricus, or both attached onto the tympanic projections of Leptarctus, it is apparent that these projections provided critical surface area for the attachment of muscles important in jaw action.
The teeth are unworn with most cusps fully intact (Fig. 5). The dental morphology most closely resembles that of L. ancipidens, but is smaller in size (Table 1). The M1 is longer than in many mustelids of similar skull size (Table 1, Fig. 6). None of the teeth anterior to P3 is preserved. Only the left P3 is present. Only partial roots of the right P3 are preserved. There are two roots with the posterior larger than the anterior of the right P3. It has a single, conical cusp, almost as high as the paracone of P4 (Fig. 6). The cusp is surrounded by a cingulum that is most prominent on the anterior and posterior ends of the tooth. The overall shape of the P4 is a rounded square with a metacone blade that projects posterobuccally (Fig. 6). The dental morphology of UOMNH F-35458 is similar overall to that of the type specimen, LACM (CIT) 206, both in shape and size (Table 1). There are differences in the proportions of the teeth between the specimens from the Olcott Formation and those from the Mascall Formation. The P4 of AMNH 18241 from Nebraska is shorter and wider than the P4s of the specimens from Oregon (Table 1). The M1 of AMNH 18241 is longer and wider than that of LACM (CIT) 206. The P4 of UOMNH F-35458 resembles closely the P4 figured by Downs (Reference Downs1956), UCMP 39102. It is longer than it is wide, unlike in L. progressus (Table 1; Lim and Martin, Reference Lim and Martin2001a). All cusps, except the hypocone of the left P4 and the metacone of the right P4, are preserved (Fig. 6). Similarly to L. mummorum, P4 has a prominent parastyle at the anterior margin of the tooth that is subequal in height to the protocone. This parastyle, as noted by Downs (Reference Downs1956) and Olsen (Reference Olsen1957) is not as strong as in L. ancipidens. Two strong cingula extend from this parastyle to the anterior margin of the protocone and the anterobuccal margin of the paracone, respectively. The paracone is the largest and most robust cusp of the P4 and, indeed, of the whole tooth row. The paracone is smaller and more acute than in L. desuii and L. martini (Lim and Martin, Reference Lim and Martin2001b; Fig. 6). A paraloph (a crest attaching the paracone to the protocone) closes off a valley between the paracone, parastyle, and protocone (Fig. 6). The protocone is located slightly anterior to the paracone. It is higher than the hypocone. The hypocone of L. oregonensis is small compared to those of L. primus (as noted by Korth and Baskin, Reference Korth and Baskin2009) and L. ancipidens (see Downs, Reference Downs1956; Olsen, Reference Olsen1957). The posterior end of the protocone is connected to the anterior end of the hypocone, although this connection is smaller and less robust than the aforementioned cingula or paraloph. There is a triangular valley between the hypocone, paracone, and protocone (Fig. 6). Another valley posterior to the hypocone is formed by the paracone, the hypocone, and the blade-like metacone. This metacone blade is longer than in L. primus, as noted by Korth and Baskin (Reference Korth and Baskin2009). A cingulum extends posteriorly to the hypocone around the posterior end of the metacone and terminates on the posterior-buccal side of the paracone. The metacone of P4 is the buccal-most cusp of the tooth row and, at its posterior end, contacts the parastylar crest of M1 (Fig. 6). It is a robust and tall blade, longer and more blade-like than in L. desuii and L. martini (Lim and Martin, Reference Lim and Martin2001b; Fig. 6). The overall shape of the M1 is a rounded square with a projection formed by the paracone and parastylar crest that extends anterobuccally and contacts the projecting metacone of the P4 (Fig. 6). The parastyle of M1 is longer and more-blade like in L. oregonensis than in L. martini (Table 1, Fig. 6).

Figure 5 Occlusal view of the dentition of Leptarctus oregonensis, UOMNH F-35458: (1) right partial P4 and partial M1; (2) left P3, partial P4, and partial M1 (reflected). Scale bar is 2 mm.

