Introduction
Barnyardgrass [Echinochloa crus-galli (L.) P. Beauv.], an annual herb of the Poaceae family, is one of the world’s most important weeds, ranking third in the worldwide order of economic impact (Bajwa et al. Reference Bajwa, Jabran, Shahid, Ali, Chauhan and Ehsanullah2015; Holm et al.Reference Holm, Doll, Holm, Pancho and Herberger1997; Maun and Barrett Reference Maun and Barrett1986). Echinochloa crus-galli is widespread in tropical and northern and southern temperate zones, where it grows mainly at low and medium altitudes and colonizes crops [maize (Zea mays L.), soybean [Glycine max (L.) Merr.], potatoes (Solanum tuberosum L.)], orchards, and vineyards, but also fallow fields, wetland margins, ruderal areas, and other habitats with exposed soil surfaces. Given the large area of its expansion and the diversity of soil, climatic, and ecological conditions it has to deal with, it is not surprising that E. crus-galli is very plastic. Its morphological, physiological, and biochemical variability is determined by both phenotypic and hereditary factors and is manifest in all developmental stages.
The high plasticity of E. crus-galli results in the formation of populations adapted to local climatic conditions (Barrett Reference Barrett1982; Barrett and Wilson Reference Barrett and Wilson1981). This adaptation of E. crus-galli populations to local conditions deserves attention, because it is likely one of the mechanisms supporting the aggressive spread of this species. The study of adaptation to the local climate is best performed in areas where linearly arranged neighboring populations grow under a climatic gradient of conditions.
A region that has the required properties and is suitable for this research is the mountainous area of the northern Carpathians in western Slovakia (central Europe). The geography of this area creates the conditions for the formation of E. crus-galli populations whose life cycle differs due to climatic adaptation. The northern part of this area consists of mountains divided in the meridian direction by parallel valleys. Each of these valleys hosts a series of E. crus-galli populations. In the direction from lowlands to highlands, the populations grow in increasingly harsh conditions and are isolated from populations in adjacent valleys by mountain ridges.
In central Europe, the length of a one-generation life cycle of E. crus-galli closely matches the length of the growing season. Seedlings emerge late in the spring, following the onset of warm weather (Brod Reference Brod1968; Li Reference Li1962; Martinková and Honěk Reference Martinková and Honěk1993), and seed dispersal occurs at the end of the vegetative season before the onset of cold weather, in August to September (Martinková and Honěk Reference Martinková and Honěk1992). Climatic conditions likely also affect the survival of the emerged seedlings. After seed dispersal, some of the dormant seeds quickly become germinable, because high temperatures on the soil surface cause their afterripening (Dinola and Taylorson Reference Dinola and Taylorson1989; Taylorson and Brown Reference Taylorson and Brown1977). However, seedlings that emerge in the autumn most likely die during the cold winters prevailing in highland conditions, because they are not resistant to frost (cf. Fischer et al. Reference Fischer, Dawson and Appleby1988). In the lowlands, seedlings survive only in years with warm winters. However, most E. crus-galli seeds enter a persistent seedbank state in which they may survive for tens of years and pass through dormancy/nondormancy cycles each year, with minimum germination in August to September and maximum germination in April to May (Honěk et al. Reference Honěk, Martinková and Jarošík1999). Based on these observations, we hypothesized that variation in E. crus-galli phenology and morphology limits spread from warm and dry lowlands to colder and wetter highland areas.
We expected that seeds from lowland populations would be more sensitive to conditions terminating dormancy than seeds from highland populations and that under the same temperature, humidity, and soil conditions, the life cycle would be longer in lowland plants than in highland plants. To determine the influence of local climatic conditions on the life cycle of E. crus-galli populations we examined (1) differences in the termination of seed dormancy in populations distributed along transects following the flows of two rivers and (2) hereditary divergence in the phenology and morphology of lowland and highland E. crus-galli populations distributed along these transects.
