INTRODUCTION
In their native distributional ranges, species live in balance with their environment and populations are controlled by biotic interactions such as predation, parasitism and disease (Otero et al., Reference Otero, Cebrian, Francour, Galil and Savini2013). However, whenever species are introduced into a new environment, they might become established and invasive if certain factors are suitable, namely the absence of predators, wide environmental tolerance and high reproductive output (DeRivera et al., Reference DeRivera, Ruiz, Hines and Jivoff2005; Angert et al., Reference Angert, Crozier, Rissler, Gilman, Tewksbury and Chunco2011). The introduction and spread of some non-indigenous species in aquatic ecosystems constitute a threat to the invaded communities (Kolar & Lodge, Reference Kolar and Lodge2001), negatively affecting the native species and leading, in extreme situations, to their replacement (Ruiz et al., Reference Ruiz, Carlton, Grosholz and Hines1997; Pranovi et al., Reference Pranovi, Franceschini, Casale, Zucchetta, Torricelli and Giovanardi2006). Moreover, introduced species might have distinct effects in different locations and consequently their impacts are difficult to predict (Machado, Reference Machado2015b). For this reason, it is crucial to understand the interactions between invasive and native species and to envisage how non-indigenous species can affect autochthonous species and community dynamics, which assumes an urgent character whenever dealing with commercially exploited and economically relevant species (Velez et al., Reference Velez, Figueira, Soares and Freitas2015a).
The Manila clam or Japanese carpet shell, Ruditapes philippinarum (Adams & Reeve, 1850), is native of the subtropical to low boreal western Pacific (Humphreys et al., Reference Humphreys, Richard, Caldow, McGrorty, West and Jensen2007). Native populations are distributed in the Philippines, South and East China Seas, Yellow Sea, Sea of Japan, Sea of Okhotsk and around the Southern Kuril Islands (Goulletquer, Reference Goulletquer1997). Bivalves are among the most invasive taxonomic groups (Sousa et al., Reference Sousa, Gutiérrez and Aldridge2009) and R. philippinarum has become permanently established in several parts of the world (Goulletquer & Héral, Reference Goulletquer and Héral1997) due to its great adaptability and resistance to a varied range of environmental parameters. Nowadays, R. philippinarum is broadly distributed along the Pacific coast of America, the Atlantic coast of Europe, and also occurs in the Adriatic and Aegean Seas (Jensen et al., Reference Jensen, Humphreys, Caldow, Grisley and Dyrynda2004, Reference Jensen, Humphreys, Caldow, Cesar, Humphreys and May2005).
The Manila clam was introduced for culture purposes in the Atlantic and Mediterranean in the early 1970s, firstly in France, afterwards in England, Spain and Italy, and more recently in Portugal (Jensen et al., Reference Jensen, Humphreys, Caldow, Grisley and Dyrynda2004, Reference Jensen, Humphreys, Caldow, Cesar, Humphreys and May2005; Moschino et al., Reference Moschino, Delaney and Da Ros2012; Goulletquer, Reference Goulletquer2015). The culture of R. philippinarum was co-responsible for the introduction of several non-native algae and invertebrates, fouling the shell or parasitizing bivalve tissues (Savini et al., Reference Savini, Occhipinti-Ambrogi, Marchini, Tricarico, Gherardi, Olenin and Gollasch2010). The Manila clam has been reported in several estuarine systems in Portugal (Gaspar, Reference Gaspar2010; Chainho, Reference Chainho2014; Chainho et al., Reference Chainho, Fernandes, Amorim, Avila, Canning-Clode, Castro, Costa, Costa, Cruz, Gollasch, Grazziotin-Soares, Melo, Micael, Parente, Semedo, Silva, Sobral, Sousa, Torres, Veloso and Costa2015; Velez et al., Reference Velez, Figueira, Soares and Freitas2015a, Reference Velez, Leandro, Figueira, Soares and Freitasb), being among the most abundant bivalves in the Ria de Aveiro and in the Sado Estuary and already reaching the status of dominant bivalve in the Tagus Estuary (Chainho, Reference Chainho2014; Chainho et al., Reference Chainho, Fernandes, Amorim, Avila, Canning-Clode, Castro, Costa, Costa, Cruz, Gollasch, Grazziotin-Soares, Melo, Micael, Parente, Semedo, Silva, Sobral, Sousa, Torres, Veloso and Costa2015; Velez et al., Reference Velez, Figueira, Soares and Freitas2015a; Carvalho, Reference Carvalho2017). This species is intensively exploited in the Tagus Estuary due to its wide distribution, high abundance and considerable commercial value. Although in 2014–2015 there were only 182 harvesters licensed for this activity, local censuses estimated more than 1700 harvesters targeting R. philippinarum using diverse fishing gears, with estimated average annual catches (unreported landings) around 10,000 tons year−1 (Ramajal et al., Reference Ramajal, Picard, Costa, Carvalho, Gaspar, Chainho, Cancela da Fonseca, Garcia, Pereira and Rodrigues2016).
