1. Introduction
The components and nature of early Precambrian basement rocks can provide the key to understanding the growth, reworking and evolution of the early Precambrian continental crust (Cawood et al. Reference Cawood, Hawkesworth and Dhuime2013). The South China Craton (SCC), one of the three major cratons in China including the North China Craton (NCC) and Tarim craton (TC), consists of the Yangtze Plate and Cathaysia Block (Fig. 1a), and has only sparse outcrops of early Precambrian basement rocks mainly on the northern margin of the Yangtze Plate (Wan et al. Reference Wan, Liu, Xie, Dong, Li, Bai and Liu2018); early Precambrian crustal growth, reworking and evolution is therefore not well understood. On this account, detrital zircons in sedimentary rocks and/or xenocrystic zircons brought to the surface from the deep crust have provided important clues for insights into the early Precambrian crustal formation and evolutionary history of the SCC, and confirmed widespread Archaean basement beneath the SCC (Zheng et al. Reference Zheng, Griffin, O’Reilly, Zhang, Pearson and Pan2006, Reference Zheng, Griffin, Li, O’Reilly, Pearson, Tang, Liu, Zhao, Yu and Su2011; Yu et al. Reference Yu, O’Reilly, Wang, Griffin, Zhou, Zhang and Shu2010). The Wulian complex is located on the northern margin of the Sulu orogenic belt formed by collision between the NCC and SCC in eastern China and consists of the Neoproterozoic metasedimentary Wulian Group, gneissic granite and meta-diorite. Recently, some studies have confirmed that the Wulian Group has tectonic affinity to the SCC based on the intrusive relationship with the Neoproterozoic gneissic granite of SCC affinity (Zhou et al. Reference Zhou, Wilde, Zhao, Zhang, Zheng, Jin and Cheng2008a ; Wang et al. Reference Wang, Xiang, Zhang, Lu, Song and Yang2012). The Wulian complex represents a tectonic wedge scraped from the Yangtze Plate during the subduction of the SCC towards the NCC and their collision during the Triassic Period (Zheng et al. Reference Zheng, Zhou, Wu and Xie2005; Zhou et al. Reference Zhou, Wilde, Zhao, Zhang, Zheng, Jin and Cheng2008a ). Detrital zircons from the Wulian Group and xenocrystic zircons from the meta-diorite (gabbro) can therefore be used to decipher the crustal formation and evolutionary history of the SCC.

Fig. 1. (a) Schematic tectonic map of China showing the major Precambrian blocks connected by Phanerozoic fold belts (modified after Zhao et al. Reference Zhao, Wilde, Cawood and Sun2001). (b) Geological sketch map of the Sulu orogenic belt and adjacent parts of central-eastern China (modified after Faure et al. Reference Faure, Lin and Le Breton2001; Hacker et al. Reference Hacker, Wallis, Ratschbacher, Grove and Gehrels2006; Zhou et al. Reference Zhou, Wilde, Zhao, Zheng, Jin, Zhang and Cheng2008b).
Detrital zircon U–Pb dating coupled with Hf isotope analysis can play a powerful role in determining the provenance, tectonic setting, maximum depositional age and tectonic affinity of sedimentary strata, as well as in gaining valuable information about major thermal events, crustal growth and evolution of source terranes (Gehrels et al. Reference Gehrels, Dickinson, Ross, Stewart and Howell1995; Amidon et al. Reference Amidon, Burbank and Gehrels2005a, b; Cawood et al. Reference Cawood, Hawkesworth and Dhuime2012; Ding et al. Reference Ding, Qasim, Jadoon, Khan, Xu, Cai, Wang, Baral and Yue2016; Long et al. Reference Long, Xu, Yuan, Zhang and Zhang2019). Conventionally, provenances and tectonic affinities of sedimentary strata can be determined by linking a population of similarly aged zircons and Hf isotope compositions in a given sedimentary sample to a specific source terrane. Detrital zircon ages generally correspond to tectono-magmatic-metamorphic thermal events, while their Hf isotopes can be used to constrain crustal growth and reworking in their source terrane.
In this study, we conducted integrated geological mapping, single-grain U–Pb dating and Hf isotope analyses on zircons from the Wulian Group, Neoproterozoic gneissic granite and meta-diorite of the Wulian complex. The results in combination with those from previous studies further confirm the tectonic affinity and provenance of the Wulian Group, and provide significant insights into early Precambrian crustal growth, recycling and evolution in the SCC.
2. Geological setting
Continental China consists of three major Precambrian continental blocks (NCC, SCC and TC) that are sutured by Phanerozoic orogenic belts (Fig. 1a; Zhao & Cawood, Reference Zhao and Cawood2012). The Qinling–Dabie–Sulu orogenic belt separating the NCC to the north and the SCC to the south were involved in multiple periods of orogeny (Fig. 1a; Ren et al. Reference Ren, Wang, Chen, Jiang, Niu, Li, Xie, He and Liu1999; Zhang et al. Reference Zhang, Zhang and Yuan2001a , 2019; Zheng, Reference Zheng2008; Dong & Santosh, Reference Dong and Santosh2016; Yu et al. Reference Yu, Zhang and Li2017, Reference Yu, Li, Zhang, Liu, Peng and Li2019a , b) and is well known as one of the largest ultra-high-pressure (UHP) metamorphic belts in the world, which formed by subduction and collision between the NCC and SCC during the Triassic Period (Zheng, Reference Zheng2008; Liou et al. Reference Liou, Ernst, Zhang, Tsujimori and Jahn2009). The Sulu orogenic belt is thought to be separated from the Qinling–Dabie orogenic belt by the Tanlu fault (Fig. 1a; Yin & Nie, Reference Yin and Nie1993), and mainly consists of HP–UHP metamorphic rocks, Neoproterozoic low-grade metamorphic rocks, and Mesozoic–Cenozoic sedimentary rocks and intrusions (Fig. 1b; Zhang et al. Reference Zhang, Tang and Zheng2014; Liu et al. Reference Liu, Liu, Liu, Wang, Cai, Liu, Wang and Ji2017; Zhao et al. Reference Zhao, Wang, Deng, Santosh, Liu, Liang and Cheng2019). The HP–UHP metamorphic rocks include various metamorphic supracrustal rocks, Neoproterozoic granitic gneisses, mafic-ultramafic rocks, and blocks of eclogite and minor blueschist that are generally considered to have tectonic affinity to the SCC (Tang et al. Reference Tang, Zheng, Wu, Gong, Zha and Liu2008; Zhang et al. Reference Zhang, Tang and Zheng2014; Liu et al. Reference Liu, Liu, Liu, Wang, Cai, Liu, Wang and Ji2017; Liu & Massonne, Reference Liu and Massonne2019). The protolith ages of the HP–UHP metamorphic rocks determined by U–Pb dating of magmatic zircons show two groups with ages of 1950–1740 Ma and 850–750 Ma, and the latter is dominant (Zhang et al. Reference Zhang, Tang and Zheng2014; Liu et al. Reference Liu, Liu, Liu, Wang, Cai, Liu, Wang and Ji2017). Previous studies have demonstrated that the HP–UHP metamorphism occurred during 250–215 Ma (Zhang et al. Reference Zhang, Yang, Wooden, Liou and Li2005; Hacker et al. Reference Hacker, Wallis, Ratschbacher, Grove and Gehrels2006; Liu & Liou, Reference Liu and Liou2011). Neoproterozoic low-grade metamorphosed rocks mainly include the Shiqiao–Pingshang low-grade metasedimentary rocks and the Wulian Group (Fig. 1b). The detrital zircon U–Pb ages suggest that the Shiqiao–Pingshang low-grade metamorphosed rocks were sourced from the NCC (Zhou et al. Reference Zhou, Wilde, Zhao, Zheng, Jin, Zhang and Cheng2008b ). Recently, high-grade metamorphic Archaean tonalite-trondhjemite-granodiorite (TTG) and Palaeoproterozoic granites, metamorphosed supracrustal rocks and mafic-ultramafic rocks have been found to occur in the Haiyangsuo on the southern margin of the Sulu orogenic belt; these rocks are comparable to those of the Jiaobei Terrane, NCC (Fig. 1b), as identified by Liu et al. (Reference Liu, Liu, Liu, Wang, Cai, Liu, Wang and Ji2017).
