INTRODUCTION
The steady growth of dog populations in urban areas increases the likelihood of transmission of zoonotic helminths, particularly Toxocara canis an ancient parasite of canids, as indicated by paleoparasitological analysis of egg fossils (Bouchet et al. Reference Bouchet, Araújo, Harter, Chaves, Duarte, Monnier and Ferreira2003). This roundworm is a nematode of the order Ascaridida, superfamily Ascaridoidea and family Toxocaridae, whose adults forms live attached to the mucosa of the small intestine, mainly in puppies and older dogs, daily producing thousands of non-infectious immature eggs that are excreted with the feces into the environment (Daryani et al. Reference Daryani, Sharif, Amouei and Gholami2009). In the soil under appropriate environmental conditions, the zygote of Toxocara begins to divide forming a third-stage larva inside the egg at approximately 4 weeks, which can survive for many years protected by the highly resistant eggshell (Brunaská et al. Reference Brunaská, Dubinsky and Reiterova1995). This evolutionary phase of the egg, now mature, is ready to infect both definite and paratenic hosts such as humans (Mizgajska-Wiktor and Uga, Reference Mizgajska-Wiktor, Uga, Holland and Smith2006). Due to the inability to complete its life cycle in this erratic host, the infection results in migration of larvae through the tissues, classified in 3 clinical syndromes: visceral larva migrans, ocular larva migrans and occult toxocariasis (Taylor and Holland, Reference Taylor, Holland, Gillespie and Pearson2001; Despommier, Reference Despommier2003).
Although dogs may be infected by accidental ingestion of mature eggs or by predation of small mammals harbouring infective larvae encysted in somatic tissues (Parsons, Reference Parsons1987; Dubinsky et al. Reference Dubinsky, Havasiova-Reiterowa, Petko, Hovorka and Tomasovicova1995), the most important route of infection is transplacentally by migration of larvae from tissues of the pregnant bitch across the uterus (Overgaauw and Knapen, Reference Overgaauw and Knapen2008). However, it is believed that the most important route for human infection, especially children, occurs when accidentally ingesting mature eggs via hand-to-mouth contact after dirtying hands with contaminated soil or consuming poorly washed raw vegetables (Glickman, Reference Glickman, Lewis and Maizels1993; Lloyd, Reference Lloyd, Palmer, Soulsby and Simpson1998). It can also occur through ingestion of infective larvae in raw or poorly cooked meat from paratenic hosts such as poultry (Morimatsu et al. Reference Morimatsu, Akao, Akiyoshi, Kawazu, Okabe and Aizawa2006), sheep (Salem and Schantz, Reference Salem and Schantz1992), pigs (Sturchler et al. Reference Sturchler, Weiss and Gassner1990) and cattle (Aragane et al. Reference Aragane, Akao, Matsuyama, Sugita, Natsuaki and Kitada1999).
Toxocara eggs have been reported to be commonly found in soil of public areas worldwide: Argentina (Alonso et al. Reference Alonso, Stein, Chamorro and Bojanich2001); Brazil (Coelho et al. Reference Coelho, Dini, Milman and Oliveira2001); England (Snow et al. Reference Snow, Ball and Bewick1987); France (Ferré and Dorchies, Reference Ferré and Dorchies2000); Italy (Giacometti et al. Reference Giacometti, Cirioni, Fortuna, Osimani, Antonicelli, Del Prete, Riva, D'Errico, Petrelli and Scalise2000); Ireland (O'Lorcain, Reference O'Lorcain1994b); Japan (Shimizu, Reference Shimizu1993); Spain (Ruiz de Ybáñez et al. Reference Ruiz de Ybáñez, Garijo and Alonso2001); Turkey (Avcioglu and Burgu, Reference Avcioglu and Burgu2008). These reports show that soil is a potential source of Toxocara infection for dogs and humans (Overgaauw, Reference Overgaauw1997a,Reference Overgaauwb). In many cities, soil contamination of public areas with Toxocara eggs is expected be high due to the large number of dogs that have access to a relatively small green space (Genchi and Traldi, Reference Genchi and Traldi1994). In particular, stray dogs are the major disseminators of eggs of this roundworm due to lack of anthelmintic treatment in comparison to domestic dogs (O'Lorcain, Reference O'Lorcain1994a).
The elimination of Toxocara eggs from the soil is viewed as a nearly impossible task, but if such a strategy could be found and safely implemented, potentially dangerous public areas could be free of this parasite (Despommier, Reference Despommier2003). Because some filamentous fungi of the soil, called nematophagous, are natural enemies of nematodes and can negatively influence the transmission of animal-parasitic nematodes by using their content as a source of nutrients (Nordbring-Hertz et al. Reference Nordbring-Hertz, Jansson and Tunlid2006), a decline in soil contamination may be possible through their use as biological controllers.
There are 3 main groups of nematophagous fungi and, of these, the egg-parasitic group is able to penetrate nematode eggs and assimilate their content or cause distortions in their developing embryo (Lysek and Sterba, Reference Lysek and Sterba1991; Jansson and Lopez-Llorca, Reference Jansson, Lopez-Llorca and Arora2004). They are nutritionally versatile and their great saprophytic ability allows them to survive in soil for long periods even in the absence of nematode eggs (Chen and Dickson, Reference Chen, Dickson, Chen, Chen and Dickinson2004).
