INTRODUCTION
The global population of land animals slaughtered for human consumption annually amounts to approximately 56 billion. The rising trend in animal breeding is connected with harmful effects on the environment, public health and global development (Koneswaran and Nierenberg, Reference Koneswaran and Nierenberg2008). Infection of domestic animals with the pathogenic protozoan Toxoplasma gondii represents a considerable threat to public health due to food-borne outbreaks leading to heavy economic and health losses worldwide. A great body of epidemiological and experimental evidence has shown that the best way to combat infectious and parasitic diseases, including toxoplasmosis, is effective vaccination.
TOXOPLASMA GONDII AND TOXOPLASMOSIS
The protozoan T. gondii (phylum Apicomplexa) is the etiological agent of toxoplasmosis. Described over 100 years ago, it is the most successful parasite on earth. It is able to infect at a very high prevalence almost all endothermic animal species including humans. The animals from the Felidae family are the only definitive hosts and play an essential role in T. gondii transmission, although the most important reservoir of the parasite is infected farm animals. Sexual reproduction of T. gondii in feline enterocytes results in intensive (upwards 108) shedding of oocysts which become infective 1–5 days after secretion. They can transmit the infection when ingested orally. Cats usually shed oocysts only once in a lifetime and for a limited period of time (a few weeks), although under special conditions (experimental infection with brain homogenate or immunosuppression) repeated oocysts shedding may occur (Elmore et al. Reference Elmore, Jones, Conrad, Patton, Lindsay and Dubey2010). Unlike other apicomplexan parasites, sexual reproduction in cats is not obligatory to complete the T. gondii life cycle and the parasite can be transmitted directly from one intermediate host to another by predation and carnivory (Boothroyd, Reference Boothroyd2009). Intermediate hosts are primarily infected after the ingestion of meat contaminated by tissue cysts full of slow-replicating, ‘dormant’ parasite forms, bradyzoites. Bradyzoites enter the lamina propria in the gut and convert into fast-growing tachyzoites which multiply extensively and disseminate throughout the body. Tachyzoites can penetrate the placenta and cause congenital infection leading to many birth defects (hydrocephalus, chorioretinitis, intracerebral calcifications), abortion or stillbirth. Under pressure from developed cellular immunity of the infected host, parasite dissemination becomes restricted and tachyzoites transform into bradyzoites enclosed in tissue cysts, primarily located in the brain, muscles and eye (Dubey, Reference Dubey, Weiss and Kim2007). Typical and sporadic transmission routes of T. gondii in the farm environment are presented in Fig. 1.

Fig. 1. Transmission routes of T. gondii in the farm environment; a dashed line means sporadic route.
Dependent on the parasite strain and its dose as well as species and immune status of the host, the course of T. gondii infection ranges from asymptomatic in immunocompetent hosts (healthy adults) to severe in immunodeficient hosts. The latter group encompasses both congenitally infected and immunocompromised individuals (e.g. AIDS patients, transplant recipients etc.). Symptomatic disease in immunocompetent humans or animals is rather seldom and can result from invasion with an atypical or highly virulent strain (Demar et al. Reference Demar, Ajzenberg, Maubon, Djossou, Panchoe, Punwasi, Valery, Peneau, Daigre, Aznar, Cotrelle, Terzan, Dardé and Carme2007; Dubey and Prowell, Reference Dubey and Prowell2013). The previous concept of subclinical toxoplasmosis in animals and humans is in the process of changing. Neurotropic affinity of the parasite and its lifelong maintenance in the central nervous system is postulated to influence a variety of host physiological processes, behaviour and mentation (Gatkowska et al. Reference Gatkowska, Wieczorek, Dziadek, Dzitko and Długońska2012; McConkey et al. Reference McConkey, Martin, Bristow and Webster2013). Besides, the oral route of infection may result in many gastrointestinal abnormalities (Prandota, Reference Prandota2012). Toxoplasma manipulates basic host cell physiology, motility and migration, signalling function and apoptotic pathway (Laliberte and Carruthers, Reference Laliberté and Carruthers2008). As recently found, T. gondii injects rhoptry proteins into many cells it does not invade. One of the most exciting findings associated with this biological phenomenon of T. gondii infection is that effector proteins injected by parasite rhoptries manipulate uninfected host cells similarly to those infected with Toxoplasma (Koshy et al. Reference Koshy, Dietrich, Christian, Melehani, Shastri, Hunter and Boothroyd2012).
INNATE AND ACQUIRED IMMUNITY TO T. GONDII
After crossing the epithelium of the small intestine, tachyzoites invade dendritic cells and Toll-like receptor (TLR) activation in dendritic cells plays a key role in the shaping of innate immunity. The prominent cytokine IL-12, produced by many accessory cells (inflammatory monocytes, macrophages, neutrophils and dendritic cells), stimulates natural killer cells (NK) to produce IFN-γ, a major mediator of T. gondii resistance which triggers potent parasiticidal activity of macrophages. Activated macrophages kill intracellular parasites. Toxoplasma gondii-infected dendritic cells assume a hypermotile phenotype and are used as a ‘Trojan horse’ to disseminate throughout the host body but this mechanism also helps to rapidly induce a systemic, specific anti-toxoplasmic immunity (Miller et al. Reference Miller, Boulter, Ikin and Smith2009; Dupont et al. Reference Dupont, Christian and Hunter2012). The role of neutrophils in protection against T. gondii is still controversial, although recently published results show that neutrophils release extracellular traps (NETs) which directly contribute to killing and protecting host cells from the parasite invasion (Abi Abdallah et al. Reference Abi Abdallah, Lin, Ball, King, Duhamel and Denkers2012).
