INTRODUCTION
Visceral leishmaniasis (VL) is a vector-borne systemic disease, caused by Leishmania protozoans, especially L. donovani and L. infantum (L. chagasi). More than 500 000 VL cases per year are reported worldwide (Desjeux, Reference Desjeux2004). Following promastigote inoculation by sandflies, parasites are passively phagocytosed by competent cells and disseminate through the blood flow towards target organs, i.e. bone marrow, spleen and liver. The main symptoms of VL are anarchic fever, hepatosplenomegaly, substantial weight loss, and finally death if left untreated. Efficient chemotherapy usually leads to a life-long immune response and allows clearance of Leishmania intracellular amastigotes and patient recovery. However, relapse or reactivation episodes frequently occur in seriously immunocompromised patients such as HIV-positive patients (Alvar et al. Reference Alvar, Canavate, Gutierrez-Solar, Jimenez, Laguna, Lopez-Velez, Molina and Moreno1997, Reference Alvar, Aparicio, Aseffa, Den Boer, Canavate, Dedet, Gradoni, Ter Horst, Lopez-Velez and Moreno2008), but also in organ transplant patients (Basset et al. Reference Basset, Faraut, Marty, Dereure, Rosenthal, Mary, Pratlong, Lachaud, Bastien and Dedet2005) and occasionally in other patients who are apparently immunocompetent (Dereure et al. Reference Dereure, Duong Thanh, Lavabre-Bertrand, Cartron, Bastides, Richard-Lenoble and Dedet2003; Gorski et al. Reference Gorski, Collin, Ritmeijer, Keus, Gatluak, Mueller and Davidson2010; Kajaia et al. Reference Kajaia, Morse, Kamkamidze, Butsashvili, Chubabria, Zenaishvili, Kokaia and McNutt2011). Reactivation can occur after several years of residence in a non-endemic area and despite a well conducted therapy. Moreover, after L. infantum (L. chagasi) infection, it is now admitted that a great number of infections can remain asymptomatic and the disease can develop later on (Michel et al. Reference Michel, Pomares, Ferrua and Marty2011).
Leishmania parasites have developed various strategies to escape from the host immune system, which have been extensively reviewed (Zambrano-Villa et al. Reference Zambrano-Villa, Rosales-Borjas, Carrero and Ortiz-Ortiz2002; Antoine et al. Reference Antoine, Prina, Courret and Lang2004; Olivier et al. Reference Olivier, Gregory and Forget2005; Kima, Reference Kima2007; Bogdan, Reference Bogdan2008). Intracellular amastigotes are characterized by having a high resistance against low pH, hydrogen peroxide (H2O2), nitric oxide (NO) and lysosomal enzymes (Mauel, Reference Mauel1996; Miller et al. Reference Miller, McGowan, Gantt, Champion, Novick, Andersen, Bacchi, Yarlett, Britigan and Wilson2000). Then, amastigotes are able to initiate an inappropriate immune response with reduced interleukin (IL-12) that allows parasite survival (Reiner et al. Reference Reiner, Zheng, Wang, Stowring and Locksley1994). Finally, Leishmania parasites can evade the immune response, by invading cell types lacking leishmanicidal effector mechanisms, using them as sanctuary cells where they can persist in a latent stage until their further release (Rittig and Bogdan, Reference Rittig and Bogdan2000). Limited data are available on potential sanctuary cells in deep sites during VL, such as the spleen or the liver.
