Obsessive–compulsive disorder (OCD) is a chronic, heritable and debilitating psychiatric disorder characterized by intrusive thoughts (obsessions) and repetitive behaviors (compulsions) that are driven by anxiety and are experienced as unwanted (Milad and Rauch, Reference Milad and Rauch2012; Pauls et al., Reference Pauls, Abramovitch, Rauch and Geller2014). The population prevalence of OCD is 2–3%, and the age of onset ranges from very early childhood into adulthood (Ruscio et al., Reference Ruscio, Stein, Chiu and Kessler2008). Although many studies have investigated the pathophysiology of OCD, the specific neural circuitry underlying OCD is still not yet clear.
As compulsive behaviors are the core overt symptoms of OCD, probing the neural substrate of compulsive behaviors has the potential to significantly advance our understanding of underlying neurobiology and inform treatment strategies. Recently, researchers have hypothesized that compulsive behaviors originate from an imbalance within the corticostriatal systems mediating cognitive control of learning and behavior (Milad and Rauch, Reference Milad and Rauch2012; Dolan and Dayan, Reference Dolan and Dayan2013; Gillan and Robbins, Reference Gillan and Robbins2014). The corticostriatal system is structured in a recurrent loop, in which information passes from the cortex through the striatum and thalamus and then back to the cortex (Shepherd, Reference Shepherd2013). These projections can be divided into three main divisions, the limbic, associative, and motor loops on the basis of the cortical and striatal regions involved and the functions performed by each (Posner et al., Reference Posner, Marsh, Maia, Peterson, Gruber and Simpson2014; Seger, Reference Seger2018). The limbic loop connecting the nucleus accumbens with limbic cortexes has been implicated in motivational, emotional, and reward processing. In contrast, the associative loop and motor loop both underlie learning and behavior, but in different ways. The associative loop is vital for consciously-controlled and goal-directed behavior. This goal-directed system encodes action-outcome associations, which are learned and executed by a neural network connecting dorsolateral prefrontal cortex (DLPFC), and the caudate (Balleine and O'Doherty, Reference Balleine and O'Doherty2010; Gillan and Robbins, Reference Gillan and Robbins2014; Jahanshahi et al., Reference Jahanshahi, Obeso, Rothwell and Obeso2015). In contrast, the putamen and motor loop underlie habitual learning and behavior. In the habitual system, behavior relies on stimulus-response associations, learned and executed by a neural network connecting the putamen, supplementary motor area (SMA) and insula (Balleine and O'Doherty, Reference Balleine and O'Doherty2010; Gillan and Robbins, Reference Gillan and Robbins2014; Jahanshahi et al., Reference Jahanshahi, Obeso, Rothwell and Obeso2015).
Researchers have argued that in OCD there is an imbalance between the automatic habitual system and the consciously-controlled goal-directed system (Dolan and Dayan, Reference Dolan and Dayan2013; Gillan et al., Reference Gillan, Morein-Zamir, Urcelay, Sule, Voon, Apergis-Schoute, Fineberg, Sahakian and Robbins2014; Gillan and Robbins, Reference Gillan and Robbins2014), with a bias towards behavior being controlled by the habitual system. Studies using an avoidance paradigm found that OCD patients exhibited hyper-activations in the caudate nucleus, which suggested that a bias towards behavioral control by the habit system may result from impairment of the goal-directed system (Gillan et al., Reference Gillan, Apergis-Schoute, Morein-Zamir, Urcelay, Sule, Fineberg, Sahakian and Robbins2015). OCD patients also showed an imbalance between the habitual system and the goal-directed system during symptom provocation (Banca et al., Reference Banca, Voon, Vestergaard, Philipiak, Almeida, Pocinho, Relvas and Castelo-Branco2015). Interestingly, one study found significant activity in the goal-directed system during an instrumental action task, that was accompanied by no changes in activity in the habit-learning system (Vaghi et al., Reference Vaghi, Vértes, Kitzbichler, Apergis-Schoute, van der Flier, Fineberg, Sule, Zaman, Voon, Kundu, Bullmore and Robbins2017b). This pattern of results suggested that the goal-directed system may dominate during some tasks, while the habit system remains silent. It should be highlighted that the silent system may not be reliably detected during functional magnetic resonance imaging (fMRI), when behavior is being controlled by another system. Furthermore, it is unknown whether this dysfunction of the goal-directed system is present only during behavioral control tasks which require behavioral flexibility and action monitoring, or whether this dysfunction is a stable and common trait for OCD, which is always present including during the resting state.
In addition to the associative and motor corticostriatal systems underlying behavioral learning and control, previous research indicates that OCD may also affect activity within the limbic corticostriatal system. One recent study found a significant hyper-influence of the OFC over the ventral striatum of subjects with OCD (Abe et al., Reference Abe, Sakai, Nishida, Nakamae, Yamada, Fukui and Narumoto2015).