An integrated response of CD4+, CD8+ and B cells is a necessary condition to provide specific and effective long-term immunity against T. gondii infection. During the early stages of infection CD4+ cells contribute to an optimal B and CD8+ T cell response, production of pivotal IFN-γ and expression of CD40 ligand which enables transmission of co-stimulatory signal from accessory CD40+ cells. Specific antigen-presenting cells (APCs, e.g. dendritic cells, macrophages and B cells) acquire parasite antigens by phagocytic or invasion pathway and then present them in a MHC II antigens context. A CD8+ T cell response is initiated when CD8+ naïve T lymphocytes recognize their cognate antigen in the context of MHC I, accompanied by co-stimulatory and cytokine signals. CD4+ T cells are necessary to promote an optimal B lymphocytes response and production of specific T. gondii antibodies, whereas effector CD8+ T cells, activated by CD4+ helper cells, become capable of destroying host cells infected with the parasite. Specific antibodies are essential for resistance, although their action is restricted only to extracellular parasites, when they lyse their host cell or before they invade a new host cell. Antibodies can block parasite invasion, opsonize parasites to facilitate their phagocytosis and activate classical complement pathway (Munoz et al. Reference Munoz, Liesenfeld and Heimesaat2011; Dupont et al. Reference Dupont, Christian and Hunter2012).
VACCINATION TARGETS AND VACCINE CONSTRUCTION
Economic losses associated with T. gondii infection in farm animals, the risk of transmission of the parasite to animals and humans, unsatisfactory chemotherapy associated with increasing drug resistance and drug residues entering the food chain justify current attempts to develop an effective prophylactic T. gondii vaccine for both humans and animals.
The premise of an effective vaccine construction against T. gondii, an obligatory intracellular pathogen, is stimulation of both main effector arms of the host immune response, cell-mediated and humoral immunity. An ideal universal vaccine would be able to protect all potential host species against all T. gondii strains and may require multiple components derived from different parasite strains and more than one life-cycle stage. It poses an extremely difficult challenge for a successful solution. For instance, the ability to induce the prominent protective cytokine, IL-12, was found only in type II parasite strains (Robben et al. Reference Robben, Mordue, Truscott, Takeda, Akira and Sibley2004). Furthermore, the protective immune mechanisms very significantly among host species, e.g. despite the crucial role of TLR11 in induction of IL-12 in mice, the receptor is non-functional in humans (Yarovinsky, Reference Yarovinsky2008). In addition, killing of T. gondii in mice depends on immunity-related GTPases (IRGs), induced by IFN-γ and sequentially loaded on parasitophorous vacuole membrane, which lead to its disintegration and release of aggressive tachyzoites. The IRGs, highly expanded in mice, are absent in humans (Howard et al. Reference Howard, Hunn and Steinfeldt2011). In the light of previous experience in T. gondii vaccine development, it seems that the construction of one universal vaccine for different host species is impossible. Instead of a universal vaccine, a range of different vaccines needs to be prepared to accomplish particular aims, as shown regarding farm animals in Fig. 2.

Fig. 2. Immunoprevention of toxoplasmosis in farm animals – main vaccination goals.
The most commonly used veterinary vaccines against parasitic diseases contain whole attenuated or inactivated parasites and were developed empirically. The earliest trials to construct a prophylactic vaccine against toxoplasmosis started almost 60 years ago and were performed with live virulent (non-persistent) or attenuated tachyzoites, killed tachyzoites, their lysates or soluble cell fractions (conventional vaccines). In the last 20 years, numerous new generation vaccine preparations have been developed and tested, for example naked DNA or RNA, recombinant antigens (single, combined or chimeric), synthetic peptides and non-pathogenic live vectors bearing selected parasite genes (as discussed, among others, by Schaap et al. Reference Schaap, Vermeulen, Roberts, Alexander, Weiss and Kim2007; Jongert et al. Reference Jongert, Roberts, Gargano, Förster-Waldi and Petersen2009; Kur et al. Reference Kur, Holec-Gąsior and Hiszczyńska-Sawicka2009; Liu et al. Reference Liu, Singla and Zhou2012; Zhang et al. Reference Zhang, Chen, Wang, Petersen and Zhu2013). The antigenic preparations, supplemented or not with potent adjuvants, inducing Th1-mediated immunity preferentially, were usually evaluated on a widely available and accepted experimental murine model (Mus musculus), which offers a variety of inbred strains with genetically determined susceptibility to T. gondii infections (Munoz et al. Reference Munoz, Liesenfeld and Heimesaat2011). The number of experimental vaccination trials in other animals is relatively limited so far.
At present, a new, rational approach to vaccine design is being developed, starting from an analysis of a parasite genome sequence to identify potential antigenic components (reverse vaccinology). Generally, construction of a designer vaccine for farm animals involves scientists of many disciplines and veterinary medicine experts and usually takes over 10 years. Immunoinformatics is capable of reducing the time and financial cost of a basic study during the vaccine development process (Patronov and Doytchinova, Reference Patronov and Doytchinova2012). The process of vaccine development comprises defining a potentially immunoprotective antigen (epitope), in relation to in vivo-expressed genes and clonal variability, delivery system and adjuvant (Rueckert and Guzmán, Reference Rueckert and Guzmán2012). To identify antigens implicated in human B cell responses, a lambda phage display library of T. gondii cDNA fragments was screened with sera of infected individuals and in this way several B cell epitopes were found in many parasite antigens like SAG1, GRA1, GRA7, GRA8 and MIC5 (Tonelli et al. Reference Tonelli, Colli and Alves2013). Bioinformatics analysis has also allowed for identification of several promising B and Th1 lymphocyte epitopes of T. gondii aspartic protease (TgAsp1) for further vaccine study (Zhao et al. Reference Zhao, Zhou, Lu, Meng, Sun, Bai, Han, Wang, Zhou, Cong, Zhao, Zhu and He2013). Despite the complex antigenic structure of T. gondii, CD8+ cell responses are restricted to a few peptide epitopes derived only from a limited number of antigens. Furthermore, these peptides can be ranked and the phenomenon of a ‘selection and ranking’ is termed immunodominance. Blanchard et al. (Reference Blanchard, Gonzales, Schaeffer, Joncker, Cheng, Shastri, Robey and Ahastri2008) identified in secretory GRA6 protein the immunodominant and protective decapeptide HF10 which is presented by the Ld MHC I molecule and recognized by a large CD8+ lymphocyte population. Further studies revealed two other tested peptides (SM9 and If9, derived from GRA4 and ROP7, respectively) as subdominant, poorly immunogenic and unable to protect mice from toxoplasmosis (Feliu et al. Reference Feliu, Vasseur, Grover, Chu, Brown, Wang, Boyle, Robey, Shastri and Blanchard2013). Immunodominance of HF10 was independent of its affinity to MHC I molecule or the frequency of relevant T cell precursors in naive mice but was determined by location of this epitope in the C-terminus of the GRA6 molecule.
