Introduction
Modern radiotherapy is a complex procedure that involves the delivery of complex intensity patterns of photon beams from various gantry angles. Intensity-modulated radiation therapy (IMRT) is an advanced radiotherapy technique that allows the radiation dose to precisely conform to the shape of complex tumours while minimising the dose to the surrounding normal tissue. A single plan can consist of more than a 100 control points and include multi-leaf collimator (MLC) leaf movements, gantry and collimator angle rotations and so on in order to produce steep dose gradients within the patient. Currently, the use of IMRT is focused on conformal avoidance of organs at risk (OAR) or dose escalation strategies to the planning target volume aimed at increasing tumour control. Because of the complex nature of treatment planning and delivery of IMRT, a stringent quality assurance (QA) program has been recommended for its safe and effective implementation in clinical practice.Reference Palta, Liu and Li 1
This requires a constant evolution of QA methods used to verify the performance of the systems. Of course, different types of systems have different QA needs. For example, the following types of X-ray radiation therapy delivery systems are different enough from each other that they demand unique QA strategies: static gantry intensity-modulation radiation therapy (IMRT), helical tomotherapy, volumetric-modulated arc therapy (VMAT) and robotic arm therapy.
A patient-specific QA process is often performed in order to ensure the accuracy of the delivered absorbed dose distribution during IMRT treatment. The QA is most often based on measurements of delivered dose to find possible deviations between the planned and the delivered dose distribution. The method used for patient-specific IMRT QA measurements depends on the equipment available at the clinic, but the result of the QA is also dependent on the evaluation procedure, for example, the choice of acceptance criteria and tolerance levels. The QA could be designed to have different intentions, either to reveal major errors, such as no MLC in the beam or use of the wrong plan, but it could also be designed to find smaller deviations between planned and delivered dose. It is important to understand what the combination of dosimetry system and valuation procedure implies in terms of what kind of deviations and/or errors will be detected.
When modern techniques, such as IMRT and VMAT, are introduced into clinical practice, extensive dosimetric QA is required to ensure accurate treatment delivery. This QA encompasses both general machine performance and QA at the patient-specific level. In our busy department, we are treating VMAT and IMRT for various body sites: prostate, lung, head and neck and breast cancer. For each patient, measurements are performed using the Delta4 phantom for the preclinical testing. Due to the unique and patented design of Delta4 phantom (Scandidos, Uppsala, Sweden) with a cylindrical, water equivalent, phantom and a high number of detectors in fixed positions in the isocentric tumour and high-gradient region, it can directly verify the delivered dose and compare this with the plan without any additional processing software.
In this study, we measure and analysed the dose distribution of different IMRT, VMAT and three-dimensional conventional radiotherapy (3D CRT) plans with the intent to provide pretreatment patient-specific QA.
Materials and Method
All measurements were carried out at University Hospital Ghent. Ninety-five patients were randomly selected in this study, IMRT-30 cases, VMAT-40 cases, Stereotatic Body Radiation Therapy-20 cases and 3D CRT-5 cases. The experiments were performed using a linear accelerator equipped with a 120-leaf millennium MLC (Varian Medical Systems, Palo Alto, CA) with the record and verify system ARIA. Two Synergy linear accelerators equipped with a conventional MLC, model MLCi2, of 1 cm leaf width (Elekta, Inc. Crawley, UK) with Mosaiq (a certified electronic medical record and practice management system). The treatment plans were generated using Eclipse 8.5 (Varian) and RayStation® v6 treatment planning system (TPS) (RaySearch Medical Laboratories, Stockholm, Sweden) respectively.
The Delta4 phantom monitors with high accuracy, the dose that is delivered to the patient. It automatically compares the delivered dose with the planned dose, ensuring that the treatment is delivered precisely and safely.
