INTRODUCTION
In Denmark, helminth infections are more prevalent in organic pig production systems compared to the intensive indoor herds (Roepstorff et al. 1992; Carstensen et al. 2002). The management practices of the Danish organic production systems are very diverse, but piglets are generally born on pasture. Weaning must not take place before the age of 7 weeks and the weaned pigs may then remain outdoors for fattening or be transferred to indoor pens (usually with deep litter) and access to an outdoor run (Danish Plant Directorate, 2005). These practices generally provide better conditions, not only for the development and survival, but also for the accumulation of the free-living parasite stages, thereby increasing the potential for transmission compared to the slatted floors and high levels of hygiene of the intensive indoor herds (reviewed by Roepstorff and Nansen, 1994).
Although Ascaris suum is the most common helminth in Danish organic pigs, Trichuris suis and especially Oesophagostomum dentatum may also be present (Roepstorff et al. 1992; Carstensen et al. 2002). Trichuris suis and O. dentatum occupy overlapping habitats in the caecum and large intestine of the pig but vary considerably in infection biology. Within 23 to 25 days after deposition on pasture O. dentatum eggs may develop into infective free-living L3-larvae (Mejer et al. 2000) that are considered to be susceptible to adverse climatic conditions. The pre-patent period may be as short as 17–19 days (Talvik et al. 1997). The worms have a low immunogenicity and may accumulate in the host over time. The parasite is therefore most prevalent in older animals like sows (Roepstorff and Nansen, 1994). Oesophagostomum spp. may reduce growth and feed utilization (Pattison et al. 1980; Hale et al. 1981) but is generally considered to be only mildly pathogenic in pigs (Stewart and Gasbarre, 1989). In contrast, T. suis is transmitted as resistant eggs that may require more than 1 year to reach infectivity on pasture (Burden and Hammet, 1976, 1979). The pre-patent period is approximately 41–45 days (Powers et al. 1960) and pigs may acquire a strong protective immunity, resulting in the elimination of the worms in some host animals (Pedersen and Saeed, 2001). Trichuris suis infections may negatively affect feed conversion and growth of the host (Hale and Stewart, 1979) but have also been known to cause mortality among heavily exposed young pigs (Powers et al. 1960; Jensen and Svensmark, 1996).
Much information has been obtained on helminth distribution within herds (Roepstorff et al. 1992; Carstensen et al. 2002) and transmission patterns in outdoor pigs of varying age (Andrews et al. 1970; Dangolla et al. 1994; Roepstorff and Murrell, 1997a,b; Mejer et al. 2000). Still, only few studies have looked into the parasite population biology in pigs, which have been exposed to infection within their first days of life. Knowledge gained within this area may therefore be important in future helminth control strategies. The present study was designed to study population dynamics and epidemiology of swine helminths in outdoor organic production systems by providing a qualitative and quantitative description of O. dentatum and T. suis infections in pigs that are born and raised on parasite contaminated paddocks. Ascaris suum was also included in the study but the data are reported elsewhere (Mejer and Roepstorff, 2006).
MATERIALS AND METHODS
Experimental design
Six experimental farrowing paddocks were contaminated with O. dentatum and T. suis eggs from late spring to early summer 2001 by experimentally infected seeder pigs (Fig. 1). Early July, 1 sow farrowed on each paddock and this was designated week 0 post- partum (p.p.) and all other events related to this point in time. The sows were removed at weaning and the offspring (experimental pigs) slaughtered serially at weeks 3 to 19 p.p. Paddock contamination levels of helminth eggs/larvae were measured regularly by analysis of soil and vegetation samples and by means of short-term exposed parasite naïve tracer pigs in late June (tracer I) and late November (tracer II). All animals were treated in accordance with the requirements of the Danish Animal Experimentation Directorate.

Fig. 1. Six farrowing paddocks were contaminated with Oesophagostomum dentatum and Trichuris suis by seeder pigs in order to study the infection patterns in serially slaughtered (S) experimental pigs born and raised on the paddocks. Tracer pigs (I+II) were turned out twice to estimate paddock infectivity and paddock samples (PS) collected at regular intervals.