Materials and Methods
Study Area
The geography of the study area (47.7°N to 49.3°N, 17.6°E to 20.3°E) is an important factor in the isolation and diversification of local E. crus-galli populations. Echinochloa crus-galli populations along transects following the flows of two rivers were examined (Figure 1). The western Váh River (440-km long) springs at 1,680 m altitude (48.897°N, 20.139°E) and flows to the Danube at 106 m altitude (47.750°N, 18.147°E). It flows 150 km in an E-W direction, then 290 km in a N-S direction. In total, 320 km of the river flows through a mountain valley, and then 120 km of the river runs in the lowlands (170 to 100 m altitude). The eastern Hron River (300 km long) springs at 950 m altitude (48.859°N, 20.216°E) and flows to the Danube at 104 m altitude (47.818°N, 18.741°E). It flows 100 km in an E-W direction, then 200 km a N-S direction. Approximately 230 km of the river flows through a mountain valley, and 70 km of the river runs in the same lowlands as Váh. In mountain areas, the two streams are separated by mountain ridges, the height of which gradually decreases from 2,000 to 900 m altitude and then abruptly terminates. In the lowlands, the flows of the two rivers are approximately 50 km apart and are not separated by terrain elevations. The climate in the lowlands, which is the source of the E. crus-galli populations further covered by the term “lowland,” is different from that of the upper mountain valleys, the source of the populations referred to as “highland.” In the E. crus-galli growing season (May to August) the average temperature in the lowlands is 18.2 ± 0.3 C, and the average temperature in the highlands is 14.9 ± 0.8 C (arithmetic mean ± SD for sites of E. crus-galli populations used in the common garden experiment). The average total precipitation for this period is 230 mm in the lowlands and 350 mm in the highland area (Matějka Reference Matějka2019; Vesecký et al. Reference Vesecký, Petrovič, Briedoň and Kárský1958).
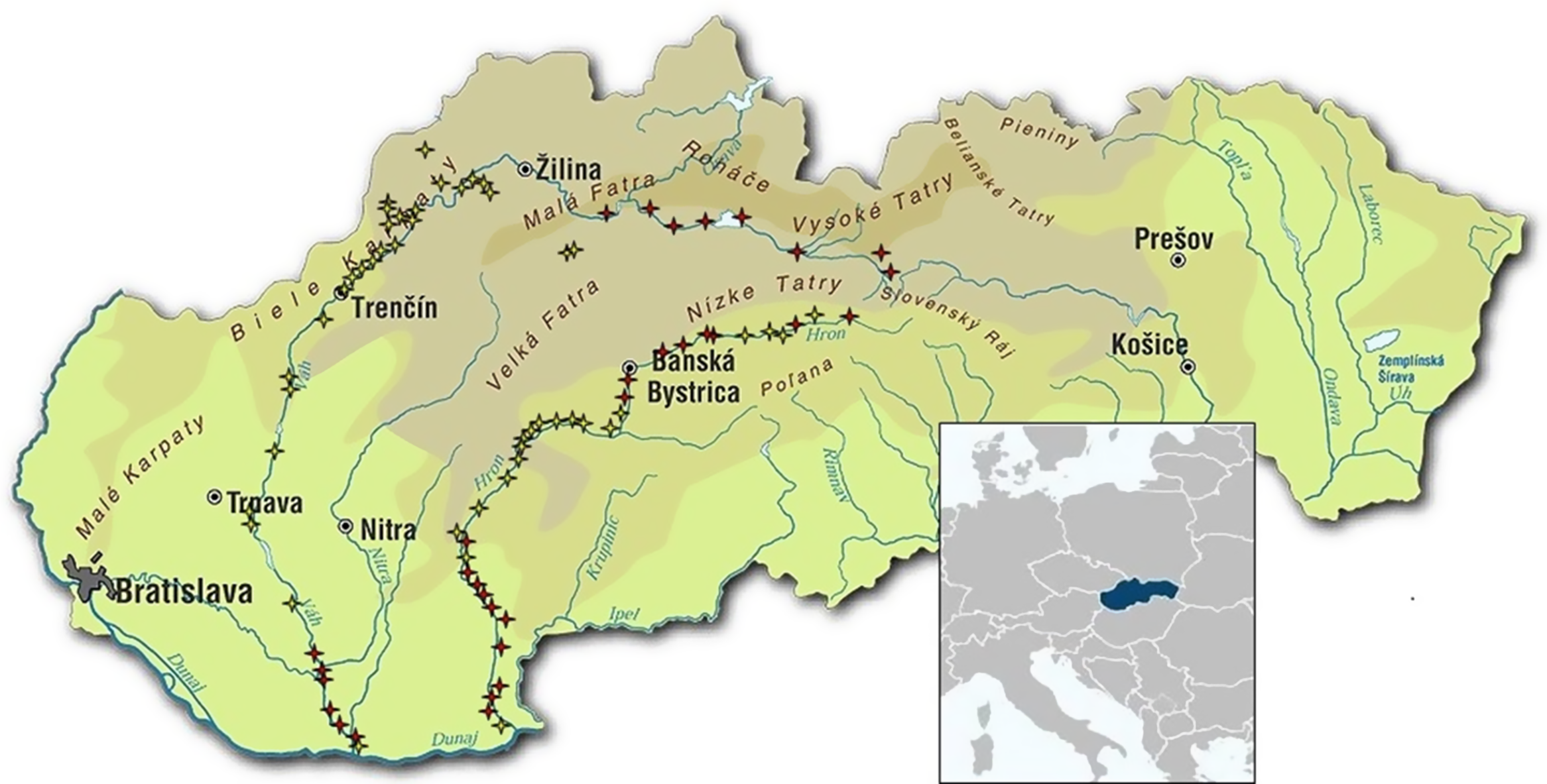
Figure 1. The position of the Echinochloa crus-galli sampling sites (+) along the Váh (left) and the Hron (right) rivers projected onto the schematic map of Slovakia (source: www.eslovensko.cz/mapy). Insert (source: www.globalassistance.cz/turisticke-informace/slovensko): the position of Slovakia (blue) in central Europe. Sites from which the seeds used in common garden experiments originated are marked in red. The two easternmost sites belong to the Hron transect.
