INTRODUCTION
Emerging infectious diseases (infections that have newly appeared in a population, or have existed but are rapidly increasing in incidence or geographic range, Morse and Schluederberg, Reference Morse and Schluederberg1990) have become an important public health threat these last decades (Daszak et al. Reference Daszak, Cunningham and Hyatt2000; King et al. Reference King, Peckham, Waage, Brownlie and Woolhouse2006). The need for integrating evolutionary concepts to better understand and prevent them has recently been advocated (Vander Wal et al. Reference Vander Wal, Garant, Calmé, Chapman, Festa-Bianchet, Millien, Rioux-Paquette and Pelletier2014). Hence, hosts, vectors and pathogens evolve in response to environmental selective pressures but also as a result of inter-specific interactions, that, in turn, strongly impacts infectious disease dynamics and emergence.
Among important zoonotic (re-)emerging agents are hemorrhagic fever-causing hantaviruses of the Hantaviridae family (Vapalahti et al. Reference Vapalahti, Mustonen, Lundkvist, Henttonen, Plyusnin and Vaheri2003). They are mainly rodent-borne (Henttonen et al. Reference Henttonen, Buchy, Suputtamongkol, Jittapalapong, Herbreteau, Laakkonen, Chaval, Galan, Dobigny, Charbonnel, Michaux, Cosson, Morand, Hugot, Sparagano, Maillard and Figueroa2008) and are responsible for two kinds of human syndromes, hemorrhagic fever with renal syndrome (HFRS), mainly in Eurasia, and hantavirus cardiopulmonary syndrome (HCPS) in the Americas. For 20 years, since reliable tools for hantavirus infection diagnostics have become available, the (re-)emergence of zoonoses associated with hantaviruses has been recognized as a growing public health concern worldwide. In Europe, an increase in amplitude and frequency of epidemics due to hantaviruses has been observed these last 20 years (Heyman et al. Reference Heyman, Ceianu, Christova, Tordo, Beersma, Joao Alves, Lundkvist, Hukic, Papa, Tenorio, Zelena, Essbauer, Visontai, Golovljova, Connell, Nicoletti, Van Esbroeck, Gjeruldsen Dudman, Aberle, Avsic-Zupanc, Korukluoglu, Nowakowska, Klempa, Ulrich, Bino, Engler, Opp and Vaheri2011). Moreover, the geographic distribution of hantaviruses is expanding (e.g. for Seoul virus Jameson et al. Reference Jameson, Logue, Atkinson, Baker, Galbraith, Carroll, Brooks and Hewson2013; Mace et al. Reference Mace, Feyeux, Mollard, Chantegret, Audia, Rebibou, Spagnolo, Bour, Denoyel, Sagot and Reynes2013). Although this may be potentially and partly explained by increased clinician awareness and easier access to diagnostic tests, a significant rise in the real number of human cases is very likely (Heyman et al. Reference Heyman, Thoma, Marie, Cochez and Essbauer2012; Reynes et al. Reference Reynes, Dutrop, Carli, Levast, Fontaine, Denoyel and Philit2015). In France, Puumala virus (PUUV) is the main agent of hantavirus infections. It induces an attenuated form of HFRS called nephropathia epidemica (NE). PUUV is transmitted to humans via aerosols contaminated by excreta of its unique and specific natural reservoir host, the bank vole Myodes glareolus, which is a forest dwelling rodent species (Brummer-Korvenkotio et al. Reference Brummer-Korvenkotio, Henttonen and Vaheri1982). Although M. glareolus exhibits a spatially continuous distribution all over France, except on the Mediterranean coast, human cases are mostly being reported in the northeast part of the country (National Reference Center for Hantavirus http://www.pasteur.fr/fr/sante/centres-nationaux-reference/les-cnr/hantavirus, but see Reynes et al. Reference Reynes, Dutrop, Carli, Levast, Fontaine, Denoyel and Philit2015). Elsewhere, PUUV can be absent or the prevalence of PUUV in bank vole populations can reach high levels without any human case being reported yet (Castel et al. Reference Castel, Couteaudier, Sauvage, Pons, Murri, Plyusnina, Pontier, Cosson, Plyusnin, Marianneau and Tordo2015). Niche modelling approaches based on climatic and environmental factors have been conducted to better predict the spatial distribution of NE disease (Zeimes et al. Reference Zeimes, Quoilin, Henttonen, Lyytikaïnen, Vapalahti, Reynes, Reusken, Swart, Vainio, Hjertqvist and Vanwambeke2015). They highlighted geographic areas of high risk of NE emergence, although neither seropositive bank voles nor human cases had been detected yet. They also failed to detect geographic areas with high levels of PUUV prevalence in reservoir populations and no human case reported. These results suggest that the drivers of NE emergence are just beginning to be elucidated, and that other important factors than abiotic ones, including evolutionary processes and PUUV and/or host genetic characteristics, could be relevant. In particular, selective pressures affecting host–pathogen interactions may promote the evolution of different defence strategies in hosts, ranging from resistance, i.e. the ability to reduce pathogen burden to tolerance, i.e. the ability to limit the damage caused by a given parasite burden (Schneider and Ayres, Reference Schneider and Ayres2008; Raberg et al. Reference Raberg, Graham and Read2009). Previous field and laboratory studies have revealed some variability in the susceptibility of bank voles to PUUV infection, as reflected by the probability to be infected with PUUV (Olsson et al. Reference Olsson, White, Ahlm, Elgh, Verlemyr, Juto and Palo2002; Kallio et al. Reference Kallio, Klingström, Gustafsson, Manni, Vaheri, Henttonen, Vapalahti and Lundkvist2006; Deter et al. Reference Deter, Chaval, Galan, Henttonen, Laakkonen, Voutilainen, Ribas Salvador, Bryja, Morand, Cosson and Charbonnel2008b ; Dubois et al. Reference Dubois, Castel, Murri, Pulido, Pons, Benoit, Loiseau, Lakhdar, Galan, Charbonnel and Marianneau2017a ) and the pattern of PUUV excretion once bank voles are infected (Hardestam et al. Reference Hardestam, Karlsson, Falk, Olsson, Klingström and Lundkvist2008; Voutilainen et al. Reference Voutilainen, Sironen, Tonteri, Tuiskunen Back, Razzauti, Karlsson, Wahlstrom, Niemimaa, Henttonen and Lundkvist2015). Moreover, Guivier et al. (Reference Guivier, Galan, Ribas Salvador, Xuéreb, Chaval, Olsson, Essbauer, Henttonen, Voutilainen, Cosson and Charbonnel2010, Reference Guivier, Galan, Henttonen, Cosson and Charbonnel2014) have shown significant differences in the level of immune related gene expression in bank voles from NE-free and endemic regions at the European and regional scales. It was suggested that this immunoheterogeneity could reflect some balance of resistance/tolerance to PUUV. Indeed PUUV infection in bank voles is chronic and mainly asymptomatic (but see Tersago et al. Reference Tersago, Crespin, Verhagen and Leirs2012) and mounting immune responses is energetically costly and can induce immunopathologies (e.g. in human infections, Vaheri et al. Reference Vaheri, Strandin, Hepojoki, Sironen, Henttonen, Mäkelä and Mustonen2013). Guivier et al. (Reference Guivier, Galan, Ribas Salvador, Xuéreb, Chaval, Olsson, Essbauer, Henttonen, Voutilainen, Cosson and Charbonnel2010, Reference Guivier, Galan, Henttonen, Cosson and Charbonnel2014) therefore proposed that bank voles from NE-endemic areas would be more tolerant to the virus as a result of co-adaptation whereas those from NE-free ones would be more resistant.