Experimental paddocks
Six identical 600 m2 (10×60 m) farrowing paddocks with second year clover grass were established in 2001. One end of each paddock was defined as the ‘area around the hut’ (10×10 m) and contained a farrowing hut (1·4 m2), a wallowing area, a water trough, a feed trough, and a smaller feed trough that was only accessible to piglets until weaning.
Seeder pigs and parasites
Three litters of 32 Landrace/Yorkshire/Duroc pigs were born late February and raised on helminth-free paddocks. The pigs were trickle inoculated from the age of 2 to 14 weeks with increasing weekly doses, corresponding to 25 O. dentatum L3-larvae and 5 T. suis eggs/kg body weight/day. In total, each pig was given approx. 52500 O. dentatum larvae and 10500 T. suis eggs. For parasite strains see Roepstorff et al. (1987) and Roepstorff and Murrell (1997b). Inoculation doses were given orally using disposable pipettes until weaning at week 7 p.p. after which the doses were mixed in the feed. Eight weeks into the infection the pigs were nose-ringed and each litter (n=9–13 pigs) was moved to an experimental paddock (i.e. week −9·5 p.p. of experimental pigs, Fig. 1). The paddocks were then contaminated by weekly rotation of the litters. Faecal samples were collected at every rotational move and the pigs were weighed every second week. During this period the pigs were fed a diet of 75% ground barley and 25% protein-mineral supplement.
Sows and experimental pigs
At approximately week −9 p.p., 6 gilts were nose-ringed and turned out on a pre-farrowing paddock lightly contaminated with T. suis. At week −1·5 p.p. (late June, see Fig. 1), 1 sow was moved to each farrowing paddock. The first week of life, all male piglets were castrated and the piglets (Landrace/Yorkshire/Duroc or Landrace/Yorkshire) remained in the farrowing hut. The second week, the piglets had access to a 1·2 m2 outdoor run and at 2 weeks p.p. they were given access to the entire paddock which the sows had access to at all time. From weeks 2 to 7 p.p. the piglets had ad libitum access to the sow feed and a commercial feed supplement for piglets. Weaning took place at 7 weeks p.p. (late August) by removing the sows. To reduce weaning diarrhoea the high protein piglet supplement was substituted 2 days before weaning with a diet consisting of almost pure ground barley for 2 weeks, whereafter the protein-mineral content was increased to 25% over a period of 3 days. The sows were fed a diet of 75% ground barley and 25% sow protein-mineral supplement. After random stratification according to sex and birth weight, 1 experimental pig/litter was slaughtered at weeks 3, 5, 7, 9, 11, 13, 15, and 19 p.p. or until no pigs were left. The experimental pigs were weighed day 3 p.p. and every fortnight starting week 3 p.p. Faeces were collected every fortnight from week 5 p.p. Faecal samples were collected from the sows at week −9 p.p., week −2 p.p., day 3 p.p., and weeks 3, 5, and 7 p.p.
Faecal egg counts and acid-insoluble ash content
Faecal samples from experimental pigs, sows, tracers, and seeder pigs were examined for parasite eggs using a concentration McMaster method with a flotation fluid of saturated NaCl and 500 g glucose/l (specific gravity: 1·27 g/ml) (Roepstorff and Nansen, 1998). As an indicator of geophagy, the content of inorganic matter was determined in feed samples (piglets and fatteners), 1 soil sample/paddock (pooled from all collected samples) and individual faecal samples (weeks 3, 7, 11, 15, and 19 p.p.) from the experimental pigs. The inorganic matter was determined as the percentage of acid-insoluble ash (AIA) in faecal dry matter (Anon., 1971). For comparison, a feed sample (weaned pigs/fatteners) and 5 faecal samples were collected from different age groups of indoor pigs (3, 7, 11, 15, and 19 weeks old).
Paddock contamination index
Based on the egg counts of the seeder pigs a relative contamination index (CI) was calculated for each helminth species and farrowing paddock. Each paddock was contaminated for 6–7 days over 3 periods. Individual epgs obtained at the start and the end of each contamination period, were used to calculate the CI by the following formula:

The CI is based on the summations of the mean epg of the individual pigs over (a) all 3 contamination periods, (b) all pigs in the litters, and (c) all days in the contamination period. The CI can be compared between paddocks when grazed by groups of pigs of approximately the same body weight. As the daily faecal volume per pig was not known the CI-value is not a measure of the total egg output on a paddock. However, by multiplying the CI of period 1, 2, and 3 with assumed faecal outputs of 400, 660, and 800 g wet faeces/day/pig (estimated according to body weight; A. Chwalibog, personal communication, 2004), respectively, the total egg output was estimated. Similar calculations were made for the experimental pigs while only the CI was calculated for the sows as faecal output was more difficult to estimate.