Source of Seed Materials
Echinochloa crus-galli seeds were collected along transects following the Váh (August 17 to 29, 2010) and Hron (August 16 to 20, 2011) rivers. The sampling sites (40 in 2010 and 39 in 2011) were approximately 5 km apart (Figure 1). At each site, seeds were harvested during the period of seed dispersal (by a mild shaking of the panicles over a tray to ensure that only ripe seeds were collected), from ˜50 plants growing within an area of ˜100 m2. The seeds of individual plants were mixed, because it was impossible to distinguish individual plants from one another in dense E. crus-galli stands.
Seed Germination Experiment
The seeds collected in wild populations at sites along the Váh and Hron transects were dried at 25 C and 40% relative humidity (RH) until September 15. The seeds were then afterripened by drying at 25 C and 40% RH until February 1 of the year following collection (i.e., for a total of ˜5 mo after harvest). The duration of afterripening was longer than in nature, because the seeds were exposed to constant temperatures that were lower than would prevail on the insolated ground surface in the open.
Germination experiments were conducted with three replicates of 50 seeds of each seed sample originating from a particular sampling site. Each group of 50 seeds was placed into a 10-cm-diameter petri dish on filter paper (Whatman no. 1, Sigma-Aldrich, St. Louis, MO) moistened by 6 ml of tap water and maintained under germination conditions at a constant temperature of 25 C and a 16-h light:8-h dark photoperiod in a climatized box. Moisture was controlled, and if necessary, more water was added. The germinating seeds (radicle ˜1-mm long) were counted and removed daily until no further germination had occurred for 10 d.
Common Garden Experiment
Seeds from sites located along the Vah River transect (6 lowland and 6 highland sites) and the Hron River transect (10 lowland and 10 highland sites) were used for the common garden experiment (Table 1). To attain maximum germinability, the seeds were stratified under natural conditions (Honěk et al. Reference Honěk, Martinková and Jarošík1999). They were packed into nylon fabric (0.24-mm mesh size, 56% open area) bags and buried in the soil in a field at a depth of approximately 20 cm. The stratification was performed at Prague-Ruzyně from September 15 of the year of sampling until April 30 of the next year, when the common garden experiment was conducted. This treatment assured ˜95% germinability (Honěk et al. Reference Honěk, Martinková and Jarošík1999), which was tested under 25 C and an 18-h light:6-h dark photoperiod before seeds were sown.
Table 1. Details of the common garden experiments at the Crop Research Institute at Prague-Ruzyně.

a Coordinates of the sites of origin of the Echinochloa crus-galli populations. m asl, meters above sea level.
The common garden experiment consisted of growing plants of lowland and highland populations at the same place. The plants were grown in the experimental common garden at the Crop Research Institute at Prague-Ruzyně. This experimental plot is located at 50.086°N, 14.300°E, at an altitude of 340 m above sea level. We tracked the time and morphological differences during the course of the development of the plants from the lowland and highland populations. The plants were grown in pots arranged on a horizontal tabletop at a height of 70 cm above the bare ground floor, in the open. The tables were overlaid with 6-cm-deep reflective tin pans, which provided protection against overheating by direct sunlight. The pans were filled with water. The water was topped up so that its level in the pans never fell below 3 cm, and the pots were also watered daily by topdressing and were thus maintained under constant full water saturation. The pots were placed on top of the pans 50 cm apart. Their position was randomly changed at weekly intervals to prevent stable competition interactions between neighboring plants.