In this context, we proposed to investigate the hypothesis that immunoheterogeneity between bank vole populations could influence the geographic distribution range of PUUV, using two complementary approaches, natural population surveys and experimental infections. We focused on bank vole populations settled on both parts of the southern limit of PUUV distribution in France (Fig. 1), i.e. in the NE-endemic area (human cases regularly reported, Jura, see National Reference Center for Hantavirus data) and in the NE-free area (no human case ever reported yet, Ain). Our first objective was to discard the possibility of a spatial barrier that could prevent or limit the transmission of PUUV from the Jura to the Ain bank vole populations. Our second objective was to test the assumption of an important regional immunoheterogeneity between bank vole populations that could reflect different levels of sensitivity to PUUV infection. We predicted bank voles from Ain to be less sensitive to PUUV infection than those from Jura. Under this hypothesis, we expected bank voles from Ain to exhibit lower probability of infection and higher levels of immune responses to PUUV infection, hence reflecting their higher levels of resistance to this hantavirus.

Fig. 1. Map of the study area. Each colour represents a site of trapping and each circle or diamond represents a trapping line. The location of the two regions (Jura and Ain) within France is shown on top left.
MATERIAL AND METHODS
Ethical statement
All animal works have been conducted according to the French and European regulations on care and protection of laboratory animals (French Law 2001-486 issued on June 6, 2001 and Directive 2010/63/EU issued on September 22, 2010). Experimental protocols have been evaluated and approved by the Animal Ethics Committee C2EA-16 (ANSES/ENVA/UPEC, CNREEA n°16).
Natural population studies
Rodent sampling
Bank voles were trapped in June and September 2014 in two adjacent regions of eastern France that have contrasted status in regard of human cases of NE. Jura is considered as an endemic zone as NE cases are regularly reported (National Reference Center for Hantavirus) whereas Ain is considered to be NE-free as a human case has never been reported yet (Fig. 1). These two areas are part of the same geological massif (Sommaruga, Reference Sommaruga1997) and they experience highly similar climatic conditions (WorldClim data, Supplementary Fig. 1).
Six sites were sampled in mixed forest of coniferous and deciduous tree species (Fig. 1, Table 1). The minimum distance between sites was 8·7 km. Six to ten lines of 20 live-traps (INRA) with about 5 m interval were set up so that each sampling site consisted of a few km2 area. Traps were baited with sunflower seeds. Each trap was geolocated. The traps were checked daily and early in the morning. Trapping session per site lasted at least three nights so that a minimum of 35 voles were caught.
Table 1. Sampling site characteristics.

a Bank voles were considered as juveniles when their weight was ⩽17·5 g (see Voutilainen et al. Reference Voutilainen, Kallio, Niemimaa, Vapalahti and Henttonen2016).
b The lungs of these two seropositive individuals were tested by qRT-PCR and were clearly PUUV negative, indicating that serological analyses revealed maternal antibodies in these juvenile bank voles.
Once trapped, animals were bled through retro-orbital sinus, killed by cervical dislocation, weighed, measured, sexed and dissected. Whole blood was stored at 4 °C until centrifugation to collect sera 24 h later. When the bladder was full of urine, we carefully opened it above a microtube to collect urine. Sera, lungs and urine were immediately placed in dry ice and then stored at −80 °C for virological and immunological analyses. Spleens were placed in RNAlater solution (Sigma) at 4 °C during one night then stored at −20 °C for population genetics and gene expression analyses.
Microsatellite genotyping
Genomic DNA was extracted from a piece of spleen using the the EZ-10 96-Well Plate Genomic DNA Isolation Kit for Animal (BioBasic) according to manufacturer's instructions, with a final elution of 400 µL in elution buffer. Genotyping was performed at 19 unlinked microsatellites loci previously published by Rikalainen et al. (Reference Rikalainen, Grapputo, Knott, Koskela and Mappes2008) using primers and cycling conditions described in Guivier et al. (Reference Guivier, Galan, Chaval, Xuereb, Ribas Salvador, Poulle, Charbonnel and Cosson2011) using an ABI3130 automated DNA sequencer (Applied Biosystems). Alleles were scored using GENEMAPPER software (Applied Biosystems).
Serological and virological analyses
Serum samples were screened by IgG ELISA as described in Klingstrom et al. (Reference Klingstrom, Heyman, Escutenaire, Sjolander, De Jaegere, Henttonen and Lundkvist2002). Due to the high cross-reactivity between the different hantavirus serotypes (Kruger et al. Reference Kruger, Ulrich and Lundkvist2001), the plates were coated with Tula virus infected and non-infected cell lysates. Previous experiments have shown that anti-PUUV positive and negative sera reacted similarly with lysates of PUUV and TULV infected cells and with recombinant PUUV nucleocapsid protein (Dubois et al. Reference Dubois, Castel, Murri, Pulido, Pons, Benoit, Loiseau, Lakhdar, Galan, Charbonnel and Marianneau2017a ).
Viral RNA was extracted from serum, lung and liver, which are target organs for PUUV (Gavrilovskaya et al. Reference Gavrilovskaya, Chumakov, Apekina, Ryltseva, Martiyanova, Gorbachkova, Bernshtein, Zakharova and Boiko1983; Bernshtein et al. Reference Bernshtein, Apekina, Mikhailova, Myasnikov, Khlyap, Korotkov and Gavrilovskaya1999), and urine when available, as it is a main route of excretion for PUUV (Hardestam et al. Reference Hardestam, Karlsson, Falk, Olsson, Klingström and Lundkvist2008), using the QIAamp Viral Mini Kit (Qiagen). Quantitative RT-polymerase chain reaction (qRT-PCR) were performed using 2·5 µL of viral RNA amplified using the SuperScript III One-Step RT-PCR system with Platinum Taq High Fidelity (Invitrogen) on LightCycler 480 (Roche Diagnostics) as described in Supplementary Table S1. Note that transcript RNA is treated by DNase to remove plasmid template. Relative amounts of viral RNA (expressed in RNA copy per mg of tissue or per μl of liquid) were calculated using a standard curve obtained with in vitro transcribed RNA. All samples were tested in duplicate to avoid false positives and negatives. The means of the duplicates were used in further analyses.
