1. Geological situation
In southern Argentina, the North Patagonian Massif (Fig. 1) comprises poorly exposed (?upper Proterozoic to) lower Palaeozoic rocks which are intruded by lower Ordovician granitoids (compare, for example, Varela et al. Reference Varela, Cingolani, Sato, Dalla Salda, Brito Neves, Basei, Siga Jr and Teixeira1997, Reference Varela, Basei, Sato, Siga Jr, Cingolani and Sato1998; González, Poiré & Varela, Reference González, Coluccia, Franchi, Caba and Dalponte2002; Pankhurst et al. Reference Pankhurst, Rapela, Fanning and Márquez2006). These units are intruded and covered by upper Palaeozoic to Jurassic plutons and Triassic, Jurassic and Tertiary volcanic rocks, respectively. The geotectonic setting and history of extra-Andean Patagonia have been interpreted in different ways, and there is still a debate about how to interpret the plate tectonics evolution through time.

Figure 1. Geological sketch map of north Patagonia and adjacent areas (modified from Cingolani et al. Reference Cingolani, Dalla Salda, Hervé, Munizaga, Pankhurst, Parada, Rapela, Harmon and Rapela1991 and Pankhurst et al. Reference Pankhurst, Rapela, Fanning and Márquez2006). Diagonal ruling – North Patagonian Massif; frame indicates the position of map of Figure 2; inset map shows location of map area and North Patagonian Massif (NPM).
On the one hand, Ramos (Reference Ramos1984, Reference Ramos1986) postulated that Patagonia represents a terrane that collided with the southwestern (South American) margin of Gondwana during the Carboniferous–Early Triassic time interval. On the other hand, Dalla Salda, Cingolani & Varela (Reference Dalla Salda, Cingolani and Varela1992, Reference Dalla Salda, Cingolani and Varela1993) and Dalla Salda et al. (Reference Dalla Salda, Dalziel, Cingolani and Varela1992, Reference Dalla Salda, Varela, Cingolani and Aragón1994) interpreted Patagonia as having been part of South America since Early Palaeozoic times. In the new model of Pankhurst et al. (Reference Pankhurst, Rapela, Fanning, Márquez, Pankhurst and Veiga2005, Reference Pankhurst, Rapela, Fanning and Márquez2006), however, the suture between Patagonia and Gondwana South America has been placed south of the North Patagonian Massif. The authors propose a NE-directed, early Carboniferous subduction, followed by the middle Carboniferous collision of the Deseado terrane. In their interpretation, the Carboniferous intrusions are related to subduction, and collision was followed by subsequent slab break-off with Permian to Triassic magmatism. The authors argue against a subduction followed by collision at the northern margin of extra-Andean Patagonia.
Palaeomagnetic studies of Rapalini et al. (Reference Rapalini, Tarling, Turner, Flint and Vilas1994) and Rapalini (Reference Rapalini1998) on the one hand suggest that Patagonia has not undergone important displacements relative to South America since Devonian and younger times. On the other hand, Rapalini (Reference Rapalini, Pankhurst and Veiga2005) does not rule out a restricted separation of Patagonia orthogonal to its northern boundary in the Early or Middle Palaeozoic and subsequent collision in the Late Palaeozoic.
The western (pre-Andean) margin of Patagonia was considered as the location of E-directed subduction during at least Late Palaeozoic times (e.g. Forsythe, Reference Forsythe1982; Davidson et al. Reference Davidson, Mpodozis, Godoy, Hervé, Pankhurst and Brook1987; Hervé, Reference Hervé1988; Cingolani et al. Reference Cingolani, Dalla Salda, Hervé, Munizaga, Pankhurst, Parada, Rapela, Harmon and Rapela1991; de Wit & Ransome, Reference De Wit, Ransome, De Wit and Ransome1992; Pankhurst et al. Reference Pankhurst, Hervé, Rojas and Cembrano1992; Martin et al. Reference Martin, Kato, Rodriguez, Godoy, Duhart, McDonough and Campos1999). The upper Palaeozoic intrusive activity in the northeastern part of extra-Andean Patagonia has been interpreted as either the effect of an inner Cordilleran arc, related to subduction in the west (e.g. Cingolani et al. Reference Cingolani, Dalla Salda, Hervé, Munizaga, Pankhurst, Parada, Rapela, Harmon and Rapela1991), or a second magmatic arc, as the response to SW-directed subduction beneath the northern margin of Patagonia, which resulted in collision with Gondwana South America (Ramos, Reference Ramos1984, Reference Ramos1986). Basei et al. (Reference Basei, Varela, Sato, Siga Jr and Llambías2002) and Llambías et al. (Reference Llambías, Varela, Basei and Sato2002) have shown that intrusions in this northeastern sector of the North Patagonian Massif are upper Palaeozoic products of such a ~NW–SE-trending magmatic arc.
The present study covers an area in the western part of the massif between Río Chico–Mamil Choique and Comallo–Paso Flores (Figs 1, 2). Geological evidence from this area has been summarized by, for example, Giacosa et al. (Reference Giacosa, Heredia, Césari, Zubia and González1999b). In particular, the area of Río Chico–Mamil Choique has been the subject of several studies (e.g. Dalla Salda et al. Reference Dalla Salda, Varela, Cingolani and Aragón1994; López de Luchi, Reference López de Luchi1994; Cerredo & López de Luchi, Reference Cerredo and López de Luchi1997, Reference Cerredo and López de Luchi1998; López de Luchi & Cerredo, Reference López de Luchi and Cerredo1996; López de Luchi et al. Reference López de Luchi, Ostera, Cerredo, Linares, Haller and Cagnoni1999, Reference López de Luchi, Ostera, Cerredo, Cagnoni and Linares2000, Reference López de Luchi, Cerredo, Wemmer and Pawling2005). In these contributions, different lithological units are separated from each other, four deformational events with a greenschist to amphibolite-facies metamorphism in the meta-clastic succession are reported, structural and geochemical features of different intrusive rocks are characterized, and various geochronological data are related to a lower Palaeozoic or upper Palaeozoic intrusive, deformational and metamorphic history. Some of their results are in accordance with those presented here. From the Comallo–Paso Flores area and further to the north, results of structural, petrological, geochemical and geochronological studies have been reported by Varela et al. (Reference Varela, Dalla Salda, Cingolani and Gómez1991, Reference Varela, Basei, Brito Neves, Sato, Teixeira, Cingolani and Siga1999, Reference Varela, Basei, Cingolani, Siga Jr and Passarelli2005). They describe three deformational episodes (Varela et al. Reference Varela, Dalla Salda, Cingolani and Gómez1991), and upper Carboniferous–lower Permian intrusions were tentatively related to the activity of a magmatic arc (Varela et al. Reference Varela, Basei, Brito Neves, Sato, Teixeira, Cingolani and Siga1999), whose existence was also postulated by Varela et al. (Reference Varela, Basei, Cingolani, Siga Jr and Passarelli2005).

Figure 2. Geological overview map of the western part of the North Patagonian Massif between Paso Flores and Gastre depicting the Palaeozoic to Triassic units. Map compiled after Ravazzoli & Sesana (Reference Ravazzoli and Sesana1977); Nullo (Reference Nullo1978, Reference Nullo1979); Proserpio (Reference Proserpio1978); Volkheimer & Lage (Reference Volkheimer and Lage1981); Llambías et al. (Reference Llambías, Llano, Rossa, Castro and Puigdomenech1984); Rapela, Pankhurst & Harrison (Reference Rapela, Pankhurst and Harrison1992); López de Luchi (Reference López de Luchi1994); Giacosa et al. (Reference Giacosa, Heredia, Zubia and González1999a) and González et al. (Reference González, Poiré and Varela2002). Location of North Patagonian Massif (NPM) in southern Argentina is shown in the inset map. Circled numbers refer to locations treated in text: 1 – Cañadon Chacay Huarruca, 2 – Cañadon La Angostura, 3 – Comallo, southwest, 4 – Comallo tonalite, 5 – Loma Carhué, 6 – Loma Miranda, east, 7 – Loma Miranda, south, 8 – Mamil Choique, south, 9 – Paso Flores tonalite, 10 – Puesto García.
As the study area is near the former (upper Palaeozoic) active western margin of Patagonia, one should expect to find evidence for related compression and plutonism. Furthermore, if extra-Andean Patagonia was an independent plate that collided with Gondwana South America, or was divided by an internal collision zone, then effects of the compressive deformation can also be recorded in this western segment. In the following text, the sequential order of structural and intrusive events, found during structural field studies in the western part of the North Patagonian Massif, is summarized (see also von Gosen, Reference Gosen2005). This will finally lead to some larger-scale regional implications along with preliminary interpretations concerning the geotectonic setting of extra-Andean Patagonia during Late Palaeozoic times.
2. Lithological units
The Cushamen Formation (Volkheimer, Reference Volkheimer1964), exposed in the Comallo and Río Chico areas (Fig. 2), comprises phyllites, quartzite layers, (injected) micaschists and gneisses, and various layers of metavolcanic rocks (e.g. López de Luchi, Reference López de Luchi1994; Cerredo & López de Luchi, Reference Cerredo and López de Luchi1998). Intercalated layers of granites, pegmatites and aplites record different relationships to the main foliation (compare also Franzese, Dias & Dalla Salda, Reference Franzese, Dias and Dalla Salda1992; Márquez et al. Reference Márquez, Giacosa, Nillni, Paredes, Fernández, Parisi, Sciutto, Garrido and Afonso2002). The migmatites northwest of Comallo also partly comprise injected schists.
