1. Introduction
The Triassic Period (252–201 Ma) remains an intriguing time interval that witnessed both unbridled eruptions of life, exemplified by the towering coral reefs that are preserved in the Northern Calcareous Alps of Austria, as well as utter devastation during two of the largest mass-extinction events of the past 600 million years that wiped out ecosystems on a global scale. Of these two extinction events the end-Permian mass-extinction (EPME) is the largest, terminating many Palaeozoic lineages of life at the same time as heralding the advent of Mesozoic organisms and laying the foundations for the so-called Modern Fauna (Sepkoski Jr, Reference Sepkoski1987, Reference Sepkoski and Walliser1996; Erwin, Reference Erwin1993). The end-Triassic mass-extinction (ETME), albeit smaller in numbers of extinct genera (Bambach, Reference Bambach2006), led to equally dramatic changes in ecosystem composition and structure and altered sedimentation style in basins around the world for millions of years (Hallam, Reference Hallam and Walliser1993; van de Schootbrugge et al. Reference van de Schootbrugge, Bachan, Suan, Richoz and Payne2013).
It is impossible to briefly summarize the many intricacies of the changes in biodiversity associated with both extinction events; however, broad trends can be described. Both extinction events led to near-annihilation of cnidarian clades and other taxa responsible for reef construction, resulting in ‘reef gaps’ that lasted millions of years (Stanley, Reference Stanley1988, Reference Stanley2003; Stanley & Beauvais, Reference Stanley and Beauvais1994). Other clades of marine invertebrates, such as molluscs including ammonoids, gastropods and bivalves, were hard hit in both instances (Kiessling et al. Reference Kiessling, Aberhan, Brenneis and Wagner2007). Following the end-Triassic extinction, Early Jurassic shallow seas witnessed recurrent euxinia over a time span of 25 million years, culminating in the Toarcian Oceanic Anoxic Event (Richoz et al. Reference Richoz, de Schootbrugge, Pross, Püttmann, Quan, Lindström, Heunisch, Fiebig, Maquil, Hauzenberger, Schouten and Wignall2012; van de Schootbrugge et al. Reference van de Schootbrugge, Bachan, Suan, Richoz and Payne2013). Widespread euxinia also characterized shallow-marine settings bordering the Tethys Ocean in the direct aftermath of the end-Permian extinction (Wignall & Hallam, Reference Wignall and Hallam1992; Grice et al. Reference Grice, Cao, Love, Böttcher, Twitchett, Grosjean, Summons, Turgeon, Dunning and Jin2005a ). Black shales deposited across both extinction events are characterized by an abundance of fossilized pigments that derive from green sulphur bacteria, indicating that euxinic conditions developed close to the surface of the oceans (Grice et al. Reference Grice, Cao, Love, Böttcher, Twitchett, Grosjean, Summons, Turgeon, Dunning and Jin2005a ; Richoz et al. Reference Richoz, de Schootbrugge, Pross, Püttmann, Quan, Lindström, Heunisch, Fiebig, Maquil, Hauzenberger, Schouten and Wignall2012). On land, forest dieback during both events was accompanied by the proliferation of opportunists and pioneers, including ferns and fern allies (Looy et al. Reference Looy, Twitchett, Dilcher, van Konijnenburg-Van Cittert and Visscher2001; van de Schootbrugge et al. Reference van de Schootbrugge, Quan, Lindström, Püttmann, Heunisch, Pross, Fiebig, Petschick, Röhling, Richoz, Rosenthal and Falkowski2009). Furthermore, both events led to major schisms in the dominant terrestrial herbivores (Benton, Reference Benton1986; Benton, Tverdokhlebov & Surkov, Reference Benton, Tverdokhlebov and Surkov2004) and apex predators, including the late Permian extinction of the pariaeosaurs and many dicynodonts and the end-Triassic loss of crurotarsans (Olsen et al. Reference Olsen, Kent, Sues, Koeberl, Huber, Montanari, Rainforth, Fowell, Szajina and Hartline2002).
A considerable number of reviews and books on either the end-Permian or the end-Triassic have been written over the past 20 years (Erwin, Reference Erwin1993; Knoll et al. Reference Knoll, Bambach, Canfield and Grotzinger1996; Hallam & Wignall, Reference Hallam and Wignall1997; Benton & Twitchett, Reference Benton and Twitchett2003; Tanner, Lucas & Chapman, Reference Tanner, Lucas and Chapman2004; Hesselbo, McRoberts & Palfy, Reference Hesselbo, McRoberts and Palfy2007; Algeo et al. Reference Algeo, Chen, Fraiser and Twitchett2011; Payne & Clapham, Reference Payne and Clapham2012; Benton & Newell, Reference Benton and Newell2014; Wignall, Reference Wignall2015), summarizing a rapidly growing body of work on either extinction event. Here, we synthesize and compare the recent work on both the end-Permian and end-Triassic extinction events. Starting with the main premise that both extinction events were triggered by the emplacement of large igneous provinces, we focus on a comparison of kill mechanisms resulting from flood basalt volcanism. The purpose of such a review is to detect similarities and/or pronounced differences that can promote an understanding of either event. Moreover, gaps in our understanding of specific aspects, or lack of data, can be brought to light.
Large-scale volcanism or extraterrestrial impacts are widely considered to have been the two main extinction triggers of mass extinctions that set in motion a chain of adverse environmental and biotic changes, the so-called proximate kill mechanisms. An extinction trigger for the EPME that has been discussed by a relatively small number of authors is the role of extraterrestrial impacts, in analogy to scenarios for the Cretaceous–Palaeogene extinction. However, there is currently no conclusive evidence for bolide impact at or near the end-Permian extinction horizon. Claims for the presence of extraterrestrial fullerenes at the Permian–Triassic boundary (Becker et al. Reference Becker, Poreda, Hunt, Bunch and Rampino2001), chondritic fragments present in a section in Antarctica (Basu et al. Reference Basu, Petaev, Poreda, Jacobsen and Becker2003) and a supposed impact crater at Bedout (Western Australia; Becker et al. Reference Becker, Poreda, Basu, Pope, Harrison, Nicholson and Iasky2004) have neither been replicated, accepted nor confirmed in any other work. The most important argument against an extra-terrestrial impact comes from the fossil record. The stepped nature of the end-Permian extinction with losses beginning in the latest Permian and continuing into the Triassic (Algeo et al. Reference Algeo, Kuwahara, Sano, Bates, Lyons, Elswick, Hinnov, Ellwood, Moser and Maynard2011; Song et al. Reference Song, Wignall, Tong and Yin2013) would require multiple giant impacts. Recent calibration of the end-Permian extinction indicates it climaxed around 252.3 Ma, spanning at least several hundreds of thousands of years (Shen et al. Reference Shen, Crowley, Wang, Bowring, Erwin, Sadler, Cao, Rothman, Henderson, Ramezani, Zhang, Shen, Wang, Wang, Mu, Li, Tang, Liu, Liu, Zeng, Jiang and Jin2011a ). Statistical treatment of last occurrences from a number of sections across China also suggest that conditions started to deteriorate 1.2 million years before the extinction horizon (Wang et al. Reference Wang, Sadler, Shen, Erwin, Zhang, Wang, Wang, Crowley and Henderson2014). Such findings are difficult to reconcile with a single catastrophic event.
Debates on the relationship between Late Triassic impacts and the end-Triassic extinction have continued to flare up. A number of impact craters have ages close to the Triassic–Jurassic boundary, but none have a size that is large enough to be considered as an impactor driving extinction (Fig. 1). The hypothesis of ‘multiple Late Triassic impacts’ centred on 214 Ma (Spray, Kelley & Rowley, Reference Spray, Kelley and Rowley1998) can be confidently put to rest based on improved dating of several of the craters (Schmieder et al. Reference Schmieder, Jourdan, Tohver and Cloutis2014), which conclusively shows that the impacts occurred over an interval of more than 50 million years rather than hours as suggested by Spray, Kelley & Rowley (Reference Spray, Kelley and Rowley1998). The Obolon crater in Ukraine was recently re-dated by Gurov et al. (Reference Gurov, Gurova, Chernenko and Yamnichenko2009) to have a Bajocian age (169±7 Ma), whereas the Lake Saint Martin crater was dated to be of Carnian age (227± 0.9 Ma) by Schmieder et al. (Reference Schmieder, Jourdan, Tohver and Cloutis2014). The most close in age, the Rochechouart crater in France with a diameter of c. 25 km, was recently re-dated to 202.8±2 Ma (Schmieder et al. Reference Schmieder, Buchner, Schwarz, Trieloff and Lambert2010), overlapping with the end-Triassic extinction. Its size is modest and of the same order as other impacts however, such as the Miocene Nördlinger Ries-Steinheim binary impact in southern Germany that had no noticeable effect on global biodiversity (Böhme, Gregor & Heissig, Reference Böhme, Gregor, Heissig, Buffetaut and Koeberl2002).

Figure 1. Overview of radiometric dated occurrences of impact craters (modified after Schmieder et al. Reference Schmieder, Jourdan, Tohver and Cloutis2014) and flood basalt flows, sills and dykes (Siberian Traps: Reichow et al. Reference Reichow, Pringle, Al’Mukhamedov, Allen, Andreichev, Buslov, Davies, Fedoseev, Fitton, Inger, Medvedev, Mitchell, Puchkov, Safonova, Scott and Saunders2009; CAMP: Marzoli et al. Reference Marzoli, Jourdan, Puffer, Cuppone, Tanner, Weems, Bertrand, Cirilli, Bellieni and De Min2011; Blackburn et al. Reference Blackburn, Olsen, Bowring, McLean, Kent, Puffer, McHone, Rasbury and Et-Touhami2013). The age for the Norian–Rhaetian boundary (205.3 Ma) is modified after Wotzlaw et al. (Reference Wotzlaw, Guex, Bartolini, Gallet, Krystyn, McRoberts, Taylor, Schoene and Schaltegger2014). The timescale was created with the free shareware TimescaleCreator v6.3.