Immunological analyses
We quantified gene expression for three candidate immune related genes, namely Tlr7, Tnf-α and Mx2, that are relevant with regard to PUUV infections (Rohfritsch et al. Reference Rohfritsch, Guivier, Galan, Chaval and Charbonnel2013; Charbonnel et al. Reference Charbonnel, Pagès, Sironen, Henttonen, Vapalahti, Mustonen and Vaheri2014; Dubois et al. Reference Dubois, Galan, Guivier, Henttonen, Voutilainen, Razzauti, Gauffre, Marianneau, Cosson and Charbonnel2017b ). TLR7 is a receptor to virus and is probably involved in the detection of ssRNA viruses like hantaviruses (Bowie and Haga, Reference Bowie and Haga2005). The proinflammatory cytokine tumour necrosis factor alpha (TNF) and the antiviral protein Mx2 are known to limit PUUV replication in humans and in cell cultures (Kanerva et al. Reference Kanerva, Melen, Vaheri and Julkunen1996; Temonen et al. Reference Temonen, Mustonen, Helin, Pasternack, Vaheri and Holthofer1996; Jin et al. Reference Jin, Yoshimatsu, Takada, Ogino, Asano, Arikawa and Watanabe2001). We used β-actin as the endogenous reference gene, as previously validated by Friberg et al. (Reference Friberg, Lowe, Ralli, Bradley and Jackson2011) in wood mice.
We analysed a subset of 170 adult bank voles including 10 adult PUUV-seropositive individuals and a number of PUUV-seronegative individuals that enabled to reach about 30 voles per site with an equal sex ratio. Total RNA was extracted from a piece of spleen using the NucleoSpin© 96 RNA kit (Macherey–Nagel) following manufacturer's instructions. As secondary lymphoid organ, the spleen has important immune functions and is the site of low levels of PUUV replication (Korva et al. Reference Korva, Duh, Saksida, Trilar and Avsic-Zupanc2009). RNA extractions were electrophoresed on 1·5% agarose gels and visualized using ethidium bromide staining to check for quality, then total RNA concentrations were measured using a NanoDrop 8000 spectrophotometers (Thermo Scientific) and normalized to 200 ng μL−1 using RNase free water. For β-actin, Tnf-α and Mx2, we used specific primers described in previous studies (Guivier et al. Reference Guivier, Galan, Ribas Salvador, Xuéreb, Chaval, Olsson, Essbauer, Henttonen, Voutilainen, Cosson and Charbonnel2010, Reference Guivier, Galan, Henttonen, Cosson and Charbonnel2014). For Tlr7, we designed the following specific primers based on the cDNA consensus sequences obtained for 12 M. glareolus samples (GenBank Accession Numbers: KX463605–KX463616): Tlr7-Mg2-F (5′-TACCAGGACAGCCAGTTCTA-3′), Tlr7-Mg2-R (5′-GCCTCTGATGGGACAGATA-3′). We generated cDNA from 4 µL of extracted RNA (800 ng per reaction), in a 20 µL reaction, using the Improm-II Reverse Transcription System (Promega), according to the conditions specified by the manufacturer for oligo (dT)15 primers. We performed a quantitative PCR on a LightCycler 480 (Roche Diagnostics), using the 384-multiwell plate format, as previously described in Guivier et al. (Reference Guivier, Galan, Ribas Salvador, Xuéreb, Chaval, Olsson, Essbauer, Henttonen, Voutilainen, Cosson and Charbonnel2010, Reference Guivier, Galan, Henttonen, Cosson and Charbonnel2014). PCR efficiencies were estimated using the LinRegPCR software (Ruijter et al. Reference Ruijter, Ramakers, Hoogaars, Karlen, Bakker, van den Hoff and Moorman2009). Candidate gene mRNA relative expression levels were estimated for each sample using the method developed by Pfaffl (Reference Pfaffl2001).
Experimental studies
Rodent sampling
Bank voles were trapped in May 2015 in two sites already sampled in 2014 (Cormaranche forest in Ain and Arbois forest in Jura) and using the same sampling protocol as described above. They were brought back to the laboratory, tested for the presence of anti-PUUV IgG, held during 3 weeks in quarantine and then retested for the presence of anti-PUUV IgG. Ten voles from Ain and nine voles from Jura, all of them being seronegative, were transferred in a ABSL-3 capacity and held individually in ISOcages N (Tecniplast). Food and water were provided ad libitum, with fresh vegetables once a week.
Experimental infections
Because there is yet no PUUV French wild-type strain available for experimental infections, we used the PUUV Sotkamo laboratory strain. It enabled to perform infections in a reproducible and similar way for all bank voles, although its passages in cell culture may have lead to some particular adaptation with regard to pathogenicity (Lundkvist et al. Reference Lundkvist, Hukic, Hörling, Gilljam, Nichol and Niklasson1997). 1·7 × 103 f.f.u of PUUV Sotkamo strain 1 : 10 diluted in DMEM (ThermoFisher Scientific) were injected by subcutaneous route in each of the 19 bank voles. From 7 to 55 days post-infection (dpi), blood, saliva and feces were collected once a week for each bank vole. We did not succeed collecting urine samples while manipulating bank voles. Approximately 200 µL of whole blood was sampled through the retro-orbital sinus and stored at 4 °C until centrifugation to collect sera 24 h later. Saliva was collected using sterile swabs subsequently placed in 300 µL of Hank's Balanced Salt Solution (Life Technologies) and vortexed for 10 s. Faces were sampled directly from the anus. All samples were stored at −80 °C until analyses. At the end of the experiment (55 dpi), all the bank voles were euthanized by cervical dislocation. Blood, lungs, liver, kidneys, urine (when available) and feces were collected and stored at −80 °C until analyzes. Three bank voles, two from Ain and one from Jura, died during the course of experimental infection (at 28 dpi) and were not considered for further analyzes.
Serological and virological analyses
PUUV IgG detection was performed on sera as described above for the natural populations. Viral RNA was extracted from all samples (sera, lung, liver, kidney, feces, saliva and urine) and PUUV RNA detection was realized using qRT-PCR for all the samples as described earlier. To improve the sensitivity of viral detection, nested RT-PCR were applied to sera, feces and saliva, using the Titan One Tube RT-PCR System (Roche) and Taq DNA Polymerase (Qiagen). Indeed, detection thresholds are lower with this assay than with qRT-PCR (1 pfu mL−1). Note that only samples whose results were doubtful using qRT-PCR were confirmed with this method to limit risks of cross-contaminations.