Selected trials on T. gondii vaccination in farm animals are described in sections below and briefly summarized in Table 1.
Table 1. Overview of T. gondii vaccination trials in sheep, goats, pigs and cats
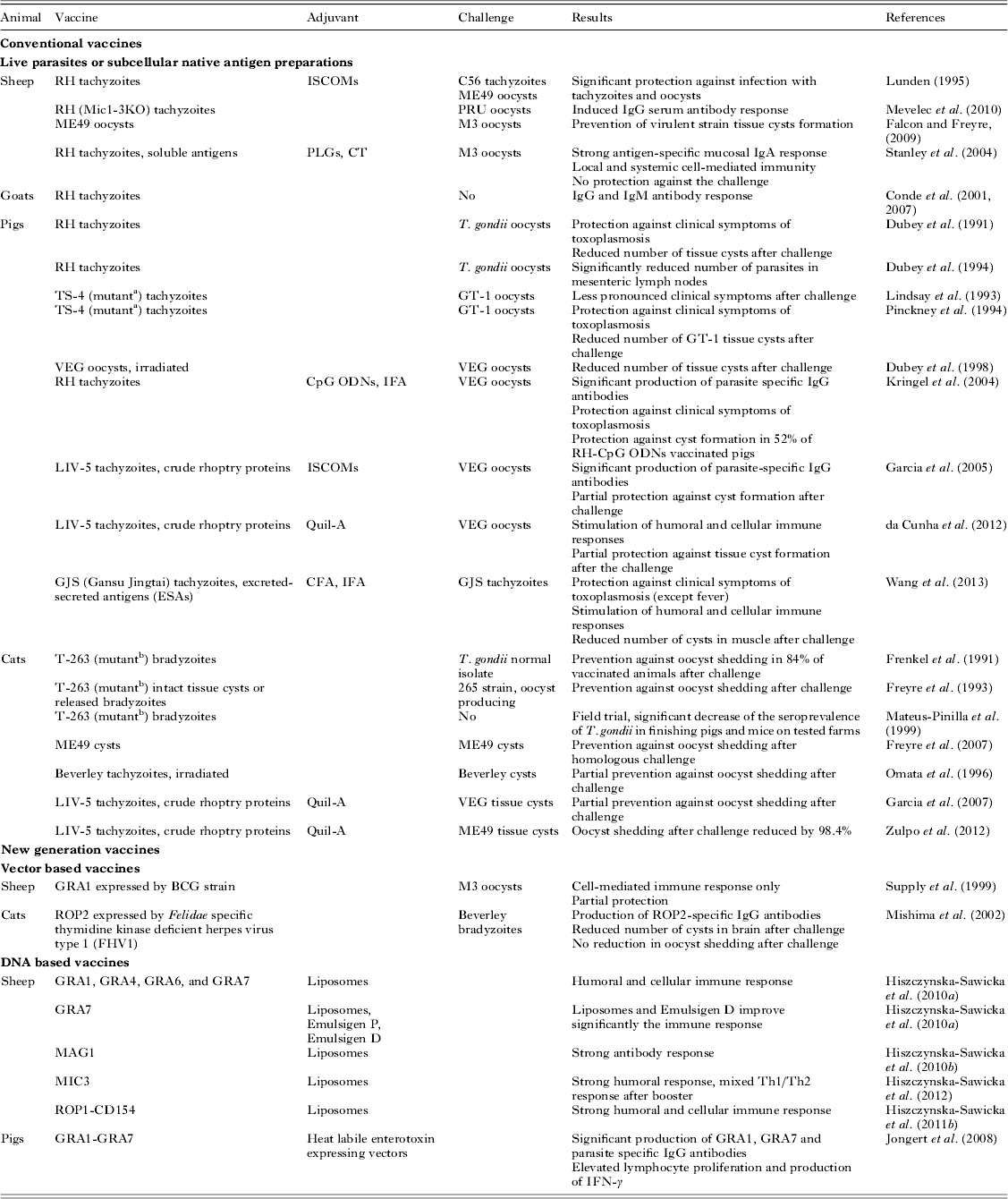
Adjuvants: ISCOMs, immunostimulating complexes; PLGs, poly(lactide-co-glycolide) particles; CT, cholera toxin; ODNs, oligodeoxynucleotides with unmethylated CpG motifs; IFA or CFA, incomplete or complete Freund's adjuvant.
a TS-4 mutant does not form tissue cysts.
b T-263 mutant does not produce oocysts in cats.
VACCINE RESEARCH IN SHEEP AND GOATS
Toxoplasma gondii infection in sheep and goats is very common and has an important economic impact on the agriculture industry in many countries. Ovine toxoplasmosis, which occurs in temperate sheep-rearing countries where the climatic conditions favour oocysts’ survival, is a significant cause of foetal loss in sheep worldwide (Innes et al. Reference Innes, Bartley, Buxton and Katzer2009a ). Past estimates of Toxoplasma infection in sheep worldwide suggest variable infection levels among ewe populations by serology. Infection rate varies from 4 to 96%, in naturally exposed sheep, depending on location and serological test used (Dubey, Reference Dubey2009). Infection in sheep and goats is a major cause of reproductive failure (early embryonic death and desorption, mummification, stillbirths, neonatal death or birth of alive but weak lambs) (Buxton et al. Reference Buxton, Maley, Wright, Rodger, Bartley and Innes2007). Clinical toxoplasmosis is responsible for 1–2% of neonatal losses per annum in the UK (Buxton et al. Reference Buxton, Maley, Wright, Rodger, Bartley and Innes2007) and it was estimated that in the European Union the loss would be between 0·7 and 1·4 million annually (Katzer et al. Reference Katzer, Bruelisauer, Collantes-Fernández, Bartley, Burrells, Gunn, Maley, Cousens and Innes2011). Animals infected with T. gondii pose a risk for human infection due to the consumption of infected meat and milk (Dubey, Reference Dubey2009; Dubey et al. Reference Dubey, Rajendran, Ferreira, Martins, Kwok, Hill, Villena, Zhou, Su and Jones2011).