Studies on the control of nematodes by fungal antagonists have identified promising egg-parasitic species of which Pochonia chlamydosporia (Goddard) Zare & Gams (syn. Verticillium chlamydosporium) and Paecilomyces lilacinus (Thom) Samson are potential biocontrol agents due to their recognized parasitic activity on eggs of plant-parasitic nematodes (Kerry, Reference Kerry, Butt, Jackson and Magan2001; Kiewnick and Sikora, Reference Kiewnick and Sikora2006) and animal-parasitic nematode eggs (Basualdo et al. Reference Basualdo, Ciarmela, Sarmiento and Minvielle2000; Araújo et al. Reference Araújo, Braga, Milani, Silva and Tavela2008; Carvalho et al. Reference Carvalho, Araújo, Braga, Araújo and Alves2010). However, P. lilacinus is an opportunistic fungus that may occasionally cause infections in humans (Blackwell et al. Reference Blackwell, Ahmed, O'Docherty and Hay2000; Carey et al. Reference Carey, D'Amico, Sutton and Rinaldi2003) as well as in animals (Foley et al. Reference Foley, Norris and Jang2002; Pawloski et al. Reference Pawloski, Brunker, Singh and Sutton2010).
Because environmental contamination with Toxocara eggs poses a risk to both animals and humans, the objective of the present study was to evaluate P. chlamydosporia isolates with respect to their ability to infect T. canis eggs on cellulose dialysis membrane and their potential as biocontrol agents of this nematode.
MATERIALS AND METHODS
Inoculation of P. chlamydosporia
Six isolates of P. chlamydosporia var. chlamydosporia (Pc-02, Pc-03, Pc-04, Pc-07, Pc-09 and Pc-10), characterized by ellipsoidal conidia arranged in heads (Zare et al. Reference Zare, Gams and Evans2001), were evaluated. These isolates, previously obtained from Brazilian soil samples by the soil plating method in a semi-selective medium according to Leij and Kerry (Reference Leij and Kerry1991), are part of the mycology collection of the Laboratory of Biological Control of Nematodes, Federal University of Viçosa, Brazil and were stored at 4°C in BD Vacutainer® glass tubes (Becton Dickinson, Brazil) containing blue silica gel on filter papers measuring 5 mm×5 mm, which were dehydrated after being impregnated with chlamydospores dispersed in a solution of distilled water and 10% powdered milk (Smith and Onions, Reference Smith and Onions1983). The tubes were closed with their original rubber stoppers and sealed with polyvinyl chloride (PVC) plastic wrap.
Two filter paper strips of each fungal isolate were aseptically transferred from one of the tubes to 90 mm×10 mm Petri dishes containing a non-synthetic cornmeal agar culture medium (20 g of corn flour and 20 g of agar per 1000 ml of distilled water). The plates were closed, sealed with PVC wrap and incubated at 25°C during 4 weeks for colony growth and production of chlamydospores. At the end of this period, the mycelial fragments and chlamydospores were aseptically harvested by gently scraping the surface of the colony with a fine brush after submersion in 15 ml of sterile ultrapure water. The fungal suspension was filtered through 2 levels of sterile gauze into a Griffin glass recipient. The chlamydospore suspension, now containing fewer mycelial fragments, was transferred to a 50 ml culture tube with screw cap (Pyrex®) and agitated for 30 sec after adding 1 drop of polysorbate 80 (Tween 80) in order to obtain a better dispersion of the chlamydospores. They were then counted microscopically (100× magnification) using a Neubauer chamber according to the classical procedures and dilutions were prepared in the desired concentrations.
Inoculum of Toxocara canis
Adult T. canis were collected from fresh feces after administration of a commercial anthelmintic agent (14·5 mg/kg of pyrantel pamoate and 5 mg/kg of praziquantel) to naturally infected mongrel pups; and the worms were washed repeatedly in tap water to eliminate residual fecal material. Female specimens were selected by morphological identification using a stereomicroscope, axenized for 5 min in a solution of sodium hypochlorite 0·5% (v/v), washed in distilled water and submersed in a physiological solution. Next, one by one they were transferred to a 90 mm×10 mm Petri dish, hysterectomized and the uterus dissected under a stereomicroscope with the aid of a scalpel to release fertilized immature eggs (Fenoy et al. Reference Fenoy, Del Hoyo and Guillen-Llera1987). The eggs and uterus fragments were removed from the plate by rising with water from a wash bottle onto a 400 mesh stainless steel sieve (38 mm opening) attached to another 500 mesh sieve (25 mm opening) and this sieve set was maintained under running water to allow filtration of the eggs.
Eggs retained in the lower sieve were transferred to a 150 ml Griffin recipient by rinsing with water from a wash bottle. Next, the suspension of eggs was poured into a 50 ml Falcon tube and centrifuged at 715 g for 5 min. The water was discarded and the decanted eggs were resuspended in a 30 ml of formalin solution 2% (v/v). After 10 min, half of the egg suspension was transferred to another Falcon tube to be washed with sterile ultrapure water for formalin removal, performing 5 centrifugations and substitution of water between each. The other half of the egg suspension remained in formalin for 30 days at 28°C under aeration from a vacuum pump to allow for embryonation of the eggs based on the method adapted from Bowman et al. (Reference Bowman, Mika-Grieve and Grieve1987). At the end of this period, about 66% of the eggs were mature and before being used, they were washed with sterile ultrapure water to remove formalin as described previously.