Being only recently naturalized in European waters, it is still difficult to ascertain the long-term consequences that such colonization might have on invaded ecosystems (Goulletquer, Reference Goulletquer1997; Goulletquer & Héral, Reference Goulletquer and Héral1997). For instance, in the Venice Lagoon (Italy) R. philippinarum has apparently replaced the congeneric Ruditapes decussatus (Linnaeus 1758) (Occhipinti-Ambrogi, Reference Occhipinti-Ambrogi2000), whereas in Poole Harbour (England) the colonization by this exotic bivalve coincided with a decline in the abundance of Scrobicularia plana and Macoma balthica (Caldow et al., Reference Caldow, McGrorty, West, leV. dit Durell, Stillman, Anderson, Humphreys and May2005). However, the decline of these species might also been caused by local marine pollution (Langston et al., Reference Langston, Chesman, Burt, Hawkins, Readman and Worsfold2003), which possibly made the naturalization of the Manila clam easy. In contrast, the coexistence pattern of both clams in the Bay of Santander (northern Spain) indicates that the Manila clam has not yet supplanted the native R. decussatus by occupying its ecological niche entirely, as it is still the dominating species in more oceanic and freshwater-influenced areas (Juanes et al., Reference Juanes, Bidegain, Echavarri-Erasun, Puente, García, García, Bárcena, Álvarez and García-Castillo2012).
Despite the morphological similarity with the European native R. decussatus, R. philippinarum grows faster and exhibits a notable reproductive capability, with early sexual maturation, high fecundity and extended spawning period (Breber, Reference Breber1985; Sorokin & Giovanardi, Reference Sorokin and Giovanardi1995; Paesanti & Pellizzato, Reference Paesanti and Pellizzato2000; Pellizzato & Da Ros, Reference Pellizzato and Da Ros2005). Previous histological studies showed that reproductive cycle, duration of gametogenesis and spawning season of R. philippinarum and R. decussatus are affected by both geographic location and metabolic activities (Rodríguez-Moscoso et al., Reference Rodríguez-Moscoso, Pazo, García and Fernández-Cortés1992; Laruelle et al., Reference Laruelle, Guillou and Paulet1994; Serdar & Lök, Reference Serdar and Lök2009). However, the reproductive activity of the non-indigenous species appeared more remarkable in terms of number of spawning events and speed of maturation, with R. philippinarum from Brittany (France) exhibiting a longer breeding period and higher number of spawning events than the sympatric R. decussatus (Laruelle et al., Reference Laruelle, Guillou and Paulet1994).
Previous studies revealed that R. philippinarum displays an invasive behaviour in the Tagus Estuary, spreading widely despite being intensively exploited by dredge harvesting (Gaspar, Reference Gaspar2010; Machado, Reference Machado2015b), and growing faster than the native R. decussatus (Moura et al., Reference Moura, Garaulet, Vasconcelos, Chainho, Costa and Gaspar2017), but until now its reproductive biology in this important estuarine system has not been studied. In this context, the present study described the reproductive cycle and estimated the size at first sexual maturity of the Manila clam, aiming ultimately to compare the reproductive strategies between the invasive R. philippinarum and the native R. decussatus, in order to infer potential harmful effects over populations of autochthonous bivalves.
MATERIALS AND METHODS
Study area and field sampling
The study area (Figure 1) is located in the Tagus Estuary (38°44′N 09°08′W), a large estuarine system (≈320 km2) in the central western Portuguese coast (Cotter et al., Reference Cotter, Pereira, Costa and Costa2013). Surrounded by the Lisbon metropolitan area, this estuary has been affected by diverse anthropogenic pressures, namely urban expansion, industrial development, agriculture, harbour infrastructures and fishing activities, with subsequent problems of aquatic pollution (Caçador et al., Reference Caçador, Vale and Catarino1996; França et al., Reference França, Vinagre, Caçador and Cabral2005). The temperature in this estuarine body of water fluctuates considerably throughout the year, ranging from 8°C in winter to 26°C during summer (Cabral et al., Reference Cabral, Costa and Salgado2001).

Fig. 1. Map of the Tagus Estuary (central western Portugal) showing the sampling sites of the Manila clam (Ruditapes philippinarum).
Bivalve fishing surveys targeting the Manila clam were performed monthly between September 2013 and December 2015. Data on the seawater temperature during the study period were kindly provided by the Marine, Environment & Technology Center (MARETEC). An additional sample comprising several individuals with a broad size range was collected in May 2016 (spawning peak of R. philippinarum in the Tagus Estuary previously established through the histological analysis made in the current study), in order to estimate the size at first sexual maturity. Sampling was performed on board a commercial fishing boat and samples were collected by professional harvesters using bivalve dredges in several sampling stations distributed within a considerably large area (~10 km2) in the zone with highest abundances of R. philippinarum in the Tagus Estuary (Figure 1). Bivalve dredges were towed for ~30 s at a constant speed of 2 knots in each sampling station. These dredges consist of a metallic frame, a toothed lower bar and a rectangular metallic grid box (spacing between grid bars of 15 mm) opening posteriorly (for further details on the dredge design and technical specifications see Gaspar et al., Reference Gaspar, Chainho and Costa2014).
Reproductive cycle
Twenty individuals of R. philippinarum were analysed monthly throughout the study period. Specimens were measured for shell length (SL) using a digital calliper (precision of 0.01 mm) and only those individuals above the minimum landing size (MLS = 40 mm SL) legally stipulated for the catches of Manila clam were used in further analyses to avoid including sexually immature individuals in the samples.
In the laboratory, the visceral mass was dissected and separated from the gills and siphons, and then the gonads were fixed in Davidson solution for 48 h and subsequently preserved and stored in 70% ethanol. Afterwards, gonadal tissues were dehydrated with serial dilutions in ethanol, infiltrated and embedded in paraffin wax. Gonads were cut into thin sections (~7 µm) using an automatic microtome, stained with haematoxylin and eosin (H&E), mounted between a glass slide and cover slip with synthetic resin and examined under a light microscope using magnifications of 10× and 20×.