Although transmission of T. gondii from the consumption of infected meat products is difficult to quantify, Toxoplasma is recognized as a food-borne risk and toxoplasmosis is regarded as a significant public health issue in many countries. In the USA, it is estimated that more than 1 million people are infected with T. gondii and approximately 5000 people develop symptomatic ocular disease annually (Jones and Holland, Reference Jones and Holland2010). The seroprevalence of Toxoplasma in sheep meat ranges from 3 to 68%, based on studies from several countries (Falcon and Freyre, Reference Falcon and Freyre2009). In the USA, seroprevalence of T. gondii in goats and sheep can be as high as 53 and 65% respectively, depending on the region of origin (Hill and Dubey, Reference Hill and Dubey2013). In Norway, the prevalence of T. gondii infection in goats was established at 17% (Stormoen et al. Reference Stormoen, Tharaldsen and Hopp2012). Similarly, sheep in Greece showed a higher seroprevalence (48·6%) than goats (30·7%) but no statistical differences, depending on region, were observed (Tzanidakis et al. Reference Tzanidakis, Maksimov, Conraths, Kiossis, Brozos, Sotiraki and Schares2012).
Reducing T. gondii contamination of meat by the meat industry is critical to prevent food-borne transmission of T. gondii to humans. Prophylactic immunization would be the most efficient way of reaching sterile immunity. The role of a vaccine against T. gondii would be to prevent parasitaemia and colonization of placenta and ideally, prevent formation of tissue cysts which are a source of infection to humans (Menzies, Reference Menzies2012).
Sheep vaccines
Live vaccine
The existing attenuated live vaccine, Toxovax™ (Schering-Plough Animal Health Ltd, New Zealand) was designed to limit the incidence of abortion in sheep related to T. gondii. It results in a decrease of abortion but does not completely eradicate the parasite (Buxton and Innes, Reference Buxton and Innes1995). The vaccine strain (S48) was originally isolated in New Zealand from an aborted lamb and after over 3000 passages in mice it has lost the ability to form tissue cysts or oocysts (Buxton et al. Reference Buxton, Thomson, Maley, Wright and Bos1991; Buxton and Innes, Reference Buxton and Innes1995). Following subcutaneous inoculation of naïve sheep (seronegative for T. gondii) with Toxovax™, the parasite multiplies in the local draining lymph node, causing a mild febrile response with peak titres of antibody reached by 6 weeks. The immunity induced by this vaccine is likely to involve both CD4+ and CD8+ T cells and IFN-γ (Montoya and Liesenfeld, Reference Montoya and Liesenfeld2004). Vaccination induces immunity that protects against abortion for at least 18 months after the initial vaccination (O'Connell et al. Reference O'Connell, Wilkins and Te Punga1988; Wilkins et al. Reference Wilkins, O'Connell and Te Punga1988; Buxton et al. Reference Buxton, Thomson, Maley, Wright and Bos1993). This vaccine is licensed for veterinary use only, is administered prior to mating and is effective after a single shot. However, vaccination needs to be repeated after 2 years.
Despite the existence of a live vaccine, the search for a non-infectious alternative is still in progress. The development of a vaccine for T. gondii needs to take into consideration several different targets. These include vaccine against congenital toxoplasmosis and vaccine against tissue cyst development in food animals such as sheep and goats. In the last two decades significant progress has been made in the identification of vaccine candidates that can successfully induce a protective immune response. The subcellular components of the parasite, such as proteins purified from parasite extracts, recombinant antigens or naked T. gondii DNA (Kur et al. Reference Kur, Holec-Gąsior and Hiszczyńska-Sawicka2009) or RNA (Dimier-Poisson et al. Reference Dimier-Poisson, Aline, Bout and Mevelec2006) were extensively tested in different animal models. Regardless of what kind of vaccine candidates will be used, each of them should induce protective cellular Th1 and humoral Th2 responses as well, both at the level of intestinal mucosa (local) and whole organism (systemic). Often, these new generation subunit vaccines require an addition of potent adjuvants enhancing the intensity of the immune response and inducing the desired Th1 profile.
Tachyzoite-based vaccines
Different vaccine candidates and different approaches have been investigated in sheep as a research model in recent decades. The killed vaccine, which contained extracts of tachyzoites, was not as successful as a live vaccine. Tachyzoites of T. gondii RH strain were used for the preparation of ISCOMs (immunostimulating complexes). Sheep injected subcutaneously on the neck produced low levels of both IgM and IgA after the first injection and then a high level of IgG but no IgM after the booster. After infection with sporulated oocysts a further increase in IgG was observed (Lunden, Reference Lunden1995).
Stanley et al. (Reference Stanley, Buxton, Innes and Huntley2004) used a crude extract of T. gondii tachyzoites encapsulated into PLG micro- and nanoparticles in a mucosal immunization strategy in sheep. Animals were immunized intranasally and then challenged orally with sporulated oocysts. It was found that mucosal immunization induced both systemic and cell-mediated immunity and strong antigen-specific mucosal IgA. However, no protection against the challenge was observed.
Also, an attenuated T. gondii strain was evaluated as a vaccine to prevent the infection of meat animals with Toxoplasma. Lambs immunized with oocysts of the relatively avirulent ME-49 strain of T. gondii, and then challenged with a virulent M3 strain, had developed complete prevention of the formation of virulent M3 cysts in lamb muscle (Falcon and Freyre, Reference Falcon and Freyre2009). In contrast, lambs that had not been immunized with an avirulent strain prior to the challenge developed muscle cysts of a virulent strain.