The Wulian complex is located on the northern margin of the southwestern Sulu orogenic belt (Fig. 1b) and consists of the Wulian Group and Neoproterozoic meta-diorite and gneissic granites (Fig. 2). The Wulian Group has been subdivided into the Haiyankou and Kunshan formations from the base to top (Fig. 2). The Haiyankou Formation can further be subdivided into lower and upper Haiyankou members. The lower Haiyankou member is mainly composed of biotite-bearing plagioclase gneisses with layers of amphibolites- and biotite-bearing leptynite, which is conventionally defined by fine granular crystalloblastic texture and major mineral assemblage of feldspar and quartz; the upper Haiyankou member comprises tourmaline-bearing leptynite, biotite schist and grey-white marble and the Kunshan Formation is mainly composed of brown-red marbles with layers of tourmaline-bearing quartzite, carbonaceous slate and mica-schist (Wang et al. Reference Wang, Xiang, Zhang, Lu, Song and Yang2012). Neoproterozoic SCC-type microfossils that occur in the Wulian Group, including Shandongsolenopora orientalis, Wuliansolenopora Kunshannensis and Wuliansolenopora jiaonanensis, have been reported by Zhao et al. (Reference Zhao, Cheng and Liu1995). In addition, both the Haiyankou and Kunshan formations are intruded by Neoproterozoic meta-diorite and gneissic granite (Fig. 2), which have emplacement ages of 778–738 Ma (Zhou et al. Reference Zhou, Zheng and Wu2003; Wu et al. Reference Wu, Zheng and Zhou2004; Wang et al. Reference Wang, Xiang, Zhang, Lu, Song and Yang2012) and have been suggested to be of SCC affinity by geochronologic, geochemical and oxygen isotope investigations (Faure et al. Reference Faure, Lin and Le Breton2001, Reference Faure, Lin, Monié, Breton, Poussineau, Panis and Deloule2003; Zhou et al. Reference Zhou, Zheng and Wu2003; Wu et al. Reference Wu, Zheng and Zhou2004; Zheng et al. Reference Zheng, Zhou, Wu and Xie2005). The Wulian complex is generally characterized by the occurrence of fold-and-thrust faults and has been considered to be a tectonic wedge formed by subduction of the SCC towards the NCC during the Triassic Period (Zheng et al. Reference Zheng, Zhou, Wu and Xie2005; Zhou et al. Reference Zhou, Wilde, Zhao, Zhang, Zheng, Jin and Cheng2008a ).

Fig. 2. Detailed geological map of the Wulian complex and sampling locations (modified after Wang et al. Reference Wang, Xiang, Zhang, Lu, Song and Yang2012).
The Jiaobei Terrane to the NW of the Sulu orogenic belt consists of a Precambrian metamorphic basement, Mesozoic magmatic rocks and Mesozoic–Cenozoic sedimentary rocks. The Precambrian metamorphic basement is composed mainly of Archean meta-mafic and meta-supracrustal rocks, Archean granitoid gneisses, Palaeoproterozoic granitoid and mafic intrusions, the Palaeoproterozoic high-grade metamorphosed Fenzishan and Jingshan groups, and the Neoproterozoic low-grade metamorphosed Penglai Group (Fig. 1b; Jahn et al. Reference Jahn, Liu, Wan, Song and Wu2008; Zhou et al. Reference Zhou, Wilde, Zhao, Zheng, Jin, Zhang and Cheng2008c , d; Liu et al. Reference Liu, Liu, Ding, Liu, Yang, Liu, Wang and Meng2013a , b, c, 2014a, b). In addition, meta-sedimentary rocks of SCC affinity across the Wulian–Yantai fault, which has been considered as a tectonic boundary between the SCC and NCC, have also been reported in the Jiaobei Terrane (Tang et al. Reference Tang, Zheng, Wu and Gong2006, Reference Tang, Zheng, Wu, Gong and Liu2007). The Jiaobei Terrane is characterized by c. 2.5, 2.7 and 2.9 Ga TTG-granitic magmatic events and c. 2.5 and c. 1.95–1.85 Ga metamorphic events that prevailed in the NCC (Zhao et al. Reference Zhao, Sun, Wilde and Li2005; Zhai & Santosh, Reference Zhai and Santosh2011; Zhao & Zhai, Reference Zhao and Zhai2013; Liu et al. Reference Liu, Liu, Ding, Liu, Yang, Liu, Wang and Meng2013a , 2015); the Jiaobei Terrane is therefore considered to have tectonic affinity to the NCC.
3. Analytical methods
3.a. Zircon U–Pb dating
Zircon grains were separated from the eight samples collected from the Wulian complex using a combination of heavy liquid and magnetic separation techniques. The zircon grains used for laser ablation inductively coupled plasma mass spectrometry (LA-ICP-MS) U–Pb dating were mounted in epoxy and polished until their cores were exposed. Cathodoluminescence (CL) images of the zircon grains were obtained using a Chroma CL emitter on a Hitachi S-3000N electron microprobe (GATAN) at the Beijing SHRIMP Centre, Chinese Academy of Geological Sciences (CAGS), Beijing, China.
Zircon U–Pb dating was carried out at the Geological Lab Centre, China University of Geosciences, Beijing, China. An Agilent 7500a quadruple ICP-MS and a Neptune ICP-MS were used for simultaneous determination of zircon U–Pb ages and trace elements with a 193-nm Excimer laser ablation system. The laser beam diameter was 30 μm and it was operated at a frequency of 10 Hz. The zircon standards were 91500 and Temora, and the glass standard was NIST612. The analytical procedures are described in detail by Song et al. (Reference Song, Niu, Wei, Ji and Su2010). The zircon U–Th–Pb data are presented in online Supplementary Table S1 (available at http://journals.cambridge.org/geo).