Gonad maturity stages in both sexes were identified and classified using a microscopic maturation scale comprising six stages of gonadal development (briefly described and illustrated in Figure 2), following Gaspar & Monteiro (Reference Gaspar and Monteiro1998). Whenever multiple developmental stages were observed simultaneously within a single gonad, the staging criterion of classifying the individual with the prevailing maturity stage (i.e. the condition observed in most gonadal tissue) was adopted.

Fig. 2. Microscopic maturation scale employed to classify the gonad maturity stages of the Manila clam (Ruditapes philippinarum), including illustrative photomicrographs and brief descriptions of the main features of the gonads at each maturity stage.
In addition, the mean gonadal index (GI) in both sexes was calculated through the following equation (Seed, Reference Seed and Bayne1976):

For each stage of gonadal development, a numerical stage ranking was assigned as follows: inactive = 0; early active = 3; late active = 4; ripe = 5; partially spawned = 2; spent = 1. Accordingly, the GI ranged from 0 (all individuals in the monthly sample in the resting phase) to 5 (all individuals in the monthly sample in the ripe stage).
A quantitative analysis of female gonadal tissues was also performed through the measurement of oocytes diameter. Photomicrographs of the histological preparations were taken with a digital camera and then oocytes were measured using the image processing and analysis software ImageJ (version 1.50b). A total of 30 oocytes of each female were measured for total diameter, independently of the stage of gonadal development. In order to minimize the subjectivity inherent to this method and to avoid biased-selection of the measured oocytes, a grid automatically generated by the imaging software was superimposed in the photomicrographs of the histological preparations and only those 30 oocytes intersected by the grid were measured for total diameter.
Size at first sexual maturity
The size at first sexual maturity (SL50), i.e. the shell length at which 50% of the population is sexually mature, was estimated using an additional sample of 88 R. philippinarum (size range: 23.9–38.6 mm SL) collected in the Tagus Estuary in May 2016, matching the maturation peak recognized through the histological analyses made in previous years and immediately before the predicted spawning season. The gonadal maturation was monitored using the histological methods described above and the individuals were considered mature when the gonad was assigned to stages III (ripe) or IV (partially spawned).
After plotting the cumulative frequency of individuals with mature gonads (stages III or IV) as a function of shell length (grouped into size classes of 1 mm SL), the size at first sexual maturity (SL50) was estimated by fitting a logistic regression to the data through the following equation (King, Reference King1995):

where P, proportion of mature individuals in the population; r, constant; SL, shell length (mm); SL50, shell length (mm) at which 50% of the individuals are mature.
Statistical analysis
Analysis of variance (ANOVA) was employed to assess the variation in mean oocyte diameter among gonad maturity stages and throughout the study period. Whenever ANOVA assumptions (normality of data and homogeneity of variances) were not fulfilled, the non-parametric Kruskal–Wallis test (ANOVA on ranks) was performed. Each time the ANOVA or the Kruskal–Wallis test detected significant differences among groups, pairwise multiple comparisons were made using Tukey or Dunn's post-hoc tests, respectively. ANOVAs were performed using the software package SigmaStat© (version 3.5), with significance level to be P < 0.05.
RESULTS
The monthly frequency of R. philippinarum at each stage of gonad maturity during the study period is presented in Figure 3. Gonadal development, maturation and ripening were clearly synchronous between males (Figure 3A) and females (Figure 3B), with both sexes displaying analogous temporal trends in reproductive dynamics throughout the year. Ripe male and female gonads (stage III) were observed mainly in April–May, followed by a period from June to September mostly with partially spawned gonads (stage IV) (Figure 3A, B). Still, during this period individuals were usually observed displaying diverse maturation stages within the same follicle, i.e. simultaneously releasing mature gametes and producing primary germ cells (spermatogonia and oogonia). Afterwards, the proportion of spent gonads (stage V) increased until December, followed by a period with clear predominance of inactive (stage 0) and early active gonads (stage I) mainly between January and March, reflecting a relatively long resting phase in the gametogenic cycle of R. philippinarum (Figure 3A, B).

Fig. 3. Monthly variation (bar charts) and annual frequency (pie charts) of gonad maturity stages of male (A) and female (B) Manila clam (Ruditapes philippinarum) throughout the study period in the Tagus Estuary.
Overall, the annual proportion of inactive gonads (stage 0: males = 34.7%; females = 36.5%), as well as the annual frequency of ripe individuals (stage III: males = 4.0%; females = 4.2%) were fairly comparable, further confirming the similarity and synchrony in the reproductive dynamics between sexes (Figure 3A, B). Still on an annual basis, partially spawned gonads (stage IV) were slightly more frequent in males (43.0%) than in females (36.1%), whereas the proportion of individuals with spent gonads (stage V) was lower in males (10.5%) compared with females (13.7%), probably reflecting more regular episodes of partial gamete release in males and complete spawning in females. The cumulative proportion of early active (stage I), late active (stage II) and ripe gonads (stage III) was fairly low (males = 11.9%; females = 13.7%), indicating a rapid process of gonad maturation, namely compared with the extended resting phase (stage 0: males = 34.7%; females = 36.5%) and spawning periods (stages IV + V: males = 53.5%; females = 49.8%) (Figure 3A, B).
The mean gonadal index (GI) exhibited a consistent oscillation throughout the study period and analogous variation between sexes (Figure 4), further confirming the synchronic gonadal development and maturation between males and females. The GI reached minimum values in winter (December–January) followed by sharp increases during spring, reaching maximum values indicative of gonad ripening in late spring (April–May). Following an intense gamete release (spawning peak), the GI decreased gradually during summer and autumn, reflecting the reduction in gonadal development and maturity towards the resting phase in the gametogenic cycle registered in winter.