Paddock infectivity
Paddock samples
Samples were collected at weeks −2 (23 June), 2 (19 July), 8 (30 August), 14 (11 October), and 20 p.p. (24 November) (Fig. 1) from the ‘area around the hut’ and ‘the rest of the paddock’. From both areas 2 soil samples, consisting of 20 subsamples, were collected by walking along 2 W-routes (Roepstorff and Nansen, 1998). The soil was sampled from the uppermost 3 cm, avoiding most plants and obvious faecal deposits. Two vegetation samples were collected from the entire paddock by cutting the grass directly above the soil surface (Roepstorff and Nansen, 1998). Each soil sample was mixed thoroughly. A 50 g subsample was then examined for O. dentatum L3 using a Baermann method (Roepstorff and Nansen, 1998) and a 5 g subsample was examined for T. suis eggs using a flotation and sieving technique (Larsen and Roepstorff, 1999). All T. suis eggs were differentiated according to the protocol of Beer (1973) and categorized as infective (containing slender larvae) or non-infective (non-embryonated to partially embryonated). Oesophagostomum dentatum L3 were isolated from the entire vegetation samples using an agar-gel method (Jørgensen, 1975) and differentiated to genus according to Alicata (1935). For each sampling, 1 soil sample (5 g) per paddock was dried at 105 °C for 24 h and the whole vegetation sample was dried for 3 weeks at approximately 25 °C to determine dry matter content.
Tracer pigs
Helminth naïve tracer pigs (Landrace/Yorkshire/Duroc) were exposed to the pastures late June (n=12, tracer IA) and late November (n=24, tracers IIA and IIB) (Fig. 1). On each occasion, approximately 10-week-old indoor pigs were first turned out on a helminth-free paddock for 2 weeks adaptation. Two (tracer I) or 4 pigs (tracer II) were then allocated to each farrowing paddock according to litter, body weight and sex (females and castrated males). Two pigs were exposed for 4 days before they were moved to parasite-free indoor pens for 10 days before they were slaughtered for O. dentatum recovery (tracers IA and IIA). The remaining pigs in the autumn were exposed to the paddocks for a total of 8 days (tracer IIB) before they were moved indoors for 37 days before being slaughtered for O. dentatum and T. suis recovery. Faecal samples were collected at turn-out on the experimental paddocks and at slaughter. Feeding was as described for the seeder pigs.
Procedures at slaughter
All pigs were euthanized using a captive bolt pistol followed by exsanguination. Oesophagostomum dentatum was recovered from the experimental pigs by processing a subsample of the large intestinal contents according to the agar-gel technique described by Slotved et al. (1996). The size of the subsamples varied from 70% (week 3 p.p.) and 50% (weeks 5 and 9 p.p.) to 10% (weeks 11–19 p.p.). All worms were preserved in 70% ethanol and the developmental stage of O. dentatum later differentiated according to the method of Goodey (1924). A second subsample (week 7 p.p.: 50%; weeks 11–19 p.p.: 10%) was rinsed thoroughly with water on a 212 μm sieve weeks 7, 11, 15, and 19 p.p. for recovery of T. suis. The sieved matter was preserved in iodine (80 g iodine and 400 g potassium iodine in 800 ml distilled water) and decolorized with 30% sodium thiosulphate before counting. The above procedure was also followed for the tracer pigs except that subsample size was 10% for O. dentatum and 20% for T. suis.
Climate data
Data for temperature and precipitation were registered by The Danish Meteorological Institute approx. 8 km from the research area. Mean values from 1982–2000 (temperature) and 1961–1990 (precipitation) were also provided.
Statistical analysis
For subsequent weeks of necropsy, O. dentatum burdens were analysed for differences in medians using the Mann Whitney U-test to identify when significant changes took place; however, results are presented as arithmetic means with min–max in the table. AIA-values for the experimental pigs were analysed similarly. Calculations were carried out using GraphPad Prism version 3 and a significance level of 5%.