In both 2011 and 2012, the seeds were sown on May 3, just before the native E. crus-galli populations usually emerge in the wild (Honěk and Martinková Reference Honěk and Martinková1991). Ten seeds were sown in each pot. Seedling emergence was recorded when the first leaves were 0.5-cm long. At the 2-leaf stage, the seedlings were thinned, leaving only the largest seedling (1 plant per pot). Before seed dispersal, the panicles were wrapped with small nylon bags to prevent loss of the dispersed seeds. The plant shoots were harvested in late August, at a time following the completed seed dispersal of the fifth tiller produced in the course of the season (Table 1). The shoots were cut 5 mm above ground level, dried at 60 C until a constant mass was reached, and weighed to the nearest 0.1 g.
Relevant meteorological characteristics, mean air temperature at 2 m above the ground surface (T) and the sum of photosynthetically active radiation (PAR), were measured ˜100 m away from the common garden experiment. The T and PAR did not differ in both years, except for the July temperatures, which were significantly higher in 2012 than in 2011 (Table 2). Meteorological data are available at https://www.vurv.cz/meteo/default.asp.
Table 2. Meteorological data relevant to Echinochloa crus-galli growth for the common garden experiments at the Crop Research Institute at Prague-Ruzyně in 2011 and 2012: average temperature (T; mean daily temperature at 2 m above the ground surface) and the daily sum of photoperiodically active radiation (PAR).a

a Data labeled by different letters indicate a significant difference.
For the experimental plants, we recorded traits that characterize adaptation to local conditions (Neuffer et al. Reference Neuffer, Bernhardt, Hurka and Kropf2011), such as development time and plant size. Date of heading of particular tillers (the time when the panicle fully emerged from the sheath of the upper leaf) and date of seed dispersal (the time when seeds of particular shoots began to drop) were recorded at 2-d intervals. Furthermore, we recorded the mass, height, and seed mass per panicle of the particular shoots and the whole-plant mass (total of all of the shoots).
Statistical Analysis
Test for Geographic Differences in Termination of Seed Dormancy
The rate of termination of seed dormancy (proportion of germinated seeds) showed skewed distribution and a nonlinear relationship with the explanatory variable. Therefore, differences in this variable were compared by generalized linear mixed models (GLMMs) with gamma error structure from the mgcv package in R (Wood Reference Wood2017). A GLMM was used to specify localities as random effects.
Tests for Hereditary Divergence between Lowland and Highland Populations
Differences in time of heading, seed mass, and tiller height were compared by GLMM with gamma error structure. Measurements of time to seed dispersal and tiller mass allocation to seeds were standardized by subtracting the mean for the particular tiller and dividing by the SD of that particular tiller. Differences in these variables between lowland and highland localities were compared using generalized least squares (GLS) from the nlme package in R (Pinheiro et al. Reference Pinheiro, Bates, DebRoy and Sarkar2019; R Core Team 2019). GLS was used because these response variables showed normal distribution and/or a linear relationship with the explanatory variable and to take into account nested structure in the data (localities nested within land and both nested within river) by including compound symmetry correlation structure in residuals (Pekár and Brabec Reference Pekár and Brabec2016).
Results and Discussion
Variation in Seed Germinability
The germinability of seeds harvested at particular sites along both the Váh and Hron river transects varied with altitude. In afterripened seeds, the percentage of germination was low (on average <2%) with the exception of a few localities where germinability of the seeds reached up to 70% (Figure 2). Germination declined significantly with increasing altitude (GLMM, F 1 = 6.9, P = 0.009), but the relationship was not significantly different between rivers (GLMM, F 1 < 0.1, P = 0.99). All sites where the germination rate exceeded 1.0% were in the lowlands, below 200 m altitude. The geographic variability in the percentage of germinable seeds in different populations was not caused by differences in seed viability. The experiment with afterripening of seeds from sites of the Hron transect was continued for 22 mo (ZM and AH, unpublished data), and after this period, the frequency of germinable seeds increased in each of the lowland and mountain populations above 85%, regardless of the original percentage of germinable seeds.

Figure 2. Relationship between altitude and the proportion of afterripened Echinochloa crus-galli seeds that germinated at 25 C. The seeds originated from populations collected at sites along the Váh and Hron transects scaled according to their altitude. The line indicates a logit model of seed germination.