The Cushamen Formation has been interpreted to be Precambrian and/or Early Palaeozoic in age (e.g. Volkheimer, Reference Volkheimer1964; Ravazzoli & Sesana, Reference Ravazzoli and Sesana1977; Proserpio, Reference Proserpio1978; Nullo, Reference Nullo1979; Volkheimer & Lage, Reference Volkheimer and Lage1981; Llambías et al. Reference Llambías, Llano, Rossa, Castro and Puigdomenech1984; Dalla Salda, Cingolani & Varela, Reference Dalla Salda, Cingolani and Varela1990; López de Luchi & Cerredo, Reference López de Luchi and Cerredo1996). This was also supported by Rb–Sr and K–Ar dates from different rock types in the western and southwestern part of the North Patagonian Massif (e.g. Linares et al. Reference Linares, Cagnoni, Do Campo and Ostera1988, Reference Linares, Haller, Ostera, Cagnoni and Galante1997; Ostera et al. Reference Ostera, Linares, Haller, Cagnoni and López de Luchi2001). Duhart, Haller & Hervé (Reference Duhart, Haller and Hervé2002), however, have shown the existence of a diamictite in the succession, similar to those of the upper Palaeozoic sedimentary rocks of the Esquel basin, which then could be late Palaeozoic in age. Such an age has been confirmed in both the Esquel and Cushamen formations by isotopic dating of detrital zircons (U–Pb, SHRIMP) by Hervé et al. (Reference Hervé, Haller, Duhart and Fanning2005). Thus, the protoliths of the Cushamen Formation should be late Palaeozoic in age and in parts might be older.
The Calcatapul Formation (Proserpio, Reference Proserpio1978) consists of a metamorphic succession of various metavolcanic rocks along with thin intercalations of phyllites and lenses of metaconglomerates (von Gosen & Loske, Reference Gosen and Loske2004). It is exposed northwest of the village of Gastre (Chubut province) and has been assigned to the Early Palaeozoic (?Silurian, ?Devonian) by Proserpio (Reference Proserpio1978). Its relationships with the Cushamen Formation and geotectonic significance are unclear.
The Mamil Choique Formation (Ravazzoli & Sesana, Reference Ravazzoli and Sesana1977) consists of a wide range of deformed and undeformed tonalites to granodiorites, granites, leucogranites, pegmatites and migmatites (compare Nullo, Reference Nullo1979). The different magmatics intruded the Cushamen Formation rocks and have been assigned to a wide span between the Precambrian and Late Palaeozoic (e.g. Ravazzoli & Sesana, Reference Ravazzoli and Sesana1977; Nullo, Reference Nullo1978, Reference Nullo1979; Proserpio, Reference Proserpio1978; Dalla Salda et al. Reference Dalla Salda, Varela, Cingolani and Aragón1994). In the Río Chico–Mamil Choique area, Dalla Salda et al. (Reference Dalla Salda, Varela, Cingolani and Aragón1994) created the lower Palaeozoic Río Chico Complex, comprising the Cushamen Formation and Mamil Choique Granitoids, the latter with an Ordovician Rb–Sr age, from the Devonian Viuda de Gallo and Permian La Pintada granite (Dalla Salda et al. Reference Dalla Salda, Varela, Cingolani and Aragón1994). These units were defined based on field observations plus petrological and geochronological data. Later, the foliated intrusive rocks between Río Chico and Mamil Choique were assigned to the Mamil Choique Granitoids and Tunnel Tonalites (e.g. López de Luchi & Cerredo, Reference López de Luchi and Cerredo1996; Cerredo & López de Luchi, Reference Cerredo and López de Luchi1998; López de Luchi et al. Reference López de Luchi, Ostera, Cerredo, Linares, Haller and Cagnoni1999). For the former, López de Luchi et al. (Reference López de Luchi, Ostera, Cerredo, Linares, Haller and Cagnoni1999) suggest a Carboniferous Rb–Sr whole-rock age which is in support of an isotopic date of Linares et al. (Reference Linares, Haller, Ostera, Cagnoni and Galante1997). On the contrary, early Permian U–Pb ages have been reported for a granodiorite and muscovite migmatite southwest of Mamil Choique (Varela et al. Reference Varela, Basei, Cingolani, Siga Jr and Passarelli2005; Pankhurst et al. Reference Pankhurst, Rapela, Fanning and Márquez2006), a middle Permian age from a granodiorite gneiss in the Sierra del Medio (Rapela, Pankhurst & Harrison, Reference Rapela, Pankhurst and Harrison1992), whereas middle to late Permian ages (based on the time scale of Gradstein et al. Reference Gradstein, Ogg, Smith, Bleeker and Lourens2004) were obtained for the La Pintada leucomonzogranites (Dalla Salda et al. Reference Dalla Salda, Varela, Cingolani and Aragón1994; López de Luchi et al. Reference López de Luchi, Ostera, Cerredo, Cagnoni and Linares2000).
For the tonalites (to granodiorites) of Río Chico (‘Tunnel Tonalite’: López de Luchi & Cerredo, Reference López de Luchi and Cerredo1996), east and northwest of Comallo and at Paso Flores (Fig. 2), U–Pb dating of zircons yielded early Permian ages (Varela et al. Reference Varela, Basei, Cingolani, Siga Jr and Passarelli2005; Pankhurst et al. Reference Pankhurst, Rapela, Fanning and Márquez2006). For tonalites, granodiorites and granites of the El Platero Formation (Volkheimer, Reference Volkheimer1964) and Laguna del Toro Granitoids (Rapela, Pankhurst & Harrison, Reference Rapela, Pankhurst and Harrison1992) in the northern part of the Chubut province (Fig. 2), a U–Pb zircon-dating (SHRIMP) of the Laguna del Toro granodiorite (Pankhurst et al. Reference Pankhurst, Rapela, Fanning and Márquez2006) and a K–Ar dating of a tonalite (Proserpio, Reference Proserpio1978) suggest that most of these rocks belong to the upper Palaeozoic suite of intrusions.
The Lipetrén Formation (Nullo, Reference Nullo1978) consists of various granites, porphyries, pegmatites and aplites and has been assigned to the Permian (e.g. Nullo, Reference Nullo1978, Reference Nullo1979; Proserpio, Reference Proserpio1978; Volkheimer & Lage, Reference Volkheimer and Lage1981) or Permo-Triassic (Cucchi, Reference Cucchi1993). In the Gastre area, the Lipetrén Formation rocks were renamed the Lipetrén Suite (or Superunit) by Rapela et al. (Reference Rapela, Dias, Franzese, Alonso and Benvenuto1991) and Rapela, Pankhurst & Harrison (Reference Rapela, Pankhurst and Harrison1992), who separated these rocks from the older Gastre Suite of intrusions also based on Rb–Sr whole-rock ages. Their Triassic ages for both units are shown in Figure 2, although an older age for parts of the Lipetrén Formation is assumed, based on the isotopic dating of the overlying Taquetrén Formation (Franzese et al. Reference Franzese, Pankhurst, Rapela, Spalletti, Fanning and Muravchik2002).
3. Structure and intrusions
3.a. D1-deformation and first stage of intrusive activity
Within the Cushamen Formation, a penetrative S1-foliation is only locally preserved in the Río Chico area (Chacay Huarruca, Cañadón La Angostura, Fig. 2). On S1-planes, a ~N–S-striking L1-lineation is defined by aligned muscovite and biotite in the metaclastic rocks and long axes of amphibole in penetratively foliated layers of amphibole–plagioclase schists.
The S1-foliation also affects intercalated, up to tens of metres thick, layers of muscovite granite, pegmatite and aplite. Within the magmatic rocks, the L1-lineation is recorded by long axes of pressure shadows at feldspar clasts and aligned muscovite and sericite. Single σ-shapes of feldspar clasts indicate a top-to-the-~N sense of shear. These granites, pegmatites and aplites seem to belong to a first stage of the intrusive activity that affected the pelitic to psammopelitic country rocks before the D1-deformation. Up to decimetre-thick pegmatite layers were injected syn-D1. They are boudinaged and/or folded within the S1-foliation and are attributed to a continuing magmatic activity of the first stage of intrusions.
3.b. Second stage of intrusive activity
After the D1-deformation of the Cushamen Formation plus granite and pegmatite layers, tonalites to granodiorites and widely distributed granites and pegmatites intruded. In the area northwest of Comallo (south of Loma Miranda, southwest of Puesto García; Fig. 2), the tonalite and country rocks were injected by muscovite granites, leucogranites and pegmatites that mostly form layers parallel to the main foliation fabric (Fig. 3). Due to the penetrative S2-foliation of the metaclastic country rocks (=S1 foliation in these intrusive rocks; see next Section), cross-cutting relationships with the older foliation in the country rocks could not be detected. Only some pegmatites are discordant and represent dykes. The margins of the tonalite contain layers, lenses and thin strips of the Cushamen Formation metapsammopelites (Fig. 4a).

Figure 3. Simplified and schematic block profiles across the contact between the tonalite and Cushamen Formation rocks south of Loma Miranda (a) and southwest of Puesto García (b), northwest of Comallo (compare Fig. 2 for locations). The tonalite and various younger magmatic layers were affected by the D2-deformation of the Cushamen Formation. Their D1-structures are equivalents to the D2-structures in the country rocks. The sense of shear is top-to-the-~E.

Figure 4. (a) View of thin alternating layers of granodiorite to tonalite and micaschists of the Cushamen Formation (arrows) at the intrusive tonalite contact northwest of Comallo (south of Loma Miranda). The penetrative S2-foliation also affected the magmatic rocks as S1-foliation. Diameter of lens cap is 6 cm. (b) Leucogranite plus pegmatite layer intruded in the Cushamen Formation southwest of Comallo. The injections follow the S2-foliation of the country rocks and enclose strips and lenses of the micaschists (arrows). Handle of hammer is 30 cm long. (c) Diffuse open folding in the tonalite to granodiorite east of Comallo. The structure is interpreted as related to local deformation during cooling of the pluton. Lens cap is 6 cm in diameter. (d) Foliated xenoliths of the Cushamen Formation metapsammopelitic rocks (arrows) within the tonalite east of Comallo. Diameter of lens cap is 6 cm. (e) Angular xenoliths of the Cushamen Formation within the granodiorite to granite of the Mamil Choique intrusions west of Comallo. The foliated xenoliths are injected by thin granitic layers (arrows). Lens cap is 6 cm in diameter. (f) Open F3-fold structure in micaschists of the Cushamen Formation northwest of Comallo (southwest of Puesto García). Diameter of lens cap is 6 cm. (g) and (h) Photomicrographs from thin-sections, crossed polarizers. (g) Isoclinal F2-fold in S2-foliation of Cushamen Formation micaschist northwest of Comallo (Puesto García). (h) Foliated tonalite northwest of Comallo (south of Loma Miranda). Note elongated and recrystallized quartz and asymmetric pressure shadow at plagioclase clast. Relative sense of shear is top-to-the-E (indicated by white half arrows).