Gene expression could not be performed on bank voles from this experiment as spleens could only be collected at 55 dpi. It was too far from the infection to expect any difference in gene expression between bank voles (Hardestam et al. Reference Hardestam, Karlsson, Falk, Olsson, Klingström and Lundkvist2008; Schountz et al. Reference Schountz, Acuna-Retamar, Feinstein, Prescott, Torres-Perez, Podell, Peters, Ye, Black and Hjelle2012), and it did not enable to study gene expression kinetics.
Statistical analyses
Natural population analyses
We analysed the genetic structure of bank vole populations using microsatellite data. We tested the conformity to Hardy–Weinberg equilibrium (HWE) for each locus and each population. We analysed linkage disequilibrium (LD) for each pair of loci using Genepop v4.2 (Raymond and Rousset, Reference Raymond and Rousset1995). Corrections for multiple tests were performed using the false discovery rate (FDR) approach as described in Benjamini and Hochberg (Reference Benjamini and Hochberg1995). We described the spatial genetic structure by estimating the genetic differentiation between each pair of sites using Weir and Cockerham's pairwise F ST estimates (Reference Weir and Cockerham1984). Significance was assessed using exact tests and FDR corrections. Several complementary analyses were performed to test for the existence of spatial barriers that could limit vole dispersal in this area, especially between Jura and Ain. We first computed a discriminant analysis of principal components (DAPC), which is a multivariate, model-free approach to clustering (Jombart et al. Reference Jombart, Devillard and Balloux2010). Because DAPC is sensitive to the number of principal components used in analysis, we used the function optim.a.score to select the correct number of principal components. We next performed an analysis of molecular variance (AMOVA) in Arlequin v3·5.1.2 (Excoffier and Lischer, Reference Excoffier and Lischer2010). Compared with the DAPC, it enabled to test specifically for genetic differences between regions, considering the potential variability between sites within each region. Finally models of isolation by distance (IBD) were applied to test for geographic–genetic correlations. IBD models were analysed independently for each region and for the whole dataset, so that disruption of gene flow between regions could also be detected. Genetic differentiation was estimated for each pair of individuals and Mantel tests were applied to test for a correlation between matrices of genetic differentiation and of Euclidean distances between individuals, using 10 000 permutations, in Genepop. We also calculated confidence intervals for the slope of the regression line by bootstrapping over loci (ABC intervals, Di Ciccio and Efron, Reference Di Ciccio and Efron1996).
We tested for variations of serological and immunological characteristics in natural populations between regions using generalized linear mixed models with the glmer function implemented in the lme4 package for R 3.1.0 (R Core Team, 2013). The fixed variables included in the models were region, sex and weight and all pairwise interactions. When they were non-significant, they were removed from the model. The factor site was included as a random factor. Chi-square tests with Bonferroni correction were applied to analyse the effect of significant variables using the package phia for R. We analysed independently PUUV serological status (using a binomial response variable) and each gene expression level Qn (using a log-transformed response variable for the three genes). To prevent confounding effects due to the potential induction of the three candidate genes expression following PUUV infection, we limited our statistical analyses to PUUV-seronegative bank voles (Guivier et al. Reference Guivier, Galan, Henttonen, Cosson and Charbonnel2014).
Experimentation analyses
We tested for regional differences in bank vole responses to PUUV experimental infection by examining the levels of anti-PUUV IgG (OD450 nm, using a normal response variable) between Jura and Ain through time. Generalized mixed linear models were applied as described above. The fixed variables included in the models were region, dpi and their interaction. Bank vole identity was included as a random effect. Possible variation in the level of viral RNA between regions was tested using a linear model with the fixed effect region.
RESULTS
Natural population studies
Population genetic structure
Three loci, Cg16H5, Cg16E4 and Cg6G11, were excluded from the genetic analyses due to the poor quality profiles obtained. Six bank voles were also excluded because they could not be amplified for most loci. Two other microsatellite loci, Cg2F2 and Cg3F12, showed deviations of HWE in four and six sites respectively, suggesting the presence of null alleles. They were removed from further analyses. Five out of 84 tests for deviation from HWE were significant with a different loci involved each time. In total 25 of 546 pairs of loci (4·58%) exhibited significant linkage disequilibrium but the loci involved were not consistent among sites. Thus, our final dataset included 271 bank voles genotyped at 14 microsatellites loci.
Spatial pairwise F ST ranged between 0·009 and 0·03. All of these estimates were significant after FDR correction (Table 2). DAPC clustering showed that the genetic variation of the six sites overlapped (Fig. 2), although the first discriminant component tended to slightly separate the two regions. The second discriminant component revealed that one site in Jura (Mignovillard) tended to be different from the three others and that the two sites in Ain tended to be separated from each other (Fig. 2). AMOVA analyses revealed an absence of significant differentiation between the two regions, and significant variation between sites within regions (Table 3). IBD patterns were highly significant whatever the dataset considered. Genetic differentiation between bank voles decreased with spatial distance, and the slope of these regressions were similar, respectively 0·003, 0·004 and 0·005 for Ain, Jura and the whole dataset, confirming an absence of spatial barrier between the two regions (Table 4).