Another study assessed the effectiveness of a mutant RH strain lacking the mic1 and mic3 genes (Mevelec et al. Reference Mevelec, Ducournau, Bassuny Ismael, Olivier, Seche, Lebrun, Bout and Dimier-Poisson2010). Ewes inoculated subcutaneously or intraperitoneally, 2 months before mating, with Mic1-3KO tachyzoites developed a mild febrile response and serum IgG antibodies, which persisted throughout the experiment. Pregnant ewes were challenged orally with sporulated oocysts of a PRU strain at mid-gestation. All unvaccinated ewes aborted, whereas 62, 91 and 64% of the lambs from vaccinated ewes were viable (tested on Bizet, Romanov and Sologonot ewes, respectively) (Mevelec et al. Reference Mevelec, Ducournau, Bassuny Ismael, Olivier, Seche, Lebrun, Bout and Dimier-Poisson2010). Moreover, subcutaneous vaccination with Mic1-3KO tachyzoites showed potential for reduction in the development of tissue cysts in lambs born to vaccinated ewes. These results are encouraging in respect to the prevention of parasite transmission from food animals to humans.
Vector-based vaccines
In recent decades there has been considerable research interest in identification of suitable subcellular vaccine candidates that can induce a protective immune response.
One of the approaches was construction and development of a BCG strain producing GRA1 antigen, one of the major excreted/secreted antigens of T. gondii (Supply et al. Reference Supply, Sutton, Coughlan, Bilo, Saman, Trees, Cesbron Delauw and Locht1999). Sheep immunized subcutaneously and boosted intravenously with the GRA1-producing BCG strain, developed GRA1-specific cell-mediated responses. However, it failed to elicit a GRA1-specific antibody response. The challenge with the oocysts induced shorter pyrexia in sheep immunized with recombinant BCG. It suggests that, in comparison to the controls, the animals immunized with the GRA1- producing BCG mounted a more rapid response, leading to a shorter period of parasitaemia, suggesting some degree of protection generated by vaccination (Supply et al. Reference Supply, Sutton, Coughlan, Bilo, Saman, Trees, Cesbron Delauw and Locht1999).
DNA-based vaccines
Since the initial demonstration that injection of naked DNA into mammalian muscles can result in expression of the encoded protein, extensive studies have been carried out on DNA vaccines development (Wolff and Budker, Reference Wolff and Budker2005). One of the advantages of these types of vaccines is the ease of their construction, preparation and administration. Primarily, DNA vaccines can be used to generate either a strong Th1 or Th2 response. This finding makes them ideal for use against parasitic infection that often requires specifically tailored immune responses in order to confer protection (Crampton and Vanniasinkam, Reference Crampton and Vanniasinkam2007). DNA vaccine technology allows for the incorporation of different genes of the parasite, starting from those involved in the adhesion/invasion process and ending with those involved in parasite development.
Latest progress in technology has allowed the identification of many genes of T. gondii that can be used for a potential DNA vaccine. Furthermore, a DNA vaccine can be developed based upon antigens expressed during different stages of parasitic infection (acute and chronic).
Although DNA immunization has been proven to be very successful in inducing an immune response in small animals like mice and hamsters, the induction of immunity in large animals such as sheep and goats has been less successful.
Recently, it was shown by Hiszczynska-Sawicka et al. (Reference Hiszczynska-Sawicka, Li, Xu, Oledzka, Kur, Bickerstaffe and Stankiewicz2010a , Reference Hiszczynska-Sawicka, Akhtar, Kay, Holec-Gasior, Bickerstaffe, Kur and Stankiewicz b , Reference Hiszczynska-Sawicka, Oledzka, Holec-Gasior, Li, Xu, Sedcole, Kur, Bickerstaffe and Stankiewicz2011a , Reference Hiszczynska-Sawicka, Li, Xu, Holec-Gasior, Kur, Sedcole, Bickerstaffe and Stankiewicz b , Reference Hiszczynska-Sawicka, Li, Boyu Xu, Akhtar, Holec-Gasior, Kur, Bickerstaffe and Stankiewicz2012) that DNA vaccines encoding different Toxoplasma antigens are able to elicit an immune response in sheep. A DNA vaccine encoding GRA7 antigen was tested in sheep with different adjuvant formulations: liposomes, Emulsigen P and Emulsigen D. The liposomes- and Emulsigen D-formulated GRA7 vaccines seem to improve significantly the immune response in sheep (Hiszczynska-Sawicka et al. Reference Hiszczynska-Sawicka, Li, Xu, Oledzka, Kur, Bickerstaffe and Stankiewicz2010a ). Additionally, liposomal formulation specifically elicited the desired Th1 immune response.
Also, intramuscular injection of the plasmid DNA encoding four different dense granule antigens (GRA1, GRA4, GRA6 and GRA7) formulated into liposomes, elicited humoral and cellular immune responses (Hiszczynska-Sawicka et al. Reference Hiszczynska-Sawicka, Oledzka, Holec-Gasior, Li, Xu, Sedcole, Kur, Bickerstaffe and Stankiewicz2011a ). Among those four GRA antigens, injection of GRA7-encoding plasmid DNA resulted in the most effective stimulation of an immune response, demonstrated by a strong IFN-γ and antibodies response.
MAG1 antigen, which is expressed in the T. gondii tissue cysts’ matrix and wall as well as MIC3 antigen, microneme protein 3 – a potential adhesion molecule, were also evaluated as vaccine candidates (Hiszczynska-Sawicka et al. Reference Hiszczynska-Sawicka, Akhtar, Kay, Holec-Gasior, Bickerstaffe, Kur and Stankiewicz2010b , Reference Hiszczynska-Sawicka, Li, Boyu Xu, Akhtar, Holec-Gasior, Kur, Bickerstaffe and Stankiewicz2012). It was reported previously that MAG1 induces specific B and T cell responses during acute and chronic stages of infection in humans (Di Cristina et al. Reference Di Cristina, Del Porto, Buffolano, Beghetto, Spadoni, Guglietta, Piccolella, Felici and Gargano2004). However, injection of sheep with the plasmid encoding MAG1 induced mainly a strong antibody response with the cellular response detected only a short time after injection. Injection of the MIC3 plasmid vaccine elicited a strong anti-MIC3 antibody response and a mixed Th1/Th2 response after the booster injection.
