Introduction
For more than 40 years, total body irradiation (TBI) has been used as an important part of patients’ conditioning regimen prior to bone marrow transplantation (BMT). Reference Kunkele, Engelhard and Hauffa1–Reference Allahverdi, Geraily, Esfahani, Sharafi, Haddad and Shirazi4 TBI in conjunction with chemotherapy is applied for immunosuppression and eradicates mutant cancer cells prior to BMT. Reference Ganapathy, Kurup, Murali, Muthukumaran, Bhuvaneshwari and Velmurugan5 Inadequate dose received by whole body in TBI reduces the chance of successful transplantation. Reference Allahverdi, Geraily, Esfehani, Sharafi, Haddad and Shirazi6 However, the organs at risk (OARs) that received dose should be maintained below the tolerance level. Reference Peters, Taylor and Turner7,Reference Webster8 Although in TBI, the prescription point is generally considered in the midline of patient thickness at the level of umbilicus, Reference Van Dyk, Galvin, Glasgow and Podgorsak9 but dose uniformity in whole body is necessary. In other words, because the targeted cells are distributed throughout the whole body including skin surface, a uniform dose delivery is necessary. Reference Van Dyk, Galvin, Glasgow and Podgorsak9
To increase dose uniformity in TBI, the radiation beam energy should be increased. But energy increment in turn leads to an increase in the depth of the build-up region and a decrease in the surface-absorbed dose. Reference Van Dyk, Galvin, Glasgow and Podgorsak9–Reference Hoseinnezhad, Geraily, Esfahani, Farzin and Gholami12 This is an important issue in some cases treated by TBI such as leukaemia, in which cancerous cells have spread to all parts of the body, including the superficial capillaries of the skin. In TBI, a surface dose (normally 1 mm deep or greater) of at least 90% of prescribed dose is required to ensure that sufficient dose is received by skin, superficial lymphatics, rib bone marrow, skull, and sternum. Reference Mekdash, Shahine, Jalbout, Chehab, Abdel Khalek and Youssef13
The purpose of this study was to evaluate the superficial dose in TBI technique. First, the TBI technique was implemented on human-like phantom, in parallel-opposed (POP) anterior/posterior (AP/PA) geometry with 18-MV photon beam. Then, absorbed dose was measured at the depth of 5 and 10 mm in eight selected points.
Materials and Methods
In this study, all irradiation steps were performed using a CLINAC 2100C/D linear accelerator (Varian Medical System, Palo Alto, CA, USA). The GAFCHROMIC-EBT3 films (ISP, Wayne, NJ, USA) were applied for surface dosimetry. In order to reduce statistical errors for dose measurements, every measurement was repeated three times. Reference Saur and Frengen14 The films were used according to the recommendations of AAPM TG-55 report. Reference Niroomand-rad, Blackwell and Coursey15
TBI treatment technique