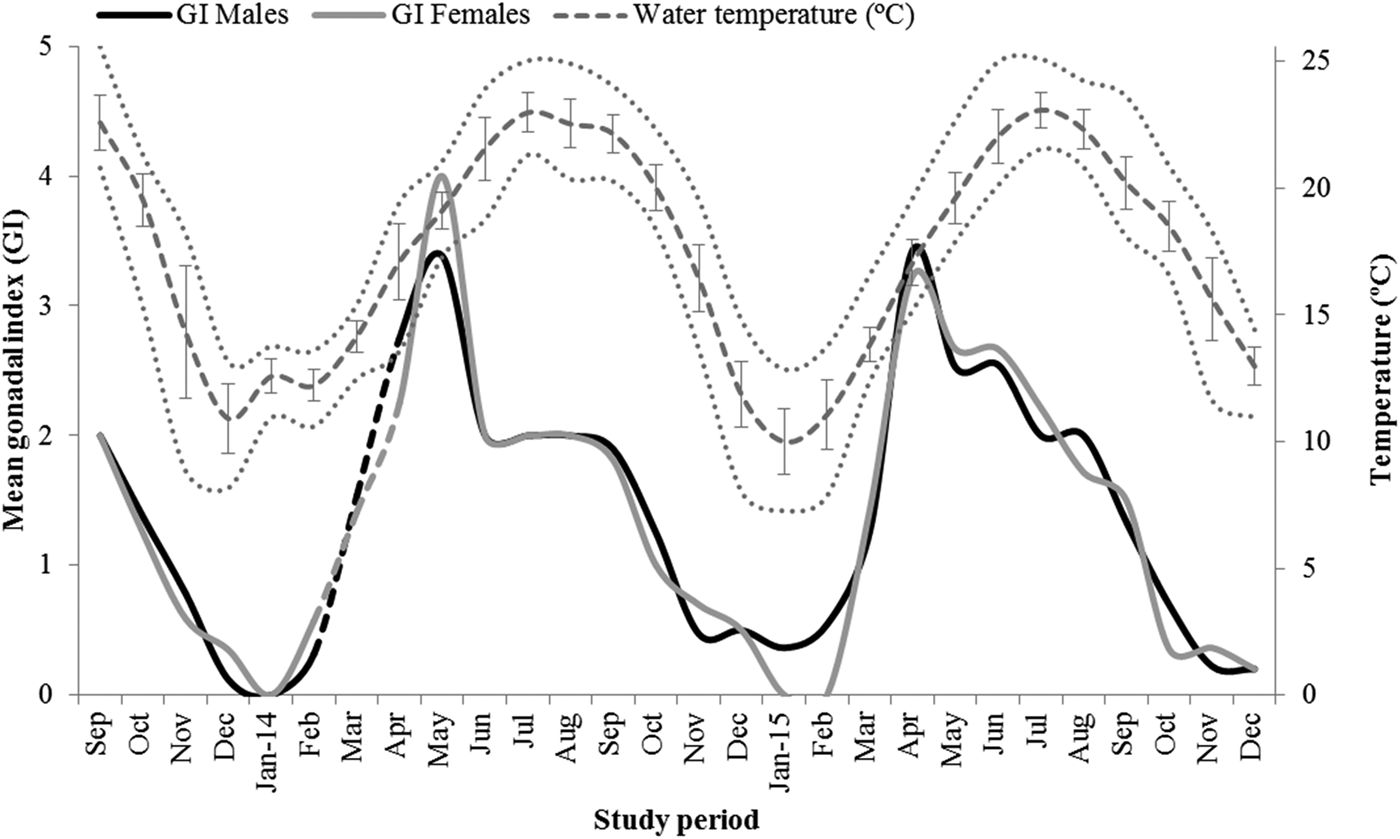
Fig. 4. Monthly variation of the mean gonadal index of male and female Manila clam (Ruditapes philippinarum) as a function of the water temperature in the Tagus Estuary. Water temperature presented as mean ± SD and range (solid and dashed lines, respectively).
The monthly variations in the GI of R. philippinarum were positively correlated with the seasonal fluctuation in water temperature in the Tagus Estuary (Pearson correlations – males: r = 0.684, P < 0.001; females: r = 0.679, P < 0.001). Gonad maturation and ripening were triggered by the continuously increasing temperatures from winter (December–January ~10–12°C) to late spring (April–May ~17–19°C) (Figure 4). In addition, gamete release occurred immediately before the period with warmest water temperatures (June–August ~21–23°C), whereas the relatively long resting phase in the gametogenic cycle coincided with the lowest water temperatures registered throughout the year in the Tagus Estuary (December–February ~10–13°C) (Figure 4).
The diameter of a total of 4881 oocytes of R. philippinarum was measured during the entire study period, with an average diameter of 22.6 ± 11.1 µm (range: 2.8–54.2 µm). The variation in oocyte diameter as a function of gonad maturity stage and its monthly fluctuation during the study period are presented in Figure 5. As expected, smaller oocytes were found in early active gonads (stage I = 9.7 ± 6.3 µm) and larger oocytes were registered in ripe gonads (stage III = 25.1 ± 10.3 µm), closely followed by oocytes still present in partially spawned (stage IV = 24.8 ± 10.2 µm) and spent gonads (stage V = 23.5 ± 11.2 µm) (Figure 5A). Mean oocyte diameter presented highly significant differences (K–W: H = 1020.062, d.f. = 4, P < 0.001) throughout gonadal maturation, with evident and continuous increases from stage I to II (Dunn test: Q = 8.109, P < 0.05) and from stage II to III (Dunn test: Q = 5.713, P < 0.05), but without significant differences in mean oocyte diameter between stages III, IV and V, i.e. although the number of large oocytes decreased markedly following gamete release, the diameter of the oocytes retained inside partially spawned follicles remained fairly constant during the spawning season (Figure 5A).