Evidence that could link Rochechouart or other (undiscovered) craters with the end-Triassic extinction has been scarce. Findings of shocked quartz in a number of shale beds in Italy (Bice et al. Reference Bice, Newton, McCauley, Reiners and McRoberts1992) have not been confirmed or replicated in other sections. A modest iridium anomaly (in the range of parts per trillion) in the Newark Basin that co-occurs with abundant fern spores has been attributed to an impact (Olsen et al. Reference Olsen, Kent, Sues, Koeberl, Huber, Montanari, Rainforth, Fowell, Szajina and Hartline2002); however, it is increasingly doubtful whether this level really represents the end-Triassic extinction event (Wotzlaw et al. Reference Wotzlaw, Guex, Bartolini, Gallet, Krystyn, McRoberts, Taylor, Schoene and Schaltegger2014), or if it corresponds to a precursor event (Cirilli et al. Reference Cirilli, Marzoli, Tanner, Bertrand, Buratti, Jourdan, Bellieni, Kontak and Renne2009). In fact, no ‘smoking gun’ has been found to implicate a large bolide impact as the cause of the end-Triassic extinction. Small iridium spikes in the Upper Triassic Blomidon Formation in Nova Scotia (Canada) in proximity to a large basalt flow are likely products of volcanic eruptions (Tanner, Kyte & Walker, Reference Tanner, Kyte and Walker2008), whereas in Austria clay spherules at the top of the regionally significant Rhaetian Kössen Formation have also been interpreted to originate from volcanic fall-out (Zajzon et al. Reference Zajzon, Kristaly, Palfy and Nemeth2012). Neither of these studies reported on co-occurring shocked-quartz minerals or any other bona fide extra-terrestrial impact indicators. Despite intensive searches other authors have failed to detect iridium, for example in marginal-marine to continental strata in Poland (Pienkowski, Niedzwiedzki & Waksmundzka, Reference Pienkowski, Niedzwiedzki and Waksmundzka2012) as well as shallow-marine strata in Denmark (Sofie Lindström, pers. comm. 2014).
Finally, widespread soft sediment deformations in sections across the UK were initially attributed to a single impact event, presumably in the Irish Sea (Simms, Reference Simms2003). However, where sections are more complete the seismites are separated by undisturbed beds, suggesting a number of large earthquakes (Lindström et al. Reference Lindström, Pedersen, van de Schootbrugge, Hansen, Kuhlmann, Thein, Johansson, Petersen, Awlmark, Dybkjaer, Weibel, Erlstrom, Nielsen, Oschmann and Tegner2015). There is in fact evidence for synsedimentary tectonic activity from the same sections (Wignall & Bond, Reference Wignall and Bond2008), for example at Pinhay Bay where the upper Rhaetian deposits contain a thick debrite bed, and evidence for polygonal faults that have deformed the lowermost Jurassic Blue Lias sediments (Fig. 2).
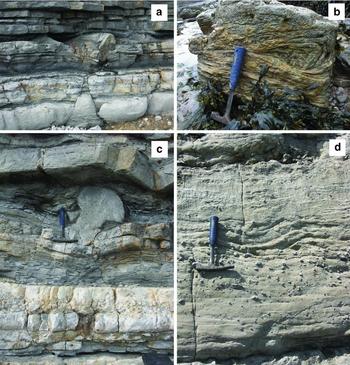
Figure 2. (a) Synsedimentary tectonics within the base of the Blue Lias Formation at Pinhay Bay (close to Lyme Regis). Compare figure 3 of Wignall (Reference Wignall2001b ). Small polygonal fault sets leading to soft-sediment deformation. (b) Slump folds within the Cotham Member of the Lilstock Formation on the foreshore of St Audrie's Bay. (c) Soft sediment deformation within the base of the Blue Lias Formation at Pinhay Bay (close to Lyme Regis). Compare figure 3 of Wignall (Reference Wignall2001b ). (d) Calcareous debris-flow bed within the upper part of the Cotham Member. Compare figure 3 of Wignall (Reference Wignall2001b ).
The evidence for widespread seismites in the Rhaetian of NW Europe could be linked to the emplacement of a large igneous province. Instead of extra-terrestrial bolide impacts, both the end-Permian and end-Triassic extinction events appear to be Earth-bound disasters. These are both coeval with the emplacement of two of the largest flood basalt provinces of Phanerozoic time, seen by most workers as the principal trigger for environmental changes leading to mass extinction (Courtillot, Reference Courtillot1994; Wignall, Reference Wignall2001a ; Palfy, Reference Palfy, Hames, Mchone, Renne and Ruppel2003; Svensen et al. Reference Svensen, Planke, Polozov, Schmidbauer, Corfu, Podladchikov and Jamtveit2009; Payne & Clapham, Reference Payne and Clapham2012; Bond & Wignall, Reference Bond, Wignall, Keller and Kerr2014). Radiometric dating of zircons obtained from ash beds close to the biostratigraphically defined Permian–Triassic boundary in the global stratotype section and point (GSSP) of Meishan in China (Bowring et al. Reference Bowring, Erwin, Jin, Martin, Davidek and Wang1998; Burgess, Bowring & Shen, Reference Burgess, Bowring and Shen2014) and from basalt flows in the Siberian Traps large igneous province (LIP) are virtually indistinguishable (Reichow et al. Reference Reichow, Pringle, Al’Mukhamedov, Allen, Andreichev, Buslov, Davies, Fedoseev, Fitton, Inger, Medvedev, Mitchell, Puchkov, Safonova, Scott and Saunders2009). Volcanism was intense during earliest Triassic time, but there are radiometric dates of younger phases in locations such as Chelyabinsk (Fig. 1).
The emplacement of the Central Atlantic Magmatic Province figures prominently in scenarios for the end-Triassic extinction (Palfy, Reference Palfy, Hames, Mchone, Renne and Ruppel2003; Nomade et al. Reference Nomade, Knight, Beutel, Renne, Verati, Feraud, Marzoli, Youbi and Bertrand2006; Hesselbo, McRoberts & Palfy, Reference Hesselbo, McRoberts and Palfy2007). Despite this general consensus, the exact timing of flood basalt eruptions and its impact on ecosystems remain to be firmly established. Central Atlantic Magmatic Province (CAMP) extrusives have been thought to straddle the Triassic–Jurassic boundary (Marzoli et al. Reference Marzoli, Renne, Piccirillo, Ernesto, Bellieni and De Min1999, Reference Marzoli, Bertrand, Knight, Cirilli, Buratti, Verati, Nomade, Renne, Youbi, Martini, Allenbach, Neuwerth, Rapaille, Zaninetti and Bellieni2004) and the eruptions estimated to have taken place over an interval of not less than 600 ka but not more than 1 Ma (Knight et al. Reference Knight, Nomade, Renne, Marzoli, Bertrand and Youbi2004; Nomade et al. Reference Nomade, Knight, Beutel, Renne, Verati, Feraud, Marzoli, Youbi and Bertrand2006). Improved dating of the actual Triassic–Jurassic boundary in sections in Peru that contain ash beds calibrated with ammonite stratigraphy place the boundary at 201.36±0.17 Ma (Schaltegger et al. Reference Schaltegger, Guex, Bartolini, Schoene and Ovtcharova2008; Schoene et al. Reference Schoene, Guex, Bartolini, Schaltegger and Blackburn2010). This age is indistinguishable from the age of the North Mountain Basalt, the oldest basalt flow in the Fundy Basin (Marzoli et al. Reference Marzoli, Jourdan, Puffer, Cuppone, Tanner, Weems, Bertrand, Cirilli, Bellieni and De Min2011). At least four major pulses of sill and dyke emplacement as well as fissure eruptions have been dated to occur directly following the biostratigraphically defined Triassic–Jurassic boundary in the NE United States (Blackburn et al. Reference Blackburn, Olsen, Bowring, McLean, Kent, Puffer, McHone, Rasbury and Et-Touhami2013) and Morocco (Knight et al. Reference Knight, Nomade, Renne, Marzoli, Bertrand and Youbi2004).
While the correspondence between the onset of CAMP volcanism and the Triassic–Jurassic boundary is certain, it is important to note that most extinction losses pre-date the period boundary. However, there is indirect evidence for volcanism prior to the onset of flood basalts in Morocco where mudstones derived from weathering of mafic volcanic rocks underlie the first lava flows (Dal Corso et al. Reference Dal Corso, Marzoli, Tateo, Jenkyns, Bertrand, Youbi, Mahmoudi, Font, Buratti and Cirilli2014). It is possible that volcanism may have occurred even earlier in undated and little explored parts of the volcanic province, such as in the Amazon Basin. Proxy evidence for eruptions starting during the early Rhaetian Stage is provided by a decline in the 187Os/188Os at this time (Kuroda et al. Reference Kuroda, Hori, Suzuki, Gröcke and Ohkouchi2010).
2. The atmosphere
2.a. Atmospheric pollution
The most immediate effect of volatile release from flood basalt volcanism would have been changes in atmospheric chemistry, with regional to global deleterious effects on terrestrial ecosystems and organisms from plants to herbivores. The example of the Laki Fissure eruption of 1783–84 is a powerful reminder of the way in which even small events can have serious consequences. Following the Laki eruption, the severe loss of livestock on Iceland was attributed to the release of large amounts of fluorine and chlorine (Grattan, Reference Grattan2005). Even larger amounts of sulphur dioxide reached continental Europe as a sulphuric haze, leading to failed crops and unusual summer weather (Chenet, Fluteau & Courtillot, Reference Chenet, Fluteau and Courtillot2005). The eruption lasted for several months and produced about 12 km3 of basalt, which is several orders of magnitude smaller than the individual eruptions that formed the Siberian Traps and CAMP large igneous provinces. Based on melt inclusions from Siberian Traps flood basalts, Black et al. (Reference Black, Lamarque, Shields, Elkins-Tanton and Kiehl2013) presented a compelling case for previously underestimated volatile loads. Not only did the Siberian Traps release large amounts of sulphur (c. 6300–7800 Gt S), but vast amounts of chlorine (c. 3400–8700 Gt Cl) and fluorine (c. 7100–13600 Gt F) were also released.