It has been consistently reported that relatives of individuals with OCD have a greatly enhanced risk of developing OCD compared with the general population (Pauls et al., Reference Pauls, Abramovitch, Rauch and Geller2014). Many studies have found that the relatives of OCD patients show similar deficits in executive functions (Zhang et al., Reference Zhang, Yang and Yang2015), such as motor inhibition, cognitive inflexibility, and impaired performance on working memory and planning (Robbins et al., Reference Robbins, Gillann, Smith, de Wit and Ersche2012). Furthermore, Chamberlain and colleagues found reduced activity in the lateral OFC, PFC, and parietal responsiveness during reversal learning in both patients with OCD and their unaffected never-treated relatives (Chamberlain et al., Reference Chamberlain, Menzies, Hampshire, Suckling, Fineberg, del Campo, Aitken, Craig, Owen, Bullmore, Robbins and Sahakian2008). Hou and colleagues found resting-state functional connectivity strength abnormalities in the left OFC, bilateral caudate nucleus and left middle temporal gyrus may be neuroimaging endophenotypes for OCD (Hou et al., Reference Hou, Zhao, Zhang, Song, Wu, Wang, Zhou, Xie, He, Guo, Qu and Li2014). Other brain structural and functional deficits have also been found in both groups, such as frontal-parietal hyper-activation (de Vries et al., Reference de Vries, de Wit, Cath, van der Werf, van der Borden, van Rossum, van Balkom, van der Wee, Veltman and van den Heuvel2014), and frontal and parietal white matter and gray matter abnormalities associated with decreased inhibitory control (Menzies et al., Reference Menzies, Williams, Chamberlain, Ooi, Fineberg, Suckling, Sahakian, Robbins and Bullmore2008b). Therefore, biomarkers shared with OCD relatives can provide rich genetic information for future research, and can help clarify whether goal-directed impairment represents a state or trait marker for OCD (Vaghi et al., Reference Vaghi, Hampshire, Fineberg, Kaser, Brühl, Sahakian, Chamberlain and Robbins2017a).
Granger causality analysis (GCA) is a well-established method to measure effective connectivity (EC) between brain regions (Zang et al., Reference Zang, Yan, Dong, Huang and Zang2012). It utilizes multiple linear regression to assess whether the past value of one time series can correctly predict the current value of another, which can be used to characterize the dynamic influences between brain regions (Deshpande et al., Reference Deshpande, Laconte, James, Peltier and Hu2009; Nakhnikian et al., Reference Nakhnikian, Rebec, Grasse, Dwiel, Shimono and Beggs2014). An advantage of GCA is that it provides information about the directionality of effects so that it is possible to determine which area exerts influence or receives influence from another. To identify impairment in the goal-directed corticostriatal system and compare it with activity within the motor and limbic corticostriatal systems, we employed GCA analysis of the three striatal networks. Networks were identified using regions of interest (ROIs) in striatal sub-regions which previous research showed were effective for eliciting each network (Di Martino et al., Reference Di Martino, Scheres, Margulies, Kelly, Uddin, Shehzad, Biswal, Walters, Castellanos and Milham2008). These ROIs included the bilateral caudate for the goal-directed system, the putamen for habit system, and nucleus accumbens for the limbic system underlying motivation and reward.
The present study was designed to examine (1) whether patients with OCD show changes in effective connectivity and direction of influences within the goal-directed behavioral learning system during the resting-state that are consistent with the deficits reported during cognitive tasks and symptom provocation; and (2) whether these patterns may serve as vulnerability markers for OCD. Our first hypothesis was that patients with OCD would exhibit significantly abnormal effective connectivity (EC) within the goal-directed cortical-striatal network. Our second hypothesis was that the unaffected first-degree relatives of OCD patients would show similar abnormal effective connectivity as OCD patients, supporting the potential use of effective connectivity as a biomarker for vulnerability to OCD.
Methods
Participants
The study sample included thirty-five patients (male = 24, female = 11), thirty-five unaffected first-degree relatives (male = 16, female = 19), and thirty-five healthy subjects (male = 18, female = 17). All subjects were from the Chinese Han population and were assessed by two trained and experienced clinical psychiatrists and psychologists.
OCD patients and their unaffected first-degree relatives were recruited through the Guangzhou Psychiatric Hospital. All patients satisfied Diagnostic and Statistical Manual of Mental Disorders, 4th edition (DSM-IV) criteria for OCD by using the Structured Clinical Interview (SCID) for DSM-IV-TR Axis I disorders (First et al., Reference First, Spitzer, Miriam and Williams2002). Patients were excluded: (a) if they were younger than 18 or older than 55 years; (b) if they had a history of brain trauma or neurological disease; and (c) if they had shown alcohol/substance abuse within 12 months prior to participation. Half of the patients stopped taking psychoactive medications one month before the scan, and the other half took their normal medications while scanning (details of the medications and dosages for on-medication subjects are provided in the online supplementary materials). Twenty patients had comorbid disorders such as anxiety or depression.
The unaffected first-degree relatives and healthy controls were screened by using the SCID for the DSM-IV-TR Axis I disorders, Research Version, Non-Patient edition (SCID-I/NP) (First et al., Reference First, Spitzer, Miriam and Williams2002). Healthy controls with a family history of axis I or II psychiatric disorders were excluded.
All subjects gave written informed consent after receiving a complete description of the study. The study was approved by the institutional research and ethics committee of Guangzhou Psychiatric Hospital.
Clinical assessments
The Yale-Brown Obsessive–Compulsive Scale (Y-BOCS) (Goodman et al., Reference Goodman, Price, Rasmussen, Mazure, Fleischmann, Hill, Heninger and Charney1989) was administered to assess the illness severity. The Obsessive–Compulsive Inventory-Revised (OCI-R) (First et al., Reference First, Spitzer, Miriam and Williams2002; Peng et al., Reference Peng, Yang, Miao, Jing and Chan2011) was used to characterize obsessive and compulsive phenomena, including obsessing, washing, checking, neutralizing, ordering and hoarding. The Beck Depression Inventory (BDI) (Beck and Steer, Reference Beck and Steer1984) was used to estimate depressive symptoms, and the State-Trait Anxiety Inventory (STAI) (Spielberger, Reference Spielberger1983) was used to measure anxiety symptoms. We performed ANOVA and χ2 tests using SPSS 16.0.