Fig. 5. Oocyte diameter at each gonad maturity stage (A) and monthly variation in oocyte diameter (B) of the Manila clam (Ruditapes philippinarum) from the Tagus Estuary. Different superscript letters denote statistically significant differences (P < 0.05) in mean oocyte diameter between gonad maturity stages. Arrows denote statistically significant differences (P < 0.05) in mean oocyte diameter between consecutive monthly samples.
Mean oocyte diameter displayed highly significant monthly oscillations (K–W: H = 1814.592, d.f. = 23, P < 0.001), evidenced by a marked seasonal variation throughout the study period, with minimum values registered during winter–early spring (mainly from January to March–April) followed by sharp increases towards May (Figure 5B), corroborating the general trend already evidenced by the monthly variation in the GI (Figure 4). Subsequently, rough and slight oscillations in oocyte diameter occurred until the end of the year (November–December), probably due to the co-occurrence of individuals in different stages of gonadal development, as well as the above mentioned individuals displaying diverse maturation stages within the same follicle (Figure 5B).
Regarding the estimation of the size at first sexual maturity (SL50), the smallest mature individuals (with gonadal maturation assigned to stages III or IV) belonged to the size class 28 mm SL and the vast majority (≥90%) of the specimens above 33 mm SL were already mature. The fitted maturity ogive revealed that the size at first sexual maturity (SL50) is reached at 29.4 mm SL, 75% of the individuals are mature at 31.4 mm SL and full maturation (100% mature individuals) is attained at 40.0 mm SL (Figure 6).

Fig. 6. Size at first sexual maturity (SL50) of the Manila clam (Ruditapes philippinarum) from the Tagus Estuary, showing the proportion of ripe (stage III) and partially spawned individuals (stage IV) as a function of shell length (grouped into size classes of 1 mm SL).
DISCUSSION
The invasive population of R. philippinarum in Tagus Estuary displayed a clearly synchronous gonadal development between sexes, as confirmed by the analogous monthly variation in both the frequency of gonad maturation stages and the mean gonadal index (GI) throughout the study period, which reflects fairly simultaneous gonad maturation and gamete release between males and females. Similarly, Dang et al. (Reference Dang, de Montaudouin, Gam, Paroissin, Bru and Caill-Milly2010) also did not detect differences in spawning chronology between males and females of the Manila clam in the Arcachon Bay (south-western France). This synchronicity in gonadal ripening and gamete release is a reproductive strategy that certainly contributed to the successful establishment of those invasive populations. However, in R. philippinarum from Galicia (north-western Spain), females started releasing gametes two months earlier than males (Rodríguez-Moscoso et al., Reference Rodríguez-Moscoso, Pazo, García and Fernández-Cortés1992).
The gonadal ripening of R. philippinarum occurred mainly in April and May in the Tagus Estuary, followed by an extensive spawning period until November–December. Throughout this period, most individuals with partially spawned gonads simultaneously released mature gametes and produced primary germ cells, an ability that allows for such an extensive spawning season. Similarly, most studies on the reproductive cycle of the Manila clam performed in other geographic areas (in both the Atlantic Ocean and the Mediterranean Sea) reported a long spawning season, beginning in late spring or summer and lasting until autumn. The only previous study on the reproductive cycle of this invasive species in Portuguese waters also reported a prolonged spawning season in the population of R. philippinarum from the Ria de Aveiro (Maia & Gaspar, Reference Maia and Gaspar2014). However, the gametic emission started and ended one month earlier (April–October) and the spawning peak occurred later (August–September) in the Ria de Aveiro (Maia & Gaspar, Reference Maia and Gaspar2014) compared with the present population from the Tagus Estuary. Gametogenesis and spawning are known to vary between geographic locations along a latitudinal gradient, due to phenotypical responses to variations in environmental conditions, mainly water temperature (e.g. Hesselman et al., Reference Hesselman, Barber and Blake1989). In this context, the lag in the spawning peak between populations of R. philippinarum from the Tagus Estuary (central western Portugal) and from the Ria de Aveiro (north-western Portugal) might be due to the delay in rising water temperature and higher values reached in the Tagus Estuary (maximum = 23°C, present study) than in the Ria de Aveiro (maximum = 20°C, Matias et al., Reference Matias, Joaquim, Matias, Moura, Teixeira de Sousa, Sobral and Leitão2013).