During VL, Kupffer cells (KC) are target cells for Leishmania in the liver and allow the formation of inflammatory granulomas that lead to parasite clearance (Murray, Reference Murray2001; Kaye et al. Reference Kaye, Svensson, Ato, Maroof, Polley, Stager, Zubairi and Engwerda2004). Hepatocytes which are not professional phagocytic cells, were shown to be permissive to L. donovani in vitro (Gangneux et al. Reference Gangneux, Lemenand, Reinhard, Guiguen, Guguen-Guillouzo and Gripon2005). Hepatic stellate cells (HSC) are another important cell type in the liver. They are localized in the Disse's spaces and account for approximately one third of the non-parenchymal cell population (Friedman, Reference Friedman2008b). HSC are at a quiescent stage in the healthy liver but can become activated in the setting of chronic hepatic inflammation. Once activated, HSC proliferate and acquire a myofibroblast-like phenotype (Friedman, Reference Friedman2008a). They can secrete pro-fibrotic cytokines such as TGF-β, IL-6 and IL-33 (Gressner et al. Reference Gressner, Weiskirchen, Breitkopf and Dooley2002; Thirunavukkarasu et al. Reference Thirunavukkarasu, Watkins and Gandhi2006; Marvie et al. Reference Marvie, Lisbonne, L'Helgoualc'h, Rauch, Turlin, Preisser, Bourd-Boittin, Theret, Gascan, Piquet-Pellorce and Samson2010), and contribute to hepatic fibrosis through production of collagen fibres and structural glycoproteins (Tsukada et al. Reference Tsukada, Parsons and Rippe2006), and inhibition of matrix degradation (Arthur, Reference Arthur2000). Interestingly, an increase of the number of HSC, the deposition of collagen fibres and the enlargement of the Disse's spaces were demonstrateded in 35 out of 47 biopsies or necropsies from VL patients (Duarte and Corbett, Reference Duarte and Corbett1987).Whereas skin and lymph node fibroblasts have been shown to be permissive to L. major, no data are available about the interaction of HSC and Leishmania donovani. Skin fibroblasts were proposed to be safe targets for L. major during cutaneous leishmaniasis (CL), which could explain life-long parasite persistence and patient relapses after efficient chemotherapy (Bogdan et al. Reference Bogdan, Donhauser, Doring, Rollinghoff, Diefenbach and Rittig2000). In this work, using human HSC in primary culture infected with L. donovani, we analysed the complex interplay between this particular cell type and L. donovani. We showed that quiescent, as well as activated HSC, are permissive to L. donovani, with no apparent impact on HSC functional characteristics following the cell exposure to parasites. Conversely, HSC had no impact on intracellular parasite survival and infection capability, indicating that these cells could be another sanctuary cell type for the parasite.
MATERIALS AND METHODS
Parasite culture
Leishmania donovani strain MHOM/SD/97/LEM3427 Zym MON-18 was isolated from a patient with VL and cryopreserved at the WHO collaborative centre of Montpellier (French Reference Center for Leishmania). This strain is regularly passed in mouse to recover promastigotes from the amastigotes of the spleen or the liver, and then it is grown in vitro on Novy-McNeal-Nicolle (NNN) blood Agar. Prior to infection, amplification of promastigotes was carried out by culture in Schneider's Drosophila medium (Gibco, Invitrogen) supplemented with 10% FCS, penicillin 200 IU/ml and streptomycin 200 μg/m for 6 days, until they reached the infective stationary phase. The infectivity of L. donovani promastigotes was assessed by efficient infection of THP-1 driven macrophages and mice.
Cell culture
Human HSC were isolated as previously described from histologically normal biopsy specimens after partial hepatectomy in adult patients from both sexes undergoing hepatic resection for liver metastases (Le Pabic et al. Reference Le Pabic, L'Helgoualc'h, Coutant, Wewer, Baffet, Clement and Theret2005; Bourd-Boittin et al. Reference Bourd-Boittin, Le Pabic, Bonnier, L'Helgoualc'h and Theret2008; Marvie et al. Reference Marvie, Lisbonne, L'Helgoualc'h, Rauch, Turlin, Preisser, Bourd-Boittin, Theret, Gascan, Piquet-Pellorce and Samson2010). Access to the biopsy material was in agreement with French laws and satisfied the requirements of the Ethics Committee of the institution. Dissociation of the liver was performed by perfusion with a pronase and collagenase solution by the company Biopredic International (Rennes, France). HSC purification was performed from the first supernatant of liver perfusion enriched in non-parenchymal cells after hepatocyte isolation. It relies on particular properties of HSC naturally rich in vitamin-A, which allow their purification by a single-step density-gradient centrifugation with Nycodenz (Sigma-Aldrich®). For this, the supernatant was centrifuged and the pellet was re-suspended with 7 ml of final volume of cold Gey's balanced salt solution (GBSS), and added with 5 ml of calcium-free GBSS Nycodenz 29%. After mixing of the tube, 1 ml of calcium-free GBSS BSA 0·3% was gently placed on the cell suspension surface. The tube was then centrifuged for 15 min at 1400 g, to allow HSC collection at the interface between Nycodenz and BSA, whereas other residual cell types fell to the bottom of the tube. Finally, HSC were washed once in GBSS and seeded on tissue-culture dishes treated by vacuum gas plasma (BD Falcon™ , USA) in DMEM GlutaMAX™ containing glucose 1 g/l and completed with 10% FCS, penicillin 200 IU/ml and streptomycin 200 μg/ml. HSC primary cultures were maintained at 37 °C with 5% CO2 with a passage every 5–10 days. Purification and culture on plastic dishes is known to spontaneously initiate HSC activation (Friedman, Reference Friedman2008b), so that quiescent HSC are only available for a few days. HSC purity was assessed using a Cy3 mouse monoclonal antibody that specifically targets human α-Smooth Muscle Actin (αSMA) (Sigma-Aldrich®). Labelling of the HSC cytoskeleton showed that activated HSC obtained after the first passage and maintained until passage 8 were 100% αSMA positive.