Even though activity within the Siberian Traps and CAMP was spread out over several hundreds of thousands of years, climactic phases are thought to have been brief, coincident with the rapid emplacement of large volumes of basalt both as sills and dykes and large surface flows (Knight et al. Reference Knight, Nomade, Renne, Marzoli, Bertrand and Youbi2004). Precise estimates of fluorine and chlorine are not available for CAMP basalts, but SO2 release was recently evaluated by Callegaro et al. (Reference Callegaro, Baker, De Min, Marzoli, Geraki, Betrand, Viti and Nestola2014) based on melt inclusions. Results from that study show that CAMP flood basalts could have released up to 8 Mt of SO2 per km3 of erupted basalts or up to 8000 Gt for the entire province, placing CAMP emissions within the same range as the Siberian Traps.
Sulphur dioxide released to the atmosphere quickly combines with hydroxyl (OH−) and oxygen to form sulphur trioxide which, after reaction with water, leads to sulphuric acid droplets. These have a cooling effect in the troposphere, and rain out as acid rain after only weeks to months. The effect of SO2 release on the atmosphere and subsequent cooling events were therefore short-lived. In contrast, the impact of sulphur deposition on soils and vegetation was likely more severe. Sulphur deposition in the form of acid rain has been invoked for the end-Permian extinction based on high sulphide concentrations in lacustrine sediments in the Karoo Basin (South Africa; Maruoka et al. Reference Maruoka, Koeberl, Hancox and Reimold2003) and from the presence of soil-pH-sensitive vanillin molecules in Italian boundary shales (Sephton et al. Reference Sephton, Jiao, Engel, Looy and Visscher2015). Evidence for acid rain related to CAMP eruptions is unfortunately even more circumstantial. A peculiar darkening of pollen and spores recovered from Triassic–Jurassic boundary sediments in Germany, Denmark and Poland has been tentatively attributed to soil acidification (van de Schootbrugge et al. Reference van de Schootbrugge, Quan, Lindström, Püttmann, Heunisch, Pross, Fiebig, Petschick, Röhling, Richoz, Rosenthal and Falkowski2009; Pienkowski, Niedzwiedzki & Branski, Reference Pienkowski, Niedzwiedzki, Branski, Keller and Kerr2014).
The effects of widespread acid rain on fresh water and forest ecosystems as well as individual plant species have been studied in great detail during the last decades of the twentieth century, when large tracts of North American and European forests started to suffer from industrial emissions of sulphur dioxide from coal-fired power plants (Bricker & Rice, Reference Bricker and Rice1993). Acid rain triggered a complex suite of changes during the 1970s and 80s that led to widespread forest dieback, especially in the so-called ‘Black Triangle’ at the borders of Germany, Czech Republic and Poland (Ulrich, Reference Ulrich1990). Intense research concluded that the most important result of acid rain was to alter soil conditions leading to the leaching of the essential cations Ca, K and Mg, which would then be quickly lost during rainfall events (Tomlinson, Reference Tomlinson2003). In addition, severe damage to root systems, due to lowered soil pH, constituted a further weakening of the affected pine forests (Tomlinson, Reference Tomlinson2003). Discolouration and loss of leaves/needles was also observed and attributed to direct impact of acid rain on the canopy (Evans, Reference Evans1984).
Volcanic sulphuric hazes and acid rain likely constituted proximate kill mechanisms for plants during both the end-Permian and end-Triassic extinction events. The effects of direct fumigation of plants with SO2 has been studied both in the field, for example in Hawaii (Tanner, Smith & Allan, Reference Tanner, Smith and Allan2007; Winner & Mooney, Reference Winner and Mooney1980a , Reference Winner and Mooney b ), as well as in mesocosm experiments (Elliott-Kingston, Haworth & McElwain, Reference Elliott-Kingston, Haworth and McElwain2014). Pines growing in the vicinity of sulphur degassing fumaroles at Campi Flegrei (southern Italy) showed significant damage to epicuticular waxes (Bartiromo et al. Reference Bartiromo, Guignard, Barone Lumaga, Barattolo, Chiodini, Avino, Guerriero and Barale2012), while fumigation of ten species of plants representative of Mesozoic conifer and fern vegetation showed consistent damage to epicuticular waxes in all specimens (Elliott-Kingston, Haworth & McElwain, Reference Elliott-Kingston, Haworth and McElwain2014). Other effects included the appearance of lesions on the stomata and distortions to the stomatal complex. Mesocosm experiments in China, a country currently heavily affected by SO2 pollution from domestic and industrial coal burning, showed that the air concentration of SO2 is more important in damaging crops than the duration of fumigation (Cao, Reference Cao1989). In controlled experiments, plant damage included a reduction in chlorophyll, inhibition of photosynthesis and a damage to plant tissues related to the presence of free oxygen radicals (Cao, Reference Cao1989). Translated to the end-Permian or end-Triassic, this would mean that brief pulses of intense flood basalt activity with high levels of sulphur dioxide emissions would be more devastating than slow long-term degassing of basalts.
In addition to gaseous volatiles, other pollutants may have had a dramatic impact on both the atmosphere and biosphere beneath it. It remains unclear whether, in the early stages of LIP emplacement, fire fountains could have been violent enough to directly inject volatiles into the stratosphere where their effect would be global rather than regional (Self et al. Reference Self, Widdowson, Thordarson and Jay2006). If so, bromine and chorine complexation with organic molecules (organohalogens) may have damaged the ozone layer, potentially leading to dangerously high levels of UV-radiation (Visscher et al. Reference Visscher, Looy, Collinson, Brinkhuis, van Konijnenburg-Cittert, Kürschner and Sephton2004). Model runs by Beerling et al. (Reference Beerling, Harfoot, Lomax and Pyle2007) indicate that collapse of the ozone layer would have been possible, assuming large amounts of chlorinated organics (e.g. ClCH3) were generated via intrusion of Siberian Traps magma into evaporites and organic-rich strata (Black et al. Reference Black, Lamarque, Shields, Elkins-Tanton and Kiehl2013).
Mutagenesis observed in the Lower Triassic herbaceous lycopsid Isoetales has been attributed to increased levels of UV-radiation under low ozone conditions (Visscher et al. Reference Visscher, Looy, Collinson, Brinkhuis, van Konijnenburg-Cittert, Kürschner and Sephton2004). The widespread occurrence of unseparated Isoetales-tetrads of the form genera Kraeuselisporites, Lundbladispora and Uvaesporites has been linked to genetic mutation either inhibiting successful reproduction or signalling the ability of lycopsids to adapt to drought-prone habitats and reproduce asexually (Visscher et al. Reference Visscher, Looy, Collinson, Brinkhuis, van Konijnenburg-Cittert, Kürschner and Sephton2004). Mutations also appear evident in pollen. Aberrant pollen, such as trisaccate forms of normally bisaccate pollen, have been described from several sections straddling the Permian–Triassic boundary in Russia and China (Foster & Afonin, Reference Foster and Afonin2005; Metcalfe et al. Reference Metcalfe, Foster, Afonin, Nicoll, Mundil, Xiaofeng and Lucas2009).
Visscher et al. (Reference Visscher, Looy, Collinson, Brinkhuis, van Konijnenburg-Cittert, Kürschner and Sephton2004) alluded to the fact that the end-Triassic extinction was equally associated with abundant unseparated tetrads belonging to Kraeuselisporites reissingerii, again derived from a lycopsid parent plant. A distinct early Hettangian spike in tetrads was noted and quantified in sections from Austria (Bonis, Kürschner & Krystyn, Reference Bonis, Kürschner and Krystyn2009), Germany (van de Schootbrugge et al. Reference van de Schootbrugge, Quan, Lindström, Püttmann, Heunisch, Pross, Fiebig, Petschick, Röhling, Richoz, Rosenthal and Falkowski2009; Heunisch et al. Reference Heunisch, Luppold, Reinhardt and Röhling2010) and Denmark and Sweden (Lindström et al. Reference Lindström, van de Schootbrugge, Dybkjaer, Pedersen, Fiebig, Nielsen and Richoz2012). Mutagenesis of Classopollis-pollen (Kürschner, Batenburg & Mander, Reference Kürschner, Batenburg and Mander2013), particularly the occurrence of malformed tetrads, has also been discussed as evidence for environmental stress. Previously unpublished data by one of the authors (BvdS) from cores in Germany and Luxemburg indicates the presence of teratological tetrads and aberrant pollen in lowest Hettangian deposits (Fig. 3), but further work is needed to quantify the occurrences. Ricciisporites tuberculatus, an enigmatic gymnosperm unseparated pollen-tetrad, likely represents another possible pollen taxon that shows aberrant morphology in the form of its bizarre and highly variable wart-like wall structure. Ricciisporites (and its pollen-producing parent plant) became one of the most prominent victims of the end-Triassic extinction event across NW Europe (van de Schootbrugge et al. Reference van de Schootbrugge, Quan, Lindström, Püttmann, Heunisch, Pross, Fiebig, Petschick, Röhling, Richoz, Rosenthal and Falkowski2009; personal observations).