A major challenge in the development of novel vaccines is the selection of relevant T. gondii antigens and their presentation to a host immune system in such a manner that they induce a strong and long-lasting protective immunity in animals. Beside the antigens participating in the process of adhesion, like MIC3, also under consideration have been antigens involved in formation of parasitophorous vacuoles, such as a rhoptry protein 1 (ROP1). The ROP1 is secreted into the parasitophorous vacuole and rapidly disappears, indicating that the protein has a role in the initial stages of invasion. Sheep injected with plasmids encoding ROP1 antigen and a ROP1-CD154 chimeric protein developed humoral and cellular immune responses with significant enhancement of response after vaccination with the chimeric protein (Hiszczynska-Sawicka et al. Reference Hiszczynska-Sawicka, Li, Xu, Holec-Gasior, Kur, Sedcole, Bickerstaffe and Stankiewicz2011b ). Co-expression of CD154 probably resulted in enhanced antigen processing and presentation, and consequently the immune response was more rapid than after vaccination with ROP1 antigen only. In research by Li et al. (Reference Li, Oledzka, McFarlane, Spellerberg, Smith, Gelder, Kur and Stankiewicz2010), sheep also responded strongly, when vaccinated with plasmid encoding ROP1. However, immune responses induced by DNA vaccines have not yet been evaluated for their ability to provide a protective effect against abortion in pregnant ewes.
Goat vaccines
To date, goats have not been extensively used as an animal model in anti-Toxoplasma vaccine design and development. Conde et al. (Reference Conde, Caballero, Rodriguez-Ponce, Ruiz and Gonzalez2001, Reference Conde de Felipe, Molina, Rodriguez-Ponce, Ruiz and Gonzalez2007) evaluated IgG and IgM response of goats experimentally infected with T. gondii RH strain by subcutaneous puncture. Detected specific IgG antibodies reacted with the whole range of peptides with the highest reactivity observed with the 30 kDa fraction (Conde et al. Reference Conde, Caballero, Rodriguez-Ponce, Ruiz and Gonzalez2001). In another work of Conde de Felipe et al. (Reference Conde de Felipe, Molina, Rodriguez-Ponce, Ruiz and Gonzalez2007), goats inoculated with tachyzoites of RH strain produced detectable IgM- and IgG-specific antibody responses. Detected IgM and IgG specifically reacted with an electroeluted fraction of 29–35 kDa parasite antigens.
In summary, an effective prophylactic vaccine against toxoplasmosis in sheep and goats, especially a new generation one, is still very desirable, and both laboratory and field studies are currently in progress.
VACCINE STUDIES IN OTHER FARM ANIMALS
Since T. gondii can essentially infect all warm-blooded animals and persist life-long in their tissues, undercooked meat and raw meat products have been recognized as two of the main sources of human invasion. Animal species farmed for consumption, including pigs, sheep and goats, are mainly held responsible for parasite transmission to humans (sheep and goats are discussed in the previous section) (Dubey, Reference Dubey1996; Kur et al. Reference Kur, Holec-Gąsior and Hiszczyńska-Sawicka2009).
Pork is the world's most widely eaten meat. As potential intermediate hosts for T. gondii, pigs are susceptible to invasion and the parasite may even cause mortality in neonatal animals. Pigs become infected through the ingestion of environmentally stable oocysts or ingestion of parasite-containing tissues of animals. However, transplacental transmission has also been reported. Although pork has been identified as the main source of parasite cysts, as the seroprevalence in poorly managed non-confinement systems can be as high as 68%, indoors breeding and good management of facilities have resulted in a great reduction of toxoplasmosis in pigs. Nevertheless, the recent demand of consumers for organic farming products may result in increased transmission of the parasite to pigs. It should be noted here that detection of a current infection in pigs requires a mouse bioassay test as the presence of antibodies or DNA is only evidence of previous exposure to the parasite. Unfortunately for consumers, pigs intended for consumption are not obligatorily tested for T. gondii infection in any country (Dubey and Jones, Reference Dubey and Jones2008; Dubey, Reference Dubey2009; Kijlstra and Jongert, Reference Kijlstra and Jongert2009; Hill and Dubey, Reference Hill and Dubey2013). Additionally, anti-toxoplasmic drugs are ineffective in parasite cyst eradication (Kijlstra and Jongert, Reference Kijlstra and Jongert2009) and may persist in meat or milk (Jenkins, Reference Jenkins2001). Taking into account a good immunological response of pigs to Toxoplasma invasion (Dubey, Reference Dubey2009) it is apparent why most vaccines research has been performed using the porcine experimental model.