THP-1 macrophage cells were propagated in RPMI medium containing 10% FCS, penicillin 200 IU/ml and streptomycin 200 μg/ml.
Cell infection
Freshly purified quiescent HSC were seeded the day before their exposure to L. donovani. Activated HSC were seeded in similar conditions. Prior to infection, THP-1 cells were plated in the bottom of culture wells in RPMI supplemented with 2 μM phorbol 12-myristate-13-acetate (Sigma-Aldrich®). In all cases, the medium was removed after overnight exposure to L. donovani (ratio cell/parasites: 1/10) and the wells were thoroughly washed to remove free promastigotes. At the end-point of each experiment, cells were stained with May-Grünwald Giemsa (MGG). The percentage of infected cells and the mean number of parasites per infected cell were recorded after microscopic examination of at least 100 cells per condition with a Zeiss Primostar microscope (magnification:1000X). Pictures were obtained with a Nikon 80i optical microscope (magnification: 600X) equipped with a numerical camera. Each experiment was repeated 3–4 times.
Fluorescent staining and confocal microscopy
Approximately 107L. donovani promastigotes were labelled with 2 μM of PKH-26 (Sigma-Aldrich®) or carboxy-fluorescein diacetate succinimidyl Ester (CFSE) (Invitrogen) during 5 min following the manufacturer's instructions. The labelled promastigotes were incubated overnight with activated HSC, directly seeded on a FlexiPERM® glass slide (Sigma-Aldrich®) (ratio cell/parasites: 1/10). The slide was vigorously washed 3 times in PBS, fixed with PBS4% formaldehyde, washed and dried. Cells were permeabilized using PBS and 0·5% Triton X-100. Then, we used the fluorescent membrane and Golgi apparatus marker AlexaFluor488-labelled wheat germ agglutinin (WGA) (Molecular Probe®, Invitrogen) or a Cy3 mouse monoclonal antibody anti-human αSMA (Sigma-Aldrich) to highlight HSC shapes, in the presence of a 100-fold excess of mouse immunoglobulins to avoid non-specific background. The cell and parasite nuclei were stained with DRAQ5 (Alexis® Biochemicals, Enzo Life Sciences). Cell slides were submitted to confocal analysis in 200 nm thick planes using a Leica TCS SP5 microscope (Leica Microsystems). Images were processed using LAS-AF software (Leica Microsystems) and images analyses were performed using ImageJ software.
HSC viability test
The viability of HSC exposed to L. donovani promastigotes was assessed by a methylene blue colourimetric assay as previously described (Meurette et al. Reference Meurette, Lefeuvre-Orfila, Rebillard, Lagadic-Gossmann and Dimanche-Boitrel2005). Briefly, activated HSC were seeded in 96-well flat-bottomed plates at a density of 105 cells per well and exposed overnight to L. donovani promastigotes at different cell/parasites ratios (1/5, 1/10 or 1/20). Cells were then thoroughly washed to remove promastigotes and maintained during 1, 3 or 7 days. At each endpoint, cells were washed twice with PBS, and fixed with absolute ethanol for 30 min. Following removal of ethanol, fixed cells were dried and stained for 5 min in methylene blue. After 3 washes with tap water, 100 μl of 0·1 M HCl per well were added. Plates were analysed using a spectrophotometer (VersaMAX™, Molecular devices) at 620 nm. The percentage of viable cells was calculated by the following formula: (optical density values of HSC exposed to L. donovani/optical density values of control HSC) × 100. HSC treated overnight with H2O2 30% or staurosporine 1 μM in complete DMEM medium were used as the cell death positive control. Statistical analysis was performed using the Mann-Whitney test to compare the viability of HSC exposed to parasites or toxic treatments compared to control uninfected and untreated HSC at each endpoint. A P-value <0·05 was considered significant and annotated *.
Long-term follow-up of infected HSC
Approximately 105 HSC from 3 different donor patients were exposed to L. donovani promastigotes (ratio cell/parasites: 1/10). The following day, the medium was changed and cells were washed with Ca2+- and Mg2+-free PBS to remove free promastigotes. The 3 cell cultures were maintained in parallel with their non-infected controls during 40 days. The medium was changed every 2 or 3 days and a passage with cell counting was performed every 10 days to quantify cell growth. A detailed observation under an inverted microscope showed the absence of free residual promastigotes in the medium before the first passage. After the fourth passage, cells were centrifuged. The cell pellet obtained from each culture was seeded in NNN blood agar, a culture medium usually used for parasite isolation from human specimens, which favours the propagation of amastigotes and their differentiation into mobile promastigotes. After several passages on NNN medium, promastigotes were recovered and amplified in Schneider's Drosophila medium and used to infect THP-1 cells as described above (Fig. 1).