Figure 6 Occlusal view of the dentition (P3–M1) of Leptarctus: (1) L. oregonensis (UOMNH F-35458, composite of left and right dentitions); (2) L. ancipidens (UF 5706; redrawn from Lim et al., Reference Lim, Martin and Wilson2001); (3) L. martini (UNSM 20843). Scale bar is 5 mm. The dentition of AMNH 18241 is figured in Matthew (Reference Matthew1924).
Leptarctus oregonensis differs from L. ancipidens in that the M1 is wider than long in the latter (see Downs, Reference Downs1956; Olsen, Reference Olsen1957; Table 1). The left M1 is missing two of the lingual cusps (protocone and hypocone). The right M1 preserves all cusps except the hypocone and the anterior surface of the metaconule (see Korth and Baskin, Reference Korth and Baskin2009 for a discussion of the metaconule in the genus Leptarctus). The four main cusps of M1 (paracone, metacone, protocone, hypocone) are subequal in heightm as in L. mummorum. There are two main valleys. The largest, deepest valley is located between the paracone, protocone, and metacone. The other, smaller valley is located posteriorly, between the metacone, metaconule, and the posterior edge of the tooth (Fig. 6). There is a strong parastylar crest (anteroexternal cingulum of Korth and Baskin, Reference Korth and Baskin2009) subequal in height to the paracone and located directly anterobuccally to it. The paracone connects to the protocone via a robust paraloph. A robust cingulum extends along the buccal margin of the tooth and connects posteriorly to the posterior end of the metacone. The lingual cingulum of the M1 of L. oregonensis is weak compared to those exhibited by L. ancipidens and L. martini. The paracone and metacone are very similar in shape.
Referred specimens
From the Mascall Formation, Oregon: UCMP 39102, a single right P4 from the Mascall type area (UCMP V4824, Mascall 10) (by Downs, Reference Downs1956); UOMNH F-35458 (UO 2993, Field Creek Road), mostly complete skull including the right P4 and M1, and the left P3, P4, and M1, but lacking the rostrum, incisors, and canines; from the Olcott Formation, Nebraska: AMNH 18241, almost complete skull of Leptarctus oregonensis lacking incisors and canines, AMNH 18270, partial right lower jaw, both specimens from the Snake Creek Quarries (23 miles south of Agate); from the Sand Canyon Beds Formation (Observation Quarry), Nebraska: F:AM 25385, partial cranium lacking the left auditory bulla, the incisors, and canines; from the Lower Snake Creek Beds (Echo Quarry): F:AM 25261, partial posterior portion of the cranium.
Remarks
Since publication of Leptarctus oregonensis by Stock (Reference Stock1930) and Downs (Reference Downs1956), many other species of Leptarctus have been recognized (Korth and Baskin, Reference Korth and Baskin2009), but no differential diagnosis with L. oregonensis has been provided for many of them. The completeness of the new specimen, UOMNH F-35458, also offers the opportunity to consider how many characters (e.g., projections of the auditory bullae) unknown for L. oregonensis before differ from other species of the genus. For these two reasons, we chose to provide an emended diagnosis.