In central Europe, seed dispersal takes place beginning in August. Freshly ripened seeds are dormant, but afterripening may cause rapid termination of dormancy. At ˜50 C, the proportion of germinating seeds can increase in a few days to >90% (Taylorson and Brown Reference Taylorson and Brown1977). Because the temperature of the insolated soil surface often exceeds 40 C (Bonan Reference Bonan2002; Martinková and Honěk Reference Martinková and Honěk2010), the dormancy of freshly ripened E. crus-galli seeds may be terminated in late summer and early autumn of the same year in which the seeds have ripened. These seeds can germinate, and seedlings will emerge before the onset of winter. The proportion of seeds germinated in autumn in natural populations likely depends on weather conditions. This important event in the life cycle of E. crus-galli has not yet been studied. The rapid termination of dormancy by afterripening puts seedlings and young plants at risk of freezing in the winter (Fischer et al. Reference Fischer, Dawson and Appleby1988). This danger is less in lowland areas (90 to 100 d in which the minimum temperature is ≤0 C, <30 d in which the maximum temperature is ≤0 C) than in highland areas (140 to 160 d in which the minimum temperature is ≤0 C, >50 d in which the maximum temperature is ≤0 C) (Vesecký et al. Reference Vesecký, Petrovič, Briedoň and Kárský1958). Temperature conditions for E. crus-galli survival in winter are not satisfactory in any of the studied areas, yet they are better in the lowlands than in highland areas. We assumed that the hard winters of the highland areas completely killed all seedlings that emerged in the autumn. This selection eliminated seeds capable of terminating dormancy after a short period of afterripening at a high temperature, so the genes that favor afterripening are selected against in highland areas. Afterripening ability is preserved only in lowland populations from below 200 m altitude. Populations originating from sites located at higher altitudes are thus fully protected against untimely emergence.
Local variations in the percentage of germinable afterripened seeds have been established in several studies (Barrett and Wilson Reference Barrett and Wilson1983). This variation usually has no consistent geographic pattern but may persist over consecutive years (Honěk and Martinková Reference Honěk and Martinková1996), thus suggesting that this trait is fixed in local populations. The biological reasons for local variability in requirements for dormancy termination have not yet been established. This study indicated that adverse winter conditions likely act selectively against the susceptibility of seeds to afterripening at high temperatures and may reinforce seed dormancy.
Geographic Variation in the Timing of Plant Development
Lowland and highland populations differed in their rate of development. Time to heading of plants from highland localities was significantly shorter than time to heading of plants from lowland localities in populations of both the Vah (GLS, F 1,818 = 28.0, P < 0.0001; Figure 3A) and Hron transects (GLS, F 1,793 = 62.6, P < 0.0001; Figure 3B). The difference between lowland and highland populations significantly increased with the increasing order of the tillers. Significant differences were also established in the time to seed dispersal (GLS, F 1,1,585 = 39.7, P < 0.0001; Figure 4), which occurred earlier in highland than in lowland populations. Under the same conditions, the development of plants from highland populations was significantly faster than the development of plants from lowland populations, likely due to the difference in thermal requirements for the completion of development. This difference can be expected. To finish development before the end of the growing season, plants from colder highland conditions likely have lower thermal requirements than plants from warmer lowland conditions. Under the same temperature conditions (in a common garden experiment), the requirements for the development of plants from highland populations are met earlier than the requirements for plants from lowland populations.

Figure 3. Relationship between tiller order and time (Julian day) of heading for lowland and highland Echinochloa crus-galli populations sown in a common garden experiment. (A) Populations from the transect along the Váh River (common garden experiment 2011). (B) Populations from the transect along the Hron River (common garden experiment 2012). Estimated linear models are shown.