The Delta4 is a cylindrical phantom made of polymethylmethacrylate (PMMA) with the dimensions of 22 cm in diameter and a length of 40 cm (Figure 1). There are two detector planes crossing each other at the center line that generally contains the treatment isocenter point. Although the system has detectors in only two planes, it features a unique interpolation algorithm that is capable of estimating doses at points where no detectors are present.
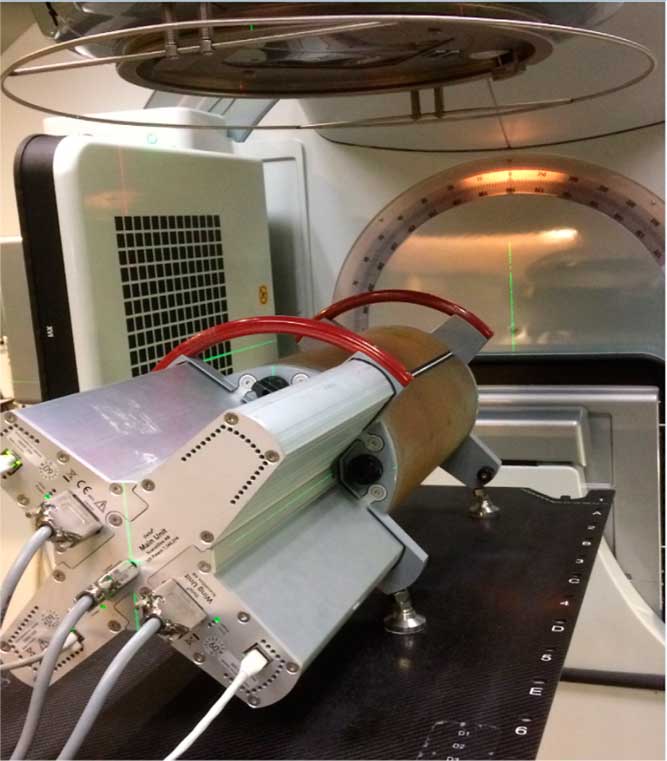
Figure 1 Delta4 phantom set up at isocenter in measurement position.
The planar main detector board divides the entire phantom into two halves, while the two wing detector boards are within the second plane but separated allowing the main detector board to pass through the centre line of the phantom.
The boards are angled at +50° from the vertical for the main board and −40° for the wings. This allows for added flexibility to make sure the beam is not parallel to the boards. The two detector planes consist of 1069 p-type Silicon diodes. Each diode is sampled per dose pulse so that the dose distribution can be evaluated on segment-by-segment, beam-by-beam, or as a composite plan from a single set of measurements. The detection area per plane is 20×20 cm2, and the diodes are placed with a spacing of 0·5 cm in the central area (6×6 cm2) and a spacing of 1 cm in the outer area. The maximum deviation of the detector positions is 0·5 mm. The lowest dose that can be measured is 1 mGy and the resolution is 0·01 mGy. 2 The device records measured dose in relation to the individual accelerator pulses by using a trigger signal from the accelerator.
After the radiation oncologist had accepted the patient treatment plan, it was transferred within the TPS to the CT scan of the Delta4 phantom in order to calculate the dose in the phantom. The recalculating dose distribution, the planning data, such as the beam arrangement, and structures from the original plan were transferred to the Delta4 system as Digital Imaging and Communication in Medicine, Radiation Therapy objects. The software has a variety of tools for displaying and analysing the difference between the measured and the calculated dose.
The agreement between calculated and measured dose distributions was analysed. The most important used parameters are dose agreement (DA) and distance to agreement (DTA). DA is the percentage or absolute difference between measured and calculated dose. It is not suitable for the high-dose gradient region, where even small spatial errors may lead to large but not significant errors. Therefore, DTA is used in high-dose gradient region. It is defined as the minimum distance, in the plane, between a measured point and the nearest point in the calculated dose distribution that has the same dose. It is important to indicate that DTA measurement depends on the choice of the correct common coordinate system between measured and calculated dose. The results depend on the choice of DTA.