The TBI technique was implemented on the human-like phantom. Reference Fahimi Monzari, Geraily and Toolee16 During irradiation, this phantom was positioned on the left and right side and irradiated in AP/PA geometry. The phantom was positioned on a TBI couch at 312-cm treatment distance. The phantom’s body surface had 5-cm distance from a 10-mm spoiler, which was embedded as a frame around the couch surface. In order to protect the lungs from receiving excess radiation, the lung shields were mounted to the spoiler at the level of the lung. Figure 1 schematically demonstrates the phantoms, Linac, spoiler and lung blocks position. Irradiation was performed with 18-MV photon beam. The total prescribed dose was 12 Gy, which had to be delivered as 2 Gy per 6 fractions (Fx) (2 Gy × 6 Fx). The prescription point was considered in the midline of the phantom at the level of umbilicus. Reference Mekdash, Shahine, Jalbout, Chehab, Abdel Khalek and Youssef13,Reference Jahnke, Jahnke and Molina-Duran17,Reference Lu, Filippi and Patel18
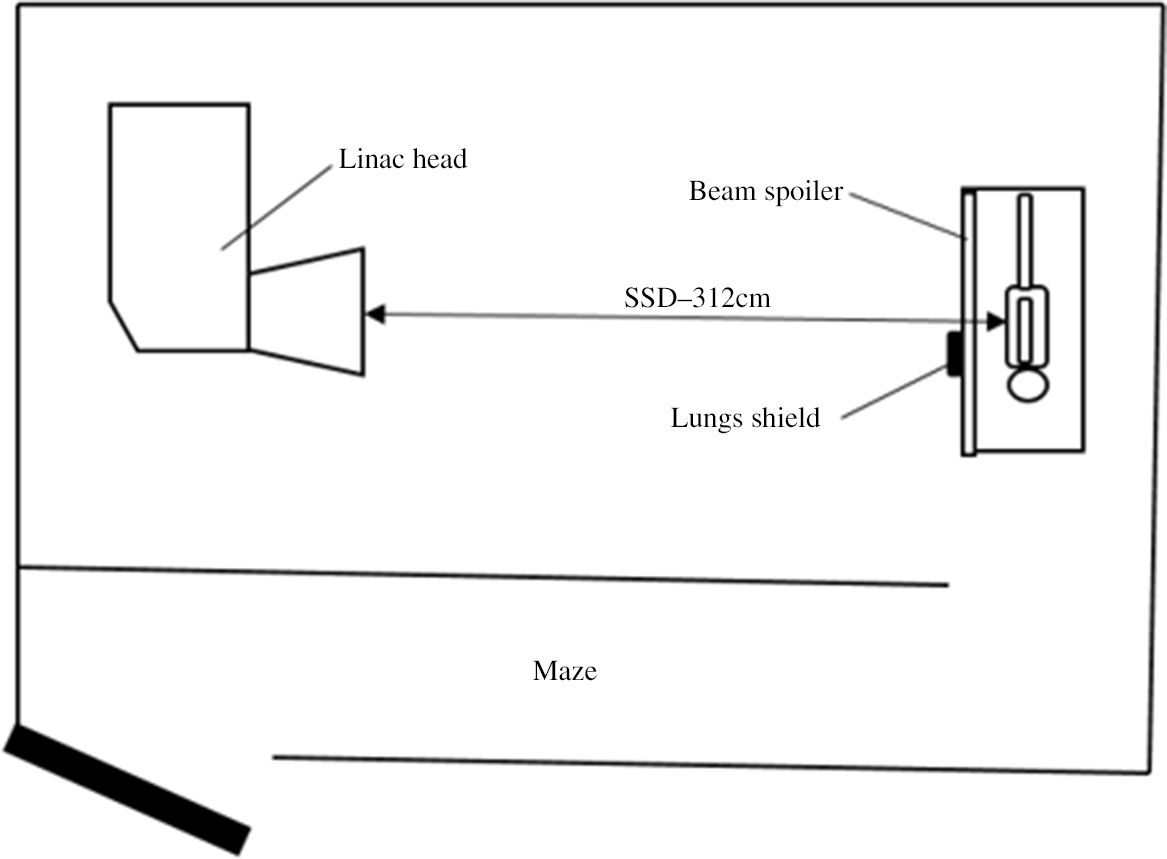
Figure 1. During total body irradiation (TBI) technique implementation, the source-surface distance (SSD) was set at 312 cm, and the gantry of the Linac rotated 90 degrees. The phantom was positioned on left/right side, and the lungs blocks were mounted to the beam spoiler at 5 cm distance from the prescription point (umbilicus).
Surface dose measurements
Prior to phantom irradiation, the GAFCHROMIC-EBT3 film ribbons were placed within the layers of phantom at eight anatomical regions including head, neck, chest (lungs), abdomen, hip, high, knee and leg. The points of measurements are shown in Figure 2. The films were previously calibrated under TBI conditions, and the calibration curve was obtained. Forty-eight hours after irradiation, the films were scanned using a Microtek 9800 XL scanner (Microteck, Taiwan) in RGB colour mode and 150 dots per inch (dpi) resolution. Reference Sim, Wong and Ng19,Reference Sorriaux, Kacperek and Rossomme20 The films were analysed with ImageJ software for pixel value determination. 21 Since the radiation dose during one fraction was below 10 Gy, therefore the red channel was chosen for film analysis. Reference Vadrucci, Esposito and Ronsivalle22 For each piece of the film, a circular region of interest (ROI) was selected at the depth of 5 and 10 mm. Finally, the value of absorbed dose was obtained using the obtained calibration curve, and the percentage of the absorbed dose at the body surface was obtained using equation 1:


Figure 2. Points of surface dose measurement during total body irradiation (TBI) technique.
where %d s is the percentage surface dose (5 or 10 mm depth), d s is the surface-absorbed d r is the absorbed dose at the prescription point in Gy.
Results
Surface dose results
The results of percentage surface dose at depths of 5 and 10 mm are presented in Figure 3. It can be seen that absorbed dose for all measurement points, except the chest wall, was approximately between 100%–122% for 5 mm depth and 105%–126% for 10 mm depth. Maximum absorbed dose was recorded in the leg and chest wall (without applying lung shield). Minimum absorbed dose was recorded in the chest wall (with applying lung shield).

Figure 3. Percentage surface dose at the depth of 5 and 10 mm at 8 anatomical selected points.
The profile of the absorbed dose at the umbilical level normalised to the prescription point (12 cm depth) is shown in Figure 4. According to this curve, the percentage absorbed dose was 100·89% and 106·08% at 5 mm and 10 mm depth, respectively.

Figure 4. The transversal absorbed dose profile at the level of umbilicus (the absorbed dose values were normalised to the absorbed dose at prescription point). Percentage absorbed dose at 5 and 10 mm is marked with dashed lines.