Differences in water temperature during periods of gonad maturation and spawning might also be responsible for the highly variable spawning patterns exhibited by populations of R. philippinarum introduced in the Atlantic Ocean and Mediterranean Sea (Çolakoğlu & Palaz, Reference Çolakoğlu and Palaz2014). In the present study, such variability was evidenced by monthly fluctuations in the GI clearly induced by the seasonal variation in water temperature in the Tagus Estuary. Gonad maturation and ripening were triggered by increasing temperatures and gamete release occurred immediately before the period with warmest water temperatures (June–August ~21–23°C), providing suitable conditions for larval development, settlement and nutrition, whereas the resting phase coincided with the period with coldest water temperatures (December–February ~10–13°C). Several studies with other bivalve species also considered seawater temperature as the main environmental factor responsible for inducing gametogenesis and spawning in bivalves (Moura et al., Reference Moura, Gaspar and Monteiro2008; Joaquim et al., Reference Joaquim, Matias, Matias, Moura, Arnold, Chícharo and Gaspar2011; Delgado et al., Reference Delgado, Silva and Juárez2013). In the specific case of R. philippinarum, laboratory experiments recreating the environmental conditions in the coastal waters of Galicia (Spain) revealed that the rate of gonadal development was directly associated with increasing water temperature (Delgado & Pérez-Camacho, Reference Delgado and Pérez-Camacho2007). Similarly, the Manila clam from Bandirma Bay (Turkey) appears to spawn following a major rise in water temperature (Çolakoğlu & Palaz, Reference Çolakoğlu and Palaz2014), whereas spawning of R. philippinarum in Simpo (Korea) occurs at water temperatures higher than 24°C (Chung et al., Reference Chung, Ryou and Lee1994).
In addition, fluctuation in water temperature also influenced the mean oocyte diameter of R. philippinarum, with minimum values recorded during winter, followed by sharp increases towards May (corresponding to the species maturation peak in the Tagus Estuary). Nevertheless, the diameter of the oocytes retained inside partially spawned follicles remained fairly constant, leading to an evident similarity in oocyte size classes after reaching gonad maturation and throughout the entire spawning season. In the Manila clam from Brittany (France), small oocytes prevail at the beginning of maturation, whereas large oocytes predominate just before spawning, denoting an accumulation of ripe oocytes before partial gametic emissions or total spawning events (Laruelle et al., Reference Laruelle, Guillou and Paulet1994). Similarly, high homogeneity in oocyte diameter in mature gonads of R. philippinarum was reported by Delgado & Pérez-Camacho (Reference Delgado and Pérez-Camacho2007).
The Manila clam population from the Tagus Estuary reaches first sexual maturity slightly below 30 mm SL (smallest mature individuals = 28.0 mm; SL50 = 29.4 mm), i.e. just before the individuals attain 1 year old (29.4 mm SL = 0.84 year old) (Moura et al., Reference Moura, Garaulet, Vasconcelos, Chainho, Costa and Gaspar2017), whereas R. philippinarum in the Ria de Aveiro reaches maturation at a considerably smaller size (20.0 mm SL) (Maia & Gaspar, Reference Maia and Gaspar2014). Unfortunately, to the authors’ best knowledge, besides the present work and the study by Maia & Gaspar (Reference Maia and Gaspar2014), there are no further data available in the literature to allow comparisons of the size/age at first sexual maturity in introduced populations of the Manila clam. Apparently, the native populations of the Manila clam from Korea and the Sea of Japan (Ponurovsky & Yakovlev, Reference Ponurovsky and Yakovlev1992; Chung et al., Reference Chung, Hur, Hur and Lee2001, Reference Chung, Chung and Lee2013; Choi et al., Reference Choi, Park and Chung2005) reach sexual maturity at a considerably smaller size than the present population of R. philippinarum introduced in the Tagus Estuary (although these individuals grow faster and achieve maturity at a comparatively younger age).
In several European estuaries, the introduced Manila clam has become a widespread and predominating species supplanting the native congeneric R. decussatus, whereas this trend has not been detected in other estuaries (Bidegain et al., Reference Bidegain, Bárcena, García and Juanes2015). In this context, the present study also aimed to assess the potential harmful effects of the invasive Manila clam over the autochthonous bivalve populations in the Tagus Estuary, which might eventually result from different reproductive strategies and dynamics between these congeneric species. For this purpose, a comparison of the main spawning seasons and/or peaks between R. philippinarum and R. decussatus is compiled in Table 1.
Table 1. Comparison of the spawning season and peak between the invasive Manila clam (Ruditapes philippinarum) and the native carpet shell clam (Ruditapes decussatus) in European water bodies.

Notes: ATL, Atlantic Ocean; ADR, Adriatic Sea; MAR, Marmara Sea; RP, Ruditapes philippinarum; RD, Ruditapes decussatus; *, month without samples; ♂, males only; ♀, females only; BC, biochemical composition; CI, condition indices; GH, gonadal histology; GS, gonad smear; GSI, gonadosomatic index; OS, oocyte size-frequency; SA, stereological analysis.
In Portuguese waters, the Manila clam introduced in the Tagus Estuary exhibited a longer spawning season compared with populations of the native congeneric R. decussatus from Ria Formosa (Banha, Reference Banha1984; Matias et al., Reference Matias, Joaquim, Matias, Moura, Teixeira de Sousa, Sobral and Leitão2013), Ria de Aveiro (Matias et al., Reference Matias, Joaquim, Matias, Moura, Teixeira de Sousa, Sobral and Leitão2013; Maia & Gaspar, Reference Maia and Gaspar2014) and Lagoa de Óbidos (Machado, Reference Machado2015a). Most of these populations start releasing gametes in late spring, but only R. philippinarum extends the period of gametic emission until November (Table 1).