The gamma analysis (γ) was performed based on the formulae by Low et al.Reference Low, Harms, Mutic and Purdy 3 , Reference Low 4 The histograms of dose deviation (DD), DTA, gamma index and the passing rates were calculated by the Delta4’s software. The acceptance criteria used in our department are 3% dose difference and 3 mm DTA and the γ-index passing rate is 95% and 90% for IMRT and VMAT, respectively. The Delta4 has the ability to measure and evaluate each field individually and also the total plan. In our Radiation Therapy department, 30 patients with different pathologies, were controlled for IMRT and VMAT by using the Delta4 phantom per week. The correction factor of Delta4 phantom was measured in box field technique (gantry angles of 0°, 90°, 180° and 270°) for a 10×10 cm2 field size, daily. The correction factor is recommended by vendor due to correction of temperature of Delta4 phantom in the storing place. The factor was applied to each patient plan.
Results
All plans were analysed using the three parameters, the %DA (limit 3%), DTA (limit 3 mm), and γ-pass rate with the 3% dose tolerance and 3 mm DTA in relation to the TPS. Table 1 summarises the results of average γ-passing rate, dose deviation and DTA of different pathologies in our center. Overall, good agreement was observed between measured and calculated doses in most cases. The gamma pass rate percentages over all plans were higher than ≤97·1% using 3% DD and 3 mm DTA. It should be noted that for all plans, our clinical standard of 90% or greater for the gamma index percentage was achieved. Myers et al.Reference