Discussion
For a successful bone marrow transplantation, the patients’ immune system is required to be suppressed. For this purpose, TBI is used in combination with chemotherapy, with the aims of uniform dose delivery (in the range of ±10% of the prescribed dose) through whole body while restricting the received dose by sensitive organs, especially the lungs, below the tissue tolerance threshold. Reference Peters, Taylor and Turner7,Reference Yao, Bernard and Turian23,Reference Lu, Filippi and Patel24 As previously mentioned, during TBI, the whole body including skin is required to receive the therapeutic dose. Reference Khan, Gibbons, Jonathan, Pine and Moyer10 In this study, the TBI technique was implemented on the human-like phantom in AP/PA geometry with 18-MV photon energy in order to investigate the surface dose measurements using GAFCHROMIC-EBT3 film dosimeters. Reference Najafi, Geraily, Esfahani and Teimouri25
Khan Reference Khan, Gibbons, Jonathan, Pine and Moyer10 states that for most TBI protocols, the received dose by patient skin required to be at least 90% of the prescribed dose. To increase the surface dose, a 10-mm spoiler was placed at 5-cm distance from the phantom’s body. This spoiler brought the depth of maximum dose to shallower depth. However, at some regions may be insufficient surface dose seen.
As it can be seen from Figure 3 at all points, except the chest wall below shielded lungs, the surface dose exceeds 90% of prescribed dose. Generally, the use of spoiler leads to superficial dose improvement due to the increase in forward scatters. However, this surface dose increment cannot be attributed only to the use of beam spoiler but also the SSD increment (from 100 cm under standard treatment condition to 312 cm under TBI condition), and large field size of irradiation for TBI technique can be responsible for this phenomena. This result is in consistent with Buston et al.’s findings. Reference Butson, Pope, Haque, Chen, Song and Whitaker26 Higher absorption dose levels in areas other than the umbilicus could be caused by two reasons, one is the oblique incidence of the radiation beam on the body surface which brings the depth of maximum dose to shallower depth, therefore in points that are not located on the central axis of the beam, such as the leg the surface dose increases. Another reason is the presence of different tissue heterogeneities with low density such as lungs in the beam path which may result in more beam penetration and absorbed dose. Reference Khan, Gibbons, Jonathan, Pine and Moyer10
According to data present in Figure 3, the surface dose at chest wall region beyond lung shielding decreases significantly. So, it is recommended to apply an additional electron beam to boost the skin dose in lung region (chest wall). Reference Mekdash, Shahine, Jalbout, Chehab, Abdel Khalek and Youssef13,Reference Chen, Wu, Chuang, Lin, Lee and Lin27,Reference Patel, Warry and Eaton28 The boosting region could be specified based on the shadow of the lung shields on the skin. Reference Mekdash, Shahine, Jalbout, Chehab, Abdel Khalek and Youssef13 To irradiate only to that portion of the chest wall skin which was shielded with lung blocks, negative blocks can be applied which should exactly match the shape of the lung blocks (Figure 5). The energy of the electron beam should be selected according to the CT scan radiography of the chest region in a way that the 90% isodose line be placed at lung–chest wall interface. For this purpose, the measurement of the electron beam PDD under TBI conditions is required. Reference Dusenbery and Gerbi29

Figure 5. The electron and photon lung blocks. It can be seen that the shape of the electron blocks is exactly match to the shape of the photon lung blocks.
Also, data presented in Figure 3 show that for most measurement points, absorbed dose at a depth of 5 mm was lower than that at 10 mm depth. This fact can also be deduced from Figure 4, in which before build-up region, absorbed dose at 5 mm depth is lower than 10 mm depth. But for chest wall region under shielded lung, this phenomenon is less highlighted. It can be attributed to the greater involvement of electron contamination produced by lung shield which may have more contribution at depth 5 mm in comparison to 10 mm depth. Reference Narayanasamy, Cruz, Saenz, Stathakis, Papanikolaou and Kirby30
The main limitation of this study was the lack of dedicated treatment planning systems for TBI dose evaluations. So, all calculations were done manually which made it, somewhat, time-consuming and difficult. It may result in restricting the evaluation of surface dose to some specific regions. Evaluating surface dose at all region of body by Monte Carlo simulation or dedicated treatment planning system for TBI is recommended for further studies.
According to this study, except for the area under lung blocks, absorbed dose at the superficial regions in all dosimetric areas was within the acceptable limits. This information is useful for evaluating the implemented TBI technique. Although this study was performed on a human-like phantom, it can provide valuable information for predicting surface dose values in the human phase.
Conclusions
Evaluation of the shallow depth absorbed dose for a TBI technique is essential for treatment effectiveness. In this study, the surface dose was evaluated during the AP/PA-TBI with 18-MV photon beam. The beam spoiler was placed near the phantom’s body surface to increase the surface dose. The GAFCHROMIC-EBT3 films were used for dose measurement within the phantom layers. According to the results, surface dose uniformity was within the acceptable range. The 10 and 20 mm absorbed dose results were more than 90% for almost all points except the chest wall region, which was due to the presence of lung blocks. This reduction of lung’s absorbed dose can be solved by applying electron boost.
Acknowledgments
This work was supported by Tehran University of Medical Sciences (grant number: 96-03-30-36299).