Six 10 ml aliquots of each suspension were deposited on glass slides marked with longitudinal lines, covered with a coverslip and counted using a stereomicroscope (40× magnification). The numbers of eggs in the suspensions were estimated based on the average of the aliquots and then adjusted to approximately 200 eggs per 50 μl. Egg viability was evaluated before being used in the tests.
Bioassays of fungi-nematode interaction
Effect of days in pathogenicity of P. chlamydosporia isolates on T. canis eggs
In vitro assays were performed to evaluate the ability of the isolates Pc-02, Pc-03, Pc-04, Pc-07, Pc-09 and Pc-10 of P. chlamydosporia to infect immature and mature T. canis eggs. The fungus-nematode interaction occurred on 4 cm×4 cm strips of cellulose dialysis membrane (Sigma-Aldrich, USA), similar to the technique described by Nordbring-Hertz (Reference Nordbring-Hertz1983) but not accommodated on 2% water agar (WA) culture medium since the chlamydospores have sufficient nutrients to guarantee the germination and growth of the mycelium to an extent that it is able to reach the eggs, and because the microscope observation is facilitated without the culture medium.
To prepare the strips for use, they were carefully washed 5 times in ultrapure water and sterilized at 121°C for 15 min, immersed in ultrapure water in a 150 ml glass Griffin recipient, closed with aluminum foil and sealed with PVC wrap. In a vertical laminar flow hood the strips were then gently placed individually on the inner surface of the bottom half of 50 mm×10 mm Petri dishes. Strips on the plates of each treatment received 20 ml of an ultrapure water suspension containing approximately 500 chlamydospores of only one of the respective isolates. The plates were closed, sealed with PVC wrap and accommodated in a plastic tray lined internally with filter paper and covered with a plastic film in order to simulate a moist chamber, thus avoiding drying of the membrane. Next, the infested plates were incubated in the dark at 25°C during 7 days for germination of chlamydospores and colonization of the membrane. At the end of this period, 50 μl of an ultrapure water suspension containing approximately 200 immature T. canis eggs were added to the Petri dishes on the colonized membranes. The same nematode suspension also was added to membranes without fungi in the Petri dishes of the control group. Plates were sealed and incubated for 21 days as described above. A transparent adhesive tape, with the same dimensions as the dialysis membrane and with printed black lines forming parallel vertical and horizontal squares of 4 mm2, was applied on the outer surface of the lower half of all Petri dishes to guide observation of the eggs.
At 7-day intervals throughout the incubation period, the plates were turned upside down to examine the T. canis eggs under a light microscope (100× magnification). The eggs were considered colonized when they were invaded by hyphae of the nematophagous fungus. The number of colonized eggs was counted and their percentage calculated in relation to the total number of eggs present.
Effect of concentration on pathogenicity of P. chlamydosporia isolates on T. canis eggs
To evaluate the effect of increasing inoculum doses of the fungal isolates 02-Pc, Pc-03, Pc-04, Pc-07, Pc-09 and Pc-10 of P. chlamydosporia on the infection of immature and mature T. canis eggs, 5 concentrations of chlamydospores were utilized (1000, 2000, 3000, 4000 and 5000), applying the methodology previously described and respecting the chlamydospore/egg relationship (10:1, 20:1, 30:1, 40:1 and 50:1). Inoculation of the suspensions containing fungal concentrations of each of the respective isolates on the cellulose dialysis membrane in the Petri dishes was also performed 7 days prior to the addition of approximately 200 T. canis eggs on the membranes of these plates and for the control group without fungi. Then, after 21 days of interaction, the percentage of colonized T. canis eggs was calculated in relation to the total number of eggs present.
Data analysis
Assays were conducted in a completely randomized design, using 7 replicates per treatment, where the experimental unit was represented by a Petri dish containing a dialysis membrane. The Tukey's test was used to compare the mean percentages of colonized eggs in the fungal treatments and Dunnett's test was used for their comparison to the control group without fungi, both at the level of 5% significance. Regression analysis was used to relate the pathogenicity of isolates with the days of interaction and their concentration. All procedures were performed using the statistical software Statistica, version 7.0 (Statsoft, 2004).
RESULTS
The modified procedure using the cellulose dialysis membrane without 2% WA as substrate allowed the fungal growth from germination of chlamydospores (Fig. 1) and facilitated observation of the nematode-fungus interaction under the light microscope. Table 1 presents the means and standard deviations of the percentages of T. canis eggs colonized by the fungal isolates at 7-day intervals over a period of 21 days in the interaction tests using immature or mature eggs. All isolates tested were able to colonize eggs in relation to the control group without fungi (P<0·05). The isolate Pc-04 was the most efficient in colonizing both immature and mature T. canis eggs. Isolates Pc-02 and Pc-09 were the least efficient and isolates Pc-03, Pc-07 and Pc-10 presented intermediate results. The isolate Pc-04 infected a greater number of eggs more quickly than the other isolates; however, its ability to infect mature and immature eggs was similar to the isolate Pc-07 at 14 and 21 days of interaction, respectively. Isolate Pc-03 did not differ from the isolate Pc-10 in parasitism of immature eggs at the 7th and 21st days of interaction. On the 7th day it was also observed that the isolates Pc-03, Pc-07 and Pc-10 did not differ in their ability to infect mature eggs. Parasitism on this evolutionary phase of the T. canis egg continued to show similarity between isolates Pc-03 and Pc-10 in the subsequent days of assessment. The isolate Pc-02 showed similarities with the isolate Pc-09 regarding its ability to infect mature eggs in all days of assessment.