Myers, Stathakis, Gutiérrez, Esquivel, Mavroidis and Papanikolaou 5 reported a similar result as well. Bedford et al.Reference Bedford, Lee, Wai, South and Warrington 6 reported that, from the gamma values, Delta4 shows slightly better agreement between measured and calculated doses than the film in a cuboid phantom. The reason could be the fact that the Delta4 measurements are absolute, whereas film is used as a relative dosimeter.
Table 1 Average γ-passing rate, dose deviation and distance to agreement of different pathologies. Indicated statistical error uncertainty is standard deviation

Figure 2 shows an example of measurements on a nasopharynx case. The treatment plan consists of seven beam IMRT. The daily correction factor was applied. The planned dose distribution was in greyscale and the measured dose in colour over the main and wing detector boards. The software shows the matrix of γ statistics points (blue pass (γ<1) and red fail (γ>1)).
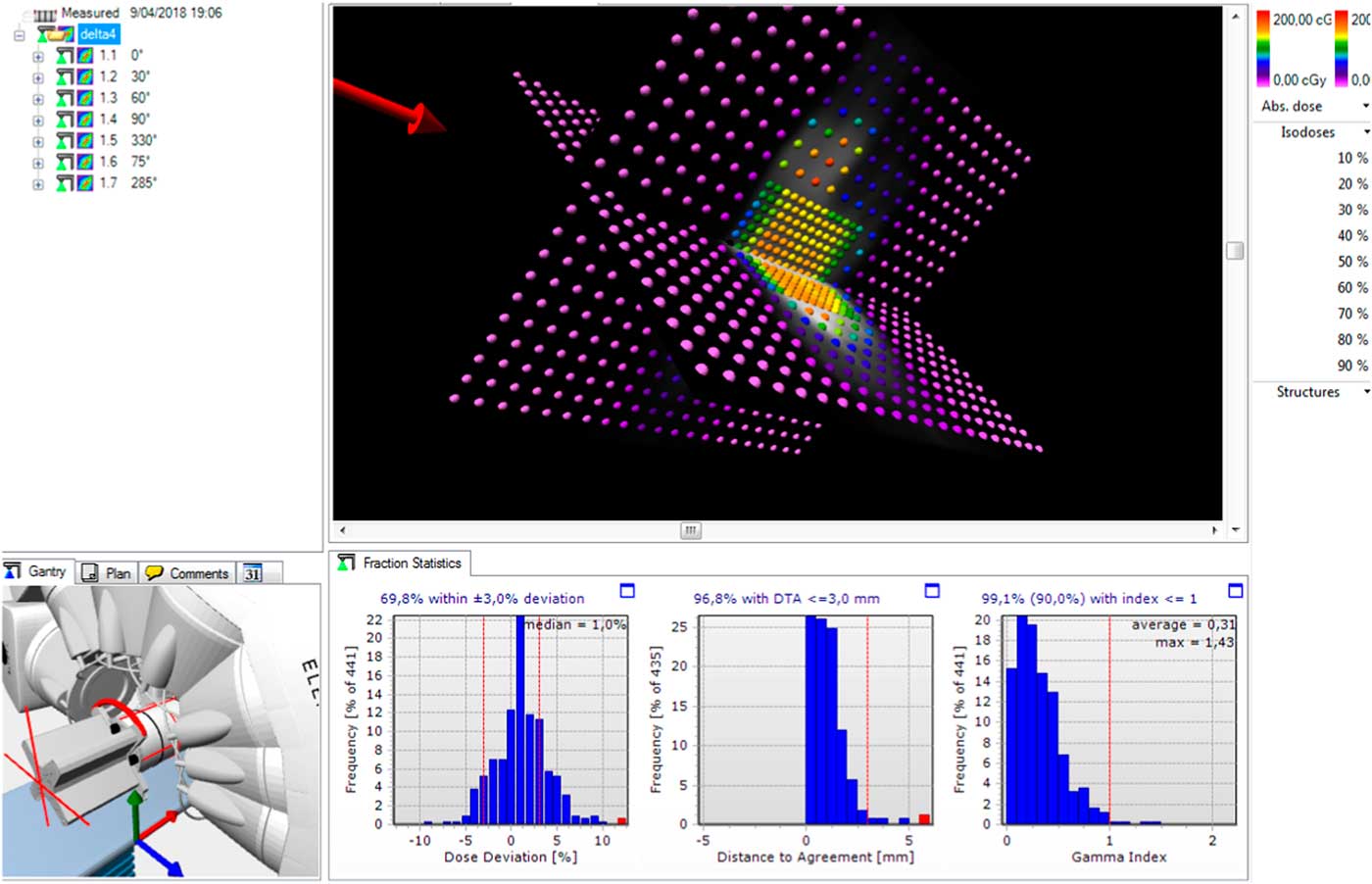
Figure 2 A screenshot from the Delta4 software showing the result of seven beams intensity-modulated radiation therapy (IMRT) delivery for a nasopharynx case. The upper panel shows the absolute dose measured in two diode arrays in 3D with color coding denoting the dose. The lower panel shows three histograms: dose deviation from the planned dose, distance to agreement from the planned dose and gamma index distribution.
Figure 3 shows the total absolute dose difference between planned and measured dose in X- and Y-axis of detector plane in nasopharynx case. The software can also analyse absolute and relative dose difference in beam per beam.