Magnetic resonance imaging acquisition
A 3.0-Tesla MR system (Philips Medical Systems, U.S.A.) equipped with an eight-channel phased-array head coil was used for data acquisition. Participants were instructed to relax with eyes closed, and stay awake without moving. Functional data were collected using gradient echo Echo-Planar Imaging (EPI) sequences [time repetition (TR) = 2000 ms; echo time (TE) = 60 ms; flip angle = 90°, 33 slices, field of view (FOV) = 240 mm × 240 mm, matrix = 64 × 64; and slice thickness = 4.0 mm]. For spatial normalization and localization, a high-resolution T1-weighted anatomical image was also acquired using a magnetization prepared gradient echo sequence (TR = 8 ms, TE = 1.7 ms, flip angle = 20°, FOV = 240 mm × 240 mm, matrix = 256 × 256, and slice thickness = 1.0 mm).
Data preprocessing
The data were preprocessed using the Statistical Parametric Mapping toolbox (SPM8, http://www.fil.ion.ucl.ac.uk/spm), and Data Processing Assistant for Resting-State fMRI (DPARSFA version 2.2, http://rfmri.org/DPARSF). Image preprocessing consisted of: (1) slice timing correction; (2) head motion correction; (3) realignment with the corresponding T1-volume; (4) nuisance covariate regression (six head motion parameters, white matter signal and cerebrospinal fluid signal); (5) spatial normalization into the stereotactic space of the Montreal Neurological Institute and resampling at 3 mm3 × 3 mm3 × 3 mm3; and (6) spatial smoothing with a 6 mm full-width half-maximum isotropic Gaussian kernel, and band-pass filtered (0.01–0.08 Hz). One OCD patient and two controls were excluded from further analysis because of a maximal absolute head motion larger than 1.5 mm or 1.5° in any of the three translation planes or rotation axes, respectively.
Regions of interest (ROIs)
Three previously validated striatal ROIs (Di Martino et al., Reference Di Martino, Scheres, Margulies, Kelly, Uddin, Shehzad, Biswal, Walters, Castellanos and Milham2008) were selected in each hemisphere: the bilateral nucleus accumbens (X = ±9, Y = 9, Z = −8), the bilateral caudate (X = ±13, Y = 15, Z = 9) and the bilateral putamen (X = ±28, Y = 1, Z = 3). ROIs were defined as a sphere surrounding the central voxel with a diameter of 6 mm. The previous study includes additional details about the anatomical delineation of these regions (Di Martino et al., Reference Di Martino, Scheres, Margulies, Kelly, Uddin, Shehzad, Biswal, Walters, Castellanos and Milham2008).
Bivariate voxel-wise Granger causality analysis
Bivariate voxel-wise GCA was applied in the present study by using the Resting-State fMRI Data Analysis Toolkit (REST version 1.8, http://resting-fmri.sourceforge.net). Granger causality analysis (GCA) is a method based on multiple linear regression for investigating whether the past value of one time series can predict the current value of another time series (Deshpande et al., Reference Deshpande, Laconte, James, Peltier and Hu2009; Zang et al., Reference Zang, Yan, Dong, Huang and Zang2012). Positive and negative GCA coefficients indicate that past activity of a brain region predicts increased or decreased, respectively, activity in the target brain region. For more details see the original paper (Zang et al., Reference Zang, Yan, Dong, Huang and Zang2012). The signed-path coefficient was used to reveal the Granger causal effects among brain regions which were considered to be normally distributed and therefore could be subjected to parametric statistical analysis for group level inference (Zang et al., Reference Zang, Yan, Dong, Huang and Zang2012). The models for signed-path coefficients were:


Specifically, we estimated the X-to-Y effects of the time series from each of the regions of interest (ROIs) in the striatum on every other brain region within the whole brain, and the Y-to-X effects of the time series of every other brain region within the whole brain on the striatal ROIs. Finally, we employed ANOVA and two-sample t tests to analyze the differences in GCA coefficients between the three groups. Gaussian Random Field theory was used for cluster-level multiple comparisons correction (cluster level p < 0.05, voxel level p < 0.01, corrected).
Data availability
The datasets generated during and/or analyzed during the current study are available to other researchers upon request of the corresponding authors.
Results
Demographic and clinical variables
The demographic and clinical characteristics of the three groups are presented in Table 1. There were no differences in gender (χ2 = 4, p = 0.13) and education levels (F = 0.38, p = 0.68) across the three groups. The age of the unaffected first-degree relative group was significantly higher than the OCD and healthy control groups (F = 22.3, p = 0.01). OCD patients had significantly higher scores on YBOC, OCI-R, BDI, and STAI than the healthy control and unaffected first-degree relative groups.