3.b. In situ zircon Lu–Hf isotope analyses
Zircon in situ Lu–Hf isotopes were analysed using a Nu Plasma II multi-collector ICP-MS coupled to a 193 nm laser ablation system Resolution M-50, ASI, at the State Key Laboratory of Continental Dynamics, Northwest University, China. Details of the instrumental conditions and analytical procedures are described by Yuan et al. (Reference Yuan, Gao, Dai, Zong, Günther, Fontaine, Liu and Diwu2008). Zircon Lu–Hf isotope analyses were conducted using a spot size of 44 μm on spots previously analysed for U–Pb dating or within the same zones. The zircon standards 91500 and MT were analysed as unknown samples and yielded weighted mean 176Hf/177Hf ratios of 0.282328 ± 0.000003 (mean square weighted deviation (MSWD), 1.8; n = 227) and 0.282534 ± 0.000004 (MSWD, 1.9; n = 109), respectively, consistent with the 176Hf/177Hf ratios reported by Yuan et al. (Reference Yuan, Gao, Dai, Zong, Günther, Fontaine, Liu and Diwu2008) for the standards. The values of ϵ Hf, T Hf and f Lu/Hf were defined in this study following Wu et al. (Reference Wu, Li, Zheng and Gao2007). Lu–Hf isotope data are listed in online Supplementary Table S2 (available at http://journals.cambridge.org/geo).
4. Results
The sampling locations, simplified descriptions and mineral assemblages of the samples for zircon LA-ICP-MS U–Pb dating and Hf isotope analyses are presented in Table 1. Zircon LA-ICP-MS U–Pb dating was conducted on one quartzite sample (LX05-2), three leptynite samples (LX06-3, LX06-4 and LX06-5), two marble samples (LX03-2 and LX07-1), one gneissic granite sample (LX03-4) and one meta-diorite sample (LX04-1) collected from the Wulian complex. The results are presented in online Supplementary Table S1. A total of 210 Hf isotope analyses were conducted on zircons from quartzite sample LX05-2, leptynite sample LX06-5, two marble samples LX03-2 and LX07-1, Neoproterozoic gneissic granite sample LX03-4 and meta-diorite sample LX04-1. The Hf isotope data are presented in online Supplementary Table S2.
Table 1. A summary of lithologies, major mineral assemblages and coordinates for the samples from the Wulian Complex in the Sulu orogenic belt, China

4.a. Zircon U–Pb ages
4.a.1. Quartzite
Sample LX05-2, a fine-grained muscovite-bearing quartzite, was collected from the Kunshan Formation to the east of Kunshan (Fig. 2). The sample shows massive structure (Fig. 3a) and comprises quartz (90%) and directionally oriented muscovite (10%) (Fig. 3b). Most zircon grains from quartzite sample LX05-2 are subhedral to anhedral, have been subjected to mechanical damage, and exhibit oscillatory and comb zoning patterns, indicating their igneous origin; in contrast, some zircons display homogeneous or nebulous internal texture or typical core–rim patterns (Fig. 4a), indicating their metamorphic origins. A total of 99 U–Pb analyses were performed on 99 zircon grains, and the 68 analyses with discordance < 10% yield various apparent 207Pb/206Pb ages ranging from 1847 to 3307 Ma (Fig. 5a and Supplementary Table S1). They exhibit variable Th/U ratios varying from 0.16 to 2.26 (online supplementary Table S1). Four U–Pb analyses (spots 33, 67, 85 and 90) for metamorphic zircons obtain apparent 207Pb/206Pb ages of 2710, 1989, 2498 and 2501 Ma with ratios of 0.36, 0.73, 1.13 and 0.60, respectively (Fig. 4a; online Supplementary Table S1). The apparent 207Pb/206Pb age histogram exhibits one dominant age population of 2600–2400 Ma with two peak ages of 2445 and 2495 Ma and minor age peaks at 2030, 2175 and 2700 Ma (Fig. 5b).

Fig. 3. Photographs of outcrops and photomicrographs of quartzite sample (a, b) LX05-2 and leptynite samples (c, d) LX06-3, (e, f) LX06-4 and (g, h) LX06-5, collected from the Wulian Group. Bt – biotite; Mus – muscovite; Pl – plagioclase; Qtz – quartz.

Fig. 4. Representative cathodoluminescence (CL) images of zircons from quartzite sample LX05-2, leptynite samples LX06-3, LX06-4 and LX06-5, marble samples LX03-2 and LX07-1, Neoproterozoic gneissic granite sample LX03-4 and meta-diorite sample LX04-1 collected from the Wulian complex. The circles show positions of U–Pb dating spots with ages (Ma).
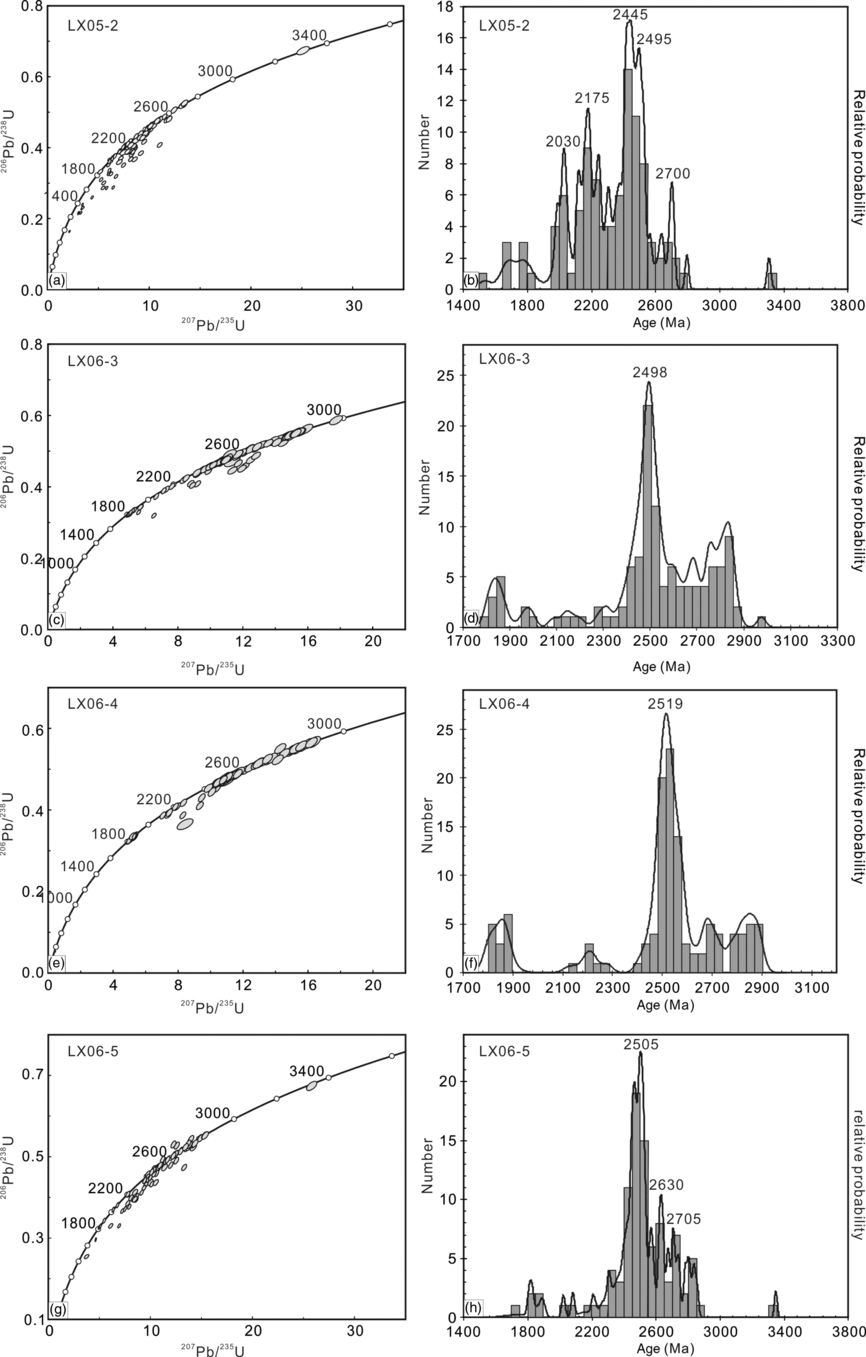
Fig. 5. U–Pb concordia and age distribution diagrams of detrital zircons from quartzite sample (a, b) LX05-2 and leptynite samples (c, d) LX06-3, (e, f) LX06-4 and (g, h) LX06-5 collected from the Wulian Group.