Fig. 1. Long-term monitoring of hepatic stellate cells (HSC) after overnight exposure to Leishmania donovani. HSC from 3 different patients were infected with L. donovani promastigotes. The following day, the medium was changed and cells were washed to remove free promastigotes. The 3 cell cultures were maintained in parallel with their non-infected controls until 40 days post-infection (D40), with a medium change every 2 or 3 days and a passage and total cell count performed every 10 days. At D40, the cells were centrifuged. The pellet obtained from each culture was seeded in NNN blood agar in order to reactivate intracellular parasites. After 1 month, the mobile promastigotes obtained were amplified in Schneider's Drosophila medium and used to infect THP-1 cells for 1, 2 or 3 days, in parallel to control promastigotes from the initial culture. Infection rates were quantified after MGG colouration.
Quantification of gene expression by real-time quantitative PCR (qPCR)
Total cellular RNA was extracted and purified using a NucleoSpin®RNAII Kit (Macherey-Nagel) and reverse transcribed with a High Capacity cDNA Reverse transcription kit (Applied Biosystems) according to the manufacturer's instructions. Real-time qPCR was performed in 96-well optical plates with Power SYBR®green PCR MasterMix (Applied Biosystems), 300 nM primers and cDNA corresponding to 30 ng of total RNA input in a final volume of 20 μl using the 7300 sequence detection system ABIPrism (Applied Biosystems). Each sample was tested in duplicate in at least 3 independent experiments, to analyse different genes using primers whose sequences are presented in Table 1. The 18S mRNA level was used for normalization of gene expression and induction was calculated by comparison to a 1-day culture of non-infected HSC. The use of 18S as a housekeeping gene for data normalization was validated using SDHA and GAPDH as other control genes (data not shown). A Mann-Whitney statistical analysis was performed to compare the gene expression between HSC exposed to parasites and control HSC at each endpoint.
Table 1. Primer sequences for qPCR analyses

RESULT
HSC are permissive to Leishmania donovani
Human activated HSC from 4 different patients were plated on slides and infected overnight with L. donovani promastigotes. Then, the medium was removed to eliminate free promastigotes, and the culture was stopped (Day 1) or fresh medium was added for 1 or 2 more day(s) (Day 2 and Day 3). After MGG staining, the presence of intracellular amastigotes was easily recognizable by their 2–5 μm flagellum-free ovoid shape, from the first day after cell exposure to L. donovani (Fig. 2A). The mean percentage of amastigote-carrying cells was 4·6 ± 0·3%, 6·0 ± 1·2%, and 4·3 ± 1·3% at Day 1, Day 2 and Day 3, respectively (Fig. 2B). The mean number of parasites per cell was low and stable during the experiment and reached 1·9 ± 0·5 at Day 1, 1·7 ± 0·5 at Day 2 and 2·1 ± 0·6 at Day 3 (Fig. 2C). It was also unchanged after 1 week (data not shown), suggesting that intracellular Leishmania were not able to significantly proliferate inside activated HSC.
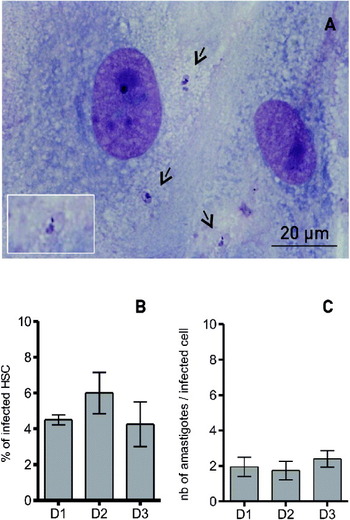
Fig. 2. Analysis of activated hepatic stellate cell (HSC) permissivity to Leishmania donovani using optical microscopy. Activated HSC were infected with L. donovani promastigotes overnight. After a 1-, 2- or 3-day culture, slides were stained with MGG and examined under optical microscopy. (A) Representative slide of infected HSC (magnification: 600X). (B) Percentage of infected cells over time. (C) Mean number of parasites per infected cell. Arrows indicate intracellular parasites. Results are expressed as mean±s.e.m. of at least 3 independent experiments.