First attempts at creating an effective vaccine involved live parasites of the highly virulent RH strain of T. gondii. The experiments were based on the observations that the RH strain does not persist in tissues of infected pigs, which made it a good candidate for a live vaccine. In 1991, Dubey et al. (Reference Dubey, Urban and Davis1991) observed that pigs inoculated subcutaneously with RH tachyzoites, after acute infection accompanied by fever, cleared the parasite from their tissues as proved by mouse bioassay. Further tests revealed that initial intramuscular vaccination with RH tachyzoites protects pigs from developing clinical symptoms of toxoplasmosis after subsequent challenge with oocysts. Even though RH vaccination resulted in fewer tissue cyst numbers compared with uninoculated control pigs, it failed at the complete prevention of cyst formation. Another study revealed that RH-vaccinated pigs, challenged with a lethal dose of T. gondii oocysts, had significantly fewer parasites in mesenteric lymph nodes compared with non-vaccinated controls, as measured 3 days after oocyst inoculation (Dubey et al. Reference Dubey, Baker, Davis, Urban and Shen1994). A slightly different approach involved live parasites, a non-virulent strain or a strain incapable of completing its life cycle. An example is the TS-4 strain, a chemically attenuated variant of the RH strain, which is non-pathogenic to mice and pigs (Dubey, Reference Dubey1996). The subcutaneous or intravenous inoculation of weaned pigs with TS-4 tachyzoites did not produce any clinical signs of infection in animals and no parasites were detected in tissues of these pigs or isolated by bioassay. Furthermore, animals inoculated twice with TS-4 had less pronounced clinical symptoms following the oral challenge with GT-1 oocysts in comparison to controls (Lindsay et al. Reference Lindsay, Blagburn and Dubey1993). Similarly, nursing pigs vaccinated with living TS-4 tachyzoites by intravenous and subcutaneous routes did not develop any symptoms of the disease and the mutant strain was not re-isolated from animal tissues. However, the vaccination did not prevent tissue cyst formation after the oocyst challenge, although pigs vaccinated subcutaneously had fewer cysts in their tissues (Pinckney et al. Reference Pinckney, Lindsay, Blagburn, Boosinger, McLaughlin and Dubey1994). Another attempted strategy was based on an application of oocysts inactivated by irradiation, since it has been demonstrated that they retain their immunogenicity in mice (Dubey et al. Reference Dubey, Lunney, Shen and Kwok1998). Outbred pigs were fed irradiated oocysts of the VEG strain and then challenged orally with active oocysts. Yet again, obtained results indicated that although less numerous, T. gondii cysts were still present in vaccinated animals even after two doses of irradiated oocysts and a subsequent challenge with only 102 active oocysts. Since generation of protective immunity often depends on an appropriate adjuvant, in another study the live vaccine was enriched in synthetic oligodeoxynucleotides (ODNs) containing non-methylated CpG motifs which are related to naturally derived bacterial DNA and are capable of inducing a Th1 immune response (Kringel et al. Reference Kringel, Dubey, Beshah, Hecker and Urban2004). Pigs were vaccinated with a T. gondii RH strain combined with different concentrations of CpG ODNs and then challenged with VEG oocysts. The CpG ODNs vaccinated animals produced significant amounts of parasite-specific IgG antibodies and had no clinical symptoms after the challenge. The most important achievement was that for 52% of RH-CpG ODNs vaccinated pigs no tissue cysts were detected using both mice and cat bioassays.
All the presented studies using a live vaccine clearly show the significant potential of active parasites. However, taking into account their possible pathogenicity, isolated parasite antigens are more often considered for vaccine preparations. The experiments performed by Garcia et al. (Reference Garcia, Gennari, Navarro, Machado, Sinhorini, Freire, Marana, Tsutsui, Contente and Begale2005) aimed at testing the possible usefulness of crude rhoptry proteins of T. gondii incorporated in the ISCOMs administered by the subcutaneous route in protection against toxoplasmosis after VEG oocysts challenge. Partial protection was observed in pigs after the challenge with VEG oocysts. Thus, the vaccine proved to be less efficient compared with live RH tachyzoites. In another experiment, pigs were immunized intranasally with crude rhoptry proteins along with widely used veterinary adjuvant Quil-A (da Cunha et al. Reference da Cunha, Zulpo, Bogado, de Barros, Taroda, Igarashi, Navarro and Garcia2012). The vaccination stimulated humoral and cellular immune responses which resulted in partial protection against cyst formation in the brain.
A fraction of excreted-secreted antigens (ESAs) of the parasite was also tested as the possible vaccine candidate for pigs (Wang et al. Reference Wang, Zhang, Wang, Yin and Wang2013). Animals were injected subcutaneously with ESA emulsified in Freund's adjuvants and then challenged intraperitoneally with T. gondii Gansu Jingtai strain (GJS) tachyzoites. Aside from fever, vaccinated animals were protected from developing other symptoms of parasite infection such as a cough or anorexia, and a significant reduction in the cyst burden in muscles was observed. Experiments that evaluated the usefulness of a DNA vaccine encoding selected parasite antigens GRA1–GRA7 were also tested in pigs (Jongert et al. Reference Jongert, Melkebeek, De Craeye, Dewit, Verhelst and Cox2008). The vaccine cocktail was enhanced by codon optimization of the encoding antigens and addition of heat-labile enterotoxin-expressing vectors as genetic adjuvants. The authors noted that intradermal immunization promotes synthesis of specific antibodies, lymphocyte proliferation and production of IFN-γ, a crucial protective cytokine in T. gondii elimination.
Although vaccine attempts for farm animals are studied mostly on innately susceptible hosts such as pigs or sheep, these are not the only meat-producing species meant for human consumption. Among others most commonly bred are cows, chickens, horses and rabbits.
Cows are generally considered poor hosts for T. gondii and, due to natural resistance, the parasite is eliminated from their tissues following infection or reduced to undetectable levels. For that reason consumption of beef and dairy products is not considered important in Toxoplasma epidemiology (Opsteegh et al. Reference Opsteegh, Teunis, Züchner, Koets, Langelaar and van der Giessen2011; Hill and Dubey, Reference Hill and Dubey2013). Horses are also considered to be resistant to invasion and although Toxoplasma has been isolated from horse meat, the infection threat is limited due to limited consumption of horse meat. Chickens, also resistant to clinical toxoplasmosis, are considered a good indicator of soil contamination with oocysts since they feed from the ground (Dubey, Reference Dubey2010). Chicken meat is considered an efficient source of infection for cats and may also be an important source of infection for humans. However, several reports suggest that processing and handling procedures commonly used prior to sale inactivate parasites and thus the prevalence of viable T. gondii in retail meat is low (Dubey et al. Reference Dubey, Hill, Jones, Hightower, Kirkland, Roberts, Marcet, Lehmann, Vianna, Miska, Sreekumar, Kwok, Shen and Gamble2005). Moreover, viable parasites are rarely isolated from the meat of chickens raised indoors. In contrast, free-range chickens are commonly infected with the parasite. Nevertheless, these are essentially kept for egg production and eggs are not considered an important source of human toxoplasmosis (Dubey, Reference Dubey2010; Hill and Dubey, Reference Hill and Dubey2013). Finally, only sporadic cases of clinical toxoplasmosis have been described in rabbits, which probably more often serve as pets (Dubey and Jones, Reference Dubey and Jones2008).