4.a.2. Biotite-bearing leptynite
Three biotite-bearing leptynite samples, LX06-3, LX06-4 and LX06-5, were collected from the Haiyankou Formation to the east of Haiyankou (Fig. 2). The thickness of the biotite-bearing leptynite outcrop is more than 100 m. The grey biotite-bearing leptynite is intruded by a Neoproterozoic granitic vein (Fig. 3c) and shows a fine-grained crystalloblastic texture and banded structure (Fig. 3c–h). These samples have a mineral assemblage of quartz (50–55%), plagioclase + K-feldspar (20–30%) and biotite (15–30%) (Fig. 3d, f, h). Zircon grains from the three leptynite samples are similar to those of quartzite sample LX05-2, exhibit mechanical damage, and comprise dominantly magmatic zircons with oscillatory zoning patterns and minor metamorphic zircons or growth rims characterized by homogeneous or nebulous internal textures (Fig. 4b–d). Totals of 120, 120 and 95 U–Pb analyses were made on the zircons from samples LX06-3, LX06-4 and LX06-5, respectively. Most of the U–Pb analyses are concordant within ± 10% (Fig. 5c, e, g; online Supplementary Table S1); they yield identical apparent 207Pb/206Pb age spectra that display one major population of 2600–2400 Ma with age peaks at 2498, 2519 and 2505 Ma, and several secondary populations of 1900–1800, 2300–2000, 2800–2600 and 2900–2800 Ma (Fig. 5d, f, h). In addition, three metamorphic zircons (spots 2, 6 and 9) from sample LX06-3 have apparent 207Pb/206Pb ages of 2087, 2499 and 2491 Ma with Th/U ratios of 0.33, 1.65 and 0.38, respectively (Fig. 4b; online Supplementary Table S1). Six metamorphic zircons (spots 5, 9, 17, 18, 19 and 28) from sample LX06-4 have apparent 207Pb/206Pb ages of 2514, 1809, 1873, 1800, 1863 and 2500 Ma with high or low Th/U ratios of 1.59, 0.28, < 0.01, 0.19, 0.09 and 0.97, respectively (Fig. 4c; online Supplementary Table S1). Three U–Pb analyses (spots 15, 17 and 18) for metamorphic zircon or overgrowth rim of sample LX06-5 yield three apparent 207Pb/206Pb ages of 2520, 2729 and 2081 Ma with Th/U ratios of 0.61, 0.38 and 0.58, respectively (Fig. 4d; online Supplementary Table S1).
4.a.3. Marble
The marble sample LX03-2 was collected from the Haiyankou Formation to the east of Haiyankou (Fig. 2). The sample is grey-white, shows brittle deformation (Fig. 6a) and comprises calcite (65%) and dolomite (35%) (Fig. 6b). Most of the zircon grains from sample LX03-2 are subhedral to anhedral and show mechanical damage and rounding. Representative CL images of the studied zircons together with spot U–Pb ages are shown in Figure 4e, indicating that sample LX03-2 contains various types of metamorphic and magmatic zircons. The U–Pb analyses performed on 120 zircons from sample LX03-2 obtain apparent 207Pb/206Pb ages varying from 733 to 3408 Ma (online Supplementary Table S1), most of which are concordant within ± 10%, whereas some of them are discordant, likely attributed to Pb loss (Fig. 7a). The concordant apparent 207Pb/206Pb age histogram shows one dominant population of 2600–2400 Ma with a peak age of 2500 Ma and several secondary populations: 814–731 Ma; 1600–1300 Ma with a peak at 1413 Ma; 2000–1800 Ma with a peak at 1935 Ma; 2200–2000 Ma with a peak at 2135 Ma; and 2800–2600 Ma with a peak at 2700 Ma (Fig. 7b). Relative to the quartzite and leptynite samples, the marble sample LX03-2 has greater populations of younger ages, implying the possible addition of new provenances. Twelve U–Pb analyses (spots 1, 4, 10, 24, 31, 32, 36, 62, 70, 95, 106 and 120) for metamorphic zircon or overgrowth rim of sample LX03-2 yield 207Pb/206Pb ages varying from 2700 to 1013 Ma; these can be subdivided into four metamorphic age populations of 2700, 2500–2539, 2036 and 1975–1845 Ma, with variable Th/U ratios ranging from 1.01 to smaller than 0.01 (online Supplementary Table S1; Fig. 4e).

Fig. 6. Photographs of outcrops and photomicrographs of marble samples (a, b) LX03-2 and(c, d) LX07-1, Neoproterozoic (e, f) granite sample LX03-4 and (g, h) meta-diorite sample LX 04-1 from the Wulian Complex. Cal – calcite; Dol – dolomite; Hb – hornblende; Pl – plagioclase; Qtz – quartz.

Fig. 7. U–Pb concordia and age distribution diagrams of zircons from marble samples (a, b) LX03-2 and (c, d) LX07-1 collected from the Wulian Group; Neoproterozoic (e, f) granite sample LX03-4 and (g, h) meta-diorite sample LX 04-1 collected from the Wulian complex.
Sample LX07-1, an impure marble, was collected from the Kunshan Formation to the NE of Zhujialaozhuang (Fig. 2). The impure marble is brown-red with strong compressional deformation, and has been intruded by Neoproterozoic gneissic granite (Fig. 6c). The sample has a mineral assemblage of calcite (75%) and quartz + feldspar (25%) (Fig. 6d). Most of the zircon grains separated from sample LX07-1 are anhedral to subhedral, elongate and prismatic in shape. Some zircons have oscillatory zoning patterns without metamorphic growth rims, whereas other zircons show homogeneous internal textures, typical of zircons crystallized in mafic igneous rocks (Fig. 4f). A total of 39 U–Pb analyses on zircons from sample LX07-1 yield apparent 207Pb/206Pb ages ranging from 2180 to 943 Ma with high Th/U ratios (mostly larger than 0.4) (Fig. 7c; online Supplementary Table S1) and display a distinctive age spectrum comprising a single dominant population of 1300–950 Ma with a peak at 1055 Ma and minor older ages (Fig. 7d).