RESULTS
Climate data
May was slightly warmer (mean: 12·5 °C) and dryer (total rainfall: 31 mm) than average (data not shown). The paddocks were irrigated at week −1 p.p. (Jun: 13·9 °C, 46 mm), week 0 p.p. (Jul: 17·9 °C, 35 mm) and week 5 p.p. (Aug: 16·9 °C, 117 mm). Though the mean weekly temperature dropped below 14 °C from week 9 p.p. (Sep: 12·2 °C, 143 mm) it was exceptionally warm from week 13 p.p. (Oct: 11·7 °C, 36 mm; Nov: 5·0 °C, 56 mm).
Paddock vegetation
Initially the vegetation was 25–35 cm high and dense. Though the nose-ringed seeder pigs reduced this especially around the hut and feeding area, the vegetation cover remained intact until the turn out of the tracer I pigs. The tracer pigs rooted and grazed intensively but did not seriously damage the vegetation due to the short period of exposure, the low stocking rate and a substantial re-growth in late June to July. As the nose-ringed sows only grazed moderately, their biggest impact was to further wear away the vegetation and compact the soil around the hut. When given access to the outdoor run and later to the whole paddock the experimental pigs explored the paddock surface, especially along the softer edges of the wallowing area. The experimental pigs gradually became more active and at 2 weeks after weaning they started rooting more deeply. At the turn-out of the tracer II pigs, the vegetation cover varied from 0% to 80%.
Pig performance
No clinical helminth infections were observed in the seeder pigs, sows, experimental pigs or tracer pigs. Half the litters of experimental pigs were born on 6 July and the other half on 8 July. On day 3 p.p. the mean number of living offspring was 10 piglets/litter. Five pigs were treated with Penovet® (Boehringer Ingelheim) against Erysipelothrix rhusiopathiae at week 3 p.p. Nevertheless, 4 of the treated and 6 untreated pigs were euthanized or died between weeks 3 and 11 p.p. In 1 litter no pigs were left after week 11 p.p. whereas 1 extra pig was slaughtered from a second litter at week 13 p.p. due to very early onset of disease. The piglets started to eat from both piglet and sow troughs at the age of 2–3 weeks. Hardly any weaning diarrhoea was observed. The mean body weights (min–max) at day 3, week 7 and week 19 p.p. were 2 kg (1–3), 20 kg (15–24), and 85 kg (75–101), respectively.
Oesophagostomum dentatum
The first low parasite egg excretion by the seeder pigs was not noted until approximately 8 weeks into the infection corresponding to week −9·5 p.p. of the experimental pigs, but it increased considerably until week −3·5 p.p. when seeding was terminated (Fig. 2). The CI-values for the seeders varied from 2×105 to 4×105 resulting in a mean estimated excretion of 220 million eggs/paddock. The sows excreted varying levels of O. dentatum eggs (Fig. 2) resulting in CI-levels from 2×103 to 32×103. Recovery of O. dentatum larvae from soil and grass varied much between samples and sampling occasions for any given paddock but overall it peaked at week 2 p.p. and then decreased until week 20 p.p., when larvae were no longer detected (Fig. 3A and B). Soil generally yielded more larvae/kg dry matter than vegetation. Intestinal worms were only recovered from 25% of the tracer IA pigs and tracer IIA pigs whereas only 17% of the tracer IIB harboured worms. The mean worm burden was 3 worms/pig on each occasion. The first experimental pigs (27%) began to excrete O. dentatum eggs at week 7 p.p. After week 13 p.p. no more pigs (85%) became egg positive though the excretion levels increased the following weeks (Fig. 2). A mean of 80 million eggs/paddock (CI: 0 to 6×103) was estimated to have been excreted by the experimental pigs. Although the first O. dentatum worm was recovered at week 3 p.p. it was not until week 7 p.p. that worms were detected in a larger proportion of animals (Table 1). Thereafter, the number of recovered worms increased with time resulting in a slow accumulation of O. dentatum in the pigs. The only significant changes in worm burdens took place from weeks 7 to 9 (P=0·041) and from week 11 to week 13 (P=0·041) for the adult populations. Some of the pigs not excreting detectable numbers of O. dentatum eggs harboured a few predominantly immature and male worms. The overall infection levels of the experimental litters seemed better to reflect the relative sow CI-levels than the seeder CI-levels.