Fig. 1. Photomicrograph by differential interference contrast of a Pochonia chlamydosporia chlamydospore in germination on the cellulose dialysis membrane after 48 h of incubation in the dark at 25°C (400× magnification).
Table 1. Mean percentage and standard deviation of immature and mature Toxocara canis eggs colonized by the isolates Pc-02, Pc-03, Pc-04, Pc-07, Pc-09 and Pc-10 of Pochonia chlamydosporia after 7, 14 and 21 days of interaction at 25°C on pre-colonized cellulose dialysis membrane with 500 chlamydospores inoculated 7 days prior to the addition of approximately 200 eggs of the nematode

P. chlamydosporia isolates | Interaction period | |||||
7 days | 14 days | 21 days | ||||
Immature eggs | Mature eggs | Immature eggs | Mature eggs | Immature eggs | Mature eggs | |
Pc-02 | 15·28d±3·04 | 8·14d±0·90 | 26·00e±4·04 | 16·86c±1·21 | 38·43c±4·65 | 22·86d±1·46 |
Pc-03 | 34·71c±5·91 | 13·28c±3·68 | 48·00d±4·83 | 23·00b±2·45 | 64·43b±4·93 | 34·57c±4·07 |
Pc-04 | 57·57a±4·35 | 22·71a±3·99 | 70·43a±5·41 | 33·43a±3·86 | 79·71a±2·14 | 59·14a±1·67 |
Pc-07 | 49·57b±3·60 | 19·14b±2·11 | 63·71b±3·90 | 29·43a±1·51 | 74·28a±3·77 | 45·57b±4·96 |
Pc-09 | 9·71e±1·49 | 7·57d±1·27 | 17·43f±2·37 | 12·71c±1·60 | 25·71d±1·97 | 19·86d±1·46 |
Pc-10 | 33·43c±3·69 | 15·71bc±1·11 | 56·14c±2·67 | 20·57b±3·31 | 62·86b±1·77 | 39·00c±4·32 |
Control | 0f | 0e | 0g | 0d | 0e | 0e |
The mean and standard deviation of the percentage of T. canis eggs colonized in the treatments with different concentrations of the fungal isolates at the end of 21 days of interaction are presented in Tables 2 and 3 for tests with immature and mature eggs, respectively. There was no colonization of eggs in the control group without fungi and all isolates tested were able to colonize eggs in relation to this parameter (P<0·05). As observed in the initial trial, isolate Pc-04 was the most infectious on the 2 evolutionary phases of T. canis eggs followed by isolates Pc-07, Pc-10 and Pc-03. However, there was no significant difference among Pc-04, Pc-07 and Pc-10 in the ability to infect immature eggs at the inoculum concentration of 5000 chlamydospores. The isolates Pc-02 and Pc-09, which presented no differences in their infective ability, were the least effective.
Table 2. Mean percentage and standard deviation of immature Toxocara canis eggs colonized by the isolates Pc-02, Pc-03, Pc-04, Pc-07, Pc-09 and Pc-10 of Pochonia chlamydosporia after 21 days of interaction at 25°C on pre-colonized cellulose dialysis membrane with 1000, 2000, 3000, 4000 and 5000 chlamydospores inoculated 7 days prior to the addition of approximately 200 eggs of the nematode

P. chlamydosporia isolates | Fungal inoculum concentration (Chlamydospores/Petri dish) | ||||
1000 | 2000 | 3000 | 4000 | 5000 | |
Pc-02 | 27·14e±2·97 | 31·43e±3·64 | 36·71e±4·57 | 43·00e±4·04 | 48·85d±5·08 |
Pc-03 | 47·00d±2·88 | 53·43d±4·31 | 59·43d±2·63 | 68·86d±6·33 | 75·57c±3·31 |
Pc-04 | 74·71a±2·98 | 81·14a±2·34 | 88·43a±1·51 | 93·86a±2·41 | 97·86a±1·07 |
Pc-07 | 66·71b±2·81 | 69·86b±2·19 | 76·00b±3·05 | 88·71b±1·97 | 93·00ab±2·08 |
Pc-09 | 24·43e±2·88 | 29·43e±3·78 | 30·86f±3·08 | 44·28e±4·68 | 48·28d±2·92 |
Pc-10 | 59·86c±2·34 | 63·00c±2·71 | 67·28c±1·97 | 82·71c±2·81 | 89·28b±1·97 |
Control | 0g |
Table 3. Mean percentage and standard deviation of mature Toxocara canis eggs colonized by the isolates Pc-02, Pc-03, Pc-04, Pc-07, Pc-09 and Pc-10 of Pochonia chlamydosporia after 21 days of interaction at 25°C on pre-colonized cellulose dialysis membrane with 1000, 2000, 3000, 4000 and 5000 chlamydospores inoculated 7 days prior to the addition of approximately 200 eggs of the nematode

P. chlamydosporia isolates | Fungal inoculum concentration (Chlamydospores/Petri dish) | ||||
1000 | 2000 | 3000 | 4000 | 5000 | |
Pc-02 | 13·14e±4·14 | 20·43e±3·82 | 22·28e±2·29 | 25·00e±3·21 | 32·00e±2·16 |
Pc-03 | 37·86d±2·73 | 42·57d±2·22 | 52·43d±3·36 | 57·14d±2·11 | 64·00d±3·87 |