Results of the quantitative analyses
We estimate that Leptarctus oregonensis would have been able to feed on prey as large as 1.85 kg (95% confidence intervals of 0.94–3.60 kg). We calculated a bite force at the carnassials of 136.45 N, and a bite force at the M1s of 177.15 N (Table 3). Our calculation of the BFQ at the carnassials of L. oregonensis yields a value of 89. Of the 30 extant mammals included in our analysis, 22 have a higher BFQ than L. oregonensis. Nonetheless, L. oregonensis has a higher BFQ than the only other mustelid included in our dataset, Meles meles Linnaeus, Reference Linnaeus1758, the European badger (BFQ of 86). The BFQ of L. oregonensis is intermediate between that of Canis aureus Linnaeus, Reference Linnaeus1758, the golden jackal (BFQ of 87), and Lynx rufus Schreber, Reference Schreber1777, the bobcat (BFQ of 90) (Table 4). The proportions of the teeth of mustelids are associated to their diets (Popowics, Reference Popowics2003). Our analysis of tooth shape (Fig. 7) supports broad differences in tooth morphology between carnivorous taxa whose diet is dominated by vertebrates and those that are omnivorous or invertivorous, although there are exceptions to this pattern (Popowics, Reference Popowics2003). Thus, omnivorous and invertivorous taxa are generally characterized by large crushing surfaces on both P4 (PRBL/P4W) and M1 (M1LL/M1W), whereas carnivorous taxa are usually typified by high values of carnassial shear (P4LB/P4W) and carnassial blade length (P4PM/P4W) (Table 5; Fig. 7.1; Popowics, Reference Popowics2003). The proportions of the P4 and M1 of Leptarctus oregonensis are most similar to those of invertivorous and omnivorous taxa (Fig. 7.1). Our canonical variate analysis (Table 5; Fig. 7.2) further supports this conclusion. The proportions of the teeth of L. oregonensis most closely resemble those of Mephitis mephitis, the striped skunk, Spilogale putorius Linnaeus, Reference Linnaeus1758, the eastern spotted skunk, and Mydaus javanensis Desmarest, Reference Desmarest1820, the Indonesian stink badger (Fig. 7.1). All three of these taxa eat large amounts of invertebrates, small amounts of plant materials, and small vertebrates (Wade-Smith and Verts, Reference Wade-Smith and Verts1982; Kinlaw, Reference Kinlaw1995; Hwang and Larivière, Reference Hwang and Larivière2003; Nowak, Reference Nowak2005).

Figure 7 Analysis of the proportions of P4 and M1 of Leptarctus oregonensis: (1) plot of the Principal Component Analysis of dental ratios. The smaller gray squares indicate the eigenvectors (labeled in gray); (2) plot of the Canonical Variate Analysis. The colors of the circles reflect the diets of the extant carnivores included in the analysis: black: hypercarnivore; dark gray: vertivore; gray: vertivore/invertivore; light gray: invertivore; white, omnivore. Leptarctus oregonensis is indicated by a white square. Abbreviations: Ac, Aonyx capensis Cuvier, Reference Cuvier1825; Al, Arctonyx collaris Cuvier, Reference Cuvier1825; Ba, Bassariscus astutus Lichtenstein, 1825; Eb, Eira barbara Linnaeus, Reference Linnaeus1758; El, Enhydra lutris Linnaeus, Reference Linnaeus1758; Gg, Gulo gulo Linnaeus, Reference Linnaeus1758; Is; Ictonyx striatus Perry, Reference Perry1810; Lc, Lontra canadensis Schreber, Reference Schreber1776; Ma, Martes americana Turton, Reference Turton1806; Mc, Mellivora capensis Schreber, Reference Schreber1776; Ml, Meles meles; Mm, Mephitis mephitis; Mj, Mydaus javanensis; Mp, Mustela putorius; Nv, Neovison vison Schreber, Reference Schreber1777; Pl, Procyon lotor; Sp, Spilogale putorius; Tt, Taxidea taxus.
Table 3 Estimate of bite force in Leptarctus oregonensis. BFQ is the bite force quotient (see text for details).

Table 4 Bite force quotients (BFQ) of the 30 extant mammals included in our analysis and Leptarctus oregonensis.

Abbreviations: A, animal-dominated diet; BF, bite force at the carnassials (N); BM, body mass (kg); BFQ, bite force quotient; LBF, log of bite force; LBM, log of body mass; O, omnivore; P, plant-dominated diet. Body mass and bite force data are from Wroe et al. (Reference Wroe, McHenry and Thomason2005), except for Leptarctus oregonensis. Diet data are from Fritzell and Haroldson (Reference Fritzell and Haroldson1982), Larivière and Pasitschniak-Arts (Reference Larivière and Pasitschniak-Arts1996), Koler-Matznick et al. (Reference Koler-Matznick, Lehr Brisbin, Feinstein and Bulmer2003), Nowak (Reference Nowak2005), and Roberts et al. (Reference Roberts, Somers, White and Nel2007).