4.a.4. Gneissic granite
Sample LX03-4 is a mylonitic granite that occurs as folded small veins in the amphibolite of the Haiyankou Formation (Fig. 6e). The sample shows an obviously mylonitic structure. Quartz has been directionally elongated and has undulose extinction, indicating strongly ductile shearing deformation, whereas plagioclase and K-feldspar are fine-grained and/or have clay mineralization (Fig. 6f). Zircon grains from this sample are dominantly euhedral, elongate or prismatic and show clear oscillatory zoning patterns (Fig. 4g). A total of 34 zircon U–Pb analyses give consistent apparent 206Pb/238U ages of 769–752 Ma with high Th/U ratios of 0.67–1.68, and yield a weighted mean age of 761 ± 7 Ma (MSWD, 0.062; n = 34) (Fig. 7e, f; online Supplementary Table S1), which is considered the emplacement age of the granite.
Sample LX04-1, a fine-grained meta-diorite, was collected from eastern Haiyankou (Fig. 2). The rock occurs as an intrusion and is extensively distributed in the study area (Figs 2, 6g). The sample comprises hornblende (75%) and plagioclase (25%) and shows ‘white eye’ texture, which is composed of plagioclase (Fig. 6h). Some zircons have oscillatory zoning patterns with metamorphic growth rims, whereas other zircons show comb zoning patterns or homogeneous internal textures with light, thin overgrowth rims. Representative CL images of the studied zircons together with U–Pb spot ages are shown in Figure 4h. A total of 48 U–Pb analyses were performed on various zircons from sample LX04-1 and yield various ages ranging from 3040 to 561 Ma with Th/U ratios ranging from 0.02 to 1.36 (Fig. 7g, h; online Supplementary Table S1). These are subdivided into two age populations of 806–560 and 1963–1778 Ma with a peak age of 1835 Ma and a few older ages (Fig. 7h). A total of 15 U–Pb analyses from the younger age population yield a weighted mean age of 760 ± 5 Ma (MSWD, 0.78; n = 15) (Fig. 7g), which is consistent with the emplacement age of granite sample LX03-4 within errors and is interpreted as the emplacement age of the sample; one older population and other older ages are considered ages of xenocrystic zircons brought to the surface from the deep crust by diorite.
4.b. Zircon Lu–Hf isotope compositions
In this study, a total of 210 Lu–Hf isotope analyses were conducted on zircons from quartzite sample LX05-2, leptynite sample LX06-5, two marble samples LX03-2 and LX07-1, Neoproterozoic gneissic granite sample LX03-4 and meta-diorite sample LX04-1. The results are presented in online Supplementary Table S2 and Figure 8.

Fig. 8. Results of Lu–Hf analyses of zircons from (a–c) quartzite sample LX05-2, (d–f) leptynite sample LX06-5, marble samples (g–i) LX03-2 and (j–l) LX07-1, gneissic (m–o) granite sample LX03-4 and (p–r) meta-diorite sample LX04-1. The analytical results are shown as plots of (a, d, g, j, m, p) 176Lu/177Hf ratios v. 176Hf/177Hf ratios, (b, e, h, k, n, q) 207Pb/ 206Pb ages v. ϵHf(t) values and(c, f, i, l, o, r) two-stage Hf model ages (T DM2).
A total of 47 zircons from quartzite sample LX05-2 exhibit very low 176Lu/177Hf ratios (generally lower than 0.002) (Fig. 8a), have 176Hf/177Hf ratios of 0.281589–0.280622 (Fig. 8a), ϵHf(t) values varying from −18.28 to +5.44 (Fig. 8b), and two-stage Hf model (T DM(Hf)C) ages of 4022–2419 Ma (Fig. 8c). A total of 40 zircons from leptynite sample LX06-5 have very low 176Lu/177Hf ratios (generally lower than 0.001) (Fig. 8d), 176Hf/177Hf ratios of 0.281515–0.280535 (Fig. 8d), negative and positive ϵHf(t) values varying from −15.42 to +7.23 (Fig. 8e), and T DM(Hf)C ages of 3948–2572 Ma (Fig. 8f). A total of 43 zircons with various U–Pb ages from marble sample LX03-2 show low 176Lu/177Hf ratios (generally lower than 0.001) (Fig. 8g) and have 176Hf/177Hf ratios ranging from 0.281054 to 0.282009 (Fig. 8g), negative and positive ϵHf(t) values varying from −6.57 to +23.35 (Fig. 8h) and T DM(Hf)C ages of 3219–1961 Ma (Fig. 8i). A total of 18 zircons from marble LX07-1 have been subjected to Hf isotope analyses and display low 176Lu/177Hf ratios (generally lower than 0.002) and 176Hf/177Hf ratios of 282516–281533 (Fig. 8j). The zircon population of U–Pb ages clustering at 1055 Ma from sample LX07-1 exhibits ϵHf(t) values ranging from –1.90 to +15.60 (mostly positive values) (Fig. 8k) and T DM(Hf)C ages clustering at 1205 Ma. The other three zircons have ϵHf(t) values of +3.92, +6.81 and –0.83 and T DM(Hf)C ages of 2513, 2268 and 2578 Ma, respectively (Fig. 8l). A total of 30 zircons from the Neoproterozoic gneissic granite sample LX03-4 display identical 176Lu/177Hf ratios (lower than 0.001) and176Hf/177Hf ratios of 281792–281680 (Fig. 8m), negative ϵHf(t) values of −22.32 to −17.61 with an average of −20.50 and old T DM(Hf)C ages of 3055–2792 Ma (Fig. 8n, o). The meta-diorite sample LX04-1 has positive ϵHf(t) values of +16.51 to +3.79 with an average of +10.24, and one-stage depleted-mantle model (T DM) ages of 1188–689 Ma (online Supplementary Table S2), whereas the zircons older than 1800 Ma display negative to positive ϵHf(t) values varying from −5.93 to +10.07 and T DM(Hf)C ages ranging from 3177 to 1849 Ma (Fig. 8p–r; online Supplementary Table S2).