In Andalucia (southern Spain), the introduced R. philippinarum also presented a longer spawning season than the native R. decussatus, releasing the gametes two months earlier than the autochthonous clam (Anon., 2001). In contrast, in Galicia (north-western Spain) there were no clear differences in these species' reproductive cycles, both with a spawning period lasting 5–6 months, but with R. decussatus spawning first than R. philippinarum (Rodríguez-Moscoso et al., Reference Rodríguez-Moscoso, Pazo, García and Fernández-Cortés1992; Rodríguez-Moscoso & Arnaíz, Reference Rodríguez-Moscoso and Arnaíz1998; Ojea et al., Reference Ojea, Pazos, Martínez, Novoa, Sánchez and Abad2004, Reference Ojea, Martínez, Novoa and Cerviño-Otero2005). The longer spawning season of the Manila clam in Portugal and southern Spain (Galicia) than in northern Spain (Galicia) might be due to higher water temperatures registered at lower latitudes. Indeed, an increasing trend in fecundity and reproductive output with decreasing latitude has been identified for diverse taxa (e.g. Thatje et al., Reference Thatje, Lovrich and Anger2004; Ward & Hirst, Reference Ward and Hirst2007; Thorsen et al., Reference Thorsen, Witthames, Marteinsdóttir, Nash and Kjesbu2010). Moreover, the longer spawning season provides a competitive advantage to R. philippinarum that allowed its colonization, establishment and predominance, also contributing to the virtual disappearance of the native R. decussatus from the Tagus Estuary.
In Brittany (north-western France), the reproductive activity of R. philippinarum displayed quicker maturation and higher number of spawning events compared with R. decussatus (Laruelle et al., Reference Laruelle, Guillou and Paulet1994). However, in the Arcachon Bay (south-western France), the Manila clam showed poor reproductive performance, with such weak gametogenesis (low condition index) and poor recruitment that the establishment of a sustainable population of R. philippinarum in Arcachon Bay was doubtful (Dang et al., Reference Dang, de Montaudouin, Gam, Paroissin, Bru and Caill-Milly2010). In the Venice Lagoon (Italy), the Manila clam exhibited an extensive spawning season spreading from May to September (Meneghetti et al., Reference Meneghetti, Moschino and Da Ros2004), while R. decussatus took only two months (August–September) releasing gametes (Breber, Reference Breber1980).
In the Çardak Lagoon (Turkey), the extensive spawning season of the Manila clam encompassed seven months and was longer than that of the native R. decussatus (Genez et al., Reference Genez, Önal and Gezen2015). In this study, the Manila clams were at a more advanced state and exhibited earlier reproductive activity throughout the year compared with the European carpet shell clams. In addition, significant differences after spawning were found between species from late autumn to early spring, with most R. philippinarum retaining the gonad follicular structure with new gametes developing inside, while R. decussatus displayed a quiescence period with a significantly higher proportion of individuals in inactive stage (Genez et al., Reference Genez, Önal and Gezen2015). Another study with the native carpet shell clam from the Çardak Lagoon reported a short spawning period of only four months followed by a resting phase between November and March (Gozler & Tartan, Reference Gozler and Tartan2000). In Bandirma Bay (Turkey), R. philippinarum exhibited continuous gametogenic activity throughout the year, with gonadal ripening starting at average water temperatures of 12°C and with the spawning season occurring between May and August (Çolakoğlu & Palaz, Reference Çolakoğlu and Palaz2014).
In Cork Harbour (southern Ireland), a study compared the gametogenic cycles of R. philippinarum and R. decussatus, revealing that the introduced Manila clam spawned for three months (September–November) while the European native carpet shell clam spawned during only two months (August–September), a difference attributed to the effect of local seawater temperature (Xie & Burnell, Reference Xie and Burnell1994). Although R. philippinarum completed the gametogenic cycle, the gonadal development and spawning were delayed by the relatively low temperature faced in southern Ireland (Xie & Burnell, Reference Xie and Burnell1994), namely compared with populations established in warmer climates (Ohba, Reference Ohba1959; Sarasquete et al., Reference Sarasquete, Gimeno and Gonzalez de Canales1990). In Drumcliff Bay (north-western Ireland), the Manila clam presented a continuous small-scale spawning event from May to July and a major spawning period between July and September (Drummond et al., Reference Drummond, Mulcahy and Culloty2006). Although individuals did not spawn in October and November, the ripe gametes observed during this period were probably resorbed into the gonad due to unsuitable water temperatures for spawning (Drummond et al., Reference Drummond, Mulcahy and Culloty2006). Such findings are roughly similar to those reported in a previous study on the reproduction of the Manila clam in southern Ireland (Xie & Burnell, Reference Xie and Burnell1994).
The reproductive dynamics in molluscs, including the seasonal regulation of gonad development, gametogenesis, breeding and spawning, are controlled by environmental cues that allow reproduction to occur at the most favourable time (Wayne, Reference Wayne2001; Harding et al., Reference Harding, Mann and Kilduff2008; Hotchkiss et al., Reference Hotchkiss, Sternberg and LeBlanc2008). In particular, water temperature is one of the most relevant environmental parameters that control the gametogenesis of molluscs (e.g. Sastry, Reference Sastry, Giese and Pearse1975; Mann, Reference Mann1979) and trigger the precise timing of breeding and spawning, therefore also being responsible for inter-annual variations in the reproductive cycle (Sternberg et al., Reference Sternberg, Gooding, Hotchkiss and Leblanc2010). In the present case, several studies have demonstrated that the gametogenic cycle of R. philippinarum depends mostly on latitude and water temperature (e.g. Yamamoto & Iawata, Reference Yamamoto and Iawata1956; Ohba, Reference Ohba1959; Holland & Chew, Reference Holland and Chew1974). In addition, the fecundity and reproductive output of the Manila clam apparently exhibit spatial and temporal variability. For instance, the spawning events of R. philippinarum varied at the kilometre scale within the Arcachon Bay (Dang et al., Reference Dang, de Montaudouin, Gam, Paroissin, Bru and Caill-Milly2010).