Fig. 2. Mean (±S.E.) number of Oesophagostomum dentatum eggs/g faeces (epg) in seeder pigs (n=32), sows (n=6) and their litters of experimental pigs born (week 0) and raised on 6 paddocks contaminated by the seeders from weeks −9·5 to −3·5. The number of experimental pigs decreased from 50 (week 5) to 9 (week 19) as pigs were slaughtered weeks 3, 5, 7, 9, 11, 13, 15, and 19. At weaning week 7 the sow was removed.
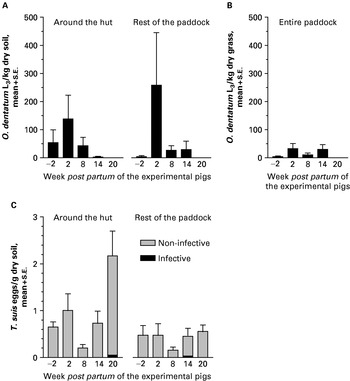
Fig. 3. Mean (+S.E.) number of Oesophagostomum dentatum L3 in soil (A) and grass (B) and infective and non-infective Trichuris suis eggs in soil (C) from different areas of 6 paddocks contaminated by seeder weeks −9·5 to −3·5. A litter of experimental pigs was born at week 0 (early July 2001) on each paddock. Two replicate samples were collected from each paddock area from 23 June (week −2) to 24 November (week 20).
Table 1. Mean Oesophagostomum dentatum and Trichuris suis intestinal worm counts (min-max) and prevalence at slaughter in 6 litters of experimental pigs born early July and raised on 6 contaminated farrowing paddocks (The data were collected through serial slaughter (1 pig/litter/day of necropsy, 27 July to 15 November). Weaning took place when the sow was removed at week 7 post-partum (p.p.).)

Trichuris suis
The seeder pigs excreted low levels of T. suis eggs reaching a maximum of 388 eggs per g faeces (epg)/pig at 9 weeks into the infection (i.e. −8·5 p.p. of the experimental pigs). This transformed into CI-values of 3×104 to 9×104 indicating that an estimated 27 million T. suis eggs/paddock may have been deposited by the seeder pigs. Four sows excreted low levels of eggs (CI: 0 to 4×104) during the latter part of lactation. Very few T. suis eggs were recovered from the soil throughout the experiment and seemingly infective eggs were first noted at week 14 p.p. (Fig. 3C). The low number of infective eggs was reflected by the T. suis worm burdens of the tracer IIB pigs as the prevalence was only 25% and the mean worm burden 4 worms/pig. Only 3 experimental pigs achieved patent T. suis infections (CI: 0 to 6×103) corresponding to a mean excretion of 1 million eggs/paddock. At week 11 p.p. the first positive faecal sample was detected (340 epg). This was the highest recorded epg for any experimental pig, corresponding to very low worm burdens. The prevalence of infected animals increased over time but never exceeded 83% (Table 1). The overall infection level of the litters did not reflect either sow or seeder CI.
AIA
The paddock soil contained 91–92% AIA. The indoor feed for weaners/fatteners contained 0·7% AIA, while feed for the experimental piglets and fatteners contained 0·6% and 1·0%, respectively. For the indoor pigs the faecal content of AIA was very low and showed very little variation with age (Fig. 4). In contrast, the AIA of the outdoor experimental pigs varied between pigs of the same age and gradually decreased from 53% at week 3 p.p. to 15% at week 19 p.p. (P=0·0004). AIA levels decreased significantly from weeks 7 to 11 p.p. (P=0·03) and again from weeks 15 to 19 p.p. (P=0·04). There was no apparent effect of litter or any significant association between worm burden and AIA level.

Fig. 4. Mean (±S.E.) acid-insoluble ash content (AIA, % of faecal dry matter) in faeces from outdoor experimental pigs and indoor control pigs. As the experimental pigs were slaughtered serially the number of sampled animals varied (week 3[ratio ]6; week 7[ratio ]19; week 11[ratio ]17; week 15[ratio ]14; week 19[ratio ]9). Five control pigs were sampled for each age group. Experimental pigs were weaned at week 7 by removal of the sow and control pigs were weaned at week 3·5.