* = The taxonomy of this species is contested (see Koler-Matznick et al., Reference Koler-Matznick, Yates, Bulmer and Brisbin2007).
Table 5 Scores of taxa included in the principal component analysis and the canonical variate analysis as well as eigenvector values of morphological indices included in the principal component analysis.

Discussion
Despite being known from several partial skulls prior to our study, Leptarctus oregonensis was a poorly understood species. Korth and Baskin (Reference Korth and Baskin2009) provided an important systematic review of the leptarctines, yet the details of the morphology of this group and the consequent systematic and ecological implications have remained unclear. The material we describe provides new information that, along with previously published and unpublished data, sheds some light on the relationships and ecology of L. oregonensis. The dental and bullar morphologies of mustelids are critical to understanding their phylogenetic relationships (Wolsan, Reference Wolsan1993; Wang et al., Reference Wang, Qiu. and Wang2004; Robles et al., Reference Robles, Alba, Moyà-Solà, Casanovas-Vilar, Galindo, Rotgers, Almécija and Carmona2010). UOMNH F-35458 provides new information on the morphology of the tympanic projections of L. oregonensis. These tympanic projections are most similar to those of L. ancipidens from the Hemingfordian of Florida. Nevertheless, the tympanic projections of the two taxa differ by the complex dorso-posterior morphology of the projections in L. oregonensis. This morphology is unique to L. oregonensis. The dental morphology of L. oregonensis is also most similar to that of L. ancipidens. These combined morphological similarities in bullar and dental morphology likely reflect a close relationship between L. oregonensis and L. ancipidens. These two taxa have been suggested before to be synonyms (Olsen, Reference Olsen1957), but Baskin (Reference Baskin1998, Reference Baskin2005) and Korth and Baskin (Reference Korth and Baskin2009) recognized L. oregonensis and L. ancipidens as different based on the more primitive dentition and the larger size of L. ancipidens (Korth and Baskin, Reference Korth and Baskin2009). The different morphologies of the tympanic projections of the bullae we describe herein confirm that latter interpretation. The two taxa are also differentiated by dental characters of the P4 and M1, including a weaker parastyle and smaller hypocone in the P4 of L. oregonensis and a M1 wider than long with a stronger lingual cingulum in L. ancipidens than in L. oregonensis (Downs, Reference Downs1956; Olsen, Reference Olsen1957).
In addition to interspecific differences, UOMNH F-35458 also provides the opportunity to assess the intraspecific variation within Leptarctus oregonensis. UOMNH F-35458 cannot be distinguished in dental or cranial morphology from the type specimen LACM (CIT) 206. However, there are several differences in cranio-dental morphology between UOMNH F-35458 and AMNH 18241. These differences are concentrated in the frontals, cranial foramina, auditory region, and the P4. The frontals of the Olcott Formation specimen are flatter than those of the newly described Mascall specimen. The parasagittal crests they bear originate farther posteriorly in the Olcott Formation specimen and diverge posteriorly rather than remaining parallel to one another as in UOMNH F-35458. The infraorbital foramen is rounder and larger in UOMNH F-35458. The mastoid process and the eustachian canal are larger in UOMNH F-35458. The dentary-squamosal articulation is stronger in UOMNH F-35458. The width of the posterior-most basin of P4 of the Olcott and Mascall specimens differ with the former in exhibiting a larger basin. The paracone is higher in UOMNH F-3554. Additionally, the proportion of the M1 differs between the two specimens, with the molar of the Mascall specimen squarer than that of the Olcott Formation specimen.