Table 1. Demographic and clinical characteristics of participants
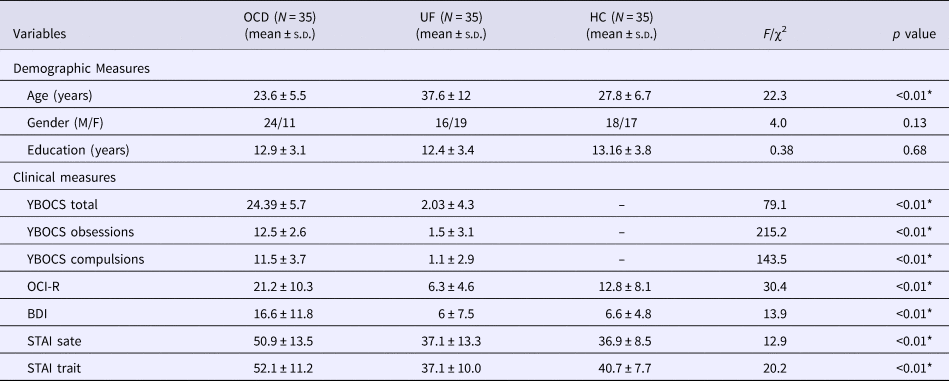
BDI, Beck Depression Inventory; HC, healthy control group; OCD, Obsessive–compulsive disorder group; OCI-R, Obsessive Compulsive Inventory-Revised; UF, unaffected first-degree relatives; YBOCS, Yale-Brown Obsessive-Compulsive Scale; SD, standard deviation; STAI, State-Trait Anxiety Inventory.
* p < 0.01.
GCA analysis of the nucleus accumbens
In the left nucleus accumbens, the results revealed (Fig. 1, Table 2) that OCD patients showed significantly greater influence from the left nucleus accumbens to the left anterior cingulate cortex, right medial orbitofrontal cortex, left middle temporal gyrus, and left superior temporal gyrus than healthy controls. In addition, OCD patients showed increased influence from the left posterior cingulate cortex, right thalamus, bilateral precuneus, and right precentral area to the left nucleus accumbens when compared with healthy controls (Fig. 2).

Fig. 1. Between-group differences in Granger connectivity from left striatal ROIs to other brain regions. HC, healthy control group; OCD, obsessive–compulsive disorder group; ROI, regions of interest; UF, unaffected first-degree relatives; Overlap, overlap regions between OCD compared with HC and UF compare with HC (GRF correction, cluster level p < 0.05, voxel level p < 0.01, corrected).

Fig. 2. Between-group differences in Granger connectivity to left striatal ROIs from other brain regions. HC, healthy control; OCD, obsessive–compulsive disorder group; ROI, regions of interest; UF, unaffected first-degree relatives; Overlap, overlap regions between OCD compared with HC and UF compare with HC (GRF correction, cluster level p < 0.05, voxel level p < 0.01, corrected).
Table 2. GCA coefficients difference among three groups of the left nucleus accumbens

BA, Brodmann's areas; HC, healthy control group; L, left hemisphere; MNI, Montreal Neurological Institute; OCD, obsessive–compulsive disorder group; R, right hemisphere; ROI, regions of interest; UF, unaffected first-degree relatives; X-to-Y, the effective connectivity from the whole brain to ROI; Y-to-X, the effective connectivity from the ROI to the whole brain (GRF correction, cluster level p < 0.05, voxel level p < 0.01, corrected).
In the right nucleus accumbens, the results revealed that OCD patients showed significantly greater influence from the right nucleus accumbens to the left orbitofrontal cortex, left inferior frontal gyrus than healthy controls (Fig. 3). In addition, OCD patients showed increased influence from the right cerebellum and left medial frontal gyrus to right nucleus accumbens when compared with healthy controls (Fig. 4).
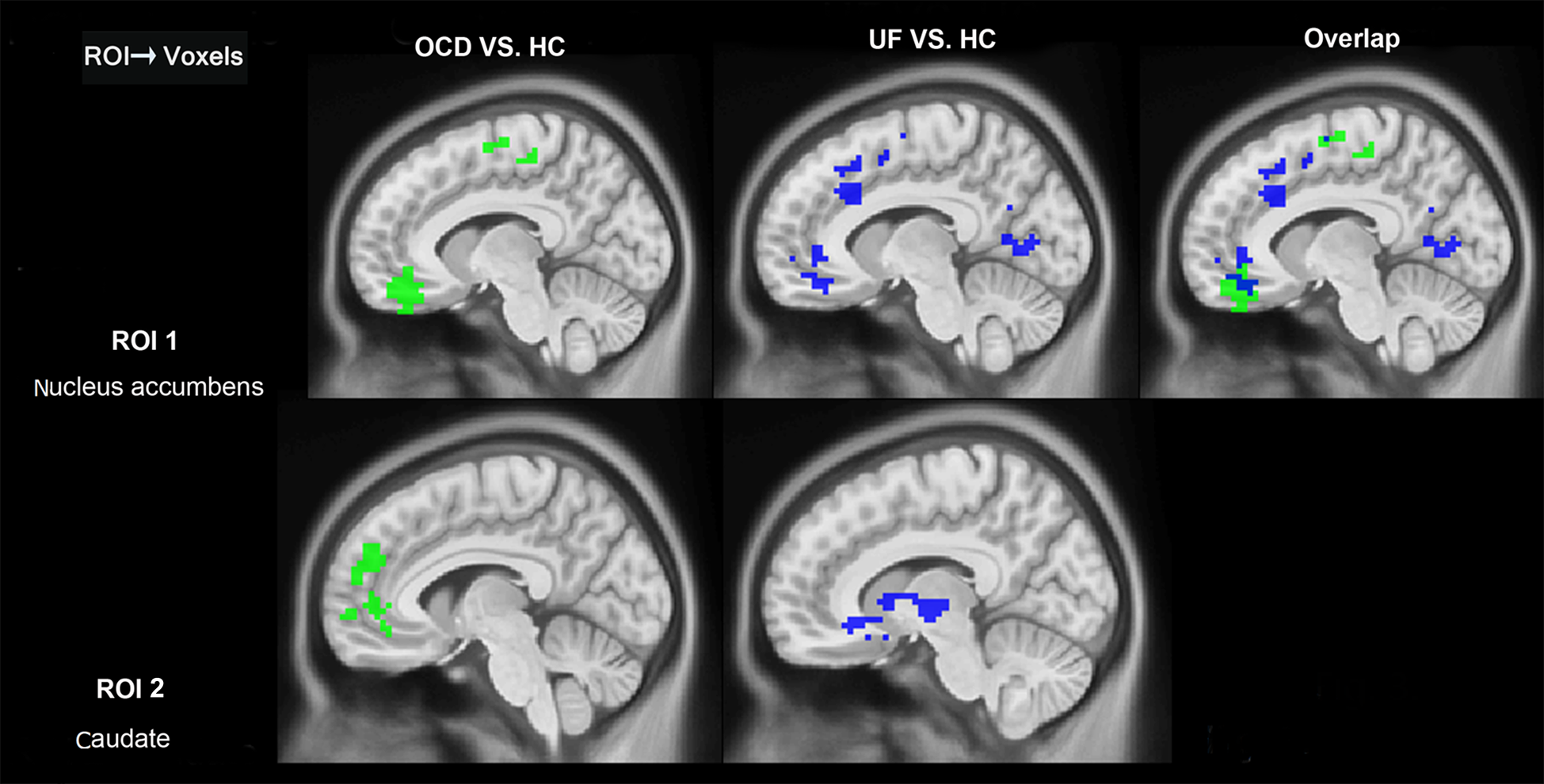
Fig. 3. Between-group differences in Granger connectivity from right striatal ROIs to other brain regions. HC, healthy control group; OCD, obsessive–compulsive disorder group; ROI, regions of interest; UF, unaffected first-degree relatives; Overlap, overlap regions between OCD compared with HC and UF compare with HC (GRF correction, cluster level p < 0.05, voxel level p < 0.01, corrected).

Fig. 4. Between-group differences in Granger connectivity to right nucleus accumbens and right caudate from other brain regions. HC, healthy control group; OCD, obsessive–compulsive disorder group; ROI, regions of interest; UF, unaffected first-degree relatives; Overlap, overlap regions between OCD compared with HC and UF compare with HC (GRF correction, cluster level p < 0.05, voxel level p < 0.01, corrected).