Southwest of Comallo, a coarse-grained muscovite granite intruded the metaclastic rocks (Fig. 5a). In the Cushamen Formation west of Comallo (Loma Carhué, Fig. 2) and just west of Río Chico, several decimetre- to metres-thick layers of muscovite granites, pegmatites and aplites were injected after the D1- and before the D2-deformation (e.g. Fig. 5b). All these intrusions have sharp contacts toward the country rocks.

Figure 5. (a) Block profile across the contact between the Cushamen Formation and a coarse-grained granite in the south (southwest of Comallo). The schematic and simplified section shows that D1-structures in the granite were created during the D2-deformation of the Cushamen Formation rocks. The sense of shear is top-to-the-~ENE. A younger leucogranite along with pegmatites intruded the contact and the metaclastic succession during the D2-event of the Cushamen Formation. They are affected only by single and diffuse S-planes that can be traced into the S1- or S2-foliation of the granite or metaclastic rocks, respectively. (b) Penetrative S2-foliation in the Cushamen Formation and equivalent S1-foliation within intercalated granites and pegmatites at Loma Carhué, west of Comallo (composite block profile, schematic and simplified). The sense of shear is top-to-the-NE. F1-folds (intrusions) and S2-planes (country rocks) are partly overprinted by open F3-folds. For locations of (a) and (b) see Figure 2.
3.c. D2-deformation
The metaclastic rocks of the Cushamen Formation along with the first and second stage magmatic intrusions are affected by a mostly penetrative S2-foliation (Fig. 4a). Like the S1-foliation, it has been described by, for example, Cerredo & López de Luchi (Reference Cerredo and López de Luchi1998). Layers of first-stage granites are bent around open F2-folds and/or are cross-cut by an S2-cleavage/foliation. Magmatic rocks of the second intrusive stage are penetratively foliated for the first time (S1; Figs 3, 5). Single granite, pegmatite and aplite layers, also within granite intrusions, are foliated, boudinaged and/or tightly to isoclinally folded around F1-structures (e.g. Fig. 5b) which correspond to the F2-folds of the country rocks. There, tight to isoclinal F2-folds on a centimetre- to several metres-scale have variable axes orientations (Fig. 6a–c, e). Centimetre-thick quartz layers, generated during the S1-foliation development, are isoclinally folded.

Figure 6. Lower hemisphere, equal area stereoplots of structural elements. In the Hoeppener plots (Hoeppener, Reference Hoeppener1955), the lineation is projected on the pole of the respective plane; arrows indicate the relative sense of shear of the hanging-walls. B2-axes in Cushamen Formation are shown in normal lower hemisphere projection. (a–c, e) L2-lineation in Cushamen Formation and equivalent lineation in intrusive rocks. (a) Tonalite and Cushamen Formation northwest of Comallo at Puesto García; (b) tonalite and Cushamen Formation northwest of Comallo, south of Loma Miranda; (c) Cushamen Formation and intrusions southwest of Comallo; (d) granodiorite to granite north of Comallo, east of Loma Miranda; (e) Cushamen Formation and intrusions at Loma Carhué; (f) Paso Flores tonalite; (g) granodiorite south of Mamil Choique; (h) Comallo tonalite.
On S2-planes of the Cushamen Formation, the L2-lineation is defined by aligned muscovite and biotite. Elongate quartz and feldspar, aligned muscovite, and long axes of partly disrupted tourmaline (pegmatites) display the equivalent L1-lineation on S1-planes within the deformed second-stage intrusive rocks (e.g. Figs 3, 5). Widely distributed feldspar σ-clasts, single S/C fabrics and shear bands indicate a top-to-the-~E (northwest and southwest of Comallo; Fig. 6a–c) or ~NE (north of Comallo and Loma Carhué: Fig. 6d, e) sense of shear parallel to the L2-lineation (=L1-lineation within the second-stage intrusions). In the Río Chico area, the L2-lineation plunges to the west or is vertical.
During the D2-deformation, centimetre- to several metres-thick pegmatite and aplite dykes are injected into the Cushamen Formation and deformed older intrusive rocks. The dykes are boudinaged and/or folded within the S2-foliation but not penetratively sheared (Figs 3, 5). At their margins, idiomorphic feldspar overgrew the foliation of the country rocks.
Southwest of Comallo (Fig. 2), the contact between a coarse-grained pre-D2 granite and the Cushamen Formation is intruded by a leucogranite body (Fig. 5a) that displays transitional and partly diffuse contacts toward pegmatite layers interpreted as dykes. These are oriented approximately parallel to the intrusive boundaries with the country rocks. The leucogranite and pegmatites are affected by a weak and diffuse (S1-) foliation that could be traced into the penetrative (S2-) foliation of the country rocks. There, dykes of both rocks are folded around F2-structures and/or are boudinaged (Fig. 5a). Within the coarse-grained granite, apophyses of the leucogranite are folded and syn-S1-pegmatite layers are boudinaged. All these magmatic injections during the D2-deformation of the Cushamen Formation are interpreted to belong to the continuation of the second stage of intrusive activity. Angular xenoliths of the deformed country rocks, enclosed by a leucogranite plus pegmatite layer southwest of Comallo (Fig. 4b), suggest that the magma emplacement was accompanied by brittle fracturing.
3.d. Third stage of intrusive activity
After the D2-deformation of the Cushamen Formation, tonalites to granodiorites and various granites intruded. Here they are broadly separated into two groups.
3.d.1. Tonalites to granodiorites
In the tonalites to granodiorites southeast of and at Río Chico, east of Comallo and at Paso Flores (Fig. 2), a diffuse layering (e.g. Tunnel Tonalite) can be interpreted as the effect of compression during cooling of the magmatics (magmatic foliation of Cerredo & López de Luchi, Reference Cerredo and López de Luchi1997). Such an explanation is also supported by single diffuse fold structures around ~N–S axes within the Comallo tonalite (Fig. 4c). The tonalites of Paso Flores and east of Comallo, and a porphyric granodiorite north of Comallo (only exposed at the eastern margin of Loma Miranda) in some parts contain metre-long angular xenoliths of the foliated Cushamen Formation (e.g. Figs 4d, 7).

Figure 7. Relationships between older granodiorite to granite intrusion and younger leucogranite with pegmatites within outcrops at the eastern margin of Loma Miranda (north of Comallo, compare Fig. 2 for location; schematic and simplified block sketch). The older intrusion is partly affected by a S1-foliation and contains angular xenoliths of the foliated Cushamen Formation. C2-shear planes are the first deformational structures in the younger leucogranite and pegmatites which partly record a compositional layering.
3.d.2. Mamil Choique granodiorites to granites
Southwest of Mamil Choique and west of Comallo, the Mamil Choique intrusive rocks record a layering displayed by differences in composition on a centimetre- to decimetre-scale, ranging from granodiorite to granite and younger leucogranites and pegmatites, but also with diffuse transitions. This layering in the intrusive rocks has been described also by, for example, López de Luchi (Reference López de Luchi1994), López de Luchi & Cerredo (Reference López de Luchi and Cerredo1996) and Cerredo & López de Luchi (Reference Cerredo and López de Luchi1998). The layers can be separated by (maximum) millimetre-thick, and mostly diffuse biotite-rich strips which are not continuous (Fig. 8).

Figure 8. Simplified and schematic block sketch to illustrate the structures within the Mamil Choique granodiorite to granite west of Comallo (scale is approximate). The compositional layering within the intrusions is recorded by alternating granodiorite and granite, younger leucogranite and pegmatite and diffuse strips and schlieren of biotite. The layering has the same orientation as the older S2-foliation within angular xenoliths of the Cushamen Formation rocks which is partly related to relics of F2-folds. The younger leucogranite and pegmatites follow the boundaries of the xenoliths or invade their S2-fabric. An open bending to folding affected the entire succession. (For location compare Fig. 2)
Parts of the intrusions contain a large amount of angular xenoliths of the Cushamen Formation, centimetres to tens of metres in size (compare Cerredo & López de Luchi, Reference Cerredo and López de Luchi1998; Fig. 4e herein). Structures of the D2-deformation within the xenoliths, partly recorded by isoclinal F2-folds, have no equivalents in the intrusions.
West of Comallo and partly southwest of Mamil Choique, the diffuse and steeply inclined layering of the intrusions strikes ~W–E to ~NE–SW (Fig. 9a, d). It is almost parallel to the orientation of the S2-foliation within the xenoliths (Fig. 9b, e; compare also Varela et al. Reference Varela, Dalla Salda, Cingolani and Gómez1991). Some large xenoliths southwest of Mamil Choique partly record variable orientations with respect to the layering. Within the layers, feldspar depicts idiomorphic shapes with random orientations. In parts it grew across layer boundaries without evidence for solid-state deformation. Therefore, the layering is interpreted to be of magmatic origin and afterwards was affected by heterogeneous shearing.

Figure 9. Lower hemisphere, equal area stereoplots of structural elements. Arrows and dot-line symbols are Hoeppener plots in which the lineation is projected on the pole of the respective plane (Hoeppener, Reference Hoeppener1955). Arrows indicate relative sense of shear/displacement of the hanging-walls. Data from Mamil Choique intrusions with Cushamen Formation xenoliths are from west of Comallo (a– c) and southwestern margin of the Sierra de Mamil Choique (SW of Mamil Choique; d–f). (g) Loma Carhué west of Comallo; (h) northwest of Comallo (southwest of Puesto García); (i) south of Loma Miranda, northwest of Comallo; (j) southwest of Comallo; (k) southern margin of Cañadón Chacay Huarruca and Cañadón La Angostura west of Río Chico; (l) just west of Río Chico river; (m) Paso Flores tonalite with brittle shear planes and reverse faults; (n) Cushamen Formation just west of Río Chico river (southwest of Río Chico village) with brittle shear planes and reverse faults; (o) conjugate brittle C1-shear zones in granodiorite and adjacent monzogranite southeast of Mamil Choique.