Nevertheless, the two specimens likely represent individuals from a single species. Korth and Baskin (Reference Korth and Baskin2009) proposed that there could be sexual dimorphism in Leptarctus oregonensis. They suggested that this sexual dimorphism might involve L. primus and L. oregonensis. The combination of differences in the tympanic and dental morphologies between the two taxa supports those as strictly different species. It may be that the differences in morphology between the specimens from the Mascall and Olcott formations represent sexual dimorphism, which has been documented in many species of extant mustelids (Lee and Mill, Reference Lee and Mill2004 and references therein). However, the concurrent morphologies among the Mascall specimens and among the Great Plains specimens may support, instead, the hypothesis that this intraspecific variation represents variation over the species’ geographical or temporal range (the Olcott and Mascall specimens may not be exactly contemporaneous). Additional specimens are needed to settle this issue.
This additional material of Leptarctus oregonensis suggests that early leptarctines were crushing animal-dominated omnivores. The skull of L. oregonensis is broad across the zygomatic arches. Broad crania are typically associated with large jaw-adducting muscles, high bite forces, and the ability to bite into hard objects (Goswami et al., Reference Goswami, Milne and Wroe2011). Multiple lines of evidence for strong jaw-closing and jaw-opening muscles (i.e., pterygoideus, temporalis, digastricus, and masseter) further support a high bite force suggestive of crushing in L. oregonensis. Based on comparisons with extant mustelids, the presence of a sagittal crest, strong-lipped and narrow glenoid fossae of the squamosals, small orbits, and dorsally curved zygomatic arches in L. oregonensis supports a carnivorous diet for this mustelid (Dumont et al., Reference Dumont, Wall, Botton-Divet, Goswami, Peigné and Fabre2016). The short snout of L. oregonensis (based on LACM CIT 206) is consistent with the interpretation of L. oregonensis as a carnivorous species with a strong bite force (Goswami et al., Reference Goswami, Milne and Wroe2011; Dumont et al., Reference Dumont, Wall, Botton-Divet, Goswami, Peigné and Fabre2016). Our estimate of the bite force relative to body mass of L. oregonensis indicates that although not durophagous, it had a stronger bite force than the extant European badger (Meles meles). This high bite force is consistent with an animal-dominated diet. Wroe et al. (Reference Wroe, McHenry and Thomason2005) showed that carnivorous species tend to have higher BFQs than omnivorous species. All of the omnivores and animals with plant-dominated diets included our analysis have lower BFQs than L. oregonensis (Table 4). The bite force of L. oregonensis is superior to that of the golden jackal, Canis aureus, a taxon that feeds on small mammals, large ones (gazelles), other vertebrates, insects, and fruits (Nowak, Reference Nowak2005). The bite force of L. oregonensis is most similar to that of Lynx rufus, a carnivorous taxon that feeds mostly on small mammals and birds, but is capable of hunting deer, a much larger prey than itself (Nowak, Reference Nowak2005). Thus, it seems that L. oregonensis would have been able to subdue small vertebrates as well as vertebrate prey larger than itself, and had a powerful bite force conferring it considerable crushing ability.
The tooth morphology of L. oregonensis also supports the interpretation of this fossil mustelid as a crushing animal. The protocone of P4 is robust, a feature associated with crushing behavior (Valenciano et al., Reference Valenciano, Abella, Sanisidro, Hartstone-Rose, Álvarez-Sierra and Morales2015). Although L. oregonensis lacks a strong lingual cingulum on M1 (unlike L. martini, for example), another feature associated with durophagy (Valenciano et al., Reference Valenciano, Abella, Sanisidro, Hartstone-Rose, Álvarez-Sierra and Morales2015), it does bear a strong hypocone on that tooth. The presence of a strong hypocone on M1 is indicative of crushing in carnivorans (Hunter and Jernvall, Reference Hunter and Jernvall1995; Popowics, Reference Popowics2003). Despite being often associated with herbivory, a hypocone is also found in a number of generalist and even a few faunivorous carnivorans (Hunter and Jernvall, Reference Hunter and Jernvall1995). The presence of a hypocone in L. oregonensis therefore cannot be interpreted as evidence for herbivory. Nevertheless, L. oregonensis possesses large crushing surfaces on both M1 and P4 that suggest the ability to process invertebrate cuticles and plant material. This crushing tooth morphology is associated with a long P4 blade that usually is interpreted as evidence for carnivory (Friscia et al., Reference Friscia, Van Valkenburgh and Biknevicius2006). Thus, the skull morphology and some aspects of the tooth morphology of L. oregonensis are consistent with a carnivorous diet, while other aspects of dental morphology suggest the consumption of invertebrates and possibly plant material.