The first-degree relatives showed similar patterns as the OCD patients. Specifically (Fig. 1, Table 2), in the left nucleus accumbens, the first-degree relatives showed significantly increased influence from left nucleus accumbens to left middle temporal gyrus and left superior temporal gyrus than healthy controls. The first-degree relatives also showed (Fig. 2, Table 2) significantly increased influence from left posterior cingulate and bilateral precuneus to the left nucleus accumbens. In the right nucleus accumbens, the first-degree relatives showed significantly greater influence from the right nucleus accumbens to the left orbitofrontal cortex than healthy controls (Fig. 3). When OCD patients were compared with first degree relatives, the OCD patients showed a significantly decreased influence of the right anterior cingulate cortex to the left nucleus accumbens.
GCA analysis of the caudate
In the left caudate, the results revealed (Fig. 1, Table 3) that OCD patients showed significantly greater influence from the left caudate to the bilateral medial orbitofrontal cortex than healthy controls. However, OCD patients showed (Fig. 2, Table 3) decreased influence from left medial frontal gyrus, bilateral anterior cingulate cortex, right superior frontal gyrus, and right middle frontal gyrus to the left caudate than healthy controls. In the right caudate, OCD patients showed significantly greater influence from the right caudate to the left medial frontal gyrus than healthy controls.
Table 3. GCA coefficients difference among three groups of the left caudate

BA, Brodmann's areas; HC, healthy control group; L, left hemisphere; MNI, Montreal Neurological Institute; OCD, obsessive–compulsive disorder group; R, right hemisphere; ROI, regions of interest; UF, unaffected first-degree relatives; X-to-Y, the effective connectivity from the whole brain to ROI; Y-to-X, the effective connectivity from the ROI to the whole brain (GRF correction, cluster level p < 0.05, voxel level p < 0.01, corrected).
Compared with healthy controls, the first-degree relatives showed (Fig. 1, Table 3) significantly greater influence from the left caudate to a bilateral medial orbital frontal gyrus, and (Fig. 2, Table 3) significantly decreased influence from right superior frontal gyrus, right middle frontal gyrus to left caudate. In the right caudate, the first-degree relatives showed significantly greater influence from the right caudate to the right putamen and inferior frontal gyrus than healthy controls (Fig. 3). The patterns of directed influence for the right caudate within the healthy control group did not overlap with those found in the OCD patients and the first-degree relatives.
GCA analysis of the putamen
In the left putamen, the results revealed that (Fig. 1, Table 4) patients with OCD showed a significantly greater influence from the left putamen to the insula, and greater influence from left middle frontal gyrus and right cerebellum to the putamen than healthy controls. In the right putamen, patients with OCD showed significantly increase EC from PCC to the right putamen and increase EC from left inferior frontal gyrus, left angular and bilateral cerebellum to the right putamen than healthy controls. In addition, first-degree relatives showed significantly greater influence from left ACC and left angular to the right putamen than healthy controls and patients with OCD (Fig. 5).
Table 4. GCA coefficients difference among three groups of the left putamen

BA, Brodmann's areas; HC, healthy control group; L, left hemisphere; MNI, Montreal Neurological Institute; OCD, obsessive–compulsive disorder group; R, right hemisphere; ROI, regions of interest; UF, unaffected first-degree relatives; X-to-Y, the effective connectivity from the whole brain to ROI; Y-to-X, the effective connectivity from the ROI to the whole brain (GRF correction, cluster level p < 0.05, voxel level p < 0.01, corrected).