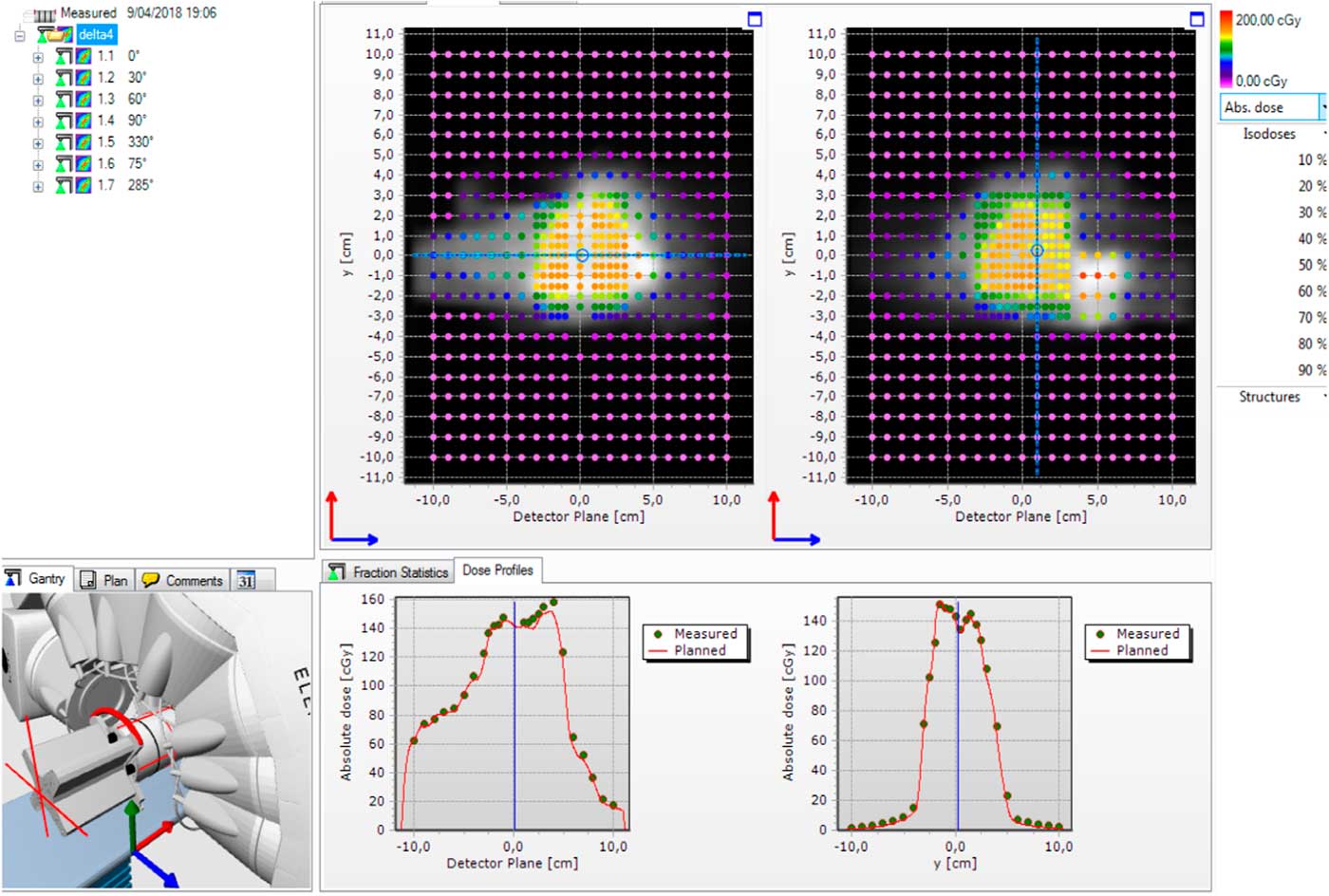
Figure 3 A screenshot from the Delta4 software showing seven beams intensity-modulated radiation therapy (IMRT) delivery. The upper panel shows the absolute dose difference in two diode arrays in 2D with color coding denoting the dose. The lower panel shows two graphs: dose profiles along X- and Y-axis.
The daily correction factor was found to be 1·02±0·0056 (SD). The temperature dependence of the diodes is another potential source of uncertainty.Reference Nilsson 7 It has shown it to be very small (i.e., 0·4%/°C). Several basic tests (linearity of segmental dose, angular sensitivity, dose rate) were carried out to examine specific performance characteristics of the Delta4 phantom. These results have not been presented here. Delta4 phantom is also fully recalibrated once a year.