This mosaic of dental characters in Leptarctus oregonensis may reflect the ecologically intermediate status of L. oregonensis in the evolution of leptarctines. The oldest leptarctine (19–22.5 Ma; Wang et al., Reference Wang, Qiu. and Wang2004), Kinometaxia from Asia, displays a long P4 blade (Wang et al., Reference Wang, Qiu. and Wang2004, fig. 3A). The oldest North American leptarctines, Craterogale and Schultzogale, also display long P4 blades and reduced or altogether missing hypocones on P4 and M1 (Gazin, Reference Gazin1936; Lim and Martin, Reference Lim and Martin2000), which are characteristics associated with carnivorous diets. The oldest leptarctines, L. ancipidens and L. oregonensis, from the Hemingfordian and early Barstovian respectively, also display long P4 blades (Fig. 6.1, 6.2). However, the younger (late Barstovian) L. primus shows a reduced P4 blade (Korth and Baskin, Reference Korth and Baskin2009). Other mid to late Miocene species (e.g., L. mummorum and L. martini) show more robust hypocones and less blade-like P4s than L. oregonensis, indicating increased crushing and possibly more omnivorous diets relative to L. oregonensis (Lim and Miao, Reference Lim and Miao2000, fig. 1.1; Korth and Baskin, Reference Korth and Baskin2009, fig. 4; Fig. 6.3). Even younger species, from the Clarendonian through Hemphillian (e.g., L. kansaensis and L. webbi), essentially lack a functional P4 blade (Lim and Martin, 2001, fig. 1A; Baskin, Reference Baskin2005, fig. 3A) and may have had more herbivorous diets than older Leptarctus species. Thus, L. oregonensis may represent the initial stage of an ecomorphological transition from an ancestrally carnivorous diet to a more derived herbivorous diet in leptarctines. Future investigations of the phylogenetic relationships of leptarctines will be critical in exploring this issue.
In light of the conflicting dietary signals of comparative anatomy, we undertook a quantitative comparison of the proportions of the P4 and M1 of Leptarctus oregonensis with modern carnivores. Our results suggest an animal-dominated omnivorous diet rich in invertebrates similar to that of the similarly sized Mephitis mephitis and Mydaus javanensis or the smaller Spilogale putorius. The morphology of the teeth of L. oregonensis is very similar to that of the teeth of Spilogale. They both share a long P4 blade as well as a large and posteriorly expanded metacone (van Gelder, Reference van Gelder1959; Kinlaw, Reference Kinlaw1995, fig. 2c). This long P4 blade could provide the sharp edges required to tear and shear invertebrates (Popowics, Reference Popowics2003) in addition to shearing vertebrate prey. The M1 of Spilogale, like that of L. oregonensis, is very broad and would offer a large surface area for grinding vegetation (Kinlaw, Reference Kinlaw1995, fig. 2c) or invertebrate cuticles (Popowics, Reference Popowics2003). Although they are very similar in dental morphology, the skull of L. oregonensis is much more robust than the skulls of S. putorius, Me. mephitis, and My. javanensis. The cranial morphology of L. oregonensis does not however prevent it from having an invertebrate-rich diet. Indeed, the proportions of the skull of L. oregonensis are similar to those of Meles meles (European badger), which includes a high proportion of earthworms in its diet in addition to some plant material and small vertebrates (Nowak, Reference Nowak2005; Myslajek et al., Reference Myslajek, Nowak, Rożen and Jędrzejewska2013). Like L. oregonensis, Meles meles displays a broad skull with a short snout, a high sagittal crest, prominent mastoid processes, and robust zygomatic arches (Hidaka et al., Reference Hidaka, Matsumoto, Hiji, Ohsako and Nishinakagawa1998; Yom-Tov et al., Reference Yom-Tov, Yom-Tov and Baagøe2003).