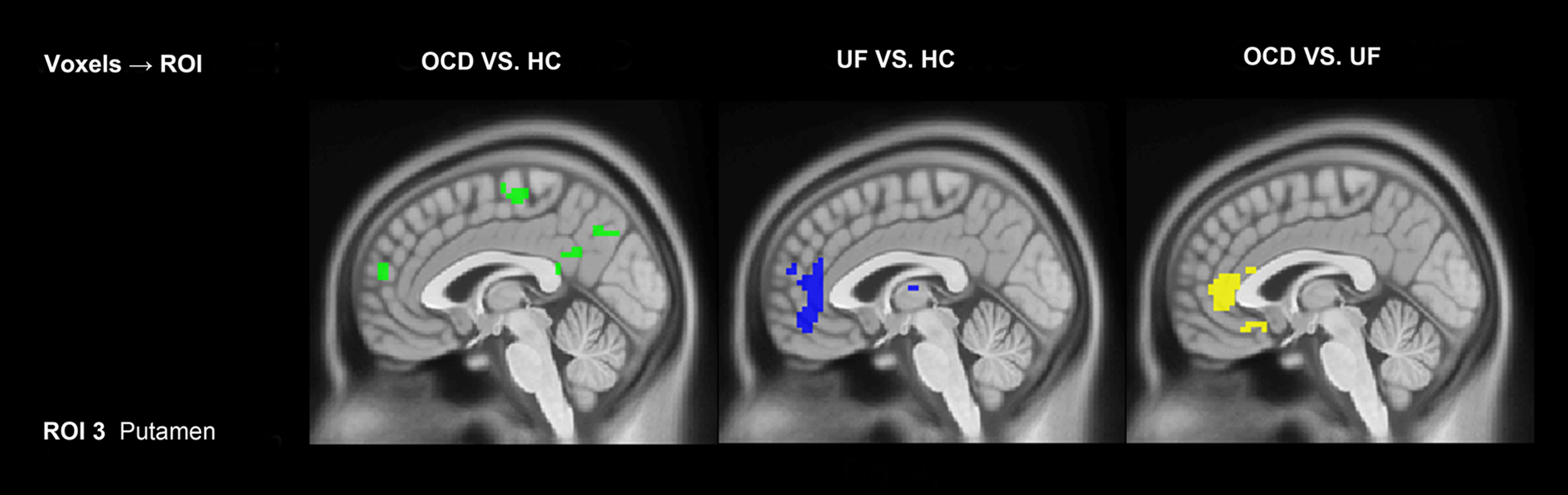
Fig. 5. Between-group differences in Granger connectivity to right putamen from other brain regions. HC, healthy control group; OCD, obsessive–compulsive disorder group; ROI, regions of interest; UF, unaffected first-degree relatives; Overlap, overlap regions between OCD compared with HC and UF compare with HC (GRF correction, cluster level p < 0.05, voxel level p < 0.01, corrected).
Further analysis of within-subjects associations between symptoms and effective connectivity of these ROIs in the OCD group did not reveal any additional significant associations.
Discussion
In the present study, we investigated EC within cortical-striatal systems in OCD patients and their unaffected first-degree relatives. We found distinct corticostriatal networks across three primary striatal regions: the caudate, nucleus accumbens, and putamen, consistent with previous studies (Di Martino et al., Reference Di Martino, Scheres, Margulies, Kelly, Uddin, Shehzad, Biswal, Walters, Castellanos and Milham2008), and consistent with the known connectivity of these regions within the associative, limbic, and motor corticostriatal loops, respectively (Jahanshahi et al., Reference Jahanshahi, Obeso, Rothwell and Obeso2015). Both OCD patients and their first-degree relatives showed changes in EC between both the caudate and nucleus accumbens and other areas of the brain, including greater EC from PCC to left nucleus accumbens, and from left caudate to OFC, and decreased EC from DLPFC to left caudate (Fig. S4). Consistent with previous results these abnormal EC patterns followed the anatomical connections present within the associative corticostriatal system (Harrison et al., Reference Harrison, Soriano-Mas, Pujol, Ortiz, López-Solà, Hernández-Ribas, Deus, Alonso, Yücel, Pantelis and Menchon2009; Hou et al., Reference Hou, Song, Zhang, Wu, Wang, Zhou, Qu, Guo, Gu, He, Xie and Li2013, Reference Hou, Zhao, Zhang, Song, Wu, Wang, Zhou, Xie, He, Guo, Qu and Li2014; Pauls et al., Reference Pauls, Abramovitch, Rauch and Geller2014; Vaghi et al., Reference Vaghi, Vértes, Kitzbichler, Apergis-Schoute, van der Flier, Fineberg, Sule, Zaman, Voon, Kundu, Bullmore and Robbins2017b), which underlies goal-directed behavior (Jahanshahi et al., Reference Jahanshahi, Obeso, Rothwell and Obeso2015). Our findings suggest that in OCD patients there is an impairment of the goal-directed behavioral learning system during the resting-state. Most importantly, the unaffected first-degree relatives of the OCD patients showed similar patterns, particularly in the associative corticostriatal circuit.