In order to demonstrate that human HSC are definitely permissive to L. donovani, we performed a confocal microscopy analysis. Activated HSC were exposed overnight to PKH-26- labelled L. donovani promastigotes and analysed by confocal microscopy in a 200 nm focal plane, after a DRAQ5 nucleus staining and an Alexa488 WGA staining (Fig. 3A–D). We observed that Leishmania parasites (red label) were actually inside HSC (green label). To ascertain this observation despite the HSC autofluorescence level, HSC were then infected with promastigotes labelled with CFSE (green label) and stained with a Cy3 antibody that target αSMA (red label) which is a specific HSC activation marker. This second staining protocol confirmed the presence of amastigotes inside the cells (Fig. 3E–H). Moreover, specific labelling also indicated that infected cells were indeed HSC and not potential residual macrophages, since the Cy3 anti-αSMA antibody did not stain the cytoskeleton of human macrophages in primary culture (data not shown).
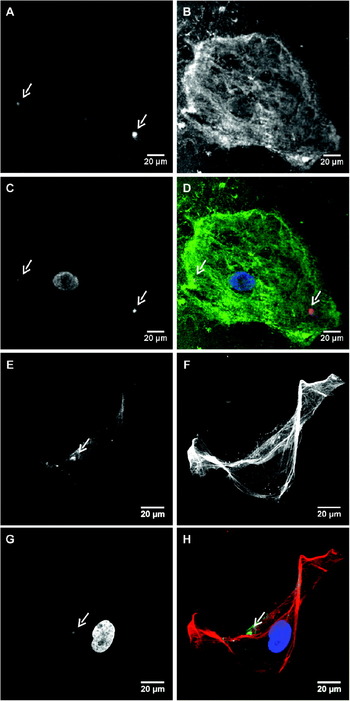
Fig. 3. Analysis of hepatic stellate cell (HSC) permissivity to Leishmania donovani using confocal microscopy. PKH-26 or CFSE labelled promastigotes were used to infect activated HSC. One day after infection, the cell shapes were highlighted using Alexa488 WGA or a Texas red anti-αSMA antibody, respectively. Nuclei were stained using DRAQ5. Examination was performed by confocal microscopy in a 200 nm thick plane. (A) PKH-26 amastigote labelling. (B) Alexa488 WGA cell staining. (C) DRAQ5 nucleus staining. (D) Overlay of PKH-26 (red), Alexa488 WGA (green) and DRAQ5 (blue) labelling. (E) CFSE parasite labelling. (F) Cy3 αSMA labelling. (G) DRAQ5 nucleus staining. (H) Overlay of CFSE (green), Cy3-α-SMA (red) and DRAQ5 (blue) labelling. White arrows show fluorescent parasites.
To assess to what the extent the HSC are in a quiescent state in a normal liver before parasite infection in vivo, we verified whether quiescent human HSC were also permissive to L. donovani (Fig. 4A). Using freshly purified quiescent HSC, we quantified approximately 5% of infected cells after MGG colouration, with no obvious parasite proliferation (data not shown). Interestingly, the presence of intracellular parasites in quiescent HSC does not prevent their progressive activation, which is morphologically characterized by cell spreading with loss of lipidic droplets and cytoskeleton densification the week following their purification (Fig. 4A–C).

Fig. 4. Observation of hepatic stellate cells (HSC) activation after Leishmania donovani infection using optical microscopy. Freshly purified quiescent HSC were infected with L. donovani promastigotes overnight. After a 1-day (A), 3-day (B) or 5-day (C) culture, slides were stained with MGG and examined under optical microscopy (magnification: 600X). Arrows show intracellular parasites.
Leishmania donovani does not affect HSC functional characteristics
In order to determine whether L. donovani can affect HSC viability, a methylene blue assay was performed on activated HSC isolated from 4 liver biopsy donors, at different time-points following overnight exposure to promastigotes and at different cell/parasite ratios. No cell toxicity due to L. donovani was observed during the 7-day period of observation, whatever the cell/parasite ratio, indicating that human activated HSC are able to survive despite cell interactions or infection with L. donovani, whereas control HSC treated with H2O2 or staurosporine displayed approximately 70% mortality (Fig. 5A–C).

Fig. 5. Quantification of viability and proliferation of hepatic stellate cells (HSC) exposed to Leishmania donovani. Human HSC were exposed overnight to L. donovani promastigotes with different cell/parasites ratio (1/5, 1/10 and 1/20). Viability was assessed after 1 day (A), 3 days (B) or 7 days (C) using a methylene blue viability test. Non-infected cells were used as the 100% viability control, and overnight treatments with H2O2 30% or 1 μM staurosporine (StS) were used as positive cell death controls. Results are expressed as mean±s.e.m. of HSC viability from 4 different patients (*P < 0·05). Human activated HSC from 3 different patients were infected overnight with L. donovani promastigotes and maintained in parallel with non-infected cells during 40 days, with a passage and cell count performed every 10 days, as described in Fig. 6. (D) Growth curve of infected and non-infected HSC cultures. Results are expressed as mean±s.e.m.

Fig. 6. Lack of leishmanicidal effects of human hepatic stellate cells (HSC). (A) Induction rates of iNOS mRNA in HSC, compared to non-infected control THP-1. Human activated HSC from 3 donors and THP-1 cells were infected overnight with Leishmania donovani promastigotes. Real-time qPCR analysis of iNOS mRNA expression was performed after 1, 2 or 3 days and normalized with 18S mRNA expression. Results are expressed as mean±s.e.m. (B) Infection rate of THP-1 cells by promastigotes recovered from long-term cultured of human activated HSC transferred on NNN medium. THP-1 cells infected with promastigotes from the initial culture were used as controls. Results are expressed as the percentage of infected cells after microscopic examination of slides stained with MGG and are mean±s.e.m. of 3 independent experiments.
Because VL is a chronic disease characterized by life-long parasite persistence, we performed a prolonged follow-up of HSC growth after serial passages during 40 days (Fig. 1). No difference in terms of cellular proliferation was observed between infected and non-infected HSC cultures (Fig. 5D).
During chronic liver injury, HSC become progressively activated, then proliferate and acquire a myofibroblast-like fibrogenic phenotype. To better understand the impact of L. donovani on HSC differentiation and functions, we quantified by real-time qPCR the expression of pro-inflammatory and fibrogenic genes known to be induced in HSC and/or to be involved in the hepatic immune response during VL. No statistically significant difference was observed in the expression of the important pro-inflammatory and/or pro-fibrotic cytokines TGF-β, IL-6, TNF-α, IL-33 and IL-1β, as well as of matrix degradation regulators MMP-2 and TIMP-1 between activated HSC exposed or not to L. donovani (Table 2).
Table 2. Quantification of pro-inflammatory and fibrogenic gene expression of hepatic stellate cells (HSC) after overnight exposure to Leishmania donovani

HSC do not affect L. donovani viability and cell infection capability
In order to determine whether intracellular L. donovani were able to survive in HSC, HSC from 3 different donor patients were exposed overnight to promastigotes and maintained in culture for 40 days. After inoculation in NNN blood agar medium maintained at 27 °C for 4 weeks (Fig. 1), mobile promastigotes in 2 of 3 cultures were observed after 1 and 2 weekly passages, respectively.
To analyse whether HSC could exert phagocytic cell functions, inducible NO synthase (iNOS) expression was analysed by real-time qPCR. A 2-fold lower expression of iNOS was observed in activated HSC compared to plated THP-1. Overnight HSC exposure to L. donovani had no effect on iNOS expression level (Fig. 6A).
Finally, to determine whether HSC can alter the virulence of L. donovani promastigotes collected from long-term cultured HSC, parasites were amplified by subculture in Schneider's Drosophila medium and inoculated onto plated THP-1 cells. The infection rates of THP-1 cells were quantified after 1, 2 or 3 days and compared to that obtained with control promastigotes. The infection rate of THP-1 infected with control promastigotes was 11·2 ± 2·1% at Day 1, 19·1 ± 2·2% at Day 2 and 13·9 ± 0·2% at Day 3, whereas it was 16·4 ± 9% at Day 1, 20·6 ± 3·6% at Day 2 and 19·3 ± 1·2% for THP-1 infected with promastigotes collected from HSC (P = ns) (Fig. 6B)
DISCUSSION
During visceral leishmaniasis (VL), Kupffer cells (KC) are acknowledged as pivotal phagocytic cells in the host defence against Leishmania donovani infection in the liver (Murray, Reference Murray2001), whereas little is known on HSC, which play a major role in the fibrotic process (Friedman, Reference Friedman2008a,Reference Friedmanb) complicating some infectious diseases (Bartley et al. Reference Bartley, Ramm, Jones, Ruddell, Li and McManus2006; Chang et al. Reference Chang, Ramalho, Ramalho, Martinelli and Zucoloto2006; Tomanovic et al. Reference Tomanovic, Boricic, Brasanac, Stojsic, Delic and Brmbolic2009) and commonly observed in human VL (Duarte and Corbett, Reference Duarte and Corbett1987; Corbett et al. Reference Corbett, Duarte and Bustamante1993; el Hag et al. Reference el Hag, Hashim, el Toum, Homeida, el Kalifa and el Hassan1994). Hepatic fibrosis due to HSC activation was shown to be correlated with the progression of VL and to regress after clinical cure of the disease (Corbett et al. Reference Corbett, Duarte and Bustamante1993; Duarte and Corbett, Reference Duarte and Corbett1987). Similarly, canine VL is associated with collagen, fibronectin and laminin deposition in the liver, and there is a correlation between fibrosis and parasite loads or severity of the disease in dogs naturally infected with L. chagasi (Melo et al. Reference Melo, Moura, Ribeiro, Alves, Caliari, Tafuri and Calabrese2009). Despite these clinical observations, the Leishmania-HSC interaction has never been studied.
In this work, we developed an original model of L. donovani infection of HSC in primary culture. For the first time, we provide evidence that human HSC in primary culture are permissive to L. donovani promastigotes using confocal microscopy. In order to bypass the autofluorescence level of HSC (Friedman, Reference Friedman2008b), 2 complementary staining protocols of parasites and cells using different fluorescent dyes were performed. In both cases, Leishmania parasites were localized inside the cells, clearly demonstrating their permissivity to L. donovani, thus raising the question of how the parasite can enter these cells. Several studies have shown that HSC can express surface markers, such as members of the HLA family (MHC-I and MHC-II), lipid presenting molecules (CD1b and CD1c), co-stimulatory proteins (CD80, CD40) and proteins involved in antigen trafficking (CD68). They can otherwise phagocytose latex bead particles, they closely interact with lymphocytes in Disse's spaces, and stimulate T-cell proliferation, suggesting that they share features of antigen presenting cells (Vinas et al. Reference Vinas, Bataller, Sancho-Bru, Gines, Berenguer, Enrich, Nicolas, Ercilla, Gallart, Vives, Arroyo and Rodes2003; Muhanna et al. Reference Muhanna, Horani, Doron and Safadi2007). Therefore, HSC could phagocytose Leishmania promastigotes like professional phagocytic cells.
In our model, the cell infection rate reached 6%, both in activated HSC and in quiescent HSC freshly purified from human liver donors. No effect on activated HSC survival and proliferation was noticed after cell exposure to parasites, showing that contact or infection with promastigotes does not induce cell death or cell cycle disruption. During our study, we analysed the pro-inflammatory and fibrogenic phenotype of activated HSC exposed overnight to the parasite. Unfortunately, it was not possible to analyse separately the mRNA expression of infected cells, because of specific technical limitations: (i) strong and wide-length autofluorescence of HSC limiting phenotype analysis by flow cytometry, and (ii) lack of availability of a cell sorter operating in a negative pressure environment adapted to sorting of living cells infected with L. donovani. Nevertheless, whole HSC exposure to parasites resulting both in a low percentage of infected cells but also in overall contacts and exposure to excreted/secreted antigens even without infection, did not disturb the HSC phenotype. Indeed, the expression of key genes which are the hallmark of HSC activation and hepatic fibrosis, such as TGF-β, IL-6, TNF-α, IL-33, IL-1β, MMP-2 and TIMP-1 (Gressner et al. Reference Gressner, Weiskirchen, Breitkopf and Dooley2002; Friedman, Reference Friedman2008b; Marvie et al. Reference Marvie, Lisbonne, L'Helgoualc'h, Rauch, Turlin, Preisser, Bourd-Boittin, Theret, Gascan, Piquet-Pellorce and Samson2010; Thirunavukkarasu et al. Reference Thirunavukkarasu, Watkins and Gandhi2006), was not modified. Furthermore, the spontaneous activation of quiescent HSC, normally observed after several days of ex vivo culture (Friedman, Reference Friedman2008b) was never altered following infection with L. donovani. These data suggest that the parasite itself has neither the capacity to stimulate HSC fibrogenic phenotype, nor the ability to contribute to the expansion of extracellular matrix and fibrosis through induction of HSC proliferation. Thus, L. donovani itself is unlikely to be directly involved in the hepatic fibrogenesis process during VL, but could induce the onset of sustained cytokine responses favouring long-term fibrosis. Indeed, HSC are indirectly modulated by the liver micro-environment via cell-to-cell interactions or more likely via paracrine or cytokine pathways. During VL, it was shown that hypertrophic KC intensively express TGF-β that activates HSC cells for extracellular matrix production (Duarte et al. Reference Duarte, de Andrade, Takamura, Sesso and Tuon2009). Thus our results spread a new light on the complex interplay between HSC and KC, which needs to be further explored to better understand the regulation of HSC proliferation and its consequences on liver injuries and hepatic fibrosis during VL.