Younger millimetre- to metre-thick leucogranites, pegmatites and aplites injected these layers, the boundaries of angular xenoliths, or they injected large xenoliths parallel to their S2-planes (Fig. 4e). They invaded an S1-foliation within a granodiorite south of Mamil Choique and by this also created a compositional layering. A layering of alternating leucogranite and pegmatite is also recorded north of Comallo. There, both intruded the boundary between a foliated granodiorite to granite in the south and a porphyric granodiorite in the north (exposed only at the eastern margin of Loma Miranda; Fig. 7).
As the layering is lacking in the syn-D2 and older intrusions, the above granitoids are assigned to the third stage of magmatic activity. Angular xenoliths of the Cushamen Formation indicate that the emplacement of the granitoids occurred at upper levels of the crust with brittle fracturing of the country rocks after cessation of their ductile D2-deformation. This is supported by the results of Cerredo & López de Luchi (Reference Cerredo and López de Luchi1998), who report that hornfelsic textures overprinted the S2-foliation.
3.e. D3-deformation
After the D2-deformation, the Cushamen Formation and intercalated intrusions were bent around open F3-folds with different vergencies on a centimetre- to several metres-scale (compare also Cerredo, Reference Cerredo1997; Cerredo & López de Luchi, Reference Cerredo and López de Luchi1998; Fig. 4f). The B3-axes strike ~NE–SW at Loma Carhué (Fig. 9g), around W–E in the Comallo area (Fig. 9h–j) and ~NNE–SSW to ~NNW–SSE west of Río Chico village (Fig. 9k, l). C3-shear planes are related to the folds and are spaced on a centimetre- to decimetre-scale. The observations suggest that the D3-deformation in these areas was the result of a variable ~W–E to ~N–S compression.
At Río Chico, east of Comallo and at Paso Flores, the tonalites to granodiorites are affected by an S1-foliation. In some parts, aligned feldspar, biotite, and ± amphibole record a ~N–S to NE–SW L1-lineation. In the Paso Flores tonalite, asymmetric feldspar σ-clasts indicate a top-to-the-N sense of shear (Figs 6f, 10), and in a granodiorite south of Mamil Choique a top-to-the-S sense of shear (Fig. 6g). A conjugate set of subvertical dextral and sinistral shear planes in the tonalite east of Comallo, along with distinct shearing parallel to single, weakly developed shear planes, indicate ~NNE–SSW compression (Fig. 6h).

Figure 10. Schematic and simplified block sketch of D1- to D3-structures within the Paso Flores tonalite (for location compare Fig. 2). The sense of shear during the D1-deformation is shown by half arrows. Note open F3-folding around a N–S-trending axis combined with C3-shear planes and reverse faults. δsl2 and δsl3 indicate slickenside lineations on C2-planes and C3-planes/faults, respectively.
In the Mamil Choique granitoids, a bending and/or open folding of the layering on a decimetre- to metre-scale around mostly ~W–E-striking axes (Figs 8, 9c, f) is related to subsequent and continuous ~N–S compression. Such a mechanism can also explain the fold interference patterns, described by Cerredo & López de Luchi (Reference Cerredo and López de Luchi1998) and interpreted as the result of two stages of folding.
C2-planes within the younger leucogranites and pegmatites east of Loma Miranda (north of Comallo; Fig. 7) and southwest of Comallo can be compared with D3-structures in the Cushamen Formation and its intercalated magmatic layers. Furthermore, a conjugate set of dextral and sinistral C2-planes, along with S-dipping reverse planes, indicate ~N–S compression in the Paso Flores tonalite (Fig. 10). This is interpreted as the continuation of the D1-deformation in the tonalite that can roughly be compared with D3-structures in the Cushamen Formation.
Within the Tunnel Tonalite of Río Chico, the S1-foliation, as probable expression of the D3-deformation in the country rocks, is cross-cut by single centimetre-thick, cataclastic C2-shear zones, which point to N–S compression. They can be compared with C4-planes and reverse faults in the intruded Cushamen Formation. These overprints by structures of the D3- and D4-deformations of the country rocks have also been described by Cerredo & López de Luchi (Reference Cerredo and López de Luchi1998). Thus, the tonalite emplacement probably took place after the D2-event of the country rocks. Cerredo & López de Luchi (Reference Cerredo and López de Luchi1998) suggest a pre-D3 emplacement of the Tunnel Tonalites.
3.f. Fourth stage of intrusive activity
The final stage of the intrusive activity is recorded by various pegmatites and aplites which were injected into the Cushamen Formation and older intrusions as centimetre- to several metres-thick dykes with different orientations. The magmatic rocks are not ductilely deformed (Figs 5a, 7, 8). In the monzogranites to leucomonzogranites at Mamil Choique, formerly assigned to the Viuda de Gallo and La Pintada granites (Fig. 2), no ductile foliation was found, and it is assumed that they also belong to this final stage of intrusive activity.
3.g. D4-deformation
The Cushamen Formation south of Río Chico village was affected by a local F4-folding (also compare, e.g. Cerredo, Reference Cerredo1997; Cerredo & López de Luchi, Reference Cerredo and López de Luchi1998) on an estimated scale of more than 100 m. Deformation under brittle conditions was accompanied by the formation of shear planes, spaced on a decimetre- to metre-scale, and a few small accommodation reverse faults. These planes indicate a top-to-the-E and -NE sense of shear or displacement (Fig. 9n). F3-folds are bent around minor E-vergent F4-structures.
Single conjugate brittle shear zones within a granodiorite and adjacent (La Pintada) monzogranite southeast of the village of Mamil Choique can be interpreted as equivalents of this deformation under ~W–E compression (Fig. 9o). The open bending of the Paso Flores tonalite around a N–S-striking axis, accompanied by a dense set of C3-shear planes and reverse faults (Figs 9m, 10), also may be part of this brittle deformation.
4. Microfabrics and metamorphism
4.a. Cushamen Formation and intercalated intrusive rocks
The locally preserved fabrics of the D1-deformation in the Cushamen Formation show that it was accompanied by the growth of muscovite and biotite and recrystallization of quartz and feldspar. In some micaschist layers, up to millimetre-long lens-shaped sericite ± chlorite ± biotite aggregates represent relics of former porphyroblasts (?andalusites). The penetrative S2-foliation led to elongation of quartz and alignment of biotite and muscovite and contains relics of isoclinal intrafolial F2-folds (Fig. 4g). Dynamic recrystallization of quartz, mica and plagioclase along with the growth of garnet took place during the D2-event. These features could also be detected in foliated granite and pegmatite layers and are related to the D1-deformation of the intrusive rocks. On a microscale, the sense of shear found in the field could be confirmed (e.g. Figs 4h, 11a). During the D2-deformation, sericite aggregates in S1-planes of the Cushamen Formation rocks are bent around F2-crenulations and are sheared and elongated in S2-foliation planes (Fig. 11b).

Figure 11. Photomicrographs from thin-sections, crossed polarizers. (a) Penetratively foliated pegmatite layer within Cushamen Formation micaschists at Loma Carhué (south of Paso Flores). Asymmetric σ-shapes of muscovite fishes and S/C-fabrics indicate a top-to-the-NE sense of shear (white half arrows). (b) F3-fold in micaschist of Cushamen Formation just west of Río Chico river. Note bending of S2-foliation combined with ductile deformation of quartz. In the foliation a sericite aggregate (arrow) is elongated. (c) Hinge zone of F3-microfold in micaschist of the Cushamen Formation (northwest of Comallo, Puesto García). F3-folding is outlasted by recrystallization of biotite and quartz and contrasts to the situation in the F3-fold of (b). (d) Xenolith of Cushamen Formation micaschist enclosed in Mamil Choique granitoid (south of Mamil Choique, ESE of Puesto Llamonao). Relics of subvertical S2-foliation are depicted by aligned and recrystallized biotite; quartz and plagioclase are also recrystallized. (e) Foliation in Mamil Choique granodiorite southwest of Mamil Choique. Note elongated and ductilely deformed quartz. (f) Local ductile deformation of quartz between feldspar clasts in Mamil Choique granitoid southwest of Comallo. The main layering is subvertical and depicted by aligned biotite. (g) Local ductile shearing in Comallo tonalite recorded by elongated quartz between feldspar clasts. At grain boundaries, finest-grained dynamically generated quartz recrystallization grains occur. (h) Distinct S1-planes in Paso Flores tonalite indicated by extremely elongated quartz between feldspar clasts. Indications of S/C-fabrics suggest a top-to-the-N sense of shear (shown by white half arrows).
After the D2-deformation, static metamorphic conditions are shown by (continuing) growth of garnet, muscovite and biotite within the micaschists, along with static annealing of quartz and growth of feldspar recrystallization grains.
In the tonalite northwest of Comallo (south of Loma Miranda and south of Puesto García; Fig. 2) the first penetrative S1-foliation (=S2-foliation in the Cushamen Formation rocks) is marked by layers of elongate and coarse quartz grains that record undulatory extinction. The old grains are coarsely recrystallized up to statically post-S1 with straightened grain boundaries forming triple junctions between recrystallization grains. Biotite recrystallization grains almost entirely replaced magmatic grains and are aligned in S1-planes. Their growth also outlasted the S1-foliation development.
Angular magmatic plagioclase records deformation twins, is partly bent and can show metamorphic overgrowths. Minor and mostly small recrystallization grains occur at grain boundaries and in strain shadows. Together with clinozoisite, partly coarse epidote grew in pressure shadows and as poikiloblasts partly across S1-foliation planes. σ-shapes of polycrystalline quartz lenses and pressure shadows at plagioclase show a relative top-to-the-E sense of shear that supports the field observations (Fig. 4h).
Based on the above microstructures, the metamorphic overprint can be estimated to have reached the middle greenschist facies during and post-D1 within the tonalite and D2 of the Cushamen Formation rocks. The observations point to an increase in temperature during and statically after D1/D2.
The overprint on a local and regional scale, however, was variable. This is shown by the phyllitic succession northwest of Río Chico, which seems to grade over a short distance into micaschists in the east and south. A short-distance and west-to-east increase in metamorphism also occurs in the Cañadón La Angostura (Dalla Salda et al. Reference Dalla Salda, Varela, Cingolani and Aragón1994; López de Luchi, Reference López de Luchi1994). These effects might be related to a variable local heat transfer from intrusions during and after the D2-deformation.
Cerredo (Reference Cerredo1997) has shown that regional metamorphism of the Cushamen Formation west of Río Chico spans a wide range from the chlorite zone in the northwest, over the biotite–garnet zone in the east, to the K-feldspar zone (migmatitic rocks) in the southeast. She reports the growth of sillimanite and suggests that the regional metamorphism was of medium-pressure type. López de Luchi & Cerredo (Reference López de Luchi and Cerredo1996) and Cerredo & López de Luchi (Reference Cerredo and López de Luchi1998) state that peak metamorphic conditions occurred synchronous with or shortly after the second deformation.
The above fabrics of a static heating in the Cushamen Formation and intercalated intrusive rocks are overprinted by a slight ductile deformation of quartz (e.g. undulation, bending) which also affects older triple junctions between quartz recrystallization grains and led to new dynamically generated recrystallization grains. Magmatic plagioclase, however, is brittly deformed. Older biotite recrystallization grains are bent, kinked and partly replaced by new recrystallization grains. Metamorphism during this deformation was in the range of the lower greenschist facies.
The second deformation in the tonalite south of Loma Miranda and south of Puesto García (=D3-deformation in the Cushamen Formation rocks) is recorded by undulation, bending and partly by kinking that affected the previously recrystallized quartz fabrics along with their straightened grain boundaries.
In the Cushamen Formation, the effects of the temperature overprint during the D3-deformation are variable in the different areas. In some parts of the Río Chico area, biotite and muscovite recrystallization continues during the F3-crenulation along with muscovite growth. Elongate quartz is bent, and only single, dynamically generated recrystallization grains occur. Just west of Río Chico, however, biotite and muscovite are bent and kinked around F3-folds without evidence for recrystallization. Biotite is widely converted into chlorite, whereas new sericite + chlorite and small dynamically generated quartz recrystallization grains occur. Retrogression during the D3-deformation in the Río Chico area has also been shown by Cerredo (Reference Cerredo1997). In the area north of Comallo, however, the metamorphic overprint mostly outlasts the F3-folding under static conditions (e.g. recrystallized mica around fold hinges (Fig. 11c), grain growth of quartz). It can be assumed that post-D2 emplacements of different intrusions caused the static heating of the deformed country rocks.
4.b. Mamil Choique granodiorites to granites
Within the Mamil Choique granodiorites to granites an initial diffuse foliation fabric is recorded by aligned and recrystallized biotite, derived from magmatic grains and partly elongate quartz. Plagioclase grains can display a preferred long-axes alignment without clear recrystallization, whereas relics of magmatic plagioclase in other thin-sections contain recrystallization grains also in strain shadows. This stage of a tempered foliation is also shown by recrystallization and grain growth of quartz leading to statically annealed textures. Muscovite and biotite grew across the weak foliation, which records continuous transitions into unfoliated parts. No clear evidence was found for a continuation of the foliation into xenoliths. It is reasonable to suggest that this deformation took place during (the final stage of) cooling of the intrusion. This is supported by well-preserved micrographic intergrowths of K-feldspar and quartz.
In the xenoliths, the old foliation is depicted by aligned and recrystallized biotite (Fig. 11d). Only in rare parts, relics of intrafolial F2-folds could be detected on a microscale. New biotite and muscovite randomly grew across the old foliation. Quartz is recrystallized and, like plagioclase and K-feldspar, records grain growth. In some parts it displays relics of its former elongate shape within the foliation. Statically heated fabrics are also recorded by triple junctions between straight plagioclase boundaries. No evidence for a younger foliation could be detected, which is also shown by thin and undeformed quartz-plagioclase ± K-feldspar veins cutting across the old fabrics.
The above fabrics in the granodiorites to granites are overprinted by a ductile deformation of quartz (Fig. 11e, f), which also affects older triple junctions between recrystallization grains (undulation, bending, kinking) and led to new dynamically generated recrystallization grains. Magmatic plagioclase grains, however, are bent and partly broken. Older biotite recrystallization grains are bent, kinked and partly replaced by new recrystallization grains. The deformation is also recorded by a crenulation, depicted by polygonal biotite recrystallization, and accompanied by quartz recrystallization. It is related to ductile open folding and bending in some outcrops, however, without formation of a new foliation. It is suggested that this stage of compression took place under temperature conditions of the lower greenschist facies.
Within the xenoliths, the effects of this deformation are recorded only by undulation, bending, kinking and sutured grain boundaries of quartz. Recrystallized and newly grown biotite is partly bent and/or kinked.
4.c. Tonalites of Río Chico, Comallo and Paso Flores
In the Tunnel Tonalite at Río Chico, the anastomosing S1-foliation is not penetrative everywhere. Between foliation planes, relics of coarse magmatic fabrics can be preserved. The S1-planes are depicted by coarse elongate quartz that is bent, kinked and records undulatory extinction and some subgrains. Sutured grain boundaries partly contain dynamically generated recrystallization grains of different sizes. Furthermore, dense chlorite marks S1-planes and is grown there at the expense of former biotite. This replacement of biotite also seems to have taken place during an advanced stage of shearing, because former biotite plates are diffusely converted into chlorite. The S1-planes flow around plagioclase and plagioclase aggregates which are partly bent and/or kinked and do not record recrystallization. To a varying extent they are filled with sericite. Extension veins in broken grains and pressure shadows are filled with quartz and quartz and/or chlorite, respectively. In some parts, S/C-fabrics, also mentioned by Cerredo & López de Luchi (Reference Cerredo and López de Luchi1998), indicate a top-to-the-~N sense of shear.
Old amphibole grains, also affected by S1, are bent, kinked and partly replaced by smaller grains and grain aggregates or actinolitic aggregates (sometimes with chlorite) in pressure shadows. Chlorite partly replaces amphibole from the margins. The above microstructures are similar to those described by, for example, Cerredo & López de Luchi (Reference Cerredo and López de Luchi1997, Reference Cerredo and López de Luchi1998).
The shearing along S1-planes took place under lower greenschist-facies metamorphism (see also Cerredo & López de Luchi, Reference Cerredo and López de Luchi1997). However, coarse elongate quartz with coarse recrystallization grains at the boundaries, also affected by ductile bending, undulation and suturing of grain boundaries, point to a previous stage of shearing under higher temperatures that also led to reorientation of amphibole and probably also the alignment of biotite (?plus recrystallization). Both stages of shearing seem to have operated along the same S1-foliation, because no cross-cutting younger foliation could be detected. This suggests a continuous shearing process under decreasing temperatures. On a microscale, no clear evidence for a magmatic foliation was found.
The cohesive cataclasite layers in the tonalite, cutting across the S1-foliation, are filled with fragments of quartz and quartz aggregates, deformed chlorite sheets with relics of biotite, and some plagioclase and amphibole fragments. The dense matrix consists of randomly grown sericite ± muscovite and cryptocrystalline quartz. Parts are filled with coarse carbonate grains that also fill secondary veins cutting across the older quartz + sericite/muscovite filling. The cataclasite layers mark the final stage of the deformational history under temperatures lower than those during the previous shearing.
In the tonalites east of Comallo and Paso Flores (Fig. 11g and h, respectively; compare also Fig. 2), the first solid-state, partly anastomosing foliation is recorded by ductile elongation of magmatic quartz enhanced between bent and partly broken plagioclase grains. Finest-grained, dynamically generated quartz recrystallization grains mostly occur at sutured grain boundaries. Aligned biotite recrystallization grains replace deformed magmatic grains. S/C-fabrics and asymmetric σ-shapes of pressure shadows at feldspar clasts in the Paso Flores tonalite (Fig. 11h) support the sense of shear found in the field.
Within the xenoliths, the effects of this younger deformation are like those described in Section 4.b.
Based on these observations, the metamorphic overprint during this deformation was in the range of the greenschist facies also supported by the growth of epidote and clinozoisite. It is suggested that it was related to comparable metamorphic conditions during the D3-deformation in parts of the Cushamen Formation rocks plus intercalated granitoids. Cerredo & López de Luchi (Reference Cerredo and López de Luchi1998) also relate the D3-deformation and metamorphic overprint in the Cushamen Formation to those in the Tunnel Tonalite and Mamil Choique granitoids. For the metamorphism of the latter they consider temperatures above 450–500°C, based on local and restricted recrystallization of plagioclase. For the local D4-deformation under brittle conditions, an anchizonal metamorphism can only be assumed. Within the monzogranites south of Mamil Choique, no evidence for a ductile foliation fabric was found.
5. Ages of events
Early Palaeozoic Rb–Sr ages from the Cushamen Formation in the Chubut province of 362±10 and 371±33 Ma have been reported by Ostera et al. (Reference Ostera, Linares, Haller, Cagnoni and López de Luchi2001). The authors refer these ages to the climax of a metamorphic event that was widely distributed in north Patagonia during early–middle Devonian times. These dates point to an early Palaeozoic or greater age for the protoliths of the Cushamen Formation. In view of other published geochronological data (see below in this Section), the possibility of a Devonian age remains for the D1-event plus metamorphism within parts of the Cushamen Formation rocks. In that context it is noteworthy that U–Pb zircon dating, performed by Varela et al. (Reference Varela, Basei, Cingolani, Siga Jr and Passarelli2005) north of Río Limay, gave one Devonian age for a deformed leucogranite, a Carboniferous age for a foliated granite and a Silurian age for a tonalitic orthogneiss. The latter sample yielded an approximate age for titanite of 360 Ma (Varela et al. Reference Varela, Basei, Cingolani, Siga Jr and Passarelli2005), which is comparable with those obtained by Lucassen et al. (Reference Lucassen, Trumbull, Franz, Creixell, Vásquez, Romer and Figueroa2004) from a migmatite (Rb–Sr, internal isochron) and a calc-silicate gneiss (U–Pb, titanite).
The present interpretation of Permian to Triassic ages of the different events in the study area is shown in the chart of Figure 12 and can be summarized as follows:
(1) The D2-deformation and accompanying prograde metamorphism of the Cushamen Formation are interpreted to be early Permian in age, based on a U–Pb dating of zircons from the tonalite northwest of Comallo (south of Loma Miranda, 279±18 Ma: Varela et al. Reference Varela, Basei, Cingolani, Siga Jr and Passarelli2005) that was affected by the penetrative S2-foliation of the country rocks.