However, as a general trend, the onset of gametogenesis and maturity of the Manila clam in northern Europe begins later in the year (Ireland: Xie & Burnell, Reference Xie and Burnell1994; Drummond et al., Reference Drummond, Mulcahy and Culloty2006) compared with populations of R. philippinarum from southern Europe, whose gamete production starts in January–February and sexual maturity is reached during late spring (Portugal: present study, Maia & Gaspar, Reference Maia and Gaspar2014; Spain: Rodríguez-Moscoso et al., Reference Rodríguez-Moscoso, Pazo, García and Fernández-Cortés1992, Anon., 2001, Ojea et al., Reference Ojea, Martínez, Novoa and Cerviño-Otero2005; France: Laruelle et al., Reference Laruelle, Guillou and Paulet1994, Dang et al., Reference Dang, de Montaudouin, Gam, Paroissin, Bru and Caill-Milly2010; Italy: Meneghetti et al., Reference Meneghetti, Moschino and Da Ros2004; Turkey: Çolakoğlu & Palaz, Reference Çolakoğlu and Palaz2014, Genez et al., Reference Genez, Önal and Gezen2015) (see further details in Table 1). The maturation rhythm detected in the present study in the Tagus Estuary is quite similar to that registered in Brittany (Laruelle et al., Reference Laruelle, Guillou and Paulet1994), since in both cases the cumulative proportion of early maturation stages and ripe gonads was fairly low compared with the extended resting phase and spawning periods. On the other hand, studies that performed direct comparisons between these two species (e.g. Laruelle et al., Reference Laruelle, Guillou and Paulet1994; Delgado & Pérez-Camacho, Reference Delgado and Pérez-Camacho2007) achieved similar results and revealed higher reproductive activity and performance in R. philippinarum than in R. decussatus (see further details in Table 1).
Overall, the present study confirmed the high reproductive potential of the Manila clam in the Tagus Estuary, probably due to favourable environmental conditions that allow prolonged spawning periods and promote its successful colonization and establishment. Similarly, several studies reported the potential of R. philippinarum outcompeting autochthonous clam species and establishing naturalized populations in diverse geographic areas with suitable environmental conditions for this species (e.g. Jensen et al., Reference Jensen, Humphreys, Caldow, Grisley and Dyrynda2004; Pranovi et al., Reference Pranovi, Franceschini, Casale, Zucchetta, Torricelli and Giovanardi2006; ICES, 2008; Genez et al., Reference Genez, Önal and Gezen2015). In contrast, other studies recorded population decline and decreased individual performance, suggesting that low condition indices and overall fitness of R. philippinarum might be due to poor trophic condition, high prevalence and intensity of disease, environmental pollution and poor efficiency of the immune system (de Montaudouin et al., Reference de Montaudouin, Lucia, Binias, Lassudrie, Baudrimont, Legeay, Raymond, Jude-Lemeilleur, Lambert, Le Goïc, Garabetian, Gonzalez, Hégaret, Lassus, Mehdioub, Bourasseau, Daffe, Paul-Pont, Plus, Do, Meisterhans, Mesmer-Dudons, Caill-Milly, Sanchez and Soudant2016). Moreover, loss of genetic variability and significant genetic differentiation detected in European populations might help to prevent new invasion waves and further spreading of R. philippinarum on this continent (Cordero et al., Reference Cordero, Delgado, Liu, Ruesink and Saavedra2017).
In this context, additional studies are crucial to improve the knowledge on the behaviour and competiveness of the Manila clam, both in the invaded Tagus Estuary and in other Portuguese water bodies where the occurrence of R. philippinarum has already been reported. Indeed, the ongoing effects of the Manila clam distribution, population dynamics and related impacts, are most helpful to determine the invasiveness potential of this species (Copp et al., Reference Copp, Vilizzi, Tidbury, Stebbing, Tarkan, Miossec and Goulletquer2016). Such information would provide valuable baseline information to propose and implement adequate conservation strategies and management measures to minimize the harmful impacts caused by this invasive species on local ecosystems and native biodiversity, especially over populations of autochthonous bivalves.
ACKNOWLEDGEMENTS
The authors acknowledge Mr Jaime Oliveira for providing the logistics for performing the fishing surveys, the crew of the fishing boat ‘Pérola Negra’ (vessel registration: VX-754-L), namely the skipper João Miguel Letra and the fisherman João Paradela, for collecting the samples, and the technical staff of the Instituto Português do Mar e da Atmosfera (IPMA, I.P.) for their helpful assistance in the laboratory sampling procedures. Thanks are also due to Dr Owen Wangensteen (Associate Editor) and three anonymous reviewers for valuable comments and suggestions that improved the revised manuscript.
FINANCIAL SUPPORT
The present study was funded within the framework of the research project ‘Amêijoa-japonesa – estado actual da população do estuário do Tejo, impactos e gestão da apanha’, coordinated by the General-Directorate for Natural Resources, Security and Maritime Services (DGRM – Portugal), funded by the Fisheries Operational Programme (PROMAR) and co-financed by the European Fisheries Fund (EFF 2007–2013), by the strategic plan of MARE – Centro de Ciências do Mar e do Ambiente (UID/MAR/04292/2013) and by national funds from the Fundação para a Ciência e Tecnologia (FCT) through the project UID/Multi/04326/2013. The author Paulo Vasconcelos was funded by a post-doctoral grant (SFRH/BPD/26348/2006) awarded by the Fundação para a Ciência e Tecnologia (FCT).