Dogs kept on farms are worth consideration. Although primary toxoplasmosis in dogs is rare (Dubey and Jones, Reference Dubey and Jones2008), and they are not consumed in most countries, dogs may be associated with a higher T. gondii distribution in the farm environment. It has been proven that sporulated oocysts can retain their infectivity after traversing through the canine intestinal tract and so dogs may be responsible for the mechanical transmission of T. gondii (Lindsay et al. Reference Lindsay, Dubey, Butler and Blagburn1997).
VACCINE STUDIES IN DOMESTIC CATS (FELIS CATUS)
Virtually all meat-producing farm animals are herbivores and as such they primarily become infected by T. gondii through ingestion of parasite oocysts deposited in the environment (Kijlstra and Jongert, Reference Kijlstra and Jongert2009). It has been known that environmentally resistant infective parasite forms are the result of sexual reproduction occurring only in the intestines of felids serving as the parasite's definitive hosts (Dubey et al. Reference Dubey, Miller and Frenkel1970). In reality, a domestic cat, essentially kept on a majority of farms, is the main culprit (Fig. 1). Furthermore, once released to the environment oocysts are practically impossible to remove (Kijlstra and Jongert, Reference Kijlstra and Jongert2009). In that respect, developing a vaccine against T. gondii invasion in cats, to prevent oocysts shedding and environment contamination, would represent another approach to human and meat-producing animals’ protection from toxoplasmosis.
As in the case of other farm animals, the first attempts aimed at creating a vaccine for cats were mostly focused on the use of live parasites. The oocyst-negative mutant of T. gondii RH strain, designated as T-263, was tested for its usefulness in protection against oocysts shedding. The experiment performed on kittens, mostly responsible for disseminating parasite oocysts in the environment, revealed that vaccination with T-263 bradyzoites prevented oocyst excretion in 84% of tested animals after being challenged with a normal parasite strain (Frenkel et al. Reference Frenkel, Pfefferkorn, Smith and Fishback1991). Moreover, two oral doses of a vaccine comprising either intact tissue cysts or released bradyzoites of the T-263 strain induced immunity that effectively prevented cats from oocysts shedding after their infection with the oocyst-producing T-265 strain (Freyre et al. Reference Freyre, Choromanski, Fishback and Popiel1993). Then, taking into account involvement of cats in farm animal exposure to T. gondii oocysts, a large-scale 3-year field study of T-263 strain-based vaccine was conducted on pig farms in Illinois (Mateus-Pinilla et al. Reference Mateus-Pinilla, Dubey, Choromanski and Weigel1999). Cats trapped between 1994 and 1995 were vaccinated orally and farms were visited repeatedly throughout 1994, 1995 and 1996 to collect test samples. The analysis of data revealed a significant decrease of seroprevalence of T. gondii in finishing pigs and mice on all tested farms, which further confirmed the possible application of the T-263 strain in veterinary vaccination of cats. Nevertheless, the extensive computer simulation of the prevention of parasite transmission on pig farms, due to feline T. gondii vaccine (T-263), on one hand supported the field trial results of vaccine effectiveness but at the same time proved that the decrease in the number of cats residing on farms has a much more pronounced impact on reducing the T. gondii infection rate in finishing pigs (Mateus-Pinilla et al. Reference Mateus-Pinilla, Hannon and Weigel2002).
The strains producing oocysts were also considered as potential vaccine candidates. When cats were inoculated with ME49 strain and then treated with sulfamerazine and pyrimethamine, only asexual stages were seen in haematoxylin-eosin stained gut sections. This led to further experiments which showed that using the ME49 strain of T. gondii resulted in complete protection against homologous and high protection against heterologous challenges (Freyre et al. Reference Freyre, Falcón, Méndez, Gastell and Venzal2007). In another study, 60Co-irradiated tachyzoites of the Beverley strain were tested as a vaccine in kittens. The vaccination partially prevented oocyst excretion after the Beverley cysts challenge. On the contrary, 60Co-irradiated tachyzoites of the RH strain had no effect on oocyst shedding following Beverley cyst administration (Omata et al. Reference Omata, Aihara, Kanda, Saito, Igarashi and Suzuki1996). There were also efforts to use defined T. gondii antigens as vaccine components. The felidae specific thymidine kinase deficient herpesvirus type 1 (FHV1) with markedly lowered pathogenicity to cats and expressing ROP2 antigen was used as a vaccine. Although intranasal administration of recombinant virus particles resulted in IgG specific ROP2 response and reduced brain parasite load following the experimental infection with Beverley strain bradyzoites, it failed at preventing oocyst shedding by cats (Mishima et al. Reference Mishima, Xuan, Yokoyama, Igarashi, Fujisaki, Nagasawa and Mikami2002). The latest experiments were based on crude rhoptry proteins adjuvanted with Quil-A to immunize cats by the intranasal route. In the first trial, two out of three cats receiving three doses of the vaccine did not excrete oocysts in their faeces (Garcia et al. Reference Garcia, Navarro, Biazzono, Freire, da Silva Guimarães Junior, Cryssafidis, Bugni, da Cunha, Hamada and Dias2007). In the second trial, where the animals were injected four times and then challenged with the ME49 strain, they produced 98·4% fewer oocysts compared with the unvaccinated group (Zulpo et al. Reference Zulpo, Headley, Biazzono, da Cunha, Igarashi, de Barros, Taroda, Cardim, Bogado, Navarro and Garcia2012).
This review of the attempts at creating an anti-Toxoplasma vaccine underlines the usefulness of live parasites. It has been noted that live vaccines are usually very potent at stimulating protective immune responses in animals. However, several drawbacks remain. Live parasites require cold storage (Booth et al. Reference Booth, James and Popiel1996), have a limited shelf-life and are accompanied by the constant risk of causing morbidity and mortality in vaccinates (Jenkins, Reference Jenkins2001; Innes et al. Reference Innes, Bartley, Maley, Katzer and Buxton2009b ). For that reason, the search for selected T. gondii-specific antigens capable of inducing a strong immune response efficient in parasite clearance from the hