Roundup Ready® (RR) (Monsanto, St. Louis, MO 63167) soybean was introduced in the United States in 1996, followed shortly thereafter by RR cotton and RR corn, with additional crops (including canola and sugar beet) also being released (Johnson et al. Reference Johnson, Hallett, Legleiter, Whitford, Weller, Bordelon and Lerner2012). However, after glyphosate (N-(phosphonomethyl)glycine) applications over many years and millions of hectares, the widespread evolution of weed populations resistant to glyphosate has occured (Johnson et al. Reference Johnson, Hallett, Legleiter, Whitford, Weller, Bordelon and Lerner2012a). Glyphosate-resistant weeds such as Palmer amaranth (Amaranthus palmeri S. Wats.), horseweed [Conyza canadensis (L.) Cronq.], common ragweed (Ambrosia artemisiifolia L.), and giant ragweed (Ambrosia trifida L.) (Heap Reference Heap2016) are examples of difficult to control weeds that have forced producers to use other means of weed control. In response to the evolution of glyphosate-resistant weeds, companies have been developing new methods of weed control and are searching for new active ingredients and modes of action, but the cost of development and the limited potential for economic return have made it difficult to bring new products to market (Johnson et al. Reference Johnson, Hallett, Legleiter, Whitford, Weller, Bordelon and Lerner2012). Monsanto, Basf, and Dow have been at the forefront of developing genetically engineered crops resistant to herbicides other than glyphosate. The introduction of crops resistant to dicamba and 2,4-D was initiated because these herbicides have shown excellent resilience, with few herbicide-resistant weeds occurring after more than 50 yr of use (Johnson et al. Reference Johnson, Hallett, Legleiter, Whitford, Weller, Bordelon and Lerner2012). Also, 2,4-D and dicamba provide excellent control of glyphosate-resistant broadleaf weeds such as horseweed, giant ragweed, common waterhemp (Amaranthus rudis Sauer), Palmer amaranth, and other broadleaf weeds (Johnson et al. 2012).
The typical plant growth–regulating (PGR) injury symptoms in soybeans can be recognized by the characteristic cupping of leaves following application of dicamba, and injury can range from cosmetic leaf injury to 80% yield loss, depending on the amount of PGR residue left in the tank and the crop growth stage at application (Steckel et al. Reference Steckel, Chism and Thompson2005). Producers determine the extent of crop damage based on injury symptoms and must decide whether to continue with the crop or to replant (Sciumbato et al. Reference Sciumbato, Chandler, Senseman, Bovey and Smith2004). Quantifying the degree of herbicide exposure is helpful in determining the probable outcome as soon as injury is discovered during the growing season (Sciumbato et al. Reference Sciumbato, Chandler, Senseman, Bovey and Smith2004). Soybeans exposed to dicamba can develop vegetative malformations and produce a lower yield; however, the extent of that damage is dependent upon rate and application timing (Andersen et al. Reference Andersen, Clay, Wrage and Matthees2004). Wax et al. (Reference Wax, Knuth and Slife1969) found that soybean is susceptible to dicamba application at both the vegetative and reproductive stages. Injury due to herbicide does not always lead to yield loss (Al-Khatib and Peterson Reference Al-Khatib and Peterson1999); soybean has the ability to recover from early-season injury depending on rate and application timing (Weidenhamer et al. Reference Weidenhamer, Triplett and Sobotka1989).
Reduced soybean yield from dicamba exposure has been reported when dicamba caused severe injury and stunting, while yield reductions greater than 10% coincided with severe soybean injury (Al-Khatib and Peterson Reference Al-Khatib and Peterson1999) such as terminal bud kill, splitting of the stem, swollen petioles, and curled, malformed pods (Weidenhamer et al. Reference Weidenhamer, Triplett and Sobotka1989). Anderson et al. (2004) concluded that spraying soybean with dicamba at the V3 growth stage resulted in at least 40% injury 48 d after treatment (DAT) at a rate of 0.0056 kg ae ha−1 with a 14% yield reduction. Dicamba was also applied at 0.0112 and 0.056 kg ae ha−1, resulting in 13.8 and 71.5% yield reduction, respectively. Kelley and Riechers (Reference Kelley and Riechers2003) found that as little as 1/10,000 of the 280 g ae ha−1 dicamba rate can produce injury symptoms in soybean. Compounding this problem is spray contamination caused by a failure to thoroughly clean a sprayer, which can cause crop injury up to several months after initial use (University of Illinois Extension 2006). Boerboom (Reference Boerboom2004) showed that dicamba residues, even when an ammonia–water solution was used, had a subsequent use rate of 0.024% from the tank and 0.63% from the spray boom when refilled with water.
The introduction of new herbicide-tolerant crops may provide many benefits for producers such as alternative control options for resistant weed species, decreased costs, and different modes of action. Along with these benefits, the use of auxin-containing herbicides may also increase concern for issues such as herbicide drift, volatilization, and spray equipment contamination. The adjuvant and solvent system used in several commercial herbicides often result in the release of herbicides that have been sequestered within the spray system, thus causing injury to sensitive crops. Injury from PGR herbicide tank residue most often occurs to cotton and soybean with the first tank of POST applied herbicide (Steckel et al. Reference Steckel, Chism and Thompson2005). Due to their chemical makeup, several herbicides, most notably Roundup WeatherMax® (glyphosate), are very effective tank cleaners for PGR herbicides (Steckel et al. Reference Steckel, Chism and Thompson2005). Unlike glyphosate, which is very water soluble and can be easily cleaned out of a sprayer with water, the PGR herbicides, although water soluble, act as weak acids, and their removal requires appreciably more time, care, and effort (Steckel et al. Reference Steckel, Chism and Thompson2005). Therefore, the objective of this study was determine whether differences occur in the level of soybean injury, height reduction, yield reduction, and ppmv dicamba analyte retained with respect to the chemical composition of an agricultural hose type and whether water or ammonia differ in their cleaning abilities.
Materials and Methods
Field and Greenhouse Experiments
Field studies were conducted in 2012, 2013, and 2015 to evaluate the sequestration potential of five agricultural hose types and different cleanout procedures while using dicamba. In 2012, a preliminary study was conducted to determine whether the five hose types led to any differences with respect to injury in soybean. After preliminary results indicated differences among hose types, the experiment was replicated and data from the preliminary trial were omitted. In 2013 and 2015, the experiment was conducted at the Black Belt Branch Experiment Station (33.256076° N, 88.553837° W) in Brooksville, MS, on an Okolona silty clay (fine, smectitic, thermic Oxyaquic Hapluderts) with 8% sand, 51% silt, 41% clay, 2% organic matter, and a pH of 6.8; and on a Brooksville silty clay (fine, smectitic, thermic Aquic Hapluderts); and at the R. R. Foil Plant Science Research Center (33.469066° N, 88.760782° W) in Starkville, MS, on a Marietta fine sandy loam (fine-loamy, siliceous, active, thermic Fluvaquentic Eutrudepts) with 71% sand, 17% silt, 13% clay, 1.03% organic matter, and a pH of 5.9. Differences from 2012, 2013, and 2015 involved the addition of an extra cleanout procedure and the addition of a rate titration followed by aqueous sample collection and analytical analysis. Planting date, planting populations, and seed variety varied among locations (Table 1).
Table 1 Planting year, location, date, population, and seed variety information for dicamba hose sequestration trials.

a Asgrow Soybean (Monsanto Agrochemical Company, 800 North Lindbergh Boulevard, St. Louis, MO 63167); Pioneer Soybean (Du Pont de Nemours, 1007 Market Street, Wilmington, DE 19898).
b Same variety used in both greenhouse runs.
Field studies conducted in 2012 and 2013 involved five different types of agricultural spray hoses by two cleanout procedures (water and ammonia). Each hose measured 3 m in length and had an inside diameter of 1.3 cm, which is enough carrying capacity to deliver a sufficient volume to treat a plot-sized area of 2 by 12 m. Hose types included: John Deere PMK 4131-08 (yellow/PVC/high tensile–strength yarn/1 ply), John Deere PMA 4086-08 (blue/linear/low-density polyethylene blend), John Deere PMA 1687-08 (green/PVC/polyurethane/high tensile–strength yarn/2 ply), John Deere PMA 1628-08 (gray/PVC/polyurethane blend/high tensile–strength yarn/2 ply), and a Goodyear hose (black/Versigard synthetic rubber). Each hose end was fitted with a female pneumatic coupling to allow for sequestration of the solution within each hose and to prevent leakage. Field studies in 2015 involved the same hose types previously described and added a cleanout (water, ammonia, and no-cleanout) along with a rate titration of dicamba at 0.56, 0.140, 0.0087, and 0.0022 kg ae ha−1 to use for comparison. Samples were collected from each hose type by cleanout procedure and rate titration. Analysis was performed on high-performance liquid chromatography–mass spectrometry (HPLC-MS).
In 2013 and 2015, herbicide treatments consisted of dicamba (Engenia®, 600 g L−1, BASF, 26 Davis Drive, Research Triangle Park, NC 27709) at 0.56 kg ae ha−1. In all years, glyphosate (Roundup WeatherMax®, 540 g ae L−1, Monsanto, St. Louis, MO 63167) was applied at 1.1 kg ae ha−1.
For soybean analysis, spray lines were filled with dicamba and glyphosate at a rate of 0.56 and 1.1 kg ae ha−1, respectively and left to equilibrate for 48 h. The spray solution was then flushed out of the lines and the hose section cleaned with one of three cleanout procedures: no cleanout or water cleanout or ammonia cleanout at a rate of 11.35 L of water per line to simulate an actual in-field cleanout procedure with the hose then left to equilibrate in its designated cleaning solution for 24 h. For the ammonia cleanout, a 1% v/v solution was used. After 24 h, lines were flushed of the designated cleaning solution and left empty for 48 h. The spray lines were then filled with glyphosate at a rate of 1.1 kg ae ha−1. This solution was then equilibrated for 48 h to aid in the release of any sequestered herbicides before collection. The solution from each hose type by cleanout procedure was then collected using CO2 to push the solution from each hose to a collection bucket. A 10 ml aliquot was taken from each collection container for chemical analysis. The remaining solution was then applied to soybean at the R2 growth stage. Each hose type by cleanout combination was replicated three times; in essence, there was only one hose type per cleanout procedure per replication. Hoses were used for the same treatment from one year to the next throughout the entirety of the study.
Herbicide treatments from hose equilibrated solutions were applied with a CO2-pressurized backpack sprayer equipped with TTI110015 wide-angle, air induction, tapered flat spray tip (TeeJet Technologies, P.O. Box 7900, Wheaton, IL 60187) at an application volume of 140 l ha−1 and a pressure of 220 kPa. Visual estimates of soybean injury were recorded 7, 14, 21, and 28 DAT using a scale of 0 to 100%, where 0=no injury and 100=total plant death. Chlorosis, necrosis, stunting, leaf cupping, epinasty, and plant height reduction as compared with the untreated control were visually evaluated to estimate injury. Plant height and plant height reduction from the check were collected 7, 14, 21, and 28 DAT. Soybean was machine harvested and yield and yield reduction were calculated.
The experiment was arranged as a factorial arrangement of treatments in a randomized complete block with factor A consisting of hose type and factor B consisting of cleanout procedure. The rate titration was averaged separately and used as a comparison. Three replications for each treatment were used in the experiment with a plot-sized area of 2 by 12 m.
Treatments described in the 2015 field studies were also evaluated in the greenhouse in 2014. The trial was replicated in the greenhouse in October and November of 2014. Soybean seeds were planted approximately 2.5 cm deep in 9.8 L plastic pots (RM3R RootMaker Pot, Stuewe and Sons, 2290 SE Kiger Island Drive, Corvallis, OR 97333) containing commercial potting soil mix (Metro-Mix® 360, Sungro Horticulture, 770 Silver Street, Agawam, MA 01001). After planting, plastic containers were surface irrigated with tap water for the duration of the experiment. Plants were thinned to four plants per container within 1 wk of emergence and grown at 35/30 C day/night temperature. Natural light was supplemented with light from sodium vapor lamps (General Electric Sodium Vapor Lamps, Lucalox LU 400, General Electric Consumer and Industrial Lighting, 1975 Noble Road, Nela Park, Cleveland, OH 44112) to provide a 16 h photoperiod.
Approximately 2 wk after thinning, when plants had reached the V3 growth stage, hose cleanout-solution spray treatments were initiated using a compressed-air spray chamber equipped with a single 80015EVS flat-fan nozzle (TeeJet Technologies) at an application volume of 140 L ha−1 and a pressure of 220 kPa. Herbicide treatments consisted of dicamba at 0.56 kg ae ha−1 and glyphosate applied at 1.1 kg ae ha−1. For the greenhouse experiments, all spray lines were filled with dicamba and glyphosate at the same rate and cleaned in the same manner as in the field experiments in 2015. The solution from each hose type by cleanout procedure was then collected using CO2 to push the solution from each hose to a collection bucket for analysis. A 10 ml aliquot was then taken from each collection bucket for chemical analysis. The remaining solution was then added to 355 ml bottles and applied to soybean at the V3 growth stage in the spray chamber.
Visual estimates of soybean injury were recorded 3, 5, 7, and 14 DAT, using a scale of 0 to 100%, where 0=no injury and 100=total plant death. Chlorosis, necrosis, stunting, leaf cupping, epinasty, and regrowth were visually evaluated to estimate injury. Plants were cut at the soil line 21 DAT, dried, and weighed to calculate dry matter and dry matter reduction from the untreated check. Three replications for each treatment were used in the experiment with one pot representing one hose per hose type by cleanout procedure for each replication. Data were pooled across site years because experimental replication was considered a random variable. Untransformed and arcsine square-root-transformed data were subjected to analysis of variance, but interpretations were similar to untransformed data; therefore, untransformed data were used for analysis. Data were analyzed using PROC GLIMMIX in SAS v. 9.4, and means were separated using Fischer’s protected LSD test at P=0.05.
Chemical Analysis
Samples from field and greenhouse studies were collected in 2014 and 2015 in 20 ml liquid scintillation vials (Sigma-Aldrich, 3050 Spruce Street, St. Louis, MO 63103) with a sample volume of 10 ml. Rinsates from field and greenhouse samples were taken at the time of the experiment and frozen for analytical analysis. Samples were collected using a 50 ml silicone pipette filler, three-way valve (Cole-Parmer Instrument Company, 625 East Bunker Court, Vernon Hills, IL 60061) attached to a 10 ml serological, sterile, individually wrapped pipette (Cole-Parmer Instrument Company). Samples were collected with one pipette per sample to eliminate cross contamination.
Dicamba analysis was performed at the University of Tennessee (Knoxville, TN 37996). Instrumentation used in the analysis began with the Agilent 1100 series, which included a quaternary pump, an auto sampler, a thermostated column compartment, and a 6120 quadruple single-quad MS (Agilent Technologies, 5301 Stevens Creek Boulevard, Santa Clara, CA 95051). The liquid phase of the analysis was acetonitrile +0.1% formic acid (70%) and water +0.1% formic acid (30%).
Samples collected from field and greenhouse studies were prepared by vortexing the aliquot solutions (Fisher Vortex Genie 2, Scientific Industries, 80 Orville Drive, Suite 102, Bohemia, NY 11716) for 30 s. A 1 ml aliquot was added to 19 ml of methanol to constitute a 0.05 dilution. For rate titration of dicamba at 0.56 and 0.14 kg ae ha−1, the dilution rate was increased to 0.00063. Dilution of the samples moved the dicamba concentration to within the linear range of the MS instrument by adding 1 ml of the aliquot solution to 19 ml of methanol and then extracting 250 µl of that solution into 19.75 ml of methanol. For the lower end of the rate titration of dicamba at 0.0087 and 0.0022 kg ae ha−1, the dilution rate of 0.05 was maintained. After dilutions were made, a final vortex of the solution was done for 30 s. A 2 ml extraction was made with a BD 10 ml syringe with Luer-LokTM (Becton, Dickinson, 1 Becton Drive Franklin Lakes, NJ 07417-1880) and passed through a 0.45 µm hydrophobic polytetrafluoroethylene (PTFE) membrane filter (09-719H, Thermo Fisher Scientific, 300 Industry Drive, Pittsburgh, PA 15275) directly into a clear glass vial with a polypropylene open top bounded with PTFE/silicone septum (Thermo Fisher Scientific).
The analysis began with an injection of methanol (to verify a lack of background carryover) followed by dicamba standards of 16.5, 30, 300, and 1000 ppb to establish linearity of MS response. A dicamba standard (30 ppb) was analyzed after every four unknown samples to verify consistency of MS detector response over time. The conservative lower limit of detection was 5 ppb, and all samples (with the exception of untreated samples) had dicamba concentrations above this amount. Three replications for each treatment were used in the experiment, with one sample representing one hose per hose type by cleanout procedure for each replication.
Hose Analysis Using Scanning Electron Microscopy (SEM)
For hose analysis using SEM, subsamples of hoses used throughout experiments were derived by randomly selecting hose types used and comparing them to hoses of the same type that were never used and have never had solution within them. The used hoses were used a total of eight times in the previous experiments. Three subsamples were cut from each hose type into 7.6 cm samples using a ratcheting hose and PVC cutting tool (Professional Ratcheting Hose and PVC Cutter 37100, Superior Tool Company, 100 Hayes Drive, Cleveland, OH 44131). Samples were then cut into smaller pieces roughly measuring 6.4 by 2.5 mm. Samples were then randomly chosen and glued to a 25.4 mm pin stub (16144, Ted Pella, 4595 Mountain Lakes Boulevard, Redding, CA 96003) using EPO-TEK® conductive Silver Epoxy and a liquid hardener (Ted Pella H-22) to affix four samples per pin stub with the outside of the hose attached to the stub for analysis of the inner tube. After 24 h the samples were coated; it was necessary to use a platinum coating to create a charge. The platinum coating was applied with an EMS 150T ES Coater (EMS, P.O. Box 550, 1560 Industry Road, Hatfield, PA 19440) using argon gas as the supply. Samples were coated in less than 1 min and left to cure for 24 h.
Samples were then loaded to a Zeiss Evo 60 EP-SEM (Zeiss International, Carl-Zeiss-Strasse 22, 73447 Oberkochen, Germany) connected to a Bruker AXS Quantax 4010 energy dispersive X-ray spectrometer (Bruker Corporation, Permoserstrasse 15, 04318 Leipzig, Germany). The Bruker software was used for graphing the elemental makeup of the sample and for creating a color-coded map of the sample in which different colors pertain to different elements. The Quantax 4010 was equipped with a silicon drift detector that provided a high-resolution and accurate map and/or graph of the sample.
Results and Discussion
Field and Greenhouse Experiments
Experiments averaged over six locations (2013 and 2015 field studies and the 2014 greenhouse studies) showed an interaction of hose type by cleanout procedure with respect to soybean injury at all evaluation dates (Table 2). Visual estimations of injury from 7 and 14 DAT are averaged over six experiments and show the black and green hose rinsates leading to greater injury than other hoses with respect to dicamba sequestration within all cleanouts. At 7 DAT, the black and green hose rinsates showed approximately 20% injury in the no-cleanout treatment (P≤0.0001), 13% in the ammonia treatment (P≤0.0001), and 13% in the water treatment (P≤0.0001). At 21 and 28 DAT, averages are based on the 2013 and 2015 field trials alone, as the greenhouse trials were terminated after 14 DAT. At 21 DAT, the black hose rinsate treatment had 28% injury (P≤0.0001) and the green hose rinsate had 30% injury (P≤0.0001). These hose types had a greater sequestration potential than the blue hose rinsate at 19% injury (P≤0.0001) with respect to the no-cleanout treatment (Table 2). Within both water and ammonia treatments at 21 DAT, the black and green hose rinsates showed greater dicamba sequestration than other hoses. At 28 DAT, the black hose rinsate showed 29% injury (P≤0.0001) and the green hose rinsate showed 31% injury (P≤0.0001), which were greater than the blue hose rinsate at 19% (P≤0.0001) with respect to the no-cleanout treatment (Table 2). Within the water and ammonia treatments at 28 DAT, the black and green hose rinsates showed greater soybean injury than the other hose types. Table 2 shows the rate titration used in field and greenhouse studies, which are averaged separately over four site years as a comparison to the amount of injury observed. Field and greenhouse trials from all dates show no indication that water or ammonia differs with respect to injury averaged within hose types but do lead to a significant reduction in injury when compared with the no-cleanout treatment. Results from field and greenhouse trials also indicate the blue hose shows the greatest potential to decrease sequestration of the dicamba analyte with respect to observed injury when compared with the check (Table 2).
Table 2 Visual estimation of injury on soybean due to dicamba rinsate from hose type by cleanout procedure with a rate titration of dicamba as comparison from field experiments in 2013 and 2015 and greenhouse 2014 at 7, 14, 21, and 28 d after treatment (DAT).

a Means within a rating date followed by a common letter are not different according to Fisher’s protected LSD test at P=0.05. A numerical LSD is given for each column group.
b Averaged over 6 site years (four field+two greenhouse).
c Averaged over 4 site years (greenhouse trials were terminated after 14 DAT).
d John Deere PMK 4131-08 (yellow), John Deere PMA 4086-08 (blue), John Deere PMA 1687-08 (green), John Deere PMA 1628-08 (gray), and Goodyear (black).
e Untreated check treatment.
f Rate titration averaged separately as comparison.
Soybean height reductions from experiments in 2013 and 2015 show differences at 7, 14, and 21 DAT due to main effect of hose type averaged over cleanout procedure (Table 3). Height reductions at 14 and 21 DAT were greatest with the black hose rinsate treatment (29% reduction from the check; P≤0.0001). At 28 DAT there was an interaction of hose type by cleanout procedure in which height reduction was influenced by dicamba retention in the black hose (36%; P≤0.0001) when compared with the yellow hose (23%; P≤0.0001) and the blue hose (13%; P≤0.0001) with respect to no cleanout (Table 3). Within the water and ammonia treatments at 28 DAT, the black hose rinsate showed 29% plant height reduction from the check (P≤0.0001); a greater effect than all other treatments. At preharvest, node reduction showed a hose type by cleanout procedure interaction in which the black and green hose rinsates resulted in greater node reduction (45 and 43%, respectively; P≤0.0001) when compared with the blue hose rinsate treatment (14%; P≤0.0065) with respect to the no-cleanout treatment (Table 3). Within the water and ammonia treatments at preharvest, the black hose rinsate showed 33 and 32% node reduction (P≤0.0001), greater than all other treatments, with the exception of the green hose rinsate with the water treatment, which showed a 27% (P≤0.0001) node reduction.
Table 3 Soybean height reduction and node reduction due to dicamba rinsate from hose type by cleanout procedure with a rate titration of dicamba as comparison from field experiments in 2013 and 2015 at 7, 14, 21, and 28 d after treatment (DAT) and preharvest.

a Means within a rating date followed by a common letter are not different according to Fisher’s protected LSD test at P=0.05. A numerical LSD is given for each column group.
b John Deere PMK 4131-08 (yellow), John Deere PMA 4086-08 (blue), John Deere PMA 1687-08 (green), John Deere PMA 1628-08 (gray), and Goodyear (black).
c Untreated check treatments.
d Rate titration averaged separately as comparison.
Percent yield reductions from field experiments in 2013 and 2015 showed differences based on main effect of hose type averaged over cleanout procedure in which the black hose had the greatest amount of dicamba sequestration, resulting in a yield reduction of 19% (P≤0.0001), which was greater compared with all treatments although the gray hose rinsate was 13% (P≤0.0001) (Figure 1). Yield reduction observed from the black hose rinsate at 19% was comparable to the 1/256X rate of dicamba at 0.00218 kg ae ha−1, which showed a 16% yield reduction (P≤0.0001). Although significant compared with the check, the blue hose, averaged over all cleanout treatments, resulted in 7% yield reduction (P≤0.0229). It is notable that this average included the blue hose with no-cleanout procedure.

Figure 1 The effect of hose type on percent soybean yield reduction when averaged over all cleanouts in four site years of 2013 and 2015 and showing rate titration as comparison. Hose type: yellow, John Deere PMK 4131-08; blue, John Deere PMA 4086-08; green, John Deere PMA 1687-08; gray, John Deere PMA 1628-08; and black, Goodyear. Capital letters denote significant differences in the treatments.
Dicamba sequestration from black and green hose types produced on average 2 g less of dry matter (P≤0.0001) compared with the check in the 2014 greenhouse studies when main effect of hose type is averaged over cleanout procedures (Figure 2). In comparison to the rate titration, the black and green hose rinsates produced the same amount of dry matter as the 1/64X rate of 0.0087 kg ae ha−1 of 9 g (P≤0.0001) when averaged over cleanout treatments and site years.

Figure 2 Soybean dry matter weight from greenhouse experiments 2014 averaged over cleanout procedures and showing rate titration as comparison. Hose type: yellow, John Deere PMK 4131-08; blue, John Deere PMA 4086-08; green, John Deere PMA 1687-08; gray, John Deere PMA 1628-08; and black, Goodyear.
Chemical Analysis
The rate titration showed a 1X (0.56 kg ae ha−1) rate of dicamba yields roughly 3,000 ppmv of the dicamba analyte (Figure 3). This concentration decreases to 671, 55, and 16 ppmv in relation with the 1/4, 1/64, and 1/256X rates, respectively. The black hose retains more of the dicamba analyte than any other hose regardless of cleanout and is comparable to the 1/256X rate of 0.0022 kg ae ha−1 in procedures tested (P≤0.0001). The green and gray hoses are comparable to the 1/256X rate of 0.0022 kg ae ha−1 with respect to no-cleanout procedure (P≤0.0001). The blue hose showed less retention of dicamba when compared with all other hose types when the water and ammonia cleaning procedures were used (P≤0.0001). When averaging the water and ammonia cleanout over black hose results, we observed on average 16 ppmv of dicamba analyte were retained, which was comparable to that of the 1/256X rate. In 2013 and 2015 there was a 19% yield reduction with respect to dicamba sequestration of the black hose averaged over cleanout procedure when compared with the check (Figure 1). This reduction would be comparable to that of a 1/256X rate, which had a 16% yield reduction from the check.

Figure 3 Hose sequestration of dicamba (ppmv) showing all hose type by cleanout procedures and rate titration as comparison. Hose type: yellow, John Deere PMK 4131-08; blue, John Deere PMA 4086-08; green, John Deere PMA 1687-08; gray, John Deere PMA 1628-08; and black, Goodyear.
During the cleanout process (water or ammonia), 12 L of water were passed through each hose separately. Each hose sequestered 392 ml of solution when 12 L of clean water were passed through the hose; this is essentially 31X the amount of fluid that the hose actually retains. Analyte retention is based solely on hose type with respect to water and ammonia cleanout when observing ppmv analyte retained (Figure 3).
When averaging analyte retained with respect to the black hose averaged over all cleanout procedures, 16 ppmv was an equivalent use rate of 0.5% when compared with the 1X (0.56 kg ae ha−1) rate averaging 3,000 ppmv dicamba analyte. These data would agree with those of Boerboom (Reference Boerboom2004), who showed that dicamba residues, even when an ammonia–water solution was used, had a subsequent percent use rate of 0.63% from the spray boom when it was refilled with water. The blue hose showed retention capabilities of less than 1 ppmv analyte of dicamba retained compared with other hose types with respect to water and ammonia cleanout, with the exception of the yellow hose, which showed less than 3 ppmv. Similarly, Kelly and Riechers (Reference Kelley and Riechers2003) found that as little as 1/10,000 use rate of dicamba may cause injury symptoms.
In this research we observed injury symptoms to varying levels with rinsates from all hose types. When averaged over all cleanouts, even with the blue hose, injury was observed and yield reductions were significant with respect to the untreated check (Table 2). In field trials, yield reduction from the check is 7% with respect to the blue hose averaged over all cleanout procedures (Figure 1). Even when the best hose was used and averaged across cleanout procedures, ppmv analyte retained was 2.03 (Figure 3), which was 0.06% of the 1X (0.56 kg ae ha−1) rate averaging 3,000 ppmv of dicamba analyte, and influenced injury, height reduction, node reduction, and yield reduction.
Hose Analysis Using SEM
Analysis using SEM may show one reason behind the potential for a certain hose type to have retention capabilities differing from those of another. An unused black hose showed holes and retention potential at a magnification of 5.14 K X (Figure 4). In comparison, the inner lining of a used black hose had started to wear over time, increasing the potential for analyte retention (Figure 5).
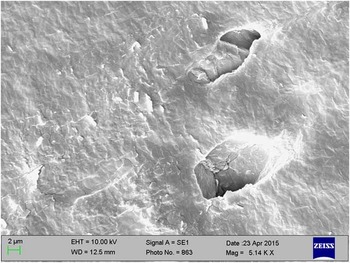
Figure 4 Scanning electron micrograph of a new Goodyear (black/Versigard synthetic rubber) hose.
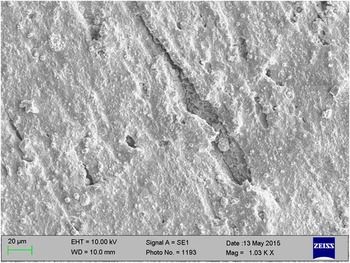
Figure 5 Scanning electron micrograph of a Goodyear (black/Versigard synthetic rubber) hose used eight times.
The visual examination of a new green hose showed imperfections in the manufacturing process of raised nodules (Figure 6) that have potential for breaking loose and creating pockets or increasing the occurrence the cracking observed with a used green hose (Figure 7). A new blue hose showed a smooth almost pattern-like structure throughout (Figure 8). Even after long-term exposure to varying pressures and chemicals, a used blue hose still showed a smooth surface but was not completely free from the effects of wear (Figure 9). Not knowing the manufacturing process (due to patent protection) makes determination of potential imperfections difficult, but the one main difference among all hose types is that the blue hose had a polyethylene core.

Figure 6 Scanning electron micrograph of a new John Deere PMA 1687-08 (green/PVC/polyurethane/high tensile–strength yarn/2 ply) hose.

Figure 7 Scanning electron micrograph of a John Deere PMA 1687-08 (green/PVC/polyurethane/high tensile–strength yarn/2 ply) hose used eight times.

Figure 8 Scanning electron micrograph of a new John Deere PMA 4086-08 (blue/linear/low-density polyethylene blend) hose.

Figure 9 Scanning electron micrograph of a used John Deere PMA 4086-08 (blue/linear/low-density polyethylene blend) hose used eight times.
In conclusion, an increase in injury and height reduction will be observed when a no-cleanout procedure is used regardless of hose type. Both water and ammonia decreased injury, height reduction, and yield reduction by dicamba when compared with no cleanout. The determination of the reduction of the sequestration of the dicamba analyte within hose type was predicated on the chemical makeup of the hose itself, with the blue hose showing the least amount of dicamba retention. Rinsates from the blue hose showed the least injury, height reduction, ppmv analyte retained, and yield reduction with respect to water and ammonia cleanout. The black hose showed the greatest potential for the sequestration of the dicamba analyte and also the greatest amount of injury, height reduction, ppmv analyte retained, and yield reduction when compared with the untreated check. These data may be helpful to producers who wish to use a single sprayer with multiple crops and crops with transgenic traits. These data would suggest that a polyethylene hose may facilitate a more thorough cleanout of dicamba before re-using the sprayer on sensitive crops. This research did not address the durability of each hose type in regard to its effective life span for use when exposed to a variety of solvent systems found in various pesticides.
Acknowledgments
Partial support for this research provided by the Mississippi Soybean Promotion Board, and BASF Corporation. The author would also like to acknowledge the University of Tennessee for their analytical and laboratory support.