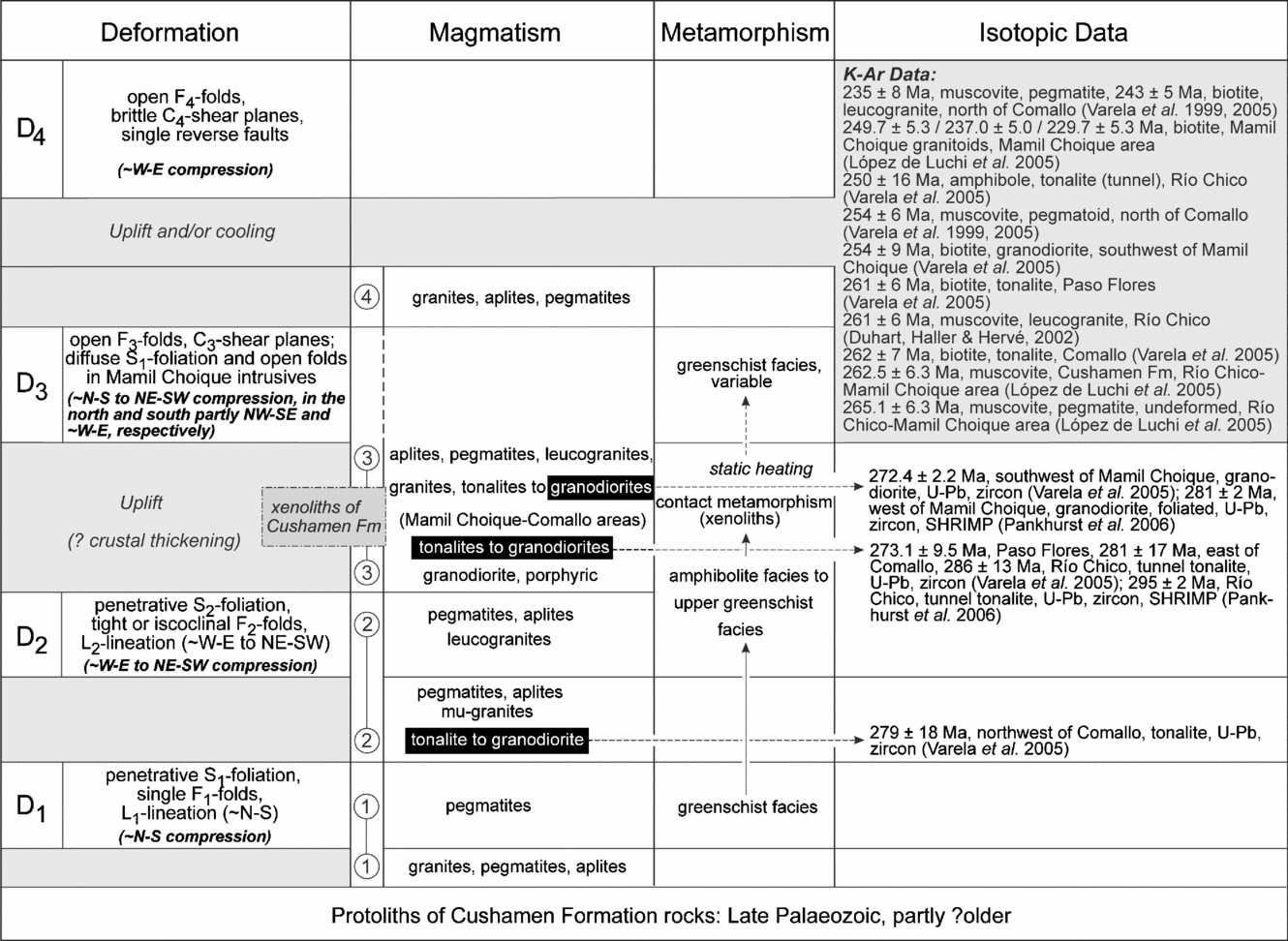
Figure 12. Simplified, schematic and interpretative chart of events within the Paso Flores–Comallo and Río Chico–Mamil Choique areas (western part of the North Patagonian Massif). The deformations and related structures refer to the Cushamen Formation, estimated orientations of compressions also to the deformed intrusions. Published isotopic dates are shown, circled numbers refer to the different stages of intrusive activity described in text. For further explanations, see text.
Here, a pre-late Palaeozoic age of the protoliths of the metasediments plus D1-deformation remains as one possibility and then would also account for the first-stage magmatic intrusions. This interpretation might be supported by a comparison with the Calcatapul Formation in the Gastre area intruded by the Permian Yancamil granite (U–Pb, zircon, 261+16−17 Ma: von Gosen & Loske, Reference Gosen and Loske2004) and afterwards affected by the first compressive deformation under lower greenschist-facies metamorphism (von Gosen & Loske, Reference Gosen and Loske2004). Based on the comparable Permian age of the second deformation plus metamorphism in the Cushamen Formation, the above age for its protoliths can be assumed. Hervé et al. (Reference Hervé, Haller, Duhart and Fanning2005) have shown, however, that at least parts of the Cushamen Formation are late Palaeozoic in age, which excludes the possibility of a greater age of the D1-deformation plus first intrusions.
(2) Based on an early Permian age of the ductile D2-deformation of the Cushamen Formation and intercalated intrusions, the post-D2 intrusions and injections of the third stage of the intrusive activity along with the deformation should be Permian in age or younger (von Gosen, Reference Gosen2005). U–Pb datings of zircons from the tonalites to granodiorites of Paso Flores, east of Comallo and Río Chico (Tunnel Tonalite), performed by Varela et al. (Reference Varela, Basei, Cingolani, Siga Jr and Passarelli2005), yielded 273.1±9.5, 281±17 and 286±13 Ma, respectively (Permian, based on the time scale of Gradstein et al. Reference Gradstein, Ogg, Smith, Bleeker and Lourens2004). For the Tunnel Tonalite, Pankhurst et al. (Reference Pankhurst, Rapela, Fanning and Márquez2006) obtained an age of 295±2 Ma (U–Pb zircon, SHRIMP). The comparable age of the tonalite northwest of Comallo suggests different stages of deformation, magmatism and metamorphism within a short time span during Permian times. This is also shown by the fact that this tonalite was affected by the penetrative S2-foliation of the Cushamen Formation, whereas those of Comallo and Paso Flores contain foliated and angular xenoliths of this formation.
Overall, the above U–Pb zircon ages from different intrusive rocks, summarized in Figure 12, do not permit establishment of a precise chronology within the Early Permian, because they partly overlap within the ranges of error. Furthermore, the precise U–Pb SHRIMP dating of zircons from the Tunnel Tonalite (Pankhurst et al. Reference Pankhurst, Rapela, Fanning and Márquez2006) as part of the third stage of the intrusive activity indicates an early Permian age. This shows that the possibility of a pre-Permian age (?late Carboniferous) for the previous D2-deformation and metamorphism of the Cushamen Formation plus intrusions cannot be ruled out. This also accounts for the D1-event in the Cushamen Formation rocks.
(3) The Permian ages of the above intrusions suggest that most parts of the Mamil Choique granodiorites to granites may also be Permian in age. This is shown by U–Pb zircon ages from a granodiorite southwest of Mamil Choique (272.4±2.2 Ma: Varela et al. Reference Varela, Basei, Cingolani, Siga Jr and Passarelli2005) and a muscovite migmatite west of Mamil Choique (281±2 Ma: Pankhurst et al. Reference Pankhurst, Rapela, Fanning and Márquez2006). Furthermore, enclosed angular xenoliths of the foliated Cushamen Formation suggest a high intrusive level of the Mamil Choique granitoids after cessation of the Permian D2-deformation. The subsequent deformation may be related to the D3-deformation of the Cushamen Formation and affected the intrusions.
(4) K–Ar datings from Loma Carhué (pegmatoid, muscovite) and east of Loma Miranda (pegmatoid, muscovite; foliated leucogranite, biotite) have given ages of 254±6, 235±8 and 243±5 Ma (Varela et al. Reference Varela, Basei, Brito Neves, Sato, Teixeira, Cingolani and Siga1999, Reference Varela, Basei, Cingolani, Siga Jr and Passarelli2005). As heating statically outlasted the ductile D3-deformation of the Cushamen Formation in these areas and equivalent shearing within the youngest leucogranite east of Loma Miranda, deformation was terminated before Triassic times. The dates can be related to a closure of the K–Ar system due to a cooling process that might have been combined with a regional uplift (see Varela et al. Reference Varela, Basei, Brito Neves, Sato, Teixeira, Cingolani and Siga1999). A K–Ar biotite age from an aplite (245±10 Ma: Linares et al. Reference Linares, Cagnoni, Do Campo and Ostera1988) and a muscovite age from an aplitic granite (244±7 Ma: Varela et al. Reference Varela, Basei, Cingolani, Siga Jr and Passarelli2005) north of Río Limay fall in the same time interval.
K–Ar biotite ages from the Comallo and Paso Flores tonalites with 262±7 and 261±6 Ma, respectively (Varela et al. Reference Varela, Basei, Brito Neves, Sato, Teixeira, Cingolani and Siga1999), and a K–Ar muscovite date from a leucogranite within the Cushamen Formation of the Río Chico area (261±6 Ma: Duhart, Haller & Hervé, Reference Duhart, Haller and Hervé2002), however, are slightly older. This also accounts for K–Ar muscovite ages from the Cushamen Formation (262.5±6.3 Ma) and an undeformed pegmatite (265.1±6.3 Ma) in the Río Chico–Mamil Choique area reported by López de Luchi et al. (Reference López de Luchi, Cerredo, Wemmer and Pawling2005) and interpreted as cooling after solid-state deformation and a regional cooling or cooling after intrusion time, respectively. As the Comallo and Paso Flores tonalites do not record a static heating after foliation development, they must have been deformed during decreasing temperature conditions of the greenschist facies. On the contrast, the Tunnel Tonalite of Río Chico yielded a K–Ar age of amphibole of 250±16 Ma (Varela et al. Reference Varela, Basei, Cingolani, Siga Jr and Passarelli2005), which is difficult to interpret. K–Ar biotite ages from foliated Mamil Choique granitoids in the Mamil Choique area, obtained by López de Luchi et al. (Reference López de Luchi, Cerredo, Wemmer and Pawling2005), yielded 249.7±5.3, 237.0±5.0 and 229.7±5.3 Ma, which are interpreted as cooling ages by the authors. The data show that the closure of the K–Ar system in biotite in different areas took place during different times. This is underlined by Carboniferous and Permian K–Ar biotite ages from the Collón Curá area north of Río Limay and Cushamen-Gastre area, respectively, reported by Varela et al. (Reference Varela, Basei, Cingolani, Siga Jr and Passarelli2005). Early Permian and Carboniferous K–Ar biotite and muscovite ages from the Mamil Choique area are reported by López de Luchi et al. (Reference López de Luchi, Ostera, Cerredo, Linares, Haller and Cagnoni1999) and interpreted as indicating a pre-Permian age for the ductile deformation.