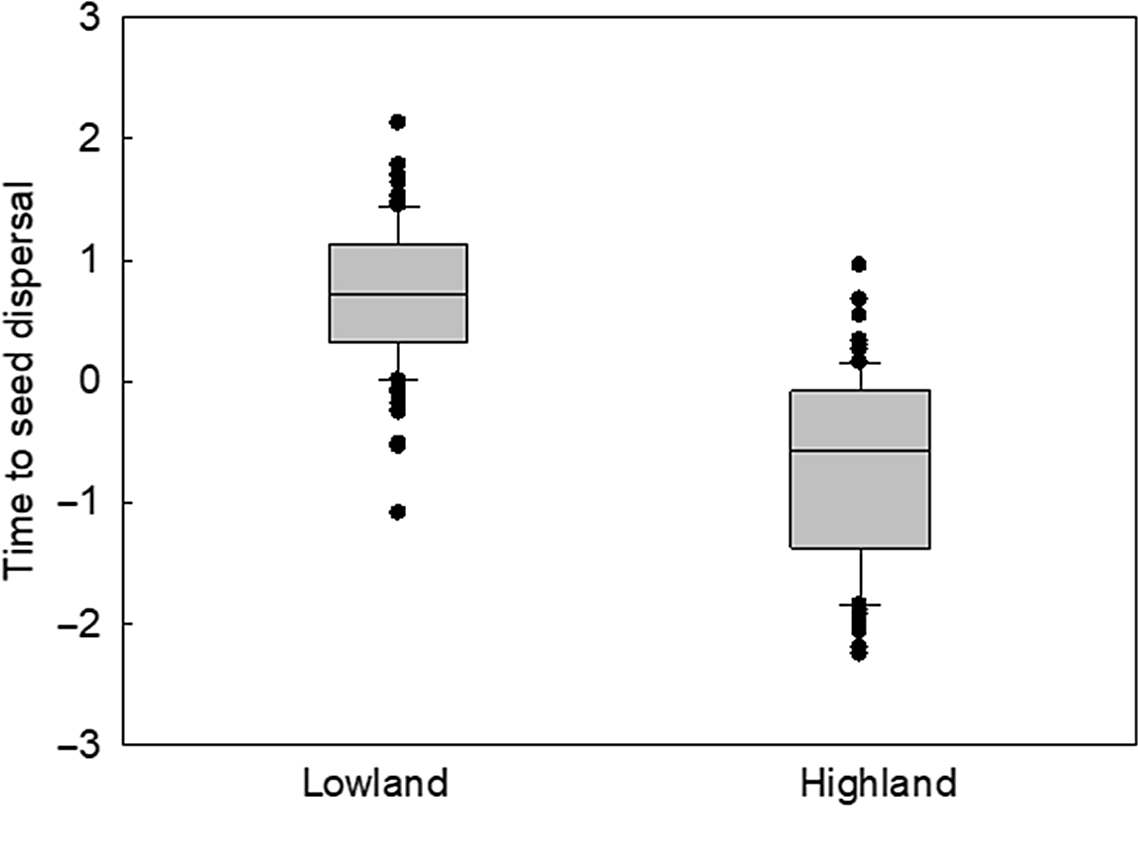
Figure 4. Comparison of z-scores of the time to seed dispersal between plants of the lowland and highland populations. The z-scores were obtained by standardization for the tiller order in Echinochloa crus-galli populations sown in a common garden experiment. Combined data of 2011 (Váh) and 2012 (Hron). The graph plots the median and the 10th, 25th, 75th, and 90th percentiles as vertical boxes with error bars and outliers (dots).
A similar differentiation in development time (likely caused by different thermal requirements for the completion of development) occurred among the E. crus-galli populations from distant latitudes (ZM, unpublished data). A pilot common garden experiment (conducted in 2016, in which the experimental site, time of sowing, and method of growing plants were the same as in the study reported here, but the seed materials were purchased from Herbiseed, Mire Lane, RG10 0NJ Twyford, UK) revealed great differences in the phenology of plants from temperate and subtropical zones. The time to heading of the first tiller was 55 ± 1.4 d (n = 12) for plants originating from the lowlands of Slovakia (˜49°N) and 62 ± 1.6 d (n = 12) for plants originating from the northern United States (˜47°N). In the population originating from the southern United States (˜34°N), only one of 12 experimental plants headed after 71 d, and in the population from Japan (˜35°N), only one of 12 plants headed after 98 d. Time to heading thus increased with decreasing latitude of the place of origin of the population. For subtropical populations from the southern United States and Japan, time to heading was so long that the entire vegetative season in central Europe was not long enough to bring all plants to heading. The time required for heading and probably also the temperature requirements for development up to this stage correlated with the latitude and climatic conditions of the place of formation of the experimental populations. Differences in the duration of plant development are obviously essential for adaptation to local conditions and should be addressed in further studies.
Geographic Variation in Plant Morphology
Variation in the characteristics of the morphology of lowland and highland plants is important. The height of the tillers decreased significantly with the order of their emergence (the sequence of the heading of the tiller) and was greater in plants from lowland populations than in those from highland populations (Figure 5). The difference was greater in plants from the Váh transect, where interaction between tiller order and plant origin (lowland vs. highland) was significant (GLMM, F 1 = 5.7, P = 0.017; Figure 5A), than in plants from the Hron transect, where there was a significant additive effect between tiller order and plant origin (lowland vs. highland) (GLMM, F 1 = 10.0, P = 0.001; Figure 5B). The smaller differences in Hron populations could be affected by the fact that, in our experiment, the plants of the Hron populations were generally smaller than the plants of the Váh populations. A similar significant difference was found for the whole-plant mass, which was greater in the lowland populations than in the highland populations (GLS, F1,1,621 = 13.1, P = 0.0003; Figure 6).