Dysfunctional connectivity of the OFC in OCD patients has been reported in a number of previous studies (Brennan et al., Reference Brennan, Rauch, Jensen and Pope2013; Anticevic et al., Reference Anticevic, Hu, Zhang, Savic, Billingslea, Wasylink, Repovs, Cole, Bednarski, Krystal, Bloch, Li and Pittenger2014). Although these studies have found abnormal functional connectivity of CSTC within OCD patients, most of these results provided only non-directional information leaving the directionality of the influences between regions unclear. Our results revealed that both OCD patients and their unaffected first-degree relatives exhibited enhanced effective connectivity from the caudate to the OFC, which suggests that dysfunction in the caudate-OFC cortical-striatal system may be a vulnerability marker of OCD and dysfunction of CSTC circuit may stem from enhanced influence from the caudate to the OFC. These findings were consistent with a previous study which revealed OCD patients and their unaffected first-degree relatives showed OFC dysfunction during reversal learning (Chamberlain et al., Reference Chamberlain, Menzies, Hampshire, Suckling, Fineberg, del Campo, Aitken, Craig, Owen, Bullmore, Robbins and Sahakian2008). The OFC and caudate are critical regions associated with the goal-directed behavioral learning system (Ostlund and Balleine, Reference Ostlund and Balleine2005; Valentin et al., Reference Valentin, Dickinson and O'Doherty2007; Wunderlich et al., Reference Wunderlich, Dayan and Dolan2012). Lesions in either of these two regions have been found to prevent the acquisition of goal-directed learning, even during the early stage of training (Yin et al., Reference Yin, Ostlund, Knowlton and Balleine2005; Balleine and O'Doherty, Reference Balleine and O'Doherty2010). Furthermore, another study found that during the reinforcement learning caudate incorporates the information about expected outcome signaled from OFC suggesting that orbitofrontal and striatal circuits dynamically encode the shift between goal-directed and habitual actions (McDannald et al., Reference McDannald, Lucantonio, Burke, Niv and Schoenbaum2011).
We found decreased EC from right DLPFC to caudate was accompanied by enhanced EC from caudate to OFC in OCD patients and their first-degree relatives. Anatomically the DLPFC projects primarily to the caudate, specifically the rostral central region of the caudate nucleus (Haber et al., Reference Haber, Kim, Mailly and Calzavara2006). Our results prompt that there are collateral but inverse connectivity between DLPFC, OFC and caudate on patients with OCD. DLPFC is a critical area for cognitive control and executive processes, including response inhibition, behavioral flexibility, and orienting of attention. Previous studies reported brain metabolic abnormalities in OCD in the right DLPFC (Park et al., Reference Park, Choi and Jeong2017). Furthermore, clinical treatment using repetitive transcranial magnetic stimulation (TMS) targeting the right DLPFC has been shown to improve obsessive symptoms (Seo et al., Reference Seo, Jung, Lim, Um, Lee and Chae2016). Dysfunction of the DLPFC may account for the poor performance of OCD patients and their first-degree relatives on some neuropsychological tests that require executive control in service to goal-directed behavior, such as Tower of London test (Cavedini et al., Reference Cavedini, Zorzi, Piccinni, Cavallini and Bellodi2010), and the Wisconsin card sorting test (Zhang et al., Reference Zhang, Yang and Yang2015; Benzina et al., Reference Benzina, Mallet, Burguière, N'Diaye and Pelissolo2016).Previous studies have identified reduced lateral prefrontal cortex activation in both OCD patients and their unaffected first-degree relatives during a reversal-learning task, leading to the proposal that lateral prefrontal cortex dysfunction may be a biomarker of OCD (Menzies et al., Reference Menzies, Chamberlain, Laird, Thelen, Sahakian and Bullmore2008a; Vaghi et al., Reference Vaghi, Hampshire, Fineberg, Kaser, Brühl, Sahakian, Chamberlain and Robbins2017a). Our results extend this work by suggesting that dysfunctional EC between DLPFC and caudate within the goal-directed system may also serve as a biomarker of OCD.
We also found group differences in effective connectivity with the nucleus accumbens. OCD patients compared with healthy controls showed greater EC from PCC to nucleus accumbens and from nucleus accumbens to the lateral temporal area. These two regions are critically involved in the default mode network (Buckner et al., Reference Buckner, Andrews-Hanna and Schacter2008), which exhibit increased activation during internal cognitive processing, but decreased activation during externally goal-directed cognitive tasks (Buckner et al., Reference Buckner, Andrews-Hanna and Schacter2008). Previous studies have found abnormal connectivity associated with OCD symptoms within DMN (Stern et al., Reference Stern, Fitzgerald, Welsh, Abelson and Taylor2012; Peng et al., Reference Peng, Xu, He, Shi, Wei, Miao, Jing, Lim, Zuo and Chan2014). Moreover, a previous study in our lab suggested that disruptions of PCC functional connectivity might be associated with family history of OCD (Peng et al., Reference Peng, Xu, He, Shi, Wei, Miao, Jing, Lim, Zuo and Chan2014). The current study further confirms our previous findings that dysfunction in the PCC is tightly associated with the goal-directed system and suggests that this dysfunction may be a biomarker for OCD.
In addition to common patterns of abnormal EC shared by OCD patients and their first-degree relatives, we also found some changes in EC that were exhibited only by OCD patients. These include changes of EC in limbic regions, including increased EC from nucleus accumbens to ACC and OFC, and decreased EC from OFC and ACC to caudate. These patterns are consistent with previous studies (Abe et al., Reference Abe, Sakai, Nishida, Nakamae, Yamada, Fukui and Narumoto2015), and indicate that OCD patients exhibit hyper-influence from OFC and ACC to the ventral striatum, areas that are known to interact within the limbic cortical-striatal loop (Jahanshahi et al., Reference Jahanshahi, Obeso, Rothwell and Obeso2015). Dysfunction of this loop may contribute to anxiety and depression symptoms which may exacerbate intrusive thoughts in OCD patients.