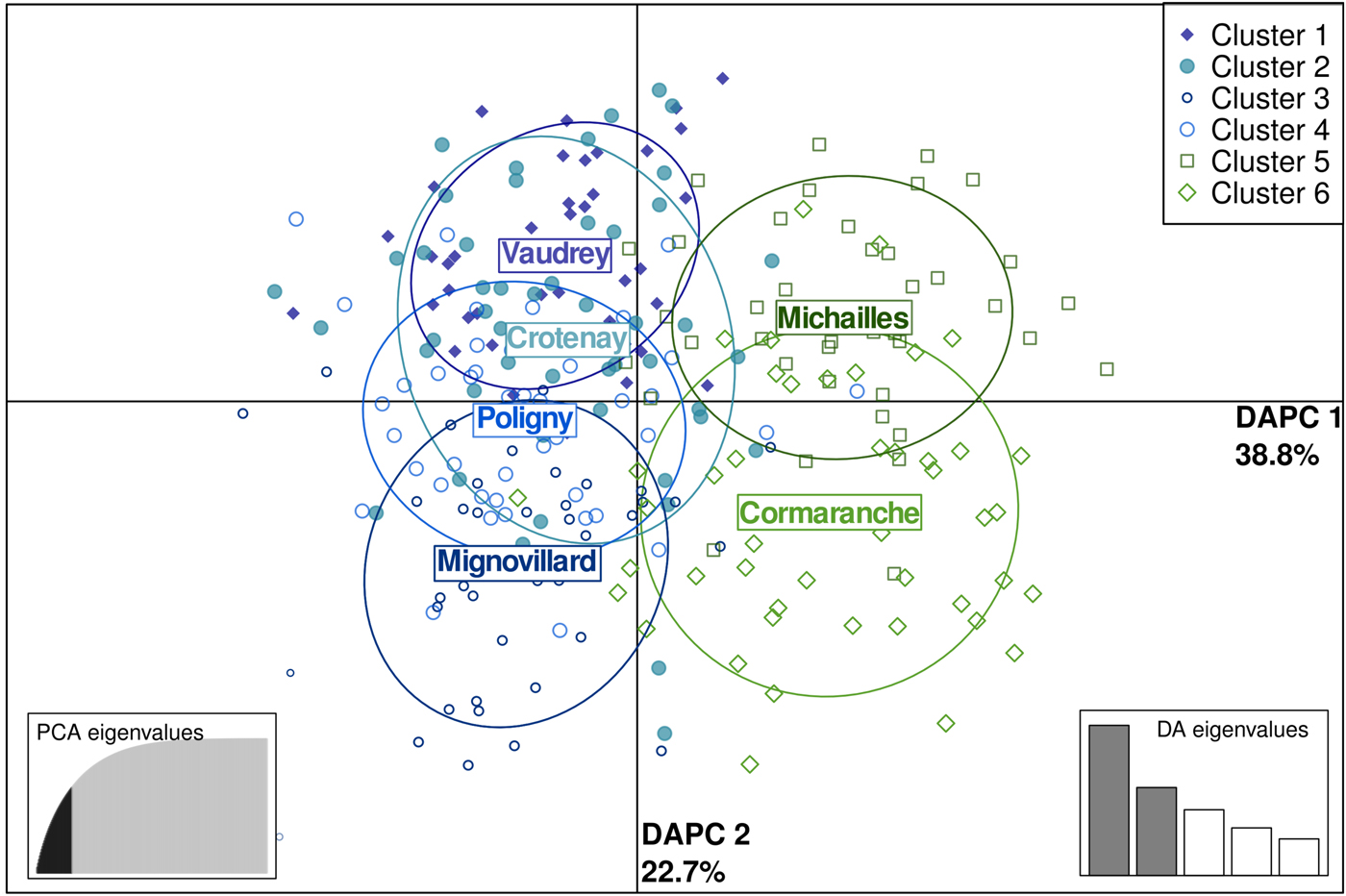
Fig. 2. Scatterplot of the first two principal components discriminating M. glareolus populations by sites using a discriminant analysis of principal components (DAPC) based on microsatellite data. Points represent individual observations. Envelopes represent population membership. Bank vole populations from Ain (NE non-endemic region) are represented in green and those from Jura (NE endemic region) in blue.
Table 2. F st estimates between sites per year (lower side) and associated P values of Fisher exact tests (upper side).

***P ⩽ 0·001.
Table 3. Results of the analysis of molecular variance (AMOVA)

The AMOVA hierarchy was based on geographical features, comparing the two regions (Ain and Jura) and the sites within the regions.
Table 4. Isolation by distance characteristics – probability (IBD p), intercept and slope calculated for each region and for the whole dataset.

95% confidence intervals (CI) for the slope of the IBD were obtained by bootstrapping over the 14 microsatellite loci.
Variations of serological, virological and immunological features in natural populations
A total of 277 bank voles were trapped in the six sites surveyed (Table 1). Among them, 12 captured in Jura were PUUV seropositive. Two of these individuals had very low OD and an absence of viral RNA in the lungs (the target organ, Gavrilovskaya et al. Reference Gavrilovskaya, Chumakov, Apekina, Ryltseva, Martiyanova, Gorbachkova, Bernshtein, Zakharova and Boiko1983). Moreover, they had a low body mass, so that we thought they were juveniles carrying maternal antibodies and not PUUV infected individuals (weight lower than 17·5 g, see Voutilainen et al. Reference Voutilainen, Kallio, Niemimaa, Vapalahti and Henttonen2016). They were therefore excluded from further analyses. Bank voles captured in Ain were all PUUV seronegative. Seroprevalence levels in Jura sites ranged between 7·1 and 10·0%, except in Mignovillard where no PUUV seropositive bank vole was detected. The best model that explained bank vole serological status only included weight (X 2 1 = 5·541, P = 0·019). Heavier bank voles were more likely to be PUUV seropositive.
The serum, lung, liver and urine of the 10 adult seropositive individuals were tested using qRT-PCR. Three sera were PUUV RNA negative and the seven other samples showed viral RNA ranging between 1·01e4 and 1·58e5 copies mg−1. The viral RNA load in the lung and liver ranged respectively, between 7·50e6 and 4·88e9 copies mg−1, and 1·79e6 and 8·63e8 copies mg−1 (Supplementary Table S2). Urine samples could be collected for only five of these 10 adult bank voles. Two of these excreta were PUUV positive as their quantitative RT-PCR cycle thresholds, C T, were lower than 35 cycles. The viral load ranged between 2·08e4 and 2·26e4 copies mg−1.
Extreme values of gene expression levels were detected for the three candidate genes (60% higher than the other values). The models were run with or without these extreme values. Mx2 gene model revealed a significant difference between regions (without outliers: X 2 1 = 4·56, P = 0·033; with outliers: X 2 1 = 6·00, P = 0·01 Fig. 3A). Bank voles from Ain over-expressed Mx2 compared with those from Jura. Tlr7 gene model included the variable sex (without outliers: X 2 1 = 13·67, P = 2 × 10−4; with outliers X 2 1 = 7·80, P = 0·005, Fig. 3B), with higher values of Tlr7 expression detected in females than in males. When including outliers, Tnf-α gene model revealed a significant effect of region, weight (X 2 1 = 9·173, P = 0·002; X 2 1 = 4·474, P = 0·034; respectively, Fig. 3 C and D) and of the interaction sex × region (X 2 1 = 5·783, P = 0·016). Tnf-α expression was higher in bank voles from Ain than those from Jura. This effect was stronger when considering males only. These results have to be taken cautiously as they turned to be non-significant when the outliers were not included in the model. Then we only found a significant effect of weight (X 2 1 = 11·50, P = 6 × 10−4), with heavier bank voles exhibiting higher levels of Tnf-α expression than lighter ones.

Fig. 3. Variations of immunological characteristics in natural bank vole populations. (A) Variations of Mx2 gene expression between individuals of the two regions, Ain (NE non-endemic region) and Jura (NE endemic region). (B) Variations of Tlr7 gene expression between males and females. (C) and (D) Variations of Tnf- α gene expression between the two regions and between sex within the two regions.