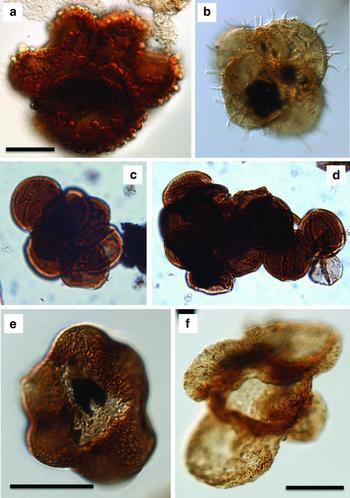
Figure 3. Aberrant pollen and spores from the end-Triassic extinction interval (scale bars are 20 μm). (a) Ricciisporites tuberculatus from the uppermost Rhaetian deposits at Larne (Northern Ireland). This enigmatic gymnosperm pollen that has distinct and highly variable surface ornamentation became one of the major victims of the ETME. (b) Kraeuselisporites reissingerii from the lowermost Hettangian deposits of Larne. These lycopsid spore-tetrads proliferate in the direct aftermath of the end-Triassic extinction, similar to that observed for the end-Permian mass extinction. The unseparated tetrads are possibly reflecting increased environmental stress. (c, d) Classopollis torosus tetrads from the lowermost Hettangian deposits in the Schandelah-1 core from north Germany. These unseparated tetrads consisting of more than 4 pollen grains likely reflect an environmental stress response. Unreduced (polyploid) Classopollis-tetrads have been interpreted by (Kürschner, Batenburg & Mander, Reference Kürschner, Batenburg and Mander2013) to explain the success of cheirolepid conifers following the ETME. (e) Trisaccate version of a normally bisaccate Vitreisporites pallidus from Rhaetian sediments in the Schandelah-1 core. (f) Quadrisaccate version of a normally bisaccate pollen grain related to Pinuspollenites minimus from the lowermost Hettangian section in the Rosswinkel core from Luxemburg.
Sulphur dioxide was perhaps the most abundant toxin released but smaller quantities of other more toxic pollutants, such as heavy metals and polycyclic aromatic hydrocarbons (PAHs), likely had a deleterious impact on terrestrial ecosystems. High concentrations of mercury (Hg) have been detected in Permian–Triassic sections in the Sverdrup Basin (Canada; Grasby, Sanei & Beauchamp, Reference Grasby, Sanei and Beauchamp2011). The only plausible source of these highly toxic metals were the Siberian Traps. Mercury may have adsorbed onto coal fly-ash particles generated during subsurface thermal metamorphism of coal beds (Grasby, Sanei & Beauchamp, Reference Grasby, Sanei and Beauchamp2011; Grasby et al. Reference Grasby, Beauchamp, Bond, Wignall and Sanei2015). It is increasingly clear that the lethal effects of large igneous provinces are strongly enhanced when lava metamorphoses crustal rocks during ascension, particularly during sill emplacement (Ganino & Arndt Reference Ganino and Arndt2009). The potential to generate additional large quantities of greenhouse gases CO2 and CH4, and pollutants including SO2, largely depends on the subsurface presence of sediments rich in coal, organic matter, petroleum or evaporites. In the Tunguska Basin in Siberia, large kilometre-wide volcanic breccia pipes occur throughout the Siberian Traps province; the effects of the Siberian Traps volcanism may have been greatly exacerbated by lava intrusions into Palaeozoic hydrocarbon reservoirs (Svensen et al. Reference Svensen, Planke, Polozov, Schmidbauer, Corfu, Podladchikov and Jamtveit2009).
For the CAMP, the relationship between thermogenesis and volcanism is harder to demonstrate. Intrusion into Permian evaporites in the Amazon Basin is suspected but has not been conclusively demonstrated (Svensen et al. Reference Svensen, Planke, Polozov, Schmidbauer, Corfu, Podladchikov and Jamtveit2009). In the Deep River and Sanford basins in North Carolina (United States) dykes and sills belonging to the CAMP are in contact with Carnian coals, where a substantial volume of coal has been transformed into natural cokes (Reinemund, Reference Reinemund1955). Van de Schootbrugge et al. (Reference van de Schootbrugge, Quan, Lindström, Püttmann, Heunisch, Pross, Fiebig, Petschick, Röhling, Richoz, Rosenthal and Falkowski2009) attributed elevated concentrations in polycyclic aromatic hydrocarbons (PAHs) in Triassic–Jurassic core samples from north Germany to the subsurface thermal metamorphism of coals and organic-rich sediments. This interpretation was based on the notion that mainly high-molecular-weight (HMW) PAH, such as coronene, were dominating PAH contents of latest Triassic samples. These 5- and 6-ring PAHs form due to high-temperature charring of organic matter and coal (Fig. 4). It is unlikely that these PAH in the late Rhaetian Triletes Beds were sourced from sills in North Carolina. It is not only the large distance which may be a factor in this theory, but the latest radiometric dating shows these sills to have ages of c. 200 Ma, that is, almost 1.5 Ma after the Triassic–Jurassic boundary (Blackburn et al. Reference Blackburn, Olsen, Bowring, McLean, Kent, Puffer, McHone, Rasbury and Et-Touhami2013).

Figure 4. Conceptual framework to explain the abundance of high-molecular-weight and low-molecular-weight PAHs in boundary strata from the end-Permian and end-Triassic extinction events. The HMW compounds are generated via high-temperature charring of organic matter, coals or hydrocarbons during LIP emplacement. LMW compounds are generated via forest fires and weathering of soils.
2.b. Climate change
The residence time of carbon dioxide in the atmosphere is considerably longer than sulphur dioxide and other pollutants, and its residence time in the global carbon cycle is of the order several hundreds of thousands of years (e.g. Katz et al. Reference Katz, Wright, Miller, Cramer, Fennel and Falkowski2005). Dramatic and rapid excursions in carbon isotope records reflecting major carbon cycle perturbations have been documented based on a variety of archives, including bulk carbonate (Payne et al. Reference Payne, Lehrmann, Wei, Orchard, Schrag and Knoll2004; Bachan et al. Reference Bachan, van de Schootbrugge, Fiebig, McRoberts, Ciarapica and Payne2012; Shen et al. Reference Shen, Cao, Zhang, Bowring, Henderson, Payne, Davydov, Chen, Yuan, Zhang, Wang and Zheng2013), macro- and microfossil skeletal carbonate (Joachimski et al. Reference Joachimski, Lai, Shen, Jiang, Luo, Chen, Chen and Sun2012), bulk organic matter (Hesselbo et al. Reference Hesselbo, Robinson, Surlyk and Piasecki2002) and organic molecules (Whiteside et al. Reference Whiteside, Olsen, Eglinton, Brookfield and Sambrotto2010; Ruhl et al. Reference Ruhl, Bonis, Reichart, Sinninghe Damste and Kürschner2011). At face value all these records, in addition to numerous others, indicate one or multiple large negative C-isotope excursions (CIE) for both the Permian–Triassic and Triassic–Jurassic boundaries. However, strong differences exist between the expression and recording of the carbon cycle perturbations in marine and terrestrial settings. The main negative CIE at the Triassic–Jurassic boundary appears strongly amplified in cuticular n-alkanes, reaching nearly –8 per mille (Ruhl et al. Reference Ruhl, Bonis, Reichart, Sinninghe Damste and Kürschner2011). In contrast, marine bulk organic (Bachan et al. Reference Bachan, van de Schootbrugge, Fiebig, McRoberts, Ciarapica and Payne2012) and carbonate carbon records often show no excursions or else much smaller negative excursions (Galli et al. Reference Galli, Jadoul, Bernasconi and Weissert2005). A major positive CIE in bulk carbonate that follows the end-Triassic extinction event in the Southern Alps and the Apennines (Italy; van de Schootbrugge et al. Reference van de Schootbrugge, Payne, Tomasovych, Pross, Fiebig, Benbrahim, Föllmi and Quan2008; Bachan et al. Reference Bachan, van de Schootbrugge, Fiebig, McRoberts, Ciarapica and Payne2012) is missing from marine bulk organic (Ruhl et al. Reference Ruhl, Deenen, Abels, Bonis, Krijgsman and Kürschner2010) and terrestrial compound-specific carbon isotopes (Whiteside et al. Reference Whiteside, Olsen, Eglinton, Brookfield and Sambrotto2010), preventing a complete understanding of Triassic–Jurassic carbon cycle dynamics.
Following a major episode of flood basalt eruption, carbon dioxide build-up in the atmosphere lasted for at least 30 ka before silicate weathering and organic carbon burial led to remediation of excess carbon (Archer Reference Archer2005; Bachan & Payne, Reference Bachan and Payne2015). The immediate result, and a proximate kill mechanism for many benthic and planktonic calcareous organisms, was ocean acidification combined with global greenhouse warming. Ocean acidification would only be effective if the injection of volcanic volatiles was rapid, of the order 10 ka or less (Bachan & Payne, Reference Bachan and Payne2015). Strong greenhouse warming due to the emission of volcanic CO2 has been inferred based on a number of proxy records from Permian–Triassic and Triassic–Jurassic boundary beds. However, direct evidence for elevated pCO2 for the end-Permian extinction is relatively scarce and mostly relies on carbon cycle modelling and carbon isotope records (Fraiser & Bottjer, Reference Fraiser and Bottjer2007). Retallack (Reference Retallack2013) sees evidence for multiple greenhouse crises based on the occurrence of palaeosols that recorded hot and dry spells during late Permian – Early Triassic time. In contrast, detailed palynological records from Permian–Triassic sections in Pakistan revealing the variations in pollen and spores, in particular the occurrence of a single spore spike, suggest only one episode of particularly humid conditions during earliest Triassic time (Hermann et al. Reference Hermann, Hochuli, Bucher, Brühwiler, Hautmann, Ware and Roohi2011).
A number of recent studies make a strong case for both increases in pCO2 and warming associated with the Triassic–Jurassic boundary. At least four pulses of pCO2 increase have been reconstructed using carbonate carbon isotope records measured on pedogenic carbonate nodules from continental sections in the Newark Basin (Schaller, Wright & Kent, Reference Schaller, Wright and Kent2011). Pedogenic carbonates from the Hartford Basin indicate CO2 levels may have risen to nearly 5000 ppm during earliest Jurassic time, a doubling from latest Triassic values (Schaller et al. Reference Schaller, Wright, Kent and Olsen2012). Plants are sensitive recorders of atmospheric CO2 via stomatal density and size, and leaf stomata indicate a strong increase in atmospheric pCO2 during earliest Jurassic time from 1000 ppm to 2000–3000 ppm (McElwain, Beerling & Woodward, Reference McElwain, Beerling and Woodward1999; Steinthorsdottir, Jeram & McElwain, Reference Steinthorsdottir, Jeram and McElwain2011). Evidence for greenhouse warming across the end-Triassic extinction has also been determined using pollen and spore records (Bonis, Ruhl & Kürschner, Reference Bonis, Ruhl and Kürschner2010). The strong increase in the cheirolepidacean pollen taxon Classopollis during earliest Hettangian time, produced by an extinct, presumed thermophilic, group of conifers, is seen as reflecting higher temperatures and more arid conditions. Such interpretations of pollen records are in line with modelling exercises using coupled ocean–atmosphere climate models. Huynh & Poulsen (Reference Huynh and Poulsen2005) have demonstrated how a four-fold increase in pCO2 can cause an increase in the severity and number of hot days and days without precipitation, as well as enhanced seasonality.