In practice, if portal dosimetry (PD) fails, which means that the plan has not passed the gamma criteria passing rate (3%, 3 mm), the patient plan is then re-measured by using the Delta 4 phantom. The same plan has passed the gamma criteria using the Delta 4 phantom. The limited size of the detector panel of the linear accelerator is a major reason for PD failure. Other patient-specific reasons for PD failure were not further investigated. Daci and MalkajReference Daci and Malkaj 8 studied Delta4 phantom and PD as dosimetry verification tools for IMRT and VMAT. They conclude that Delta4 was more accurate in measurements, however, the electronic portal imaging device is more time efficient.
Discussion
The Delta4 phantom can be set-up easily, and positional errors can be reduced to the minimum. It is important that the initial calibration and the commissioning process of Delta4 are accomplished with rigorous and careful measurements to ensure that patient-specific QA tests are accurate. It provides a thorough data analysis and a relatively faster way to take measurements without necessitating further QA systems. Measurements take place on the two planes of the phantom, where a 3D dose distribution of the dose can be computed by the software of the phantom through an interpolation method.
According to the study by Nelms et al.,Reference Nelms, Zhen and Tomé 9 a field-by-field evaluation is unsuitable for IMRT. Their study showed that gamma passing rates with the criteria of (3%, 3 mm) are not sensitive to clinically relevant patient dose errors on a field by field basis, which is confirmed by our study as well. Sadagopan et al.Reference Sadagopan, Bencomo, Martin, Nilsson, Matzen and Balter 10 also confirmed that the Delta4 device is accurate and reproducible in comparing to an ion chamber and film.
For a VMAT plan, where roughly 33% of the arc intersects with the couch, the effect of the couch should be modelled in the TPS, although the dosimetric effect of this is often not a straightforward process on a routine basis. Our previous studyReference Paelinck, Vanderstraeten, Srivastava, Olteanu and De Wagter 11 suggests that the tabletop modeling results are in better agreement between measurements and calculations, both for total plans and individual beams. This agreement further improves when proper correction factors are applied.
Nilsson et al.Reference Nilsson, Hauer and Bäck 12 investigated dose distributions passing the gamma evaluation (5%, 5 mm) according to the recommendation of the International Commission on Radiation Units and Measurements report 83 13 with the use of the Delta4 dosimetry system. We also confirmed the passing gamma criteria (3%, 3 mm). Chandraraj et al.Reference Chandraraj, Stathakis, Manickam, Esquivel, Supe and Papanikolaou 14 used four commercial devices [PTW 2D-ARRAY seven29 (PTW Freiburg GmbH, Germany), IBA I’MatriXX (IBA dosimetry, GmbH, Schwarzenbruck, Germany), EDR2 film (Kodak, Rochester, NY) and Delta4 phantom)] and concluded that Delta4 measurements met the gamma criteria in all pathologies.
The Delta4 phantom consists of two orthogonal detector planes located at a specific position inside the cylindrical phantom. The detector points are located on these two planes and the absorbed dose can only be determined in these points. The spinal cord is located in the dorsal region, and the parotid glands are located in the left and right periphery. These positions are not covered by any detector points in the phantom. The OAR is thereby located between the detector planes. It seems that these organs are not able to be measured by existing software in our department. Delta4DVH software module only allows comparison in patient anatomy of the planned and the delivered patient dose. The real dose deviation in each organ is evaluated by Delta4DVH software. The software immediately determines if the dose to an organ at risk is too high or not.
Conclusion
The Delta4 software and phantom system offer a fast set-up, measurement control and three-dimensional analysis that are highly adapted to the complexities of modulated irradiation. The results indicate that Delta4 phantom is an effective and efficient method for patient-specific QA. Delta4 phantom has proven to be fast and reliable for patient-specific QA of the VMAT, step-and-shoot IMRT and 3D CRT. Patient-specific QA using Delta4 phantom has not been compared against another QA verification system due to the pressures of time and other QA systems are not available in the department at the present time. The work could be further extended in the future.
Acknowledgement
None.