5. Discussion
5.a. Provenance and tectonic affinity of the Wulian Group
The tectonic affinity of the Wulian Group has long been debated. Some people have proposed that the Wulian Group is equivalent to the Palaeoproterozoic Fenzhishan Group in the Jiaobei Terrane, NCC (SBGMR, 1987, 1997; Faure et al. Reference Faure, Lin and Le Breton2001; Zhai, Reference Zhai2002; Zhao et al. Reference Zhao, Fang and Yu2003; Lin et al. Reference Lin, Faure, Monie and Breton2004), and has NCC affinity (SBGMR, 1987, 1997; Zhai, Reference Zhai2002). Others have suggested that the Wulian Group has tectonic affinity to the SCC (Faure et al. Reference Faure, Lin and Le Breton2001, Reference Faure, Lin, Monié, Breton, Poussineau, Panis and Deloule2003; Wu et al. Reference Wu, Zheng and Zhou2004; Zheng et al. Reference Zheng, Zhou, Wu and Xie2005) and have interpreted the Wulian Group as the Sulu segment of the Beihuaiyang unit in the Dabie Terrane (Zhou et al. Reference Zhou, Zheng and Wu2003; Wu et al. Reference Wu, Zheng and Zhou2004; Zheng et al. Reference Zheng, Zhou, Wu and Xie2005). Single U–Pb analyses of detrital zircons from the six samples of the Wulian Group show that one quartzite and three leptynite samples display identical age spectra, remarkably characterized by one dominant age population with a peak at c. 2.5 Ga and several minor age populations (Fig. 5b, d, f, h). The marble sample LX03-2 collected from the lower sequence of marble shows the addition of new younger age compositions (Fig. 7b), suggesting the appearance of new source rocks. In contrast, the marble sample LX07-1 collected from the upper sequence of marble displays a distinctive age spectrum dominated by one age population of 1250–950 Ma with a peak at 1055 Ma (Fig. 7d), indicative of one new dominant provenance. Generally, the detrital zircon age spectrum obtained from the Wulian Group can be subdivided into one predominant age population of 2600–2400 Ma with a peak at c. 2.5 Ga and several secondary age populations of > 3000, 3000–2800, 2800–2600, 2200–2000, 1900–1800, 1500–1300, 1250–950 and 810–660 Ma (Fig. 9a). Most detrital zircons analysed in this study display oscillatory zoning in CL images (Fig. 4), indicative of magmatic origin and provenances with magmatic events. In addition, some zircons with U–Pb ages of c. 2.7, 2.55–2.45, 2.1–2.0 and 1.95–1.8 Ga show homogenous metamorphic overgrowth rims or internal structures (e.g. zircon grains 33, 67, 85 and 90 in Fig. 4a; grains 2, 6 and 9 in Fig. 4b; grains 5, 9, 17, 18, 19 and 28 in Fig. 4c; grains 15, 17 and 18 in Fig. 4d; and grains 1, 4, 10, 31 and 32 in Fig. 4e); some exhibit very low Th/U ratios (< 0.1) (online Supplementary Table S1), indicative of provenances of c. 2.7, 2.5, 2.1–2.0 and 1.95–1.80 Ga metamorphic events.

Fig. 9. Zircon U–Pb age spectra representing (a) the Wulian Group in this study and reported by Reference Zhou, Wilde, Zhao, Zhang, Zheng, Jin and ChengZhou et al. (2008a), where only ages with discordance < 10% have been adopted; (b) the early Precambrian basement of the NCC (Wan et al. Reference Wan, Liu, Dong, Xie, Kröner, Ma, Liu, Xie, Ren and Zhai2015, Reference Wan, Liu, Xie, Dong, Li, Bai and Liu2018); and (c) the Yangtze Plate (Wan et al. Reference Wan, Liu, Xie, Dong, Li, Bai and Liu2018).
Generally, detrital zircon age populations older than c. 1.8 Ga in the Wulian Group can be consistent with the early Precambrian tectono-magmatic thermal events that took place in the NCC, as shown by Figure 9a and b. In particular, the distinctly predominant age population of 2400–2600 Ma with a peak at c. 2.5 Ga corresponds well to the strongest c. 2.5 Ga tectono-magmatic thermal events represented by TTGs, mafic-ultramafic intrusions and synchronous metamorphism in the NCC (Liu et al. 2011, Reference Liu, Liu, Ding, Liu, Yang, Liu, Wang and Meng2013a , 2017; Wan et al. Reference Wan, Liu, Xie, Dong, Li, Bai and Liu2018). However, all tectono-magmatic events and rocks with ages consistent with the detrital zircon age populations of the Wulian Group have also been reported in the SCC (Fig. 9a, c; Hu et al. Reference Hu, Liu, Chen, Qu, Li and Geng2013; Zhang & Zheng, Reference Zhang and Zheng2013; Wan et al. Reference Wan, Liu, Xie, Dong, Li, Bai and Liu2018). The Archaean magmatic zircon ages in the Yangtze Plate exhibit four major peaks at c. 2.5, 2.7, 2.9 and 3.3 Ga (Fig. 9c; Wan et al. Reference Wan, Liu, Xie, Dong, Li, Bai and Liu2018). In addition, U–Pb ages of detrital zircons from the sedimentary and xenocrystic zircons brought to the surface in the SCC also display a major peak at c. 2.5 Ga (Zheng et al. Reference Zheng, Griffin, O’Reilly, Zhang, Pearson and Pan2006, Reference Zheng, Griffin, Li, O’Reilly, Pearson, Tang, Liu, Zhao, Yu and Su2011; Yu et al. Reference Yu, O’Reilly, Wang, Griffin, Zhou, Zhang and Shu2010; Hu et al. Reference Hu, Liu, Chen, Qu, Li and Geng2013; Zhang & Zheng, Reference Zhang and Zheng2013). It seems clear that both the NCC and the SCC can be candidates for provenance of the Wulian Group based on the similarity of their early Precambrian zircon ages.
However, it is worth noting that the Wulian Group contains detrital zircons of the middle Neoproterozoic age population (Figs 7b, 9a) and was intruded by Neoproterozoic gneissic granite and meta-diorite with emplacement ages of c. 0.76 Ga (Fig. 6c, e). Such Neoproterozoic syn-rifting bimodal magmatic activity during 0.9–0.7 Ga remarkably occurred in the SCC (Li et al. Reference Li, Li, Kinny, Wang, Zhang and Zhou2003), whereas it is rare in the NCC and has therefore been considered an important signature in distinguishing the SCC from the NCC (Zheng et al. Reference Zheng, Zhou, Wu and Xie2005). Moreover, previous investigations of geochronology, geochemistry and oxygen isotopes of the Neoproterozoic granites that occur in the Sulu orogenic belt (or on the northern margin of the Yangtze plate) have demonstrated that the Neoproterozoic granite has tectonic affinity to the SCC (Wu et al. Reference Wu, Zheng and Zhou2004; Zheng et al. Reference Zheng, Zhou, Wu and Xie2005; Tang et al. Reference Tang, Zheng, Wu, Gong, Zha and Liu2008). Furthermore, metamorphic events corresponding to metamorphic ages of c. 2.7, 2.55–2.45, 2.1–2.0 and 1.95–1.80 Ga have been reported in the SCC (Qiu et al. Reference Qiu, Gao, McNaughton, Groves and Ling2000; Zhang et al. Reference Zhang, Zheng, Wu, Zhao, Gao and Wu2006b ; Sun et al. Reference Sun, Cheng, Zhao, Wilde, Ye, Guo, Chen and Yuan2008; Wu et al. Reference Wu, Zheng, Gao, Jiao and Liu2008, Reference Wu, Gao, Gong, Xiang, Jiao, Yang, Liu and Yuan2009; Jiao et al. Reference Jiao, Wu, Yang, Peng and Wang2009; Gao et al. Reference Gao, Yang, Zhou, Li, Hu, Guo, Yuan, Gong, Xiao and Wei2011; Yin et al. Reference Yin, Lin, Davis, Zhao, Xiao, Li and He2013; Wan et al. Reference Wan, Liu, Xie, Dong, Li, Bai and Liu2018), whereas c. 2.7 and 2.1–2.0 Ga metamorphic events have never been reported in the NCC. In addition, SCC-type Neoproterozoic microfossils have been identified in the quartzite and marble of the Wulian Group (Zhao et al. Reference Zhao, Cheng and Liu1995). We therefore propose that the SCC should be the major provenance of the Wulian Group.