DISCUSSION
The present study has introduced a contamination index (CI) to provide a means of comparing pasture contamination between paddocks though it is acknowledged that the obtained CI is theoretical and can not provide accurate data on deposited numbers of eggs. The CI can, however, be used to bring the present low transmission levels into perspective. Despite the millions of O. dentatum eggs estimated to have been excreted on the paddocks in the spring, overall O. dentatum transmission was very low. Similarly, Andrews et al. (1970) also found low infection levels in pigs born and raised on pasture while more substantial transmission rates have been recorded in other outdoor studies (Dangolla et al. 1994; Nansen et al. 1996). The difference in results may reflect that free-living O. dentatum larvae are relatively sensitive to climatic conditions as transmission may be more substantial during rainy periods (Roepstorff and Murrell, 1997a).
In the present study the weather was relatively warm and dry until late summer and despite irrigation, the combination of dry weather with shorter and sparser vegetation may have been detrimental to the survival of O. dentatum eggs and larvae (Rose and Small, 1981; Kraglund et al. 2001). Tall herbage is considered to protect eggs and larvae better against desiccation and high temperatures (Rose and Small, 1981; Kraglund et al. 2001). Still, irrespective of whether faeces containing eggs was protected by a soil cover or by tall herbage, Kraglund et al. (2001) and Larsen (1996) never recovered more than 1% of the deposited eggs as infective larvae from faecal pats even after only 1 week on pasture plots. Thus, even short-term survival of free-living O. dentatum stages may be very low. Poor larval survival combined with a short stay of only 4 days on the paddocks may thus explain why so few O. dentatum were recovered from the tracer pigs in June.
Despite a high mortality it is known that some larvae may survive for a whole grazing season on pasture plots when deposited in May (Kraglund et al. 2001). This may also have been true for the present study as infective O. dentatum larvae were detected until mid-October. However, it is also likely that a substantial number of the recovered larvae originated from eggs excreted in late July and August by the sows. Eggs deposited as late as August have been shown to develop to infectivity within 1 week after deposition when the mean weekly temperature was above 13 °C (Kraglund et al. 2001). It is therefore possible that transmission to the experimental pigs depended on the continuous deposition of eggs by the sows. Despite a high excretion level it is questionable whether the experimental pigs contributed significantly to the pasture infectivity as their egg counts were relatively low until late September, whereafter it is unlikely that much development took place (Kraglund et al. 2001).
The contamination by the seeder pigs was much less for T. suis than for O. dentatum. Although T. suis eggs are considered to be more resistant than O. dentatum larvae they may still suffer from a high mortality. Thus, Kraglund (1999) found that only 28% and 45% of the deposited T. suis eggs were recovered 4 weeks after deposition on tall herbage plots in late May and early July, respectively. It therefore seems reasonable that many T. suis eggs may also have died during the early phase of the present experiment, though eggs were present on the paddocks throughout the study. The contribution of the sows was probably of little significance due to their very low faecal egg counts and the slow embryonation of the eggs (Burden and Hammet, 1979; Kraglund, 1999; Larsen and Roepstorff, 1999; Roepstorff and Murrell, 1997b).
As for O. dentatum, both tall herbage (Kraglund, 1999) and soil cover (Larsen and Roepstorff, 1999) may increase the survival of T. suis eggs although the embryonation rate may be decreased compared to deposition in short herbage (Kraglund, 1999). Irrespective of how T. suis egg development was presently influenced by biotic and abiotic factors, the overall embryonation was considered to be slow as soil and tracer data indicate that very few T. suis eggs were infective by late autumn. Assuming a pre-patent period of 41–45 days (Powers et al. 1960) it is likely that the T. suis infections of the sows originated from the pre-farrowing paddock. The present data support previous findings that although some T. suis eggs can become infective to pigs within 9–10 weeks during a Danish summer (Mejer et al. 2000) most eggs do not complete development within one summer season (Burden and Hammet, 1979; Kraglund, 1999; Larsen and Roepstorff, 1999; Roepstorff and Murrell, 1997b). None of the eggs excreted by sows and experimental pigs are likely to have become infective in 2001 as eggs deposited from late August to November may not become infective until the next year (Roepstorff and Murrell, 1997a; Kraglund, 1999). Pigs may develop a strong immunity to T. suis infections and worm expulsion normally occurs by weeks 9–11 p.i. (Pedersen and Saeed, 2001). Still, the autumn tracers suggest that the light infections of the experimental pigs were due to low paddock infectivity and not due to expulsion.