Figure 5. Relationship between tiller order and height (cm) for lowland and highland Echinochloa crus-galli populations sown in a common garden experiment. (A) Populations from the transect along the Váh River (common garden experiment 2011). (B) Populations from the transect along the Hron River (common garden experiment 2012). Estimated inverse models are shown.

Figure 6. Comparison of z-scores of whole-plant mass between Echinochloa crus-galli plants of lowland and highland populations. Combined data of Váh (common garden experiment 2011) and Hron (common garden experiment 2012) populations. The graph plots the median and the 10th, 25th, 75th, and 90th percentiles as vertical boxes with error bars and outliers (dots).
There was a significant decline in the total mass of seeds produced per shoot and a significant difference between the lowland and highland populations along the Váh (GLMM, F 1 = 4.4, P = 0.035; Figure 7A). Similarly, there was a significant decline in the total mass of seeds produced per shoot and a significant difference between the lowland and highland populations along the Hron (GLMM, F 1 = 5.3, P = 0.021; Figure 7B). Although the differences in seed mass per tiller between lowland and highland populations were significant, they were small compared with the differences in the mass of the vegetative parts of the shoots, which were also larger in plants from lowland than highland populations (not shown, cf. Figure 5). As a result, the ratio of seed mass produced per tiller to total tiller mass (i.e., the combined mass of the vegetative part of a tiller and the seeds) was significantly greater in plants of highland populations than in plants of lowland populations (GLS, F 1,1,603 = 69.6, P < 0.0001; Figure 8). The plants of highland populations thus allocated more aboveground biomass to reproduction than the plants of lowland populations.

Figure 7. Relationship between tiller order and total seed mass (g tiller−1) in lowland and highland Echinochloa crus-galli populations sown in a common garden experiment. (A) Populations from the transect along the Váh River (common garden experiment 2011). (B) Populations from the transect along the Hron River (common garden experiment 2012). Estimated inverse models are shown.
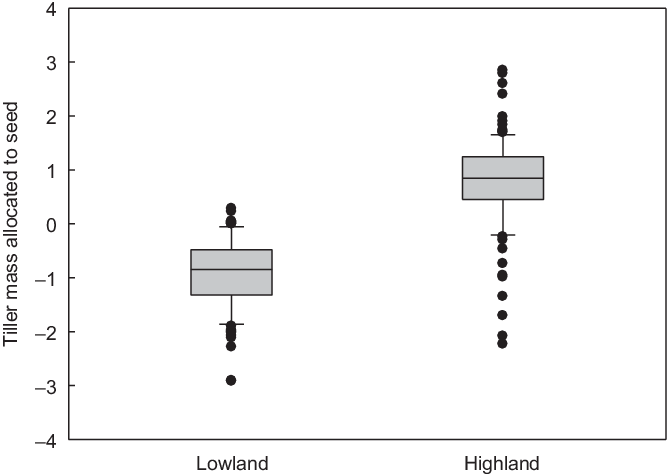
Figure 8. The differences between Echinochloa crus-galli plants of lowland and highland populations in percentage of whole-shoot mass allocated to seed in the first to seventh tillers (z-scores). Populations from the Hron sown in a common garden experiment in 2012. The graph plots the median and the 10th, 25th, 75th, and 90th percentiles as vertical boxes with error bars and outliers (dots).
The experiment revealed the relationship between the length of development and plant size. Plants from highland populations developed faster than plants from lowland populations at the expense of height. The difference was due to a reduction in the vegetative parts of the shoots, while the biomass allocated to seed production was little affected.