(5) The timing of the local D4-event within the Cushamen Formation west of Río Chico is difficult to assess. It can be compared with the D3-event in the occurrence south of the Río Chico–Mamil Choique area described by Franzese, Dias & Dalla Salda (Reference Franzese, Dias and Dalla Salda1992). As these authors pointed out, it is possible to relate these fold structures to similar ones in the Carboniferous Esquel Formation. Furthermore, it is possible to relate them to the Andean ‘Río Chico Thrust’ of Giacosa et al. (Reference Giacosa, Heredia, Césari, Zubia and González1999b).
6. Synthesis and discussion
The structural evolution in the study area began with the D1-deformation affecting the Cushamen Formation clastic rocks and first-stage intrusions. It was followed by the main compressive D2-deformation and continuing prograde greenschist- to amphibolite-facies metamorphism also overprinting the second-stage and older intrusive rocks (Fig. 12). The results from the Loma Carhué area are in accordance with those of Giacosa et al. (Reference Giacosa, Heredia, Césari, Zubia and González1999b) and Giacosa & Heredia (Reference Giacosa and Heredia2004), who relate the shearing to their NE-vergent ‘Carhué ductile shear zone’. Whether the shearing in the present study area was related to limited shear zones is unclear, based on the lack of exposed shear zone boundaries.
The third-stage intrusions (Mamil Choique granodiorites to granites; tonalites of Río Chico, Comallo and Paso Flores) are interpreted as having been emplaced after local uplift (Fig. 12). Except for the Tunnel Tonalite, they contain angular xenoliths of the deformed Cushamen Formation country rocks. This tonalite and the Mamil Choique granitoids have been interpreted as emplaced in a compressional setting shortly after the peak of the regional metamorphism in the Cushamen Formation (Cerredo & López de Luchi, Reference Cerredo and López de Luchi1998; López de Luchi et al. Reference López de Luchi, Ostera, Cerredo, Linares, Haller and Cagnoni1999), which is consistent with the evidence found here. In the interpretation of López de Luchi et al. (Reference López de Luchi, Cerredo, Wemmer and Pawling2005), the Mamil Choique granitoids represent an episode of crustal thickening. The compressive D3-deformation in the study area also affected the third-stage intrusions under greenschist-facies metamorphism. Overall, the successive deformational (D1 to D3) and metamorphic history, accompanied by continuous emplacements of granitic sheets and dykes in the metasedimentary succession along with bigger intrusions, suggests only one single orogenic development, as was also interpreted by Cerredo & López de Luchi (Reference Cerredo and López de Luchi1998). The D2- and D3-deformations are tentatively considered as part of a general collisional evolution by López de Luchi & Cerredo (Reference López de Luchi and Cerredo1996).
Based on the structural analyses, the different stages of broadly estimated compression in the study area are summarized in Figure 13. For the Cushamen Formation, a local ~N–S compression, related to the D1-deformation in the Río Chico area, was followed by ~W–E to SW–NE compression during the D2-deformation in the Comallo area as well (Fig. 13a). WSW–ENE to SW–NE compression during the D1-deformation of the Calcatapul Formation in the southeast (von Gosen & Loske, Reference Gosen and Loske2004) can be compared with that during the D2-event within the Cushamen Formation in the study area. The D3-deformation in the Cushamen Formation is documented by ~NNE–SSW to NW–SE compression in the north and ~W–E compression in the south (Fig. 13c).

Figure 13. Overview maps of the western part of the North Patagonian Massif between Paso Flores and Gastre with broadly estimated orientations of compressions (black and grey arrows). (a, c) D1- to D4-deformations within the Cushamen and Calcatapul formations. (b, d) D1- and D2-deformations within the various intrusions. Relationships between compressions in the intrusions and deformations within the Cushamen Formation country rocks or its xenoliths are shown as symbols; maps are as in Figure 2.
The pattern of estimated compressions in the various granitoids (Fig. 13b, d) shows a comparable picture in which at least two stages can be distinguished, as follows:
(1) The Permian tonalite northwest of Comallo records ~W–E to WSW–ENE compression, which is equivalent to that during the D2-deformation of the Cushamen Formation country rocks (Fig. 13b). This is consistent with that of the granites within the Cushamen Formation affected by the same deformational event (~W–E to NE–SW compression), whereas the local older granites in the Río Chico area also depict ~N–S compression related to the D1-deformation.
(2) The Permian tonalites of Paso Flores, east of Comallo, and Río Chico, along with the (Mamil Choique) granodiorites and granites, show a ~N–S to NE–SW compression during their first deformational stage (Fig. 13d). Their structural relationships with the Cushamen Formation rocks, and enclosed foliated angular xenoliths of the latter, suggest that their deformation took place after the D2-deformation of the Cushamen Formation that had affected the Permian tonalite northwest of Comallo. A comparable ~N–S compression could be estimated for a granodiorite to tonalite of the Laguna del Toro Granitoids in the northern part of the Chubut Province (Fig. 13d). This stage of compression can broadly be assigned to the D3-deformation in parts of the Cushamen Formation. The estimated ~NE–SW compression in granodiorites and/or tonalites in the Mamil Choique area is interpreted as being related to this deformation.
The results show that at least one change in the estimated orientations of compression occurred (not taking into account the D1-deformation). Based on the U–Pb zircon ages from different intrusive rocks, both the ~W–E to SW–NE and ~NE–SW to N–S compression during the first (Fig. 13a, b) and second (Fig. 13c, d) stage, respectively, took place during Permian times.
The causes for this change in the orientation of compression and the differences between the northern and southern parts of the study area are not clear. It is possible that it was related to post-Palaeozoic movements, including those during Andean compression. Strike-slip faulting with block rotations, also reactivating older structures, might have played a role. It is noteworthy that Geuna et al. (Reference Geuna, Somoza, Vizán, Figari and Rinaldi2000) report a 25–30° clockwise rotation of upper Jurassic–lowermost Cretaceous rocks from the Cañadon Asfalto basin, comparable with the results of palaeomagnetic studies from Mesozoic localities in the Deseado Massif (Vizán, Somoza & Taylor, Reference Vizán, Somoza, Taylor, Pankhurst and Veiga2005). This suggests that local rotations in the study area might have modified initial structural orientations, although direct evidence has not been found in the field. Furthermore, it can be assumed that this change was related to a change in relative movements of (parts of) extra-Andean Patagonia (see next Section).
7. Geotectonic implications
In the North Patagonian Massif, structures of upper Palaeozoic compressive deformation occur in scattered outcrops. Therefore, it is unclear whether deformation is intensified towards or restricted to distinct collision zones at its northern and/or southern margin. This also applies to distinct vergencies of structures in these areas, possibly related to continental collision(s).
The new model of Pankhurst et al. (Reference Pankhurst, Rapela, Fanning, Márquez, Pankhurst and Veiga2005, Reference Pankhurst, Rapela, Fanning and Márquez2006) proposes a lower Carboniferous, NE-directed subduction beneath the North Patagonian Massif interpreted as part of Gondwana South America. In their model, the Deseado terrane collided with the autochthonous Gondwana margin during the Carboniferous, shortly after 320 Ma (Pankhurst et al. Reference Pankhurst, Rapela, Fanning, Márquez, Pankhurst and Veiga2005), and the widely distributed upper Palaeozoic intrusions are related to this subduction and slab break-off after collision.
Taking this interpretation, the first- and second-stage intrusions in the study area might be seen as products of an upper Palaeozoic magmatic arc related to ~NE-directed subduction beneath the North Patagonian Massif. The existence of a magmatic arc has been postulated by, for example, Varela et al. (Reference Varela, Basei, Cingolani, Siga Jr and Passarelli2005). The main lower Permian D2-deformation in the study area, indicating ~W–E to NE–SW compression, does not seem to fit with a Carboniferous age of collision along a NW–SE suture zone. However, this deformation might have been the effect of a continuous deformational process in the Permian after initial collision followed by the third deformation. In that context it is possible that the first deformational event in the Cushamen Formation rocks reflects the earlier stage of collision.
Reported evidence from different coastal areas and the Cordillera of Chile has shown that an active plate margin already existed at least during the Late Palaeozoic (e.g. Hervé, Reference Hervé1988; Cingolani et al. Reference Cingolani, Dalla Salda, Hervé, Munizaga, Pankhurst, Parada, Rapela, Harmon and Rapela1991; Pankhurst et al. Reference Pankhurst, Hervé, Rojas and Cembrano1992; Martin et al. Reference Martin, Kato, Rodriguez, Godoy, Duhart, McDonough and Campos1999), although the ages of the deposits are not definitely clear everywhere, and previously expected (upper) Palaeozoic rocks have turned out to be Triassic in age (e.g. Fang et al. Reference Fang, Boucot, Covacevich and Hervé1998; Hervé & Fanning, Reference Hervé and Fanning2001). Hervé (Reference Hervé1988) mentions that upper Palaeozoic granitoids are absent south of 38°S (except a small massif east of Valdivia), and that the upper Palaeozoic magmatic belt trends southeastwards along northern Patagonia, tracing out the old continental margin. In this context, the Liquiñe–Ofqui Fault east of the Coast Range (Fig. 1) might have been responsible for important dextral strike-slip movements (Hervé, Reference Hervé1988). Pankhurst et al. (Reference Pankhurst, Hervé, Rojas and Cembrano1992) propose an initial development of the fault during the Middle Jurassic and suggest that it roughly follows the ancient margin between the Palaeozoic accretionary complex and the pre-existing continental block of Patagonia.