Variations of serological and virological features during experimental infections
Three bank voles died before the end of the experiment and were not considered in the analyses. Among the 16 remaining bank voles experimentally infected with PUUV (eight from Ain and 8 from Jura), 14 seroconverted by the end of the study. The two bank voles that did not seroconvert at the end of the experiment (55 dpi) originated from Ain (Supplementary Table S3). Considering bank voles that seroconverted, our model showed a lower OD450 nm in voles from Ain than those from Jura (X 2 1 = 4·126, P = 0·042, see Fig. 4) and a significant variation of OD450 nm through time (X 2 1 = 195·129, P = 2·10−16).

Fig. 4. Variation of the optical density in ELISA test (OD450 nm) over time for the 14 bank voles that seroconverted during the experimental infections. Barplots and error bars represent the mean OD450 nm value ± s.d. for all the individuals in each region. The hatched line represents the threshold above which the bank voles were considered to be PUUV seropositive.
Viral RNA was detected by qRT-PCR in the sera of a single bank vole from Ain at 14 and 20 dpi. Nested RT-PCR allowed detecting PUUV RNA at 8 dpi in the sera of two bank voles from Ain and of four bank voles from Jura (Supplementary Table S4). In addition, PUUV RNA could not be detected in any feces or saliva samples tested using qRT-PCR or nested RT-PCR.
At the end of the experiment (55 dpi), viral RNA was detected by qRT-PCR in the lungs of 13 bank voles (including one that did not seroconvert) over the 16 infected during the experiment. The relative amount of PUUV RNA ranged between 4·34e5 and 4·07e7 RNA copies mg−1 (Supplementary Table S5). The statistical model revealed that PUUV viral load in lungs did not vary between the two regions. The amounts of viral RNA detected in the other organs were lower and concerned less individuals, in particular in liver: only four individuals showed positive results, and less than 3·07e6 RNA copies mg−1 were detected (Supplementary Table S5). Finally, no viral RNA could be detected in saliva or urine samples (the latter being collected only at 55 dpi), and only two bank voles (one from each region) had PUUV RNA in their feces (Supplementary Table S5).
Finally, our results revealed a strong inter-individual variability in the serological–virological relationships. All bank voles but one that seroconverted had at least one organ/excreta with viral RNA at 55 dpi. Moreover, one bank vole that had not seroconverted at 55 dpi exhibited viral RNA in its lungs and kidneys (Supplementary Table S5).
DISCUSSION
Contrasted PUUV epidemiological situations between the French regions Ain and Jura
To our knowledge, this is the first bank vole serological study ever conducted in the French Ain region with regard to PUUV. It is probably because no NE case had been reported there yet (National Reference Center for Hantaviruses) and PUUV studies remained focused on endemic areas. Deepening our understanding of infectious disease emergence requires to broaden our research out of the areas where diseases are well described, to better identify the factors limiting or facilitating emergence success (e.g. for plague in Gascuel et al. Reference Gascuel, Choisy, Duplantier, Debarre and Brouat2013; Tollenaere et al. Reference Tollenaere, Jacquet, Ivanova, Loiseau, Duplantier, Streiff and Brouat2013). None of the 90 bank voles captured in Ain during this field survey was seropositive for PUUV antibodies. This result tends to confirm the absence of PUUV circulation in these bank vole populations, or at such a low level that it cannot be detected using ecological surveys. An extensive survey in more sites or in different years could still be performed to assert more firmly the absence of PUUV circulation in bank vole populations settled south from Jura. In the opposite, and as expected from the existence of known NE cases, we found PUUV seropositive bank voles in all but one site from Jura. Seroprevalence levels were very similar to those observed in the Ardennes epidemic region or in previous studies conducted in Jura (ranging from 0 to 18%, Deter et al. Reference Deter, Chaval, Galan, Gauffre, Morand, Henttonen, Laakkonen, Voutilainen, Charbonnel and Cosson2008a ; Guivier et al. Reference Guivier, Galan, Chaval, Xuereb, Ribas Salvador, Poulle, Charbonnel and Cosson2011). Although no bank vole from Mignovillard forest had anti-PUUV antibodies, we know from previous studies that PUUV may circulate in this site (Deter et al. Reference Deter, Chaval, Galan, Gauffre, Morand, Henttonen, Laakkonen, Voutilainen, Charbonnel and Cosson2008a ). The existence of these two adjacent regions with contrasted epidemiological situations with regard to PUUV reinforced the need to better assess the risk of PUUV emergence and persistence in Ain. It emphasized the necessity to question whether PUUV infected bank voles from Jura could migrate to Ain, thus enhancing the chance for PUUV to be introduced there, and whether PUUV could persist in bank vole populations from Ain once introduced.
High bank vole gene flow between the two French regions Ain and Jura
We developed a population genetic approach to evaluate the possibility that a spatial barrier could prevent bank vole gene flow from Jura to Ain, what would limit the chance of PUUV introduction in this latter region through the dispersal of infected animals. Indeed PUUV is transmitted directly between bank voles, from contaminated excreta or through bites, without any vector (Korpela and Lähdevirta, Reference Korpela and Lähdevirta1978; Yanagihara et al. Reference Yanagihara, Amyx and Gajdusek1985). PUUV introduction from one site to another may therefore strongly result from the introduction of an infected bank vole, either through wood transport or natural dispersal. For example, Guivier et al. (Reference Guivier, Galan, Chaval, Xuereb, Ribas Salvador, Poulle, Charbonnel and Cosson2011) showed that despite frequent local extinction of PUUV in hedge networks, the virus was frequently re-introduced in these habitats through the emigration of seropositive bank voles coming from close forests.
Our results revealed weak spatial genetic structure between sites and regions, with levels of genetic differentiation ranging between 1 and 3%. These estimates are very similar to the levels of genetic differentiation observed in previous studies conducted at the same geographical scale on bank voles using the same genetic markers (Guivier et al. Reference Guivier, Galan, Chaval, Xuereb, Ribas Salvador, Poulle, Charbonnel and Cosson2011) or on other rodent species (Arvicola scherman, Berthier et al. Reference Berthier, Galan, Foltete, Charbonnel and Cosson2005). These results revealed high population size and/or high gene flow between populations (Wright, Reference Wright1951). We did not find any signature of disrupted gene flow between bank voles from the two regions. The spatial structure observed was mainly due to isolation by distance, i.e. migration rates being inversely proportional to the geographic distance between populations. This pattern is also frequently observed in micromammals, including rodents, when studied at this geographical scale (Aars et al. Reference Aars, Dallas, Piertney, Marshall, Gow, Telfer and Lambin2006; Berthier et al. Reference Berthier, Charbonnel, Galan, Chaval and Cosson2006; Bryja et al. Reference Bryja, Charbonnel, Berthier, Galan and Cosson2007). The fact that the rates of isolation by distance did not differ within region and over the whole dataset, corroborated the hypothesis of an absence of spatial barrier disrupting gene flow between Jura and Ain. As such, the possibility for PUUV infected bank voles to disperse from Jura to Ain, and consequently, the possibility for PUUV introduction in Ain seemed likely.