3. The continents
3.a. Pathogens
Forest dieback was both a consequence and potentially a cause of the end-Permian and end-Triassic extinction events. Loss of vegetation cover can trigger secondary extinctions among herbivores, particularly insects and herbivorous reptiles (Roopnarine, Reference Roopnarine2006). In addition, deforestation leading to extreme fragmentation of forested landscapes can lead to further extinction, as shown for example by a survey of extinction rates among small mammals in a Thai tropical forest (Gibson et al. Reference Gibson, Lynam, Bradshaw, He, Bickford, Woodruff, Bumrungsri and Laurance2013). Forest fragmentation has been claimed as a major factor in driving secondary extinctions in herbivorous reptiles across the end-Permian extinction (Benton & Newell, Reference Benton and Newell2014).
Palynological records from across Europe provide evidence for complete loss of tree-bearing vegetation reflected in a strong decline in pollen abundance at the end of the Triassic. In several locations (notably in North Germany), typical dominant Rhaetian pollen taxa including Ovalipollis, Rhaetipollis, Ricciisporites and Classopollis, disappear completely from upper Rhaetian assemblages (van de Schootbrugge et al. Reference van de Schootbrugge, Quan, Lindström, Püttmann, Heunisch, Pross, Fiebig, Petschick, Röhling, Richoz, Rosenthal and Falkowski2009; Heunisch et al. Reference Heunisch, Luppold, Reinhardt and Röhling2010), recording the loss of tree-bearing vegetation. This was accompanied by the spread of ferns and fern allies, including horsetails, which likely represent hardy taxa that were able to cope with adverse conditions during latest Rhaetian time. In this interval the dominant spore taxon Polypodiisporites polymicroforatus, that makes up 35% of the palynomorph assemblages in Germany and Denmark, most likely derives from a schizaeaceous fern that bears all the hallmarks of a true pioneering species as it occurs in low frequencies in lower Rhaetian assemblages prior to the environmental changes. Such behaviour is typical for opportunistic early successional species that do well in the background, but thrive during ecosystem collapse.
Heat stress has been suggested as a factor in a 95% turnover in Late Triassic plant species in eastern Greenland (McElwain, Beerling & Woodward, Reference McElwain, Beerling and Woodward1999). However, global drying due to extreme hothouse conditions contradicts the success of ferns and fern allies following the end-Permian and end-Triassic extinction events. The spread of a fern-dominated vegetation was consistent with an increase in seasonality, perhaps with brief periods of extreme rainfall alternating with longer dry spells. Analysis of palaeosol-types across the Permian–Triassic boundary appears to provide evidence for such a scenario (Retallack, Reference Retallack2013). However, it remains unclear how climate change triggered the extinction of entire plant groups – including the disappearance of the Permian Southern Hemisphere glossopterids and cordaites, arguably two groups of plants with very different ecological preferences – or the extinction of the peltasperms during the end-Triassic event.
Stress, for example due to climate change leading to drought or frost damage, is unlikely to have been a direct cause driving the extinction of land plants. However, stress does make plants more susceptible to infection with pathogens (Wargo, Reference Wargo1996). Moreover, elevated global temperatures can lead to increased virulence of plant pathogens, particularly of fungi (Fig. 5). As for their host plants, fungal pathogens adapt to fluctuations in temperature, humidity and seasonality (Yáñez-López Reference Yáñez-López2012) and rising temperatures will extend the geographic range of most pathogens into higher latitudes (Chakraborty, Tiedemann & Teng, Reference Chakraborty, Tiedemann and Teng2000). Fungi have been implicated in the end-Permian extinction based on the widespread occurrence of the palynomorph Reduviasporonites in Permian–Triassic boundary sediments (Eshet, Rampino & Visscher, Reference Eshet, Rampino and Visscher1995; Visscher et al. Reference Visscher, Brinkhuis, Dilcher, Elsik, Eshet, Looy, Rampino and Traverse1996; Steiner et al. Reference Steiner, Eshet, Rampino and Schwindt2003). Initially interpreted as the remains of saprotrophic (wood-decaying) fungi (Visscher et al. Reference Visscher, Brinkhuis, Dilcher, Elsik, Eshet, Looy, Rampino and Traverse1996), Reduviasporonites has recently been re-interpreted as the remains of a soil-borne pathogen similar to the extant Rhizoctonia (Visscher, Sephton & Looy, Reference Visscher, Sephton and Looy2011). Today, this globally occurring fungal species causes root rot in a large number of different host species.

Figure 5. (a) Disease triangle, a conceptual framework introduced by Stevens (Reference Stevens, Horsfall and Dimonds1960) to explain interactions between the environment, pathogens and plants in driving disease. Environmental changes influence both pathogens and plants. For example, acid rain may weaken the immune defence of plants, while at the same time warming may lead to increased virulence of pathogens (e.g. root rot fungi). Together these factors drive increased risk of infection and disease as a proximate kill mechanism. (b) Tolerance curve as a function of temperature for pathogenic fungi (modified from Yáñez-López, Reference Yáñez-López2012) showing optimal range and absence in regions with exceptionally low (high latitudes) and/or high temperatures (low latitudes).
Some authors have questioned the role of Reduviasporonites in the end-Permian extinction, based on the low abundance of the fungal hyphae in high-latitude sections in Greenland and Norway (Hochuli et al. Reference Hochuli, Vigran, Hermann and Bucher2010); however, low abundance or even absence are to be expected considering the optimal thermal tolerance of pathogenic fungi (Yáñez-López Reference Yáñez-López2012; Fig. 6). Such an optimal tolerance of the fungi in warmer climates is fully consistent with the very high abundances of Reduviasporonites in low-latitude sites in Italy (Visscher et al. Reference Visscher, Brinkhuis, Dilcher, Elsik, Eshet, Looy, Rampino and Traverse1996), Israel (Eshet, Rampino & Visscher, Reference Eshet, Rampino and Visscher1995) and the Karoo (Steiner et al. Reference Steiner, Eshet, Rampino and Schwindt2003).

Figure 6. Comparison of extinction rates for poorly buffered and moderately buffered groups of calcareous organisms during the end-Permian (modified from Payne & Clapham, Reference Payne and Clapham2012) and end-Triassic extinction events (Hautmann, Benton & Tomasovych, Reference Hautmann, Benton and Tomasovych2008). Cnidarians were among the hardest-hit groups in both extinction events, leading to prolonged periods without significant reef build-ups (‘reef gaps’).
It is not only plants which are sensitive to diseases; vertebrate populations can also be decimated by diseases. Well-known examples are the ravaging of Tasmanian devil populations (decline is of the order 70%) by the spread of a facial tumour disease caused by a parasitic cancer (Siddle et al. Reference Siddle, Kreiss, Eldridge, Noonan, Clarke, Pyecroft, Woods and Belov2007). A parasitic fungus killing frogs worldwide is another telling example. Although it needs to be stressed that amphibians appear not to have been the main victims of either the end-Permian or end-Triassic extinctions, amphibians might be the first group of organisms to suffer from the ongoing ‘sixth’ mass extinction (Wake & Vredenburg, Reference Wake and Vredenburg2008). Global populations of amphibians are currently threatened by single fungal pathogen Batrachochytrium dendrobatidis (Bd). This fungus has in some cases led to the extirpation of entire species in less than 2 years and it is feared 30% of all 6300 amphibian species could fall victim to this single pathogen (Wake & Vredenburg, Reference Wake and Vredenburg2008). In addition, a related fungus B. salamandrivorans is threatening mainly salamanders and newts across Europe (Martel et al. Reference Martel, Blooi, Adriaensen, Van Rooij, Beukema, Fisher, Farrer, Schmidt, Tobler, Goka, Lips, Muletz, Zamudio, Bosch, Lotters, Wombwell, Garner, Cunningham, Spitzen-van der Sluijs, Salvidio, Ducatelle, Nishikawa, Nguyen, Kolby, Van Bocxlaer, Bossuyt and Pasmans2014). The strongly increased global incidence of skin-infecting Bd since 1998 has been related to global warming, although some authors argue that a lack of data does not allow such a link to be made (Rohr et al. Reference Rohr, Raffel, Romansic, McCallum and Hudson2008).
3.b. Fire
Forest fires are an unlikely proximate extinction mechanism because many plants prosper after burning, while some need fire to germinate. Increased incidence of forest fires during mass-extinction events are likely a reflection of important changes in climate, for example increased storminess, leading to more lightning strikes, and longer dry spells (Belcher et al. Reference Belcher, Mander, Rein, Jervis, Haworth, Hesselbo, Glasspool and McElwain2010). Pyrofusinite, a vague term to describe charcoalified wood particles in coal petrology studies, has been detected in charcoal layers in proximity to the end-Permian extinction horizon in a number of sections across southern China (Shen et al. Reference Shen, Crowley, Wang, Bowring, Erwin, Sadler, Cao, Rothman, Henderson, Ramezani, Zhang, Shen, Wang, Wang, Mu, Li, Tang, Liu, Liu, Zeng, Jiang and Jin2011a ). In addition, the high abundance of polycyclic aromatic hydrocarbons (PAHs) in core material from Australia and outcrops in China and Canada has also been used to infer widespread forest fire activity during latest Permian time (Grice, Nabbefeld & Maslen, Reference Grice, Nabbefeld and Maslen2007; Nabbefeld et al. Reference Nabbefeld, Grice, Summons, Hays and Cao2010). PAH concentrations follow fluctuations in black carbon particles with preserved wood structures in the GSSP section at Meishan, indicating a link between PAH and forest fire activity (Shen et al. Reference Shen, Sun, Lin, Liu and Chai2011b ). At Meishan the most dominant PAH are the 3- and 4-ring unsubstituted PAHs such as phenantrene, chrysene and benzo(e)pyrene, while the HMW PAHs (including coronene) occur in very low abundance. A volcanic link for fly-ash occurrences in Arctic Canada (Grasby, Sanei & Beauchamp, Reference Grasby, Sanei and Beauchamp2011) has recently been called into question by Hudspith, Rimmer & Belcher (Reference Hudspith, Rimmer and Belcher2014) based on observations that peat-burning can also produce similar vesiculated chars. It should however be stressed that massive flood basalt volcanism producing pyrogenic substances (PAH, soot) does not exclude the simultaneous raging of forest fires; in some instances, volcanism is in fact the likely trigger for widespread forest fires.
Increased burning of vegetation has also been implicated in the end-Triassic extinction based on high abundances of charcoal in Greenland (Belcher et al. Reference Belcher, Mander, Rein, Jervis, Haworth, Hesselbo, Glasspool and McElwain2010), Germany (Uhl & Montenari, Reference Uhl and Montenari2011) and Poland (Marynowski & Simoneit, Reference Marynowski and Simoneit2009). Charcoal abundance in Greenland is coupled to elevated concentrations of PAH, particularly retene, which shows a single spike at the locally defined Triassic–Jurassic boundary (between plant beds 5a and 5b). Interestingly, low charcoal abundance in plant bed 4 correlates with high amounts of the HMW compounds coronene and benzo(g,h,i)perylene (Williford et al. Reference Williford, Grice, Holman and McElwain2014). Similar mismatches between charcoal abundance and the presence of pyrogenic PAHs have been observed in Poland (Marynowski & Simoneit, Reference Marynowski and Simoneit2009) where some beds with high PAH do not contain charcoal. What is clear is that beds with high charcoal abundance mainly contain elevated concentrations of dibenzofurans and 3- and 4-ring PAH of pyrolytic origin.
3.c. Weathering and erosion
Acid rain, elevated pCO2, increased seasonality and reduced plant cover all conspired to substantially increase weathering and erosion on the continents across the end-Permian and end-Triassic extinctions. Furthermore, forest fires count among the most effective ecological disturbance, leading to strongly enhanced mass-wasting and erosion as a result of the stripping of vegetation. Changes in river systems from meandering to anastomosing as observed in the Karoo Basin (South Africa; Ward, Montgomery & Smith, Reference Ward, Montgomery and Smith2000) may provide evidence for a strong increase in rainfall and bed load, coeval with a dieback in tree-forming vegetation. More detailed sedimentological investigations, paired with vertebrate palaeontology, however suggests that droughts prevailed during earliest Triassic time in the Karoo (Smith & Botha-Brink, Reference Smith and Botha-Brink2014). Perhaps the stripping of vegetation was the key in this setting to alter river morphology. Elsewhere, in the Ural Mountains and on the Russian Platform, the late Permian Period witnessed the abrupt arrival of coarse siliciclastics on top of muddy floodplain sediments (Newell et al. Reference Newell, Sennikov, Benton, Molostovskaya, Golubev, Minikh and Minikh2010). Increased transfer from continents to oceans seems apparent in a seven-fold increase in siliciclastic sedimentation rates that have been determined from well-dated sections in China (Algeo & Twitchett, Reference Algeo and Twitchett2010). Smothering has been invoked as an additional disturbance during the end-Permian extinction on epi- and infaunal marine invertebrate communities (Algeo & Twitchett, Reference Algeo and Twitchett2010).
A strong increase in woody material (phytoclasts) in Permian–Triassic boundary sections in the Italian Dolomites has been interpreted as evidence for forest dieback and increased erosion of soils (Visscher et al. Reference Visscher, Brinkhuis, Dilcher, Elsik, Eshet, Looy, Rampino and Traverse1996). Boundary clays contain abundant low-molecular-weight PAHs, such as alkylated napthalenes and benzofurans, interpreted as evidence of increased input of degraded plant material (polysaccharides) (Sephton et al. Reference Sephton, Looy, Veefkind, Visscher, Brinkhuis and de Leeuw1999, Reference Sephton, Looy, Brinkhuis, Wignall, de Leeuw and Visscher2005; Watson et al. Reference Watson, Sephton, Looy and Gilmour2005). Alternatively, these PAHs were directly sourced by forest fires or volcanism, or may be diagenetic products of higher-molecular-weight PAHs. In all cases, they most likely represent increased soil erosion on the continents.
There is little information about changes in river morphology before and/or after the end-Triassic extinction. In the North German Basin, Upper Triassic deposits contain a series of massive sandstone beds that represent regional marker horizons. The upper Rhaetian Exter Formation, which also includes the extinction interval recorded in the fine-grained Triletes Beds, contains a massive coarse-grained sandstone at its base. Similar coarse-grained sandstones are known from Denmark and Sweden (Lindström et al. Reference Lindström, van de Schootbrugge, Dybkjaer, Pedersen, Fiebig, Nielsen and Richoz2012). There is clear evidence for increased chemical weathering rates during late Rhaetian time from the occurrence of the clay mineral kaolinite in boundary beds. In the German Basin, the Triletes Beds show an enrichment in kaolinite relative to illite (van de Schootbrugge et al. Reference van de Schootbrugge, Quan, Lindström, Püttmann, Heunisch, Pross, Fiebig, Petschick, Röhling, Richoz, Rosenthal and Falkowski2009), and elevated abundance of kaolinite in boundary beds has been documented in Austria (Zajzon et al. Reference Zajzon, Kristaly, Palfy and Nemeth2012) and Poland (Pienkowski, Niedzwiedzki & Branski, Reference Pienkowski, Niedzwiedzki, Branski, Keller and Kerr2014). Chemical weathering rates appear to have remained high, as the clay mineral assemblage in the Hettangian Blue Lias Formation in the UK continues to show abundant kaolinite (Deconinck et al. Reference Deconinck, Hesselbo, Debuisser, Averbuch, Baudin and Bessa2003).
4. The oceans
4.a. Changes in ocean temperature
Due to strengthened greenhouse climate conditions across the Permian–Triassic and Triassic–Jurassic boundaries, ocean temperatures may have risen to lethal levels (Sun et al. Reference Sun, Joachimski, Wignall, Yan, Chen, Jiang, Wang and Lai2012; Song et al. Reference Song, Wignall, Chu, Tong, Sun, Song, He and Tian2014). Surface water temperatures have been reconstructed from oxygen isotope records derived from conodont apatite in China and Iran (Sun et al. Reference Sun, Joachimski, Wignall, Yan, Chen, Jiang, Wang and Lai2012; Schobben et al. Reference Schobben, Joachimski, Korn, Leda and Korte2014). In equatorial waters, temperatures rose by an astounding 15°C from 21 to 36°C during the earliest Triassic Griesbachian Stage (a period of only 800 ka). A slight cooling during Dienerian time was followed by further warming during late Smithian time, when equatorial sea-surface temperatures may have exceeded 40°C (Sun et al. Reference Sun, Joachimski, Wignall, Yan, Chen, Jiang, Wang and Lai2012). Such temperatures can be regarded as lethal for many marine organisms, especially cephalopods and marine reptiles that have high oxygen demands at high temperatures. High ocean water temperatures are also thought to explain dwarfism (the ‘Lilliput’ effect) observed in many groups of organisms inhabiting equatorial waters across the Permian–Triassic boundary (Sun et al. Reference Sun, Joachimski, Wignall, Yan, Chen, Jiang, Wang and Lai2012). Interestingly, oxygen isotopes from conodonts track the bulk carbonate carbon isotope record from the Nanpanjiang Basin (China), showing a near-perfect match between negative C-isotope excursions; this is suggestive of a massive release of isotopically light CO2 and warming episodes.
A dramatic rise in temperatures has also been observed for the end-Triassic extinction, although the data density is less than for the Permo-Triassic interval. Conodonts became both rare and extremely small during latest Triassic time, which makes them less suited for measuring oxygen isotopes. More importantly, conodonts count as one of the rare animal groups to experience 100% extinction at the end of Triassic time. Belemnite rostra, the preferred skeletal calcite for obtaining Mesozoic palaeotemperatures, only become abundant during Sinemurian time, well after the extinction event. Some records have been published that use oyster calcite as an archive of temperature changes (van de Schootbrugge et al. Reference van de Schootbrugge, Tremolada, Bailey, Rosenthal, Feist-Burkhardt, Brinkhuis, Pross, Kent and Falkowski2007; Korte et al. Reference Korte, Hesselbo, Jenkyns, Rickaby and Spötl2009), but both studies derive from small and fairly restricted basins in the UK. Nonetheless, the oyster calcite oxygen isotope records from Lavernock Point, St Audrie's Bay and Lyme Regis document a 10°C rise in sea-surface temperatures from latest Triassic – earliest Jurassic time (Korte et al. Reference Korte, Hesselbo, Jenkyns, Rickaby and Spötl2009). Interestingly, the oxygen isotope record from Lavernock Point follows the bulk carbonate carbon isotope record, providing further evidence for a coupling between temperature changes and the global carbon cycle. Temperatures appear to have stabilized around early–late Hettangian time.
4.b. Marine anoxia
Ocean de-oxygenation and the spread of ‘dead zones’ is a major threat to oceans and marine life today. ‘Dead zones’, such as those which develop each year in the Gulf of Mexico (Diaz & Rosenberg, Reference Diaz and Rosenberg2008), result from a combination of factors including enhanced runoff, triggering both salinity stratification as well as eutrophication which then leads to enhanced primary production (Murphy, Kemp & Ball, Reference Murphy, Kemp and Ball2011). Ultimately, the sinking of dead algal matter drives excess oxygen consumption in bottom waters. Both the end-Permian and end-Triassic extinction events were associated with widespread anoxic to euxinic conditions (Hallam & Wignall, Reference Hallam and Wignall1997). Initially, dysoxia was recognized mainly from sedimentological and petrographic observations of laminated, pyritic and organic-rich sediments in Tethyan sections, for example in the Italian Dolomites (Wignall & Hallam, Reference Wignall and Hallam1992; Twitchett, Reference Twitchett1999). In Japan, the end-Permian extinction was marked by the replacement of red, radiolarian cherts with pyritic, black shales and siliceous mudstones that persist until the Middle Triassic (Isozaki, Reference Isozaki1997; Wignall et al. Reference Wignall, Bond, Kuwahara, Kakuwa, Newton and Poulton2010; Algeo et al. Reference Algeo, Chen, Fraiser and Twitchett2011).
During the climactic phase of the end-Permian extinction, dysoxic conditions intensified globally (Wignall & Twitchett, Reference Wignall, Twitchett and MacLeod2002; Bond & Wignall, Reference Bond and Wignall2010) and in some places photic zone euxinia developed, that is, a photic zone saturated in hydrogen sulphide. Biomarkers for green sulphur bacteria, photosynthetic lithotrophs that need H2S and light, have been detected in boundary shales in Meishan (China; Cao et al. Reference Cao, Love, Hays, Wang and Shen2009), Australia (Grice et al. Reference Grice, Cao, Love, Böttcher, Twitchett, Grosjean, Summons, Turgeon, Dunning and Jin2005a ) and in outcrops and cores from western Canada (Hays et al. Reference Hays, Beatty, Henderson, Love and Summons2007). Shallowing of euxinia from the seafloor into the water column has also been inferred from the size of pyrite framboids (Wignall, Newton & Brookfield, Reference Wignall, Newton and Brookfield2005; Shen et al. Reference Shen, Lin, Xu, Li, Wu and Sun2007), as well as positive excursions in carbonate-associated sulphur (CAS) isotopes (Kampschulte & Strauss, Reference Kampschulte and Strauss2004; Riccardi, Arthur & Kump, Reference Riccardi, Arthur and Kump2006). Positive excursions in CAS isotopes have been explained by strongly increased pyrite burial whereby bacterial sulphate reduction leads to removal of 32S, leaving the residual sulphate reservoir enriched in the heavy 34S (Newton et al. Reference Newton, Pevitt, Wignall and Bottrell2004). In places where anoxia was not strongly developed, such as in Oman, the recovery of benthic ecosystems proceeded at a higher pace, suggesting that prolonged anoxic conditions in shallow seas contributed to the overall slow recovery rates (Twitchett et al. Reference Twitchett, Krystyn, Baud, Wheeley and Richoz2004).
Remarkably little is known about the end-Triassic sulphur cycle. What is known is that anoxic conditions started to become prevalent in basins bordering the Tethys Ocean as early as during Rhaetian time. Thick black shales accumulated during late Rhaetian time in the Lago Negro Basin in southern Italy (Ciarapica, Reference Ciarapica2007) and local basins in Austria, such as the Kössen and Eiberg basins, also contain late Rhaetian organic-rich shales alternating with limestones (Holstein, Reference Holstein2004). Similar to that observed for the end-Permian event, euxinic conditions then spread into shallow epicontinental seaways across NW Europe during earliest Hettangian time (although this was after the mass extinction) (Hallam, Reference Hallam1995). High concentrations of isorenieratane, a carotenoid biomarker specific for green sulphur bacteria, occur in basal Jurassic black shales in Germany, Luxemburg and the UK (Richoz et al. Reference Richoz, de Schootbrugge, Pross, Püttmann, Quan, Lindström, Heunisch, Fiebig, Maquil, Hauzenberger, Schouten and Wignall2012; Jaraula et al. Reference Jaraula, Grice, Twitchett, Böttcher, LeMetayer, Dastidar and Opazo2013). Even though biomarkers cannot be detected in each section with black shales, due to issues with diagenesis and maturity of the sediments, syngenetic pyrite framboids also point to euxinia in Canada and England (Wignall et al. Reference Wignall, Zonneveld, Newton, Amor, Sephton and Hartley2007; Wignall & Bond, Reference Wignall and Bond2008). Few Triassic–Jurassic sulphur isotope records exist that may underpin chemocline excursions, but a large positive excursion in bulk sulphur isotope values has been interpreted as reflecting intensified sulphate reduction in the Panthalassa Ocean (Williford et al. Reference Williford, Foriel, Ward and Steig2009) even though there is no sedimentary or petrographic evidence for ocean floor anoxia in the Panthalassa Ocean in Japan (Hori et al. Reference Hori, Fujiki, Inoue and Kimura2007; Wignall et al. Reference Wignall, Bond, Kuwahara, Kakuwa, Newton and Poulton2010). Sulphate reduction may have been occurring within a mid-water oxygen minimum zone.
The release of H2S to the atmosphere from euxinic waters has been advocated as an additional mechanism to lead to build-up of SO2 in the atmosphere during the end-Permian extinction (Kump, Pavlov & Arthur, Reference Kump, Pavlov and Arthur2005). However, model calculations show such a mechanism would require the development of euxinia in large parts of the Tethys and Panthalassa oceans, as well as repeated overturn of deep water (Harfoot, Pyle & Beerling, Reference Harfoot, Pyle and Beerling2008). The spread of euxinic conditions was likely spatially complex and may have been limited to shallow seas during latest Triassic time, although some recent evidence from Haida Gwaii (western Canada) suggest that open ocean sites in the Panthalassa were also euxinic (Kasprak et al. Reference Kasprak, Sepulveda, Price-Waldman, Williford, Schoepfer, Haggart, Ward, Summons and Whiteside2015). Anoxia had not developed everywhere at the time of the Triassic–Jurassic boundary; no evidence for anoxia has been found in Tibet, for example (Hallam et al. Reference Hallam, Wignall, Yin and Riding2000). In addition, in both deep-water and shallower Tethyan sections, such as in Italy (Galli et al. Reference Galli, Jadoul, Bernasconi and Weissert2005, Reference Galli, Jadoul, Bernasconi, Ciriili and Weissert2007) and Montenegro (Crne et al. Reference Crne, Weissert, Gorican and Bernasconi2010), boundary clays are not particularly organic rich.
As pointed out by Payne & Clapham (Reference Payne and Clapham2012), sustained anoxic to euxinic conditions following the end-Permian extinction would only be possible if surface waters became eutrophied, driving high rates of primary productivity (Meyer & Kump, Reference Meyer and Kump2008a ; Meyer, Kump & Ridgwell, Reference Meyer, Kump and Ridgwell2008; Meyer et al. Reference Meyer, Yu, Jost, Kelley and Payne2011). An increased gradient in organic carbon isotope records along a margin to basin transect has been taken as evidence for an enhanced biological pump governing Early Triassic oceans (Meyer et al. Reference Meyer, Yu, Jost, Kelley and Payne2011). The end-Permian extinction is also associated with the appearance of abundant acritarch and prasinophyte remains in sections worldwide from Greenland to Australia (Grice et al. Reference Grice, Twitchett, Alexander, Foster and Looy2005b ). Although no carbon isotope transects exist for the end-Triassic extinction, changes in phytoplankton from dinoflagellate-dominated assemblages to assemblages dominated by acritarchs and prasinophytes are apparent (Richoz et al. Reference Richoz, de Schootbrugge, Pross, Püttmann, Quan, Lindström, Heunisch, Fiebig, Maquil, Hauzenberger, Schouten and Wignall2012; van de Schootbrugge et al. Reference van de Schootbrugge, Bachan, Suan, Richoz and Payne2013).
4.c. Acidification
As a result of unabated CO2 emissions from fossil fuel burning, ocean pH is set to decline from 8.2 to 7.8 by the end of the current century (IPCC, 2014). Ocean acidification is increasingly seen as a major threat to the health of benthic and planktonic ecosystems in the oceans today. Aragonitic scleractinian corals, and therefore entire reefs, are predicted to be at risk in particular (Hoegh-Guldberg et al. Reference Hoegh-Guldberg, Mumby, Hooten, Steneck, Greenfield, Gomez, Harvell, Sale, Edwards, Caldeira, Knowlton, Eakin, Iglesias-Prieto, Muthiga, Bradbury, Dubi and Hatziolos2007). Ocean acidification has been suggested as having caused the extinction of heavily calcified organisms with poor buffering capacities, including rugose, tabulate and scleractinian corals, brachiopods and bivalves, during both the end-Permian (Knoll et al. Reference Knoll, Bambach, Payne, Pruss and Fischer2007) and end-Triassic extinction events (Greene et al. Reference Greene, Martindale, Ritterbush, Bottjer, Corsetti and Berelson2012b ), although supporting geochemical evidence is enigmatic.
Boron isotope records, obtained from bulk carbonates sampled in Oman, provide no evidence for acidification during the end-Permian mass extinction but instead reveal a brief (c. 10 ka) excursion suggestive of acidification during Early Triassic time, which may be implicated with later extinction losses (Clarkson et al. Reference Clarkson, Kasemann, Wood, Lenton, Daines, Richoz, Ohnemueller, Meixner, Poulton and Tipper2015). Sedimentary evidence, in the form of a limestone truncation surface seen in uppermost Permian deposits in Turkey, has been ascribed to short-term under-saturation leading to dissolution (Fig. 7). Payne et al. (Reference Payne, Lehrmann, Follett, Seibel, Kump, Riccardi, Altiner, Sano and Wei2007) advocated this as evidence for acidification, although others see evidence here for an emergence surface (Wignall & Bond, Reference Wignall and Bond2008). Newly emerged evidence from the Nanpanjiang Basin in China suggests that submarine dissolution may have been more widespread (Lehrmann et al. Reference Lehrmann, Bentz, Wood, Goers, Dhillon, Akin, Li, Payne, Kelley, Meyer, Schaal, Suarez, Yu, Qin, Li, Minzoni and Henderson2015). However, these sedimentary clues considerably pre-date the B-isotope evidence for acidification.

Figure 7. (a) Uppermost Rhaetian mudstones with giant megalodontid bivalves in the Mount Messapion section (Greece). These large bivalves abruptly disappear at the Triassic–Jurassic boundary (e.g. Romano et al. Reference Romano, Masetti, Barattolo, Carras, Barattolo and Roghi2008), providing a dramatic example of a crisis in biocalcification during the ETME. (b) Topmost bed of the Rhaetian Zu Limestone Formation in the Val Adrara section (Southern Alps, Italy). This bed contains abundant recrystallized scleractinian corals and other bioclasts, and is abruptly overlain by thin-bedded limestones of the Malanotte Formation (see also Bachan et al. Reference Bachan, van de Schootbrugge, Fiebig, McRoberts, Ciarapica and Payne2012 for lithological logs). (c) Abundant early diagenetic cements in the Hettangian Valle Agricola section (Apennines, Italy). Similar sedimentary features also characterize the Hettangian deposits further south in the Monte Cefalo section close to Naples (see Bachan et al. Reference Bachan, van de Schootbrugge, Fiebig, McRoberts, Ciarapica and Payne2012), perhaps suggesting carbonate supersaturation in shallow oceans due to a breakdown in biological carbonate precipitation. (d) Photograph of the Permian–Triassic boundary bed in the Çürük Dag section in Turkey showing sharp irregular contact between a Gymnocodium packstone and a pelsparite.
The most characteristic attribute of carbonate platforms during the Permo-Triassic interval is the presence of stromatolitic or thrombolitic build-ups as seen in Turkey (Baud, Richoz & Marcoux, Reference Baud, Richoz and Marcoux2005) and China (Kershaw, Zhang & Lan, Reference Kershaw, Zhang and Lan1999), suggesting supersaturation. Strongly supersaturated seawaters during Early Triassic time are also reflected by the deposition of syngenetic carbonates in the form of aragonite fans (Woods et al. Reference Woods, Bottjer, Mutti and Morrison1999). An abundance of seafloor cements has been noted in Hettangian carbonate platform sections exposed in the Southern Alps (Italy) by Jadoul & Galli (Reference Jadoul and Galli2008) and linked to changes in saturation state. Further south in the Italian Apennines, massive cements and tepee-structures characterize much of the Lower Jurassic deposits (Fig. 7). The occurrence of crystal fans in the Fernie Formation in Canada has also been advocated as evidence for changes in ocean pH (Greene et al. Reference Greene, Bottjer, Corsetti, Berelson and Zonneveld2012a ), although these latter occurrences resemble late diagenesis ‘beef calcite’ which has recently been linked to the generation of hydrocarbons (Cobbold & Rodrigues, Reference Cobbold and Rodrigues2007; Cobbold et al. Reference Cobbold, Zanella, Rodrigues and Løseth2013). True aragonite fans were observed by Bachan et al. (Reference Bachan, van de Schootbrugge, Fiebig, McRoberts, Ciarapica and Payne2012) in the Southern Alps (Italy).
Ocean acidification is increasingly seen as a mechanism to explain the abrupt disappearance of reef ecosystems and more than 95% extinction in scleractinian corals during latest Rhaetian time (Martindale et al. Reference Martindale, Berelson, Corsetti, Bottjer and West2012). Sedimentological evidence for ocean acidification, such as the presence of carbonate-devoid boundary clays in both the Tethys and Panthalassa oceans, has been discussed by Hautmann, Benton & Tomasovych (Reference Hautmann, Benton and Tomasovych2008). Abrupt changes in carbonate sedimentation are apparent in many sections bordering the Tethys Ocean in Italy and Greece (Bachan, van de Schootbrugge & Payne, Reference Bachan, van de Schootbrugge and Payne2014). The disappearance of reef organisms in the Zu Formation in the Val Adrara section (Italy) was also extremely abrupt; however, this may have been partly due to regression and erosion at the contact between the Zu Formation and the overlying Malanotte Formation. Trends in shell thickness have been used to argue against acidification across the ETME (Mander, Twitchett & Benton, Reference Mander, Twitchett and Benton2008). Nevertheless, large megalodontid bivalves in Tethyan shallow-marine carbonate platform sections in Italy and Greece not only disappeared abruptly, but the surviving faunas contained species that were strongly reduced in size (Hautmann, Reference Hautmann2004). At Mount Messapion in a section close to Athens (Greece), the abrupt disappearance of the huge megalodontid bivalves is both puzzling and striking (Romano et al. Reference Romano, Masetti, Barattolo, Carras, Barattolo and Roghi2008; Fig. 7). These bivalves may have grown to such large sizes because of the presence of photosymbionts, and their extinction and reduction in size could potentially have been triggered by disturbances in this symbiotic partnership.
5. Converging scenarios: similarities and differences
Scenarios for the end-Permian and end-Triassic extinction events are increasingly converging, and both are now commonly linked to the emplacement of the large igneous provinces of the Siberian Traps and the Central Atlantic Magmatic Province. Overall, the following scenario is relevant to both the end-Permian and end-Triassic extinction. Flood basalt volcanism released large quantities of sulphur dioxide, carbon dioxide, thermogenic methane and potentially large amounts of pollutants such as HF, HCl, halocarbons and toxic aromatics and heavy metals into the atmosphere. Volatile loads were likely exacerbated by country-rock – lava interactions depending on the intrusions of sills into organic-rich and/or evaporitic and petroleum-rich crustal rocks. The released volatiles exerted a range of influences on atmospheric chemistry, leading to tropospheric transport of pollutants and sulphuric acid aerosols and a potential breakdown in ozone concentrations in the stratosphere. Acid rain likely had an impact on freshwater ecosystems and may have triggered forest dieback, whereas increased UV radiation from decreased ozone shielding may have triggered malformations in plant reproductive systems. The stripping of vegetation due to environmental pollution and climate change, perhaps aided by repeated burning, greatly enhanced weathering and erosion rates, ultimately driving high nutrient input into shallow oceans. Eutrophication of surface waters drove high primary productivity and, coupled with high water temperatures, led to widespread anoxia and repeated photic zone euxinia.
These scenarios are supported by much of the reviewed literature and data; however, differences and strong similarities may be noted between the end-Permian and end-Triassic extinctions (Fig. 8). Such a comparison may give insight into scenarios that are more strongly supported, and highlight our gaps in understanding. Overall, there is more global evidence for environmental change associated with the end-Permian extinction. There is clearly a lack of key evidence from the Southern Hemisphere for the end-Triassic extinction (Fig. 8). The end-Permian has also been studied more intensely, perhaps explaining a more global coverage. There is therefore some bias when comparing the extinction events. Nevertheless, strong evidence exists for anoxia and photic zone euxinia associated with both extinction events based on biomarkers, trace element records and pyrite framboids. On a more local scale charcoal records point to forest fires as a common denominator, while palynological and palaeobotanical evidence suggests that forest dieback was prevalent during both events. Together, these phenomena may have led to increased weathering and erosion on the continents, although the evidence collected so far for changes in clay mineral assemblages seems more robust for the end-Triassic extinction. While there is general consensus that both extinctions are also partly related to greenhouse warming, only direct evidence for a rise in pCO2 (from stomata and pedogenic carbonates) comes from the end-Triassic extinction.

Figure 8. Comparison of proximate kill mechanisms for the end-Permian and end-Triassic extinction showing quality of evidence and locations where key evidence has been presented (non-exhaustive). (a) 1, Italy (Dolomites); 2, South Africa (Karoo Basin); 3, Canada (Sverdrup Basin); 4, China (Nanpanjiang Basin); 5, Australia; 6, Siberia (Tunguska Basin); 7, Svalbard; 8, Pakistan; 9, Israel; 10, Oman; 11, Turkey (Taurus Mountains); 12, Greenland; 13, Iran; 14, Japan; 15, Antarctica. (b) 1, Germany (north and south); 2, Poland; 3, Greenland; 4, Austria (GSSP and nearby sections); 5, United States (eastern); 6, Sweden (Scania); 7, Denmark; 8, Hungary; 9, Italy (Southern Alps, Apennines); 10, England (Somerset); 11, Luxemburg; 12, Canada (Haida Gwaii); 13, United States (southwest); 14, Canada (Williston Basin).
Acid rain remains difficult to demonstrate for both extinction events, and only very circumstantial evidence is available for the end-Triassic extinction. However, acid rain proxy records (sulphur isotopes in fresh water, biomarkers from soils) for the end-Permian extinction may equally be questioned. Other lines of evidence are generally more spurious for the end-Triassic extinction. Pollution with heavy metals or hydrocarbons is evident from a number of sites for the end-Permian extinction but, except for PAH records from Germany and Poland, such records are lacking for the end-Triassic. Evidence for severe stress (diseases, increased radiation) among primary producers from the abundance of fungal spores and mutated spores and pollen is clearer for the end-Permian extinction. However, recent findings of mutations in common Triassic pollen species close to the Triassic–Jurassic boundary in the United States should lead to more intense investigations of pollen and other records. In the oceans, evidence for acidification and lethal hot temperatures seems more abundant for the end-Permian extinction. This is partly due to a few easily accessible carbonate deposits or suitable calcitic micro- and macrofossils straddling the Triassic–Jurassic boundary that allow reconstructions of oxygen isotope records or other temperature proxy records. Both flood basalt provinces (Siberian Traps, CAMP) are situated in the Northern Hemisphere, and much can be learned from interhemispheric comparisons in order to understand the truly global impact of flood basalt eruptions. However, it is clear that more data are needed from the Southern Hemisphere for the end-Triassic extinction.
Acknowledgements
We thank Henk Visscher (Utrecht) for discussions and providing background information. Carolien van der Weijst (Utrecht) is thanked for providing pictures of palynomorphs. David Bond and an anynomous reviewer are thanked for their helpful comments that strengthened the final manuscript.