Contrary to the first stage of compression in the study area, the second stage is not compatible with a regime of orthogonal subduction beneath the ~N–S-trending active margin in the west (present coordinates). Although strike-slip tectonics with related block rotations as the possible cause for the differing structural pattern cannot be ruled out, such a compression can be referred to relative ~NE- to ~N-directed movements (or vice versa) of extra-Andean Patagonia. It is important to note that Martin et al. (Reference Martin, Kato, Rodriguez, Godoy, Duhart, McDonough and Campos1999) found a switch in the main pattern of compressive deformation in the Andean foothills and coast ranges of south-central Chile. They proposed that dextral-oblique convergence along this segment of the Gondwana margin initiated during middle Permian times. Therefore, it can be assumed that dextral transpression in that part of the Gondwana margin (Martin et al. Reference Martin, Kato, Rodriguez, Godoy, Duhart, McDonough and Campos1999) also contributed to the ~NE–SW to N–S compression found here. Such an interpretation can also explain the change in compression within the study area.
On a larger scale, both stages of compressive deformation in this western segment of the North Patagonian Massif (Fig. 14) can be seen in conjunction with compressive deformation in its northeastern sector assigned also to the Late Palaeozoic. There, a NW–SE-trending magmatic arc of such an age has been postulated by Ramos (Reference Ramos1984) and Llambías et al. (Reference Llambías, Varela, Basei and Sato2002). It has been interpreted as related to ~SW-directed subduction beneath the northern margin of extra-Andean Patagonia that was followed by collision (Ramos, Reference Ramos1984, Reference Ramos1986). Based also on geophysical evidence of Chernicoff & Zappettini (Reference Chernicoff and Zappettini2004), the present northern boundary has been established to follow the Huincul Fault in the west with a further eastwards continuation along the Río Negro valley (Ramos, Riccardi & Rolleri, Reference Ramos, Riccardi and Rolleri2004; Fig. 14 herein). The estimated ~NE–SW directions of compression in the northeastern segment of extra-Andean Patagonia can be compared with those north of the inferred suture against Gondwana South America (e.g. von Gosen, Reference Gosen2003; Fig. 14 herein) and related to the long Gondwanide fold-and-thrust belt. The NE-directed shearing in the Cerro de Los Viejos granite mylonite (La Pampa province) has been described by Tickyj & Llambías (Reference Tickyj and Llambías1994) and Tickyj et al. (Reference Tickyj, Dimieri, Llambías and Sato1997) and interpreted as the result of collision of Patagonia. Based on studies in the Sierras Australes fold-and-thrust belt north of Patagonia, Sellés Martínez (Reference Sellés Martínez1989) proposed a sinistral transpressive kinematics for the collision, whereas von Gosen, Buggisch & Dimieri (Reference Gosen, Buggisch and Dimieri1990) suggested an initial compression in the Sierras followed by sinistral transpression. It should be noted that Cobbold et al. (Reference Cobbold, Gapais, Rossello, Milani, Szatmari, de Wit and Ransome1992) report Permo-Triassic ~NE–SW contraction in the northeastern part of extra-Andean Patagonia and in the Sierras Australes in the north.

Figure 14. Simplified map of southern South America with position of North Patagonian and Deseado massifs (map after Urien & Zambrano, Reference Urien and Zambrano1996 and Pankhurst et al. Reference Pankhurst, Rapela, Fanning and Márquez2006). Double arrows show the estimated orientations of upper Palaeozoic compressions based on this study, von Gosen (Reference Gosen2003) and references therein. Information from Cerro de los Viejos (La Pampa province) is from Tickyj & Llambías (Reference Tickyj and Llambías1994) and Tickyj et al. (Reference Tickyj, Dimieri, Llambías and Sato1997). Contraction in the segment of the Chilean active margin is related to dextral-oblique convergence that initiated during the Middle Permian (Martin et al. Reference Martin, Kato, Rodriguez, Godoy, Duhart, McDonough and Campos1999). The inferred collision zone south of the North Patagonian Massif (Pankhurst et al. Reference Pankhurst, Rapela, Fanning and Márquez2006) and the present northern margin of Patagonia (Ramos, Riccardi & Rolleri, Reference Ramos, Riccardi and Rolleri2004) are shown.
If the above interpretations turn out to be correct, then Patagonia is bounded by an upper Palaeozoic, ~N–S subduction zone along its western margin and a ~NW–SE collision zone along its northern margin with a comparable age. Patagonia then might be interpreted as a separate plate although its origin and distance from the southwestern Gondwana margin remain unclear. It should be noted that Rapalini (Reference Rapalini, Pankhurst and Veiga2005), based on palaeomagnetic studies, does not rule out a restricted separation of Patagonia orthogonal to its northern boundary in the Early or Middle Palaeozoic and subsequent collision in the Late Palaeozoic.
Including the additional upper Palaeozoic suture zone in the Patagonia area (Pankhurst et al. Reference Pankhurst, Rapela, Fanning, Márquez, Pankhurst and Veiga2005, Reference Pankhurst, Rapela, Fanning and Márquez2006) implies the existence of two crustal pieces that were amalgamated with the southwestern Gondwana margin during late Palaeozoic times (compare also Ramos, Reference Ramos, Pankhurst and Veiga2005). It should be noted that both postulated suture zones at the northern and southern margins of the North Patagonian Massif are not constrained yet by relics of ophiolites and/or ocean-floor deposits and high-pressure metamorphism (see also Ramos, Reference Ramos1984). This also applies to an increase in deformation and metamorphism towards these zones. Furthermore, the existence, locations and fillings of possible, subduction-related back-arc basins are unclear. It can be speculated only that a separation of extra-Andean Patagonia from Gondwana South America was the effect of such a back-arc extension related to subduction in the south. In that context, it is noteworthy that Dalziel & Grunow (Reference Dalziel and Grunow1992) suggested that the Gondwanide orogeny may have been caused by arc–continent collision during closure of an upper Palaeozoic to lower Mesozoic marginal basin mainly floored by stretched continental crust. Trouw & De Wit (Reference Trouw and De Wit1999) proposed that the orogeny resulted from episodic compressive to transpressive deformation in the back-arc region of a wide Andean-type active margin.
In both models of collision at the northern and southern margin of the North Patagonian Massif, however, the suture zones should join the subduction zone at the western Patagonia margin and might have led to a more complex kinematic interplay during upper Palaeozoic movements. As a first step to elucidate the kinematic history, ~W–E to NE–SW and ~NE–SW compressions seem to have been controlled by relative plate movements in the northern and western part of the North Patagonian Massif, respectively, and were followed by ~N–S to NE–SW compression in its western sector.
8. Conclusions
Studies in the western sector of the North Patagonian Massif permit distinction of successive stages of structural and intrusive events as follows:
(1) The first two deformations plus increasing metamorphism in the metasedimentary succession of the Cushamen Formation were accompanied by intrusions of tonalites to granites, pegmatites and aplites. A published isotopic date of a tonalite suggests that the second deformational event was Permian in age, which also accounts for the younger overprints and combined intrusive activity. In view of a published isotopic date, the protoliths of the Cushamen Formation metasedimentary rocks and first deformational overprint should be late Palaeozoic in age. Overall, an onset of the deformational and magmatic history during the Carboniferous cannot be ruled out.
(2) To a varying extent, widely distributed tonalites to granites of a second group of intrusions were affected by compressive deformation that can be related to the third event within the Cushamen Formation country rocks. Enclosed foliated angular xenoliths of the latter suggest emplacements due to brittle fracturing after cessation of penetrative ductile shearing within the country rocks. Deformational structures in the intrusive rocks are interpreted as related to compression during (the final stage of) cooling and a younger ductile overprint.
(3) Various K–Ar biotite ages are interpreted as the effect of cooling due to regional uplift in this part of the North Patagonian Massif during late Permian–Triassic times. Also in the wider surroundings, however, Carboniferous and Permian dates suggest a complex and differential cooling history through time. Minor evidence for a latest-stage folding can roughly be related to that in the Tecka–Tepuel area or at the Andean Río Chico Thrust.
The estimated ~W–E to NE–SW compression during the second (Permian) deformational episode might be related to E-directed subduction beneath the western margin of Patagonia or continuous compression during collision of the Deseado terrane south of the North Patagonian Massif. The younger ~N–S to NE–SW compression, however, is not compatible with a regime of orthogonal subduction at the western Patagonia margin. It might have been related to a change to oblique subduction or continuous collision-related compression within extra-Andean Patagonia. It cannot be excluded that younger block rotations caused its deviating orientation. Like the previous stage of deformation, it probably also had an effect on compression in the northern sector of extra-Andean Patagonia that has been interpreted as the location of collision with the southwestern margin of Gondwana South America. If the suture zone south of the North Patagonian Massif is inserted into this scenario, then Patagonia might have consisted of two different crustal pieces amalgamated with Gondwana South America during Late Palaeozoic times.
Acknowledgements
I am grateful to C. Prozzi and D. Gregori (Bahía Blanca) for all the help during field work in Argentina. Discussions with H. Miller (Munich), V. A. Ramos (Buenos Aires), E. Llambías, A. Sato and P. González (La Plata) improved the knowledge of northern Patagonia. Their help and support are also gratefully acknowledged. Many thanks to J. Ranalli (Mendoza) for his engagement, help and many discussions during field work in Patagonia (2001), and to E. Bouhier (Dirección de Minas, San Antonio Oeste/Río Negro) for his support also in solving logistical problems. I am grateful to P. González, E. Llambías, R. Varela (La Plata), M. López de Luchi (Buenos Aires) and A. P. M. Vaughan (Cambridge) for comments, corrections and suggestions on a previous version of the manuscript. Thanks also to M. López de Luchi and an anonymous reviewer for comments and suggestions that helped improve the present manuscript. The studies were enabled by grants from the German Research Foundation (DFG, Proj. GO 405/4-1 and 4-2), which is gratefully acknowledged. This article is a contribution to IGCP 436.