The Wulian Group has been subjected to strong deformation. Its initial sedimentary sequences have been seriously changed by folding and faulting processes (Fig. 6a, c, e). The Neoproterozoic granite is characterized by the occurrence of mylonitic structure. Different strata usually show tectonic unconformities or fault contacts with each other. Accordingly, the Wulian complex has been interpreted as a tectonic wedge (or accretionary wedge) that formed during subduction and collision of the SCC towards the NCC during the Triassic Period (Zheng et al. Reference Zheng, Zhou, Wu and Xie2005; Zhou et al. Reference Zhou, Wilde, Zhao, Zhang, Zheng, Jin and Cheng2008a ). The marble sample LX07-1 displays zircon age components different from those in quartzite sample LX05-2, leptynite samples LX06-3, LX06-4 and LX06-5, and marble sample LX03-2. Sample LX07-1 only has a single dominant age population with a peak at 1055 Ma, whereas the other samples show multiple age populations, particularly early Precambrian ages (Figs 5b, d, f, h, 7b, d), suggesting that they were sourced from different source rocks and deposited in different tectonic environment at different times. These results further demonstrate that the Wulian complex might be a tectonic accretionary wedge consisting of different strata and rocks that underwent deformation, metamorphism and displacement caused by subduction and collision of the SCC and NCC during the Triassic Period.
5.b. Constraints on early Precambrian SCC crustal growth, reworking and evolution
Detrital zircon U–Pb ages coupled with Hf isotope data can be used to provide unique insights into crustal growth and recycling in the provenance, given that zircon U–Pb and Hf model ages represent the timing of magmatic events involved in crustal melting and the separation of juvenile crust from the mantle, respectively (Kinny & Maas, Reference Kinny and Maas2003; Wu et al. Reference Wu, Li, Zheng and Gao2007; Zheng et al. Reference Zheng, Zhang, Zhao, Wu, Li, Li and Wu2007). In general, negative ϵHf(t) values of zircons imply a derivation by re-melting ancient crustal material, whereas positive ϵHf(t) values suggest an origin by re-melting juvenile crust. The detrital zircon U–Pb ages of the Wulian Group display multiple age populations of > 3000, 3000–2800, 2800–2600, 2600–2400, 2200–2000, 1900–1800, 1500–1300, 1250–950 and 818–660 Ma, suggesting multiple magmatic events. In addition, some metamorphic zircons and/or growth rims yield ages of c. 2.7, 2.5–2.45, 2.1–2.0 and 1.9–1.8 Ga, indicating multiple metamorphic events in the SCC. Hf isotope analyses of detrital zircons from the Wulian Group exhibit both positive and negative ϵHf(t) values (Fig. 10a), indicating that both ancient and juvenile crusts were involved in recycling. The T DM(Hf)C ages display several populations of 1500–900 Ma with a peak at 1150, 2100–1700 and 3200–2300 Ma, with one dominant peak at 2975 Ma and several secondary peaks at 2740, 2515 and 2315 Ma, and > 3200 Ma with a peak at 3270 Ma, suggesting multiple episodes of crustal growth. In particular, major crustal growth took place during c. 3.4–2.5 Ga with a dominant peak at c. 2.96 Ga (Fig. 10b).

Fig. 10. Comprehensive zircon ages v. (a) ϵHf(t) values and (b) two-stage Hf model ages (T DM2) of samples from the Wulian complex. CHUR – chondrite.
Two zircons with U–Pb ages of 3307 and 3347 Ma have negative ϵHf(t) values of −2.49 and −4.91 and two-stage T DM(Hf)C ages of 3772 and 3948 Ma, respectively (Fig. 10a; online Supplementary Table S2), indicating that the source rocks of the zircons were derived from reworking of ancient Precambrian crust and that juvenile crust was extracted from the mantle during the Eoarchaean Era. The 3.45–3.2 Ga rocks (mostly TTG gneisses) have been discovered in the Kongling area of the Yangtze plate and mostly exhibit negative ϵHf(t) values and Palaeo-Eoarchaean T DM(Hf)C ages (Reference Zhang, Zheng, Wu, Zhao, Gao and WuZhang et al. 2006a ; Jiao et al. Reference Jiao, Wu, Yang, Peng and Wang2009; Wei et al. Reference Wei, Wang, Wang, Shan and Guo2009; Gao et al. Reference Gao, Yang, Zhou, Li, Hu, Guo, Yuan, Gong, Xiao and Wei2011; Guo et al. Reference Guo, Gao, Wu, Li, Chen, Hu, Liang, Liu, Zhou, Zong, Zhang and Chen2014, Reference Guo, Wu, Gao, Jin, Zong, Hu, Chen, Chen and Liu2015). In addition, many xenocrystic zircons and detrital zircons with Palaeoarchaean ages have been reported in the SCC (Qiu et al. Reference Qiu, Gao, McNaughton, Groves and Ling2000; Reference Zhang, Zheng, Wu, Zhao, Gao and WuZhang et al. 2006a ; Zheng et al. Reference Zheng, Griffin, O’Reilly, Zhang, Pearson and Pan2006, Reference Zheng, Griffin, Li, O’Reilly, Pearson, Tang, Liu, Zhao, Yu and Su2011; Gao et al. Reference Gao, Yang, Zhou, Li, Hu, Guo, Yuan, Gong, Xiao and Wei2011; Guo et al. Reference Guo, Wu, Gao, Jin, Zong, Hu, Chen, Chen and Liu2015; Wan et al. Reference Wan, Liu, Xie, Dong, Li, Bai and Liu2018). These data confirm that crustal growth and reworking of ancient continental crust had taken place earlier than the Palaeoarchaean Era in the SCC.
The detrital zircon ages of 3000–2400 Ma display multiple age peaks at 2500, 2700 and 2840 Ma (Fig. 9a), corresponding to multiple crustal reworking events mainly represented by TTG and granitic magmatism, which have been reported in the Douling, Kongling, Yudongzi, Zhongxiang and Dabie areas in the Yangtze plate (Reference Zhang, Zhang, Tang and WangZhang et al. 2001b , 2006a, 2010; Wu et al. Reference Wu, Gao, Gong, Xiang, Jiao, Yang, Liu and Yuan2009, Reference Wu, Zhou, Gao, Liu, Qin, Wang, Yang and Yang2014; Gao et al. Reference Gao, Yang, Zhou, Li, Hu, Guo, Yuan, Gong, Xiao and Wei2011; Wei & Wang, Reference Wei and Wang2012; Chen et al. Reference Chen, Gao, Wu, Guo, Hu, Liu, Zong, Liang and Geng2013; Reference Wang, Wang, Du, Deng and YangWang et al. 2013a , b, 2018; Guo et al. Reference Guo, Wu, Gao, Jin, Zong, Hu, Chen, Chen and Liu2015; Hui et al. Reference Hui, Dong, Cheng, Long, Liu, Yang, Sun, Zhang and Varga2017; Wan et al. Reference Wan, Liu, Xie, Dong, Li, Bai and Liu2018). These zircons exhibit both positive and negative ϵHf(t) values, indicating multiple episodes of reworking of both ancient and juvenile crustal rocks during 3000–2400 Ma.