Our interpretation of an invertebrate-rich diet for L. oregonensis may explain the conflicting results of our analyses of its craniodental morphology. Indeed, the study of extant small carnivorans shows that the morphological overlap among invertivorous carnivorans and both carnivorous and omnivorous species can sometimes prevent the assignment of a taxon to one of these three dietary categories (Friscia et al., Reference Friscia, Van Valkenburgh and Biknevicius2006). Our interpretation is consistent with data on body mass and energetic constraints on carnivore diets. Our estimate of maximum prey size for L. oregonensis suggests that it would have been able to consume many of the small mammals known from the Mascall Formation, including the leporid Hypolagus, many rodents (e.g., Protospermophilus and Nototamias), and the shrew Pseudotrimylus (Maguire, Reference Maguire2013). In fact, we estimate that L. oregonensis might have been able to prey upon animals as large as 3.6 kg. Additionally, many mustelids can kill prey much larger than themselves (Meers, Reference Meers2002, appendix). Nevertheless, studies of extant carnivores have shown that carnivorans the size of L. oregonensis tend to consume prey smaller than themselves and include a large portion of invertebrates in their diet (Carbone et al., Reference Carbone, Mace, Roberts and Macdonald1999). Thus, L. oregonensis may have been primarily an invertivore that supplemented its diet with vertebrate prey and possibly some vegetation.
The possible dietary shift through the evolution of leptarctines from carnivory to omnivory suggested by L. oregonensis may be a response to the radiation of neomustelids in North America during the Miocene (Baskin, Reference Baskin1998; Sato et al., Reference Sato, Wolsan, Prevosti, D’Elía, Begg, Begg, Hosoda, Campbell and Suzuki2012). There is some evidence that modern musteloids (including the families Mustelidae, Procyonidae, and Mephitidae; Flynn et al., Reference Flynn, Finarelli, Zehr, Hsu and Nedbal2005) partition and compete for resources. For example, the omnivorous diet of Spilogale is in part a consequence of sympatry with the larger stripped skunk (Mephitis) and carnivorous weasels (Mustela) (Kinlaw, Reference Kinlaw1995). The diversification of carnivorous neomustelids during the Miocene in North America may have led to character displacement in the Leptarctinae and a shift towards more omnivorous diets. A proper test of this hypothesis awaits a broader study of the changes in diet within Leptarctinae in a phylogenetic context.
Acknowledgments
We thank S. Foss for bringing this specimen to our attention, J. Baskin, K. Cashman, R. Dorsey, S. Foss, T. Fremd, S. Hopkins, G. Wilson, and H. Wagner for discussions, M. Smith for preparing UOMNH F-35458, J. Orcutt for discussions and specimen transportation, J. Samuels for help with measurements of UOMNH F-35458, S. Mc Leod and V. Rhue (LACM), P. Holroyd (UCMP), George Corner, Ross Secord, and Bob Hunt (UNSM), and R. O’Leary (AMNH) for access to collections, specimen photos, or locality information. S. Hopkins and G. Wilson provided space, input, and technical support. M. Turner made figure 6. N. Famoso facilitated the final stages of this study. B. Hunda, E. Scott, J. Baskin, K. Prassack, K. Smith, and anonymous reviewers made constructive suggestions that greatly improved the manuscript. JC was funded by the UO Department of Geological Sciences Ewart Baldwin Award and the University of Washington Department of Biology Chair’s Research Assistantship during part of this research.