Pc-04 | 66·57a±3·36 | 72·86a±2·26 | 77·14a±2·34 | 78·43a±1·51 | 83·00a±1·73 |
Pc-07 | 58·71b±2·56 | 62·43b±2·37 | 66·86b±2·11 | 72·43b±1·51 | 76·43b±2·07 |
Pc-09 | 12·86e±1·34 | 17·57e±1·72 | 21·71e±1·49 | 26·43e±2·07 | 27·71f±3·73 |
Pc-10 | 46·28c±2·81 | 54·71c±2·56 | 61·86c±2·26 | 68·00c±2·08 | 70·57c±3·86 |
Control | 0g |
In Figs 2 and 3, respectively, linear regression curves show the effect of time (days) on the parasitism of immature and mature T. canis eggs by P. chlamydosporia isolates at intervals of 7 days over a 21-day interaction period. The positive slope of the trend curves indicated a direct relationship between the percentage of colonized eggs and the increase in interaction time (P<0·01). The linear model better explained the relationship between the colonization of T. canis eggs and levels of inoculum of the fungal antagonists expressed by the equations. Angular coefficients of the regression curves and coefficients of determination (R2) for the assay using immature eggs, corresponding to treatments with the P. chlamydosporia isolates, were: Pc-02 (11·5714, R2=0·86); Pc-03 (14·8571, R2=0·86); Pc-04 (11·0714, R2=0·84); Pc-07 (12·3571, R2=0·89); Pc-09 (8·8571, R2=0·84); Pc-10 (14·7143, R2=0·87), while values of linear regression and R2 for the assay using mature eggs were: Pc-02 (7·3571, R2=0·95); Pc-03 (10·6428, R2=0·87); Pc-04 (18·2143, R2=0·91); Pc-07 (13·2143, R2=0·91); Pc-09 (6·1428, R2=0·92); and Pc-10 (11·6428, R2=0·82).

Fig. 2. Linear regression graph of the percentage of immature Toxocara canis eggs colonized by the isolates Pc-02, Pc-03, Pc-04, Pc-07, Pc-09 and Pc-10 of Pochonia chlamydosporia after 7, 14 and 21 days of interaction at 25°C on pre-colonized cellulose dialysis membrane after inoculation with 500 chlamydospores 7 days prior to the addition of approximately 200 eggs.

Fig. 3. Linear regression graph of the percentage of mature Toxocara canis eggs colonized by the isolates Pc-02, Pc-03, Pc-04, Pc-07, Pc-09 and Pc-10 of Pochonia chlamydosporia after 7, 14 and 21 days of interaction at 25°C on pre-colonized cellulose dialysis membrane after inoculation with 500 chlamydospores 7 days prior to the addition of approximately 200 eggs.
The effect of different chlamydospore concentrations from the P. chlamydosporia isolates on parasitism of immature and mature T. canis eggs during 21 days of interaction is represented graphically in Figs 4 and 5, respectively. The positive trend curves, explained by a linear model, indicated a direct relationship between the variables, i.e. a linear increase in the proportion of colonized eggs with increase in the concentration of chlamydospores (P<0·01). Angular coefficients of the regression curves and R2 for the assay using immature eggs, corresponding to treatments with the P. chlamydosporia isolates, were: Pc-02 (5·5000, R2=0·80); Pc-03 (39·0857, R2=0·87); Pc-04 (5·9000, R2=0·93); Pc-07 (7·1428, R2=0·91); Pc-09 (6·2571, R2=0·82); Pc-10 (7·8571, R2=0·89). Angular coefficients of the regression curves and R2 for the assay using mature eggs, corresponding to treatments with the P. chlamydosporia isolates, were: Pc-02 (4·2285, R2=0·81); Pc-03 (6·6857, R2=0·91); Pc-04 (3·8428, R2=0·83); Pc-07 (4·5428, R2=0·91); Pc-09 (3·8571, R2=0·85); Pc-10 (6·2285, R2=0·89). A significant linear relationship can be observed between variables with more than 80% effect on the average percentage of nematode eggs colonized, which can be explained by the increase in the days of interaction and the increase in the concentration of fungal inoculum.
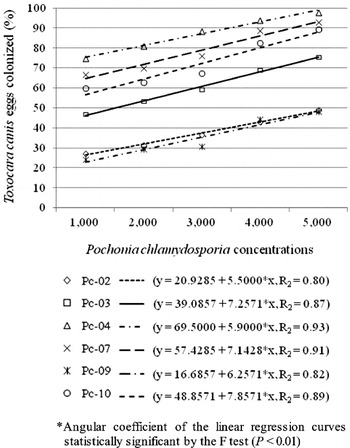
Fig. 4. Linear regression graph of the percentage of immature Toxocara canis eggs colonized by the isolates Pc-02, Pc-03, Pc-04, Pc-07, Pc-09 and Pc-10 of Pochonia chlamydosporia after 21 days of interaction at 25°C on pre-colonized cellulose dialysis membrane after inoculation with 1000, 2000, 3000, 4000 and 5000 chlamydospores 7 days prior to the addition of approximately 200 eggs.