The absence of modification in functional characteristics of infected HSC raises the hypothesis that they could be sanctuary cells for persisting L. donovani parasites. In this work, it was shown that HSC were able to harbour parasites during long-term primary culture (>1 month) and survive without killing parasites. This inability to get rid of Leishmania parasites could be explained by their low iNOS expression level compared to THP-1 cells. Indeed this key enzyme is essential for efficient phagocytic function, and is strongly induced in professional phagocytic cells (Green et al. Reference Green, Nacy and Meltzer1991; James, Reference James1995). This lack of iNOS induction probably contributes to make these cells a safe target for L. donovani. After a 40-day culture and serial passages of HSC, intracellular parasites were able to reactivate in Novy-Nicolle-McNeal (NNN) medium and to convert into promastigotes, for which the infectivity for THP-1 cells was not altered. This observation demonstrates that L. donovani can persist in a latent intracellular stage inside HSC until their further release. The sensitivity to leishmanicidal drugs of these latent intracellular parasites could be an interesting aspect to explore.
Sanctuary cells have been described as immune privileged safe targets for pathogens such as Toxoplasma gondii, Leishmania major or herpes virus, that contribute to life-long pathogen persistence even after efficient chemotherapies (Bogdan, Reference Bogdan2008). During cutaneous leishmaniasis (CL), immature myeloid precursor cells have been described as safe targets for L. major (Mirkovich et al. Reference Mirkovich, Galelli, Allison and Modabber1986), as well as skin and lymph node fibroblasts (Bogdan et al. Reference Bogdan, Donhauser, Doring, Rollinghoff, Diefenbach and Rittig2000). During VL, a few cell types were postulated to function as safe targets, especially sialoadhesin-positive stromal macrophages of the bone marrow (Leclercq et al. Reference Leclercq, Lebastard, Belkaid, Louis and Milon1996; Cotterell et al. Reference Cotterell, Engwerda and Kaye2000) and hepatocytes which show a low permissivity level and limited cell damage (Gangneux et al. Reference Gangneux, Lemenand, Reinhard, Guiguen, Guguen-Guillouzo and Gripon2005). Interestingly, Bogdan and co-workers reported that persisting L. major parasites were largely associated with skin and lymph node fibroblasts lacking leishmanicidal effectors (Bogdan et al. Reference Bogdan, Donhauser, Doring, Rollinghoff, Diefenbach and Rittig2000). Our results suggest that quiescent and activated myofibroblast-like HSC are able to phagocyte viscerotropic Leishmania parasites during VL and to behave in a similar way as fibroblasts during CL. This could be an evading mechanism for Leishmania parasites that may explain patient relapses after a cured VL episode. Furthermore, we observed that HSC were permissive to L. infantum (data not shown), suggesting that this phenomenon could occur whatever the parasite species involved and explain asymptomatic carriage frequently observed in endemic areas (Michel et al. Reference Michel, Pomares, Ferrua and Marty2011). As KCs regulate HSC growth during VL through TGF-β synthesis (Duarte et al. Reference Duarte, de Andrade, Takamura, Sesso and Tuon2009), they could contribute to amplify the number of safe targets cells for Leishmania parasites.
In conclusion, human HSC in our model can harbour persisting L. donovani parasites and could be a sanctuary cell subset in the liver during VL, which opens new insights into the patho-physiology of immune evasion, asymptomatic carriage and clinical relapse after treatment.
ACKNOWLEDGEMENTS
We thank Dr Katia Bourd-Boittin, Dr Sandrine Jouan-Lanhouet, Christelle Manuel, Annie L'Helgoualc'h, Nicolas Vu and Dr Tiphaine Mergey for punctual technical assistance. We thank the Rennes Human Biological Resource Center (CHRU Pontchaillou, Biosit, CNRS UMS 3480, Inserm US 18) and Jacques Le Seyec for contribution to human tissue sampling. We thank Dr Stephanie Dutertre from Biosit platform IBiSA MRic-Photonics for assistance for confocal microscopy. We thank the Biosit Histopathology platform H2P2 of Faculté de Médecine for use of their histology facilities. We thank Cindy Nikolic for her contribution to the artwork of the figures.
FINANCIAL SUPPORT
OR was granted by the ‘Ministere de l'Enseignement Superieur et de la Recherche’. This work was supported by the ‘Institut de Parasitologie de l'Ouest’ and a grant from the CPER Bretagne – 360b 2007-2013/ European funding FEDER.