The detrital zircon ages of 2300–1800 Ma with three age peaks at 1850, 2100 and 2160 Ma exhibit both positive and negative ϵHf(t) values and various T DM(Hf)C ages of 3.9–1.9 Ga, recording multiple episodes of reworking of ancient Archaean crust and Palaeoproterozoic juvenile crust, which is consistent with crustal reworking and growth recorded by zircons of the Palaeoproterozoic rocks discovered in the SCC (Wang et al. Reference Wang, Chen and Luo2008; Xiang et al. Reference Xiang, Zhang, Zhou, Zhong, Zeng, Liu and Jin2008; Liu et al. Reference Liu, Zhou, Zhang, Zhong, Zeng, Xiang, Jin, Lu and Li2009; Peng et al. Reference Peng, Wu, Wang, Jiao, Liu and Yang2009; Xiong et al. Reference Xiong, Zheng, Yu, Su, Tang and Zhang2009; Yu et al. Reference Yu, O’Reilly, Zhou, Griffin and Wang2012; Yin et al. Reference Yin, Lin, Davis, Zhao, Xiao, Li and He2013; Guo et al. Reference Guo, Wu, Gao, Jin, Zong, Hu, Chen, Chen and Liu2015; Qiu et al. Reference Qiu, Yang, Lu, Tan and Cai2015).
Several zircons with ages of 1540–1400 Ma exhibit ϵHf(t) values varying from +14.76 to −5.38 (two of which are close to the depleted mantle value), indicating one episode of reworking of the Palaeoproterozoic crust and contemporaneous juvenile crust. The detrital zircon age population of 1250–940 Ma with an age peak at 1055 Ma is the dominant age population of marble sample LX07-1 (Fig. 7d). As shown in the CL images of the zircons (Fig. 4f), most detrital zircons are mainly sourced from mafic igneous rocks, whereas minor detrital zircons are sourced from felsic igneous rocks. The former rocks exhibit positive zircon ϵHf(t) values and younger T DM(Hf)C ages (Figs 8k, l, 10a; online Supplementary Table S2), suggesting that mafic igneous rocks were extracted from the depleted mantle. In contrast, the latter rocks have negative or close to chondritic zircon ϵHf(t) values and older T DM(Hf)C ages (Figs 8k, l, 10a; online Supplementary Table S2), recording the reworking of the ancient continental crust. Greentree et al. (Reference Greentree, Li, Li and Wu2006) also reported a zircon U–Pb age of 1142 ± 16 Ma for a tuff layer from Laowushan. In addition, Zhang et al. (Reference Zhang, Gao, Wu, Shi, Yan and Li2007) obtained a zircon U–Pb age of 1032 ± 9 Ma from a tuff of the Kunyang Group. Yang et al. (Reference Yang, Geng, Du, Ren, Wang, Zhou and Yang2009) reported a zircon U–Pb age of 1014 ± 8 Ma for gneissic granite on the western margin of the Yangtze plate. Recently, increasing numbers of c. 1.0 Ga mafic dykes, dacites, granites and volcanic rocks have been discovered in the southwestern Yangtze plate (Yang et al. Reference Yang, Geng, Du, Ren, Wang, Zhou and Yang2009; Chen et al. Reference Chen, Sun, Wang, Zhao and Zhou2014, Reference Chen, Sun, Zhou and Wang2018; Zhu et al. Reference Zhu, Zhong, Li, Bai and Yang2016). Generally, the late Mesoproterozoic – early Neoproterozoic rocks that have been discovered in the SCC are very limited, and might represent bimodal magmatism linked with an extensional setting.
The middle Neoproterozoic gneissic granite and meta-diorite in the Wulian complex have identical emplacement ages of c. 0.76 Ga, representing middle Neoproterozoic syn-rifting bimodal magmatic activity in the Sulu orogenic belt. The middle Neoproterozoic gneissic granite has negative ϵHf(t) values with an average of −20.50 and old T DM(Hf)C ages of 3055–2792 Ma (Fig. 8n, o; online Supplementary Table S2), suggesting that it was derived from partial melting of ancient Archean continental crust. The meta-diorite displays positive ϵHf(t) values with an average of +10.24 and one-stage depleted-mantle model (T DM) ages of 1188–689 Ma (Fig. 8q, r; online Supplementary Table S2), indicating that the meta-diorite originated from the depleted mantle. Furthermore, such bimodal magmatic activity occurred extensively on the northern margin of the Yangtze plate as well as the whole SCC (Zheng et al. Reference Zheng, Zhang, Zhao, Wu, Li, Li and Wu2007, Reference Zheng2008) and has been considered to be linked with continental rifting triggered by mantle plume (Li et al. Reference Li, Li, Kinny, Wang, Zhang and Zhou2003).
6. Conclusions
-
(1) The Wulian Group has tectonic affinity to the SCC and was mainly sourced from early Precambrian basement rocks, SCC rocks of age c. 2.5 Ga in particular.
-
(2) The detrital zircon U–Pb ages of the Wulian Group display multiple age populations of > 3000, 3000–2800, 2800–2600, 2600–2400, 2200–2000, 1900–1800, 1500–1300, 1250–950 and 818–660 Ma, suggesting multiple episodes of magmatic activity. In addition, some metamorphic zircons and/or growth rims record multiple metamorphic events at c. 2.7, 2.55–2.45, 2.1–2.0 and 1.95–1.80 Ga in the SCC.
-
(3) The detrital zircons have both positive and negative ϵHf(t) values, indicating that the source rocks were derived from multiple reworking of both ancient and juvenile crustal rocks. The major crustal reworking took place at c. 2.5 Ga. The major crustal growth took place during c. 3.4–2.5 Ga with a dominant peak at 2.96 Ga and several secondary peaks at 3.27, 2.74 and 2.52 Ga.
-
(4) Hf isotope data for the two oldest zircons with ages of 3307 and 3347 Ma imply the recycling of ancient continental crust (> 3.35 Ga) and crustal growth prior to c. 3.95 Ga in the SCC.
-
(5) The gneissic granite and meta-diorite have identical emplacement ages of c. 0.76 Ga; the former rock was derived from remelting of ancient continental crust, whereas the latter rock originated from the depleted mantle, confirming syn-rifting bimodal magmatic activity on the northern margin of the Yangtze Plate during middle Neoproterozoic time.
Acknowledgements
We thank the editor Kathryn Goodenough and two referees for their constructive comments. This work was funded by the National Natural Science Foundations of China (grant nos 41430210, 41772195 and 41973048), the Basic Scientific Foundation of CAGS (grant no. YYWF201703), the Natural Science Foundation of Shandong Province (grant no. ZR2016DM01), the Geological Survey Fund of the China Geological Survey (grant no. DD20190358) and the Basic Scientific Research Foundations of the Institute of Geology, CAGS (J1214 and J1404).
Supplementary material
To view supplementary material for this article, please visit https://doi.org/10.1017/S0016756820000485