In areas of the cellulose dialysis membrane containing a cluster of eggs there was intense mycelial growth and production of numerous chlamydospores. Immature eggs were more susceptible to infection than mature eggs. The following general descending order of aggressiveness was observed in the tests: Pc-04, Pc-07, Pc-10, Pc-03, Pc-02 and Pc-09. In all assays performed there was no fungal development or infection of eggs on the control plates group, in which there were no changes in morphology and size of eggs. At the end of the 21-day experimental period, the plates of the control group, inoculated with immature eggs, presented 43·85% immature eggs (Fig. 6A), 30·92% in the cell division phase (Fig. 6B) and 25·23% in the mature phase (Fig. 6C). In the treatments, embryonation of a greater number of eggs colonized by the isolates Pc-02 and Pc-09 was observed in comparison to those colonized by the isolates Pc-04, Pc-07 and Pc-10.

Fig. 5. Linear regression graph of the percentage of mature Toxocara canis eggs colonized by the isolates Pc-02, Pc-03, Pc-04, Pc-07, Pc-09 and Pc-10 of Pochonia chlamydosporia after 21 days of interaction at 25°C on pre-colonized cellulose dialysis membrane after inoculation of 1000, 2000, 3000, 4000 and 5000 chlamydospores 7 days prior to the addition of approximately 200 eggs.

Fig. 6. Photomicrographs by differential interference contrast of the evolutionary stages of Toxocara canis eggs occurring over a 21-day incubation period in the dark at 25°C on cellulose dialysis membrane (400× magnification). (A) Unembryonated egg (one-cell stage); (B) embryonating egg (morula stage); (C) embryonated egg (larval stage).
Immature eggs in the early stages of infection (Fig. 7A), in the advanced stage of colonization (Fig. 7B), destroyed by colonization (Fig. 7C and D), larvae colonized within eggs (Fig. 8A), larvae exiting the eggs as a consequence of the shell being weakened by fungal colonization (Fig. 8B) and non-colonized hatched larvae migrating through the membrane (Fig. 8C) were all visualized in all fungal treatments. Hatched colonized larvae (Fig. 9A and B) were visualized in treatments with the isolates Pc-04, Pc-07 and Pc-10. Although immature eggs have also been colonized in the assays with mature eggs, only colonized mature eggs were counted.
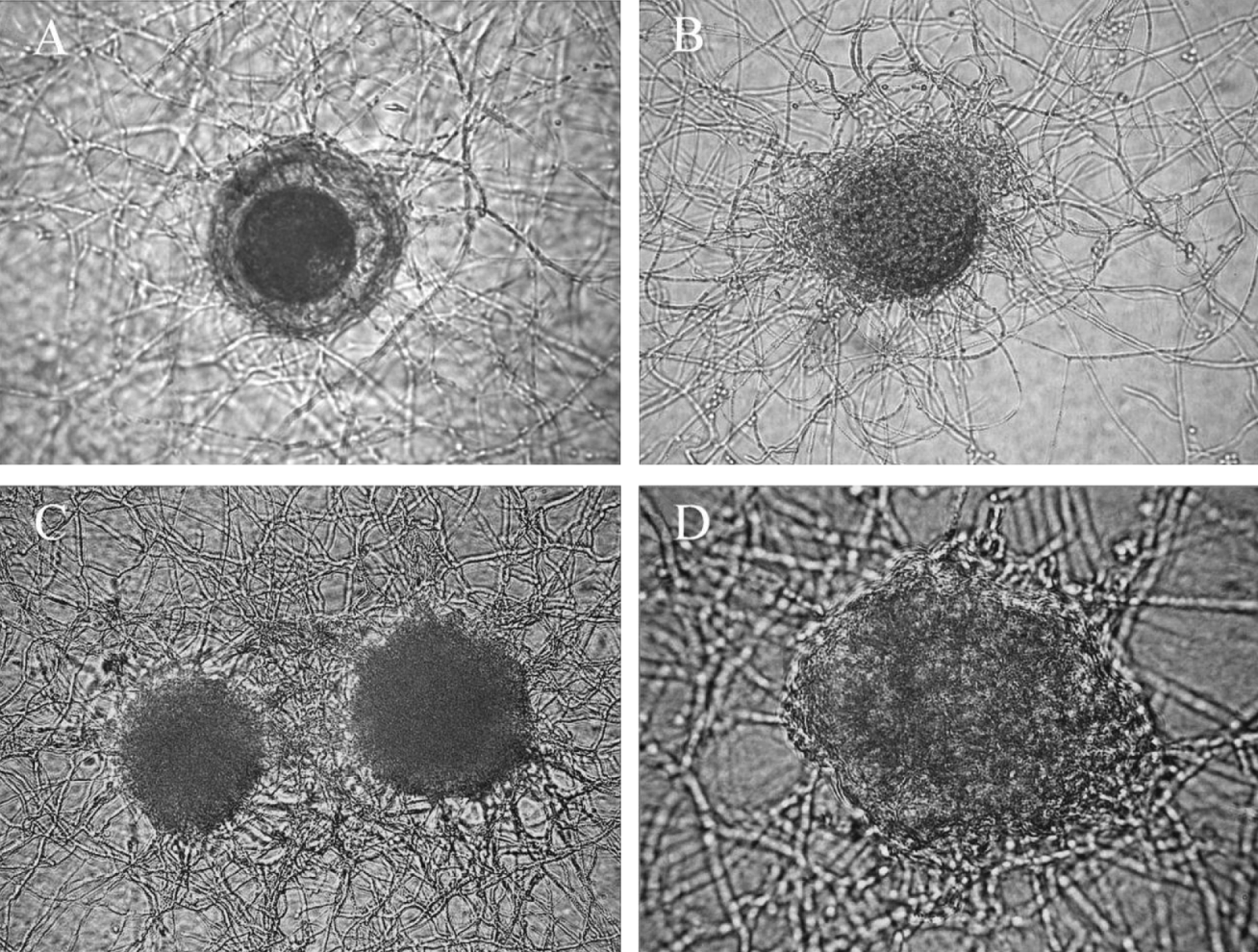
Fig. 7. Photomicrographs by differential interference contrast of infection and colonization of immature Toxocara canis eggs by Pochonia chlamydosporia over a 21-day interaction period on cellulose dialysis membrane in the dark at 25°C. (A) Early infection of the egg (200× magnification); (B) intense mycelial growth around the egg (200× magnification); (C) colonized egg (200× magnification); (D) egg destroyed by colonization (400× magnification).

Fig. 8. Photomicrographs by differential interference contrast of infection and colonization of mature Toxocara canis eggs by Pochonia chlamydosporia over a 21-day interaction period on cellulose dialysis membrane in the dark at 25°C. (A) Larva colonized inside the egg (200× magnification); (B) larva emerging from egg shell weakened by colonization (200× magnification); (C) hatched non-colonized larva migrating over membrane (100× magnification).

Fig. 9. Photomicrographs by differential interference contrast of infection and colonization of mature Toxocara canis eggs by Pochonia chlamydosporia over a 21-day interaction period on a cellulose dialysis membrane in the dark at 25°C. (A) Colonized egg and hatched larvae (200× magnification); (B) colonized hatched larvae (200× magnification).
DISCUSSION
Use of the cellulose dialysis membrane proved to be an efficient and convenient method for visualization of the interaction between the microorganisms studied. All levels of inoculum of P. chlamydosporia tested were associated with colonization of T. canis eggs, but its greatest infective activity, observed in the higher levels, showed that the efficacy of this antagonist is dependent on the concentration used. The concentration of a biological control agent is a prerequisite for its survival in the soil, since this environment is characterized by high competitiveness among microorganisms (Sayre and Walter, Reference Sayre and Walter1991). Therefore, field studies are needed to evaluate the appropriate P. chlamydosporia concentration to be used for control of T. canis. In the control of plant-parasitic nematodes, the inoculum of this nematophagous fungus has been generally applied at a rate of 5000 chlamydospores per gramme of soil (Kerry, Reference Kerry, Butt, Jackson and Magan2001).
Longer contact periods between P. chlamydosporia isolates and T. canis eggs on the cellulose dialysis membrane resulted in more efficient parasitism. This indicates that the efficiency of this fungus on T. canis eggs is also subordinated to the time of interaction, enabling a better antagonistic action on the resistant eggshell belonging to this nematode, and also increasing the chances for infecting a greater number of them. The need for a longer contact period with ascarid eggs for P. chlamydosporia to be more efficient was reported by some researchers (Araújo et al. Reference Araújo, Braga, Milani, Silva and Tavela2008; Carvalho et al. Reference Carvalho, Araújo, Braga, Araújo and Alves2010).
The nematophagous egg-parasitic fungi can infect both immature and mature eggs of plant-parasitic nematodes (Chen and Dickson, Reference Chen, Dickson, Chen, Chen and Dickinson2004; Nordbring-Hertz et al. Reference Nordbring-Hertz, Jansson and Tunlid2006). However, immature eggs, especially in the early development phase, are more easily infected than mature eggs containing second-stage juveniles (Kerry, Reference Kerry, Butt, Jackson and Magan2001). In the present study, the fact that isolates of P. chlamydosporia were more infective on immature T. canis eggs suggests that the susceptibility of this egg phase is not restricted to plant-parasitic nematodes, but is also presented by animal-parasitic nematodes. Parasitism observed on hatched larvae of T. canis by P. chlamydosporia was a divergent behaviour of the fact that nematophagous egg-parasitic fungi are considered to be parasites of immobile stages of the nematodes. To date, colonization of the larvae of a target nematode by P. chlamydosporia, as occurred on T. canis infective larvae, had not yet been reported in the literature on biological control of nematodes. It is likely that such P. chlamydosporia behaviour is related to the slow motion of T. canis larvae on the cellulose dialysis membrane, which allowed the action of this fungal antagonist. There is also the possibility that this hatched larva suffered deleterious fungal action during eggshell degradation that consequently caused its death, and allowed its colonization. Although some T. canis larvae were not destroyed within the eggs, their exit as a consequence of eggshell weakening caused by P. chlamydosporia action also represents an important antagonism exerted by this fungus that would compromise the life cycle of this roundworm because it would not survive in the environment if its outer larval cuticle were to consist of only a single layer that does not provide adequate protection (Brunaská et al. Reference Brunaská, Dubinsky and Reiterova1995).
The long incubation period of T. canis eggs in 2% formalin did not affect embryogenesis, corroborating other studies that used this liquid compound for development of the infective larval stage inside the egg (Alcântara-Neves et al. Reference Alcântara-Neves, Santos, Mendonça, Figueiredo and Pontes-de-Carvalho2008; Ming-Shun et al. Reference Ming-Shun, Chien-Wei, Wen-Yun, Ting-Chang, Kua-Eyre, Yun-Ho, Chun-Chao and Chia-Kwung2008; Watthanakulpanich et al. Reference Watthanakulpanich, Smith, Hobbs, Whalley and Billington2008). According to Levine (Reference Levine1968), T. canis eggs can survive for 8 days in 40% formalin and for a month in 10% formalin. Its resistance to a range of chemicals and environmental conditions is due to the 5 structural layers that make up the eggshell (Aycicek et al. Reference Aycicek, Yarsan, Sarimehmetoglu, Tanyuksel, Girginkardesler and Ozyurt2001). The eggshell of geohelminths such as T. canis provides stable physiological conditions necessary for development of the embryo, allowing it to survive for several years in the soil to which it was exposed (Lysek et al. Reference Lysek, Malinsky and Janisch1985). However, the T. canis eggshell is likely to be damaged by the biological action of P. chlamydosporia, as can be seen in DIC photomicrographs that accurately illustrate the damage it inflicts.
Little is known regarding the infection mechanism of eggs of animal-parasitic nematodes by nematophagous fungi, but it is believed to be similar to the infection of eggs of plant-parasitic nematodes (Lysek and Krajci, Reference Lysek and Krajci1987). This infection process has been extensively studied and its onset occurs with the formation of an appressorium at the tips of trophic hyphae on the surface of the eggshell, which it then penetrates (Nordbring-Hertz et al. Reference Nordbring-Hertz, Jansson and Tunlid2006). This penetration by the appressorium is the result of the joint action of pressure and enzymatic degradation on a small surface of the eggshell (Lopez-Llorca et al. Reference Lopez-Llorca, Maciá-Vicente, Jansson, Ciancio and Mukerji2008). Exo-enzymes produced by P. chlamydosporia, such as VCP1 (protease) and CHI43 (chitinase), play an important role in this infection process degrading the eggshell and body wall of juvenile nematodes, serving as virulence factors (Tikhonov et al. Reference Tikhonov, Lopez-Llorca, Salinas and Jansson2002; Huang et al. Reference Huang, Zhao and Zhang2004), and they, along with other enzymes, may be involved in the infection of T. canis eggs.
Partial degradation of the vitelline layer by the enzyme VCP1 is the main mode of action of P. chlamydosporia on the egg surface, exposing the chitin wall (Segers et al. Reference Segers, Butt, Keen, Kerry, Beckett and Peberdy1996). After the vitelline layer is traversed by fungal hyphae, enzymatic dissolution of the chitin and lipid layers of the nematode eggshell occurs (Morgan-Jones et al. Reference Morgan-Jones, White and Rodrígues-Kábana1983). In the present study, it is possible that differences in aggressiveness of the P. chlamydosporia isolates tested against T. canis eggs may be related to differences in the levels of chitinase activity produced by each isolate as suggested by Zhang et al. (Reference Zhang, Wu and Cai2009) when observing different rupture rates in Meloidogyne incognita eggs caused by P. chlamydosporia isolates with different chitinase activities. Such differences between isolates of the same genus showed the need to carry out preliminary tests to select fungal agents with the greatest potential for biological control of parasitic nematodes, justifying our study. Besides ovicidal capacity, parameters such as speed of growth, saprophytic ability and production of chlamydospores should also be taken into consideration in the selection of biocontrol agents (Abrantes et al. Reference Abrantes, Santos, Bourne, Ciancio, Lopez-Llorca, Kerry, Mota, Tzortzakakis, Verdejo-Lucas, Kerry and Bourne2002). Complex interactions involving abiotic and biotic factors occur in the soil and may limit the action of nematophagous fungi isolates such as P. chlamydosporia (Monfort et al. Reference Monfort, Lopez-Llorca, Jansson and Salinas2006) and therefore the isolates of higher performance selected in laboratory tests should be evaluated repeatedly in environmental conditions before being considered suitable biological control agents of animal-parasitic geohelminths. This will be the topic of a subsequent study using the isolate Pc-04.
Female specimens of T. canis used in the present study were obtained by oral administration of drugs commonly used for deworming infected dogs. Viability of the eggs extracted was not reduced, since a large percentage of these eggs became embryonated in the control group without fungi, under the experimental conditions adopted. This lack of damage to the T. canis eggs corroborates with other studies that used conventional anthelmintic drugs to promote the release of adult worms from dogs in order to obtain eggs (Pecinali et al. Reference Pecinali, Gomes, Amendoeira, Pereira Bastos, Martins, Pegado, Pereira Bastos, Bozza and Castro Faria Neto2005; Pinelli et al. Reference Pinelli, Brandes, Dormans, Fonville, Hamilton and Van Der Giessen2007; Jin et al. Reference Jin, Akao and Ohta2008). This validates the conclusion that these drugs would not affect the development of T. canis eggs in the environment under favourable conditions and therefore the feces with adult worms of wormed animals should be collected and destroyed to avoid soil contamination.
The strategy of transmission and survival of T. canis, characterized by high fertility of the females and extreme resistance of the eggs for long periods in soil, indicates that there is an accumulation of infective eggs in the soil over the years. The isolate Pc-04 of P. chlamydosporia has great capacity to destroy T. canis eggs on cellulose dialysis membranes under laboratory conditions. However, field studies should be conducted to verify whether it plays an important role in reducing the environmental burden with T. canis eggs, and consequently reduce the incidence of toxocariasis in definitive and paratenic hosts.
ACKNOWLEDGEMENTS
The authors thank the National Council for Scientific and Technological Development (CNPq), an agency dedicated to the promotion of scientific and technological research and to the formation of human resources for research in Brazil, for its financial support and the Federal University of Viçosa (UFV) for its professionalism and competence in developing education, research and extension, without whom this work would not have been accomplished.