One purpose of the present study was to investigate when piglets first become infected when born and raised on contaminated pastures as pigs are active and could become infected early in life through their rooting behaviour. Oesophagostomum dentatum and T. suis were, however, not recovered until the pigs were 7 and 11 weeks old, respectively, except for 1 immature O. dentatum in a 3-week-old piglet. The size of this single worm indicated that it could have been picked up around the time the piglets were given access to the outdoor run. Infective O. dentatum larvae were clearly present when the piglets were born but the almost complete lack of detectable infections in the youngest piglets suggests that transmission within the farrowing huts was negligible. Trichuris suis eggs did not become infective until quite late in the experiment but a few A. suum were detected in 3 out of 6 pigs at week 3 p.p. (Mejer and Roepstorff, 2006). As the transmission of the 2 parasites follows the same route, it is therefore likely that the piglets could also have been neonatally infected with T. suis if infective eggs had been present.
The levels of faecal inorganic matter demonstrated a large difference between the outdoor and indoor pigs. As all feed types contained a minimum of inorganic matter, it is reasonable to conclude that the high faecal AIA content of the outdoor pigs was the result of soil consumption. Vegetation samples were not examined but it is unlikely that ingested vegetation can account for the difference. Faecal AIA content has previously been used as an indicator of geophagy in swine (Beyer et al. 1994) and humans (Wong et al. 1988). Some pastured fatteners tended to be more geophagous than others and mean individual AIA varied from 4·3 to 43·4% of the faecal dry weight (Mejer et al. 1998). Beyer et al. (1994) detected a mean of 7·9% AIA in the faeces of feral hogs. These studies did not consider the host age but the values lie within the variation recorded in the older experimental pigs in the autumn of the present study. Due to the complexity of the present serial data, statistical analysis was not carried out but there was no apparent positive association between geophagy and parasite burden. Previous attempts to make such correlations have been inconclusive (Wong et al. 1991; Mejer et al. 1998). The reason may be that infective stages are heavily aggregated in the environment as illustrated by the present data on free-living O. dentatum larvae and as observed for A. suum eggs (Roepstorff et al. 2001). Surprisingly, geophagy seemed to decrease and not increase as the pigs became more active with increased age in the present study. The reason may be that as the pigs grew they consumed relatively more feed than soil, resulting in a reduced AIA content in the faeces. As the inorganic content is expressed as a percentage of faeces dry weight this may have been of particular importance during the first 7 weeks when the milk-based diet was gradually replaced by solid food.
It is possible that O. dentatum has a rather opportunistic transmission strategy in pigs on pasture. As even short-term egg and larval mortality often seems high, it is possible that a continuous excretion of eggs may only result in a substantial transmission rate when the microclimatic conditions are particularly favourable. In the present study, the build-up of O. dentatum infection in pigs born and raised on a recently contaminated pasture was negligible. This observation fits with the recent finding that organic herds with strictly outdoor production generally had low Oesophagostomum infection levels (Carstensen et al. 2002). The situation seems different for T. suis as the very slow embryonation of eggs means that T. suis probably do not cause severe short-term problems. Even though T. suis eggs face a high mortality, it is not as severe as for O. dentatum (Larsen, 1996; Kraglund, 1999) and the long-term implications for T. suis may therefore be more severe as the eggs may survive for many years on pasture (Hill, 1957; Burden et al. 1987). Correspondingly, Carstensen et al. (2002) found outbreaks of clinical trichuriosis in 2 long-established organic swine herds. The long-term survival of T. suis especially will therefore be monitored in a follow-up study of the present 6 paddocks.
J. Olesen, F. Andersen, M. Høg, M. Pearman, N. Hansen, S. Abrahamsen, K. Henriksen, C. Vinther and the students at the Danish Centre for Experimental Parasitology are gratefully appreciated for their help. The project was financed by The Danish Research Centre for Organic Farming and The Danish National Research Foundation.