Considering this possibility, the reason why PUUV, which is known to circulate in Jura since the early 2000s, has not reached the Ain NE-free region needs to be questioned. It could be explained by the low progression of PUUV from northern to southern populations, and the emergence of NE in Ain would only be a question of time. It could also be due to differences in metapopulation functioning between bank vole populations in Ain and Jura. Higher genetic drift, lower migration rates between populations could account for lower probability of PUUV persistence in Ain (Guivier et al. Reference Guivier, Galan, Chaval, Xuereb, Ribas Salvador, Poulle, Charbonnel and Cosson2011). However, we did not find any evidence supporting this hypothesis based on the population genetic analyses conducted using microsatellites. We therefore explored the possibility that bank voles from Ain and Jura could present different levels of sensitivity to PUUV infections that would in turn lead to contrasted PUUV epidemiology in these two regions.
High levels of immunoheterogeneity between bank voles
The assessment of immunological variations in wild populations has recently been at the core of eco-epidemiological studies, enabling the analysis of their environmental and evolutionary causes as well as the prediction of their epidemiological consequences (Jackson et al. Reference Jackson, Begon, Birtles, Paterson, Friberg, Hall, Ralli, Turner, Zawadzka and Bradley2011). Here we described strong individual variability of bank vole immune responses from both natural population survey and experimental infections. In the wild, susceptibility, defined here as the probability of being infected with PUUV, strongly depended on the weight of bank voles, which is a proxy for age and/or body condition. Heavier/older bank voles are likely to disperse more than younger ones and have accumulated more opportunities to be exposed to PUUV (see for details Voutilainen et al. Reference Voutilainen, Kallio, Niemimaa, Vapalahti and Henttonen2016). In addition, these heavier/older bank voles could also suffer from immunosuppression due to high levels of corticosterone associated with breeding or to decreased body condition (Beldomenico et al. Reference Beldomenico, Telfer, Gebert, Lukomski, Bennett and Begon2008). Moreover, we revealed variable amounts of viral RNA between bank voles, for all organs and excreta considered, which may result from differences in the time since infection or individual characteristics. The low number of animals that could be handled in highly secured animal facilities (ABSL3) precluded testing the impact of individual factors on the probability of getting infected and on their specific sensitivity. Nevertheless, the experiments conducted allowed controlling for PUUV strain or quantity injected, and some variability was still detected both in anti-PUUV antibody production (e.g. 2 out of 16 bank voles did not seroconvert 55 days post-infection) and in the amount of viral RNA detected in organs and excreta. It was therefore relevant to analyse whether immune responsiveness also differed between bank voles. Considering three immune related candidate genes, namely Tlr7, Mx2 and Tnf-α, we found that sex was an important factor mediating inter-individual immunoheterogeneity. In the wild, females over-expressed Tlr7 gene compared with males. These observations corroborated common patterns observed in vertebrates showing that females are more immunocompetent than males (Klein and Flanagan, Reference Klein and Flanagan2016). Indeed, TLR7 is involved in the recognition of diverse viruses (Heil et al. Reference Heil, Hemmi, Hochrein, Ampenberger, Kirschning, Akira, Lipford, Wagner and Bauer2004; Lund et al. Reference Lund, Alexopoulou, Sato, Karow, Adams, Gale, Iwasaki and Flavell2004) and impaired expression and signalling by TLR7 may contribute to reduced innate immune responses during chronic viral infections (Hirsch et al. Reference Hirsch, Caux, Hasan, Bendriss-Vermare and Olive2010). The sexual dimorphism in Tlr7 gene expression is observed in bank voles for the first time. This gene is linked to the X chromosome, and it belongs to the 15% of X-linked genes escaping inactivation and being expressed from both the active and inactive X chromosome (Plath et al. Reference Plath, Mlynarczyk-Evans, Nusinow and Panning2002). This could explain the over-expression of Tlr7 gene observed in females compared with males. Surprisingly, we did not find any evidence of higher levels of Tnf-α gene expression in female compared with male bank voles, as was previously detected by Guivier et al. (Reference Guivier, Galan, Henttonen, Cosson and Charbonnel2014). Higher expression levels were even detected in males compared with females in the region Ain. This pattern was driven by three bank voles with extreme values that might correspond to recently infected males, and could blur the general picture observed in the Ardennes (Guivier et al. Reference Guivier, Galan, Henttonen, Cosson and Charbonnel2014). Altogether, this high inter-individual immunoheterogeneity, which was observed in the wild and confirmed when performing experimental infections, is important with regard to PUUV epidemiology as it highlighted bank voles with increased likelihood of being infected, excreting and further transmitting the virus (e.g. super-spreaders, Lloyd-Smith et al. Reference Lloyd-Smith, Cross, Briggs, Daugherty, Getz, Latto, Sanchez, Smith and Swei2005).
Regional bank vole immunoheterogeneity – an explanation for the contrasted PUUV epidemiology observed in adjacent French regions?
Evidence of immunoheterogeneity was found between Jura and Ain, two regions with contrasted NE epidemiological statuses. It concerned several immune pathways, including the antiviral and adaptative immune responses of bank voles to PUUV infections. Bank voles from Ain mounted lower levels of anti-PUUV antibodies than those from Jura during PUUV experimental infections. Unfortunately, this regional pattern could hardly be interpreted in terms of rodent sensitivity to PUUV as the role of IgG during hantavirus infections remains unclear (review in Schonrich et al. Reference Schonrich, Rang, Lutteke, Raftery, Charbonnel and Ulrich2008). Indeed, the presence of IgG can protect rodents from subsequent challenges but does not participate in eliminating the virus (review in Easterbrook and Klein, Reference Easterbrook and Klein2008; Schountz and Prescott, Reference Schountz and Prescott2014). The similar amounts of viral RNA observed in bank voles from Ain and Jura at the end of the experiment could support the hypothesis that sensitivity to PUUV did not differ between these two regions. However, this result has to be taken cautiously as it is likely that variability in virus replication and excretion might only be visible sooner after the infection (Hardestam et al. Reference Hardestam, Karlsson, Falk, Olsson, Klingström and Lundkvist2008; Dubois et al. Reference Dubois, Castel, Murri, Pulido, Pons, Benoit, Loiseau, Lakhdar, Galan, Charbonnel and Marianneau2017a ). Because we observed more bank voles from Jura than Ain with PUUV RNA in their sera 8 days after the infection, we cannot refute the possibility of a regional heterogeneity in sensitivity to PUUV.