Overall, we found little change in EC with the putamen. Consistent with functional connectivity study in OCD patients (Stern et al., Reference Stern, Fitzgerald, Welsh, Abelson and Taylor2012; Jhung et al., Reference Jhung, Ku, Kim, Lee, Kim, An, Kim, Yoon and Lee2014; Bernstein et al., Reference Bernstein, Mueller, Schreiner, Campbell, Regan, Nelson, Houri, Lee, Zagoloff, Lim, Yacoub and Cullen2016), we found a small region of the insula that showed increased EC from the putamen in OCD patients only. We found no changes in EC with areas such as the premotor cortex and SMA that are involved in the habit system, which is associated with automatic behaviors (Dolan and Dayan, Reference Dolan and Dayan2013). We did not find abnormal EC between SMA and other motor areas and the putamen. Therefore, we conservatively suggest that the compulsions and deficits in cognitive performance in OCD patients are mainly associated with abnormal activity in the goal-directed system rather than the habit system. While as the human behavior is an artful coordinate between the goal-directed system and habitual system, we speculate that an abnormal EC in the goal-directed system may potentially be associated with an imbalance of the function between goal-directed system and habitual system. For example, the previous study using a symptom provocation task found that OCD patients showed deactivation in caudate-prefrontal circuits and hyper-activation in the putamen, which suggested that an imbalance between habitual systems and goal-directed neural systems exists in OCD patients during symptom provocation (Banca et al., Reference Banca, Voon, Vestergaard, Philipiak, Almeida, Pocinho, Relvas and Castelo-Branco2015).
More generally, differences between OCD patients and their first-degree relatives may explain why OCD patients suffered from the typical symptoms of OCD, while their first-degree relatives failed to show compulsive symptoms. In addition, we found little overlap abnormal EC in the right ROIs of OCD patients and first-degree relatives. This may reflect the cortical asymmetries and different processing between left and right hemisphere. Our previous work has also found that OCD patients and siblings exhibited leftward asymmetry in the ACC (Peng et al., Reference Peng, Li, Shi, Shi, Yang, Chan and Shen2015). The association between cortical asymmetries and function difference in OCD patients remains to be further studied.
The present findings have to be interpreted in light of several limitations. (1) OCD is a heterogeneous disorder (Pauls et al., Reference Pauls, Abramovitch, Rauch and Geller2014), and previous studies have suggested that different symptoms of OCD may be associated with different neural mechanisms (Harrison et al., Reference Harrison, Pujol, Cardoner, Deus, Alonso, Lopez-Sola, Contreras-Rodriguez, Rea, Segalas, Blanco-Hinojo, Menchon and Soriano-Mas2013). (2) The sample of OCD first-degree relatives included both siblings and parents, resulting in a significantly higher age of first-degree relatives than the OCD group and control group. To test whether this difference in age might have affected the results, we repeated the analyses comparing OCD, and healthy control groups with age-matched OCD siblings only. The results did not differ significantly (see online Supplemental Materials). (3) The OCD patients in the present study were for the most part being treated with antidepressants along with Cognitive-Behavioral Therapy, and it is possible that the results are affected by these medications. (4) The onset time and illness duration varied across OCD patients. Previous studies have suggested that childhood-onset OCD is a distinct neurodevelopmental form of the disorder (Pauls et al., Reference Pauls, Abramovitch, Rauch and Geller2014). (5) Because of the small OCD sample size in the current study and differences across subjects in the severity of comorbidity, it is difficult to test whether our results are affected by comorbidity; further studies using the larger sample, different subtypes and drug-naive samples are required to confirm our preliminary results.
In conclusion, we used GCA analysis to investigate EC within the limbic, association, and motor cortical-striatal networks in OCD patients and their first-degree relatives. We found that both OCD patients and their first-degree relatives showed abnormal EC in the associative network, specifically between caudate and prefrontal cortex, associated with goal-directed learning and behavior. In addition, OCD patients and first-degree relatives also had abnormal patterns of connectivity within the limbic corticostriatal network, specifically between nucleus accumbens and OFC, associated with reward processing. The results suggest that dysfunction in the goal-directed cortical-striatal system may be a vulnerability marker for obsessive-compulsive disorder.
Supplementary material
The supplementary material for this article can be found at https://doi.org/10.1017/S0033291719001429
Acknowledgements
This study was partly supported by the National Natural Science Foundation of China (31871113 and 31671135), the project of Shenzhen Science and Technology Innovation Committee (JCYJ20160429185235132, JCYJ20160427192001852), the Sanming Project of Medicine in Shenzhen (SZSM201612079, SZSM201512009) and the Guangdong Province Universities and Colleges Pearl River Scholar Funded Scheme (2016) to Qi Chen. And we thank Zhen Wei, who works in the Shenzhen Maternity and Child Healthcare Hospital, for her suggestions on the patient recruitment.
Author contributions
Chenjie Dong, Qi Chen and Ziwen Peng designed the study, preprocessed the data and wrote the manuscript. Jingjing Liang and Qiong Yang collected data and collaborated in manuscript writing. Carol A. Seger collaborated in manuscript writing and revision. Qi Chen revised the manuscript and processed the data. Hongying Han, Yuping Ning and Qiong Yang helped with patient recruitment and psychiatric assessment. All authors read and approved the final manuscript.
Conflict of interest
The authors declare no competing financial interests.
Ethical standards
The authors assert that all procedures contributing to this work comply with the ethical standards of the relevant national and institutional committees on human experimentation and with the Helsinki Declaration of 1975, as revised in 2008.