Factors of Echinochloa crus-galli Adaptability
As documented in a number of studies (Barrett Reference Barrett1982; Barrett and Wilson Reference Barrett and Wilson1981; Martinková and Honěk Reference Martinková and Honěk2011; Norris Reference Norris1996; Norris et al. Reference Norris, Elmore, Rejmánek and Akey2001), E. crus-galli typically exhibits a high phenotypic plasticity. The plants are sensitive to variations in abiotic (soil quality, nutrients, temperature, and light) and biotic (population density) conditions that affect the morphological and physiological features of the plants, such as the total plant mass and its allocation to the roots and shoots, foliage size, number of shoots, number of seeds in the panicle, and seed size. Earlier studies have also shown interpopulation genetic variability at the molecular and morphological levels (Altop and Menan 2011; Barrett Reference Barrett1982; Kaya et al. Reference Kaya, Demirci and Tanyolac2014). This plasticity enables E. crus-galli to survive and reproduce in heterogeneous and unpredictable environments (Clements et al. Reference Clements, DiTommaso, Jordan, Booth, Cardina, Doohan, Mohler, Murphy and Swanton2004; Maun and Barrett Reference Maun and Barrett1986; Moran and Alexander Reference Moran and Alexander2014; Sultan Reference Sultan1987). Plasticity in different environments favors survival and reproduction. This plasticity could initiate the formation of local populations observed in this study, which differed in their inherited characteristics of development length and plant size.
In the territory of Slovakia, the formation of these inherited differences can be dated relatively accurately to the period of 30 to 40 yr before the execution of our study. The differences between lowland and highland populations likely have arisen during adjustment to the highland climate, to which they had to adapt as they expanded along watercourses of the Váh and Hron, from mild lowland conditions to harsh highland conditions, from the area of their original occurrence to the newly occupied areas on the upper reaches of the rivers. In central Europe, E. crus-galli is a naturalized archeophyte (Pyšek et al. Reference Pyšek, Sádlo and Mandák2002). Regardless of its long presence, the massive spread of E. crus-galli into the mountain valleys of Slovakia began as late as the 1970s. Up to this time, E. crus-galli colonized the lowlands in the south of this territory, on the lower reaches of both the Váh and Hron rivers (Hejný Reference Hejný1957, Reference Hejný1960). Mapping of the occurrence of harmful weed species (Anonymous 1974, 1975) showed that E. crus-galli was absent in the montane valleys. The reasons for its spread are a matter of speculation. We believe that the spread was mainly due to alterations of cultivated crops (growing maize in submontane areas) and a change in cultivation systems leading to the incorporation of large quantities of E. crus-galli seeds into the soil.
The effect of expansion on the phenotypic properties of E. crus-galli populations is easily observable, precisely because E. crus-galli spread along linear transects isolated from surrounding populations by mountain ridges. This isolation allowed only gradual adaptation to the conditions of changing altitude. The validity of this hypothesis about the emergence of clinal variability in the phenology and morphology of E. crus-galli is supported by the fact that similar changes in E. crus-galli phenology occurred in parallel along collateral river transects of the Váh and Hron. The differences in the rate of development and in the size of the plants persisted from the parent generation to the first filial generation. The contribution of genetic and phenotypic causes of this inheritance is a matter for further research (Kingsolver and Pfennig Reference Kingsolver and Pfennig2007; Moran and Alexander Reference Moran and Alexander2014).
The way in which climate affects the population biology and ecophysiology of E. crus-galli has so far been insufficiently studied. The specific geographic conditions of our study were suitable for determining the relationship between E. crus-galli traits and the local climate on a small scale: E. crus-galli populations were arranged along two parallel transects with a similar gradient of climatic conditions, which were isolated from each other. The variability of phenological and morphological characteristics, parallel in both transects, provided further proof of the existence of a relationship between the local climate, the length of development, and the size of E. crus-galli plants. Even small climatic differences have contributed to the creation of local populations with heritable characters adapted to local conditions. The extent to which the inheritance of E. crus-galli traits arises from genetic or epigenetic factors needs to be further investigated. However, it can be assumed that this heritable adaptation to local conditions is part of a globally successful strategy for the spread of E. crus-galli.
We make three observations: (1) the lowland and highland populations of E. crus-galli differ in several phenological and morphological features, (2) this interpopulation variability is correlated with the geographic and climatic conditions of the site, and (3) the observed differences in phenology and plant size clearly contribute to coping with local climate conditions. The selection of heritable differences enabling adaptation to local climatic conditions likely contributed to expanding the geographic area of E. crus-galli, and similar population processes can help spread other weed species with global distribution.
Acknowledgments
We thank Hana Smutná, Helena Uhlířová, Jana Kohoutová, and Jan Štrobach for excellent technical assistance. This work was supported by institutional support from the Ministry of Agriculture of the Czech Republic (RO0418). The authors also thank American Journal Experts (certificate–the verification code: 8105-23AC-C8DF-0C6E-9FD1) for their professional English language editing services. No conflicts of interest have been declared.