The immune-related gene expression analyses conducted using wild bank voles also provide arguments in favour of this hypothesis. Rodents from Ain over-expressed Mx2 and Tnf-α genes compared with bank voles from Jura. These results are congruent with previous studies conducted in the French Ardennes and over Europe (Guivier et al. Reference Guivier, Galan, Ribas Salvador, Xuéreb, Chaval, Olsson, Essbauer, Henttonen, Voutilainen, Cosson and Charbonnel2010; Guivier et al. Reference Guivier, Galan, Henttonen, Cosson and Charbonnel2014). These immune related genes were chosen to represent some of the main pathways underlying bank vole immune responses to PUUV infections. They encode respectively for TNF, a proinflammatory cytokine and Mx2, an antiviral protein, that both limit PUUV replication (Kanerva et al. Reference Kanerva, Melen, Vaheri and Julkunen1996; Temonen et al. Reference Temonen, Mustonen, Helin, Pasternack, Vaheri and Holthofer1996; Jin et al. Reference Jin, Yoshimatsu, Takada, Ogino, Asano, Arikawa and Watanabe2001). Besides, the overproduction of these molecules could induce immunopathologies (Li and Youssoufian, Reference Li and Youssoufian1997; Wenzel et al. Reference Wenzel, Uerlich, Haller, Bieber and Tueting2005; Bradley, Reference Bradley2008) that could drive a balance of resistance/tolerance to PUUV (Guivier et al. Reference Guivier, Galan, Ribas Salvador, Xuéreb, Chaval, Olsson, Essbauer, Henttonen, Voutilainen, Cosson and Charbonnel2010; Guivier et al. Reference Guivier, Galan, Henttonen, Cosson and Charbonnel2014). Altogether these regional differences in bank vole responses to PUUV could reflect potential divergence with respect to resistance and tolerance strategies. These differences may be mediated by a subset of individuals within populations, as suggested by bank voles exhibiting extreme values of immune related gene expression and mostly explaining the significant differences observed. Within population evolution from resistance to tolerance has previously been demonstrated in an unmanaged population of Soay sheep (Hayward et al. Reference Hayward, Nussey, Wilson, Berenos, Pilkington, Watt, Pemberton and Graham2014) and in wild populations of field voles (Jackson et al. Reference Jackson, Hall, Friberg, Ralli, Lowe, Zawadzka, Turner, Stewart, Birtles, Paterson, Bradley and Begon2014). Further assessment of bank vole health and fitness would be required to confirm this hypothesis of tolerant and resistant phenotypes with regard to PUUV infections (Raberg et al. Reference Raberg, Graham and Read2009). It would also be interesting to address the issue of potential physiological trade-offs between inflammatory and antibody-mediated responses (Lee and Klasing, Reference Lee and Klasing2004). The patterns observed in this study could suggest that bank voles from the NE-endemic region would promote an adaptative immunity in response to PUUV infection instead of a more costly and potentially damaging inflammatory response, potentially as a result of co-adaptation between bank vole and PUUV (Easterbrook and Klein, Reference Easterbrook and Klein2008).
Limitations of the study
All these results have to be taken cautiously as immunoheterogeneity could be assessed using a larger array of markers. Transcriptomics approaches could for example be applied, what would contribute to a better description of bank vole immune phenotypes and sensibility to PUUV (Friberg et al. Reference Friberg, Lowe, Ralli, Bradley and Jackson2011; Campbell et al. Reference Campbell, Torres-Perez, Acuna-Retamar and Schountz2015). Comparing results observed from large datasets of individuals sampled in natural populations and from experimental infections could help disentangling the effects of confounding factors from direct influence of PUUV infections. Besides, the difficulties to isolate PUUV strains prevented the possibility to perform such experimental infections using a wild PUUV strain that would not exhibit low pathogenicity due to cell-culture adaptation (Lundkvist et al. Reference Lundkvist, Hukic, Hörling, Gilljam, Nichol and Niklasson1997). Further efforts should be made to isolate wild Jura PUUV strain and replicate this experimental work, in order to provide a better representation of infections that occur in the field.
CONCLUSIONS
This study highlighted the importance of combining natural population surveys and experimental approaches in addressing questions related to immunoheterogeneity and its potential consequences for epidemiological questions. Here the natural population survey enabled to study a large number of animals and to describe inter-individual and inter-population variability in immune responsiveness that may further blur the results obtained from experimental infections. Besides, the experiments enabled to control for the time since exposure to PUUV and to follow immune responses kinetics. The diverse array of eco-evolutionary approaches developed here brought important answers to evaluate the global risk of NE emergence in a NE-free region. Our results supported the possibility for PUUV introduction in the region Ain via the dispersal of PUUV seropositive bank voles from Jura. They also indicated the possibility of PUUV circulation in this NE-free region as experimental infections revealed that bank voles from Ain are sensitive to PUUV. Therefore, NE emergence in this region might only be a question of time. But several arguments also indicated that PUUV persistence might be unlikely. Lower susceptibility to PUUV could account for the absence or low number of PUUV infected bank voles in the Ain region, and also potentially for the weak level of PUUV excretion in the environment. Further investigations are now required to identify the mechanisms underlying bank vole immunoheterogeneity between adjacent NE-free and endemic regions, in particular with regard to abiotic (e.g. climate, resource availability) or biotic conditions (e.g. spatially varying pathogen communities, see for the bank vole Behnke et al. Reference Behnke, Barnard, Bajer, Bray, Dinmore, Frake, Osmond, Race and Sinski2001; Ribas Salvador et al. Reference Ribas Salvador, Guivier, Xuereb, Chaval, Cadet, Poulle, Sironen, Voutilainen, Henttonen, Cosson and Charbonnel2011; Razzauti-Feliu et al. Reference Razzauti-Feliu, Galan, Bernard, Maman, Klopp, Charbonnel, Vayssier-Taussat, Eloit and Cosson2015; Loxton et al. Reference Loxton, Lawton, Stafford and Holland2016). Sociological and human behavioural factors would then be the ultimate step to analyse what limits PUUV transmission between M. glareolus and humans.
SUPPLEMENTARY MATERIAL
The supplementary material for this article can be found at https://doi.org/10.1017/S0031182017001548
ACKNOWLEDGEMENTS
Data used in this work were partly produced through the genotyping and sequencing facilities of ISEM (Institut des Sciences de l'Evolution-Montpellier) and Labex CeMEB (Centre Méditerranéen Environnement Biodiversité).
FINANCIAL SUPPORT
We are grateful to EU grants GOCE-CT-2003- 010284 EDEN and FP7-261504 EDENext that have partially funded this research. A. Dubois PhD was funded by an INRA-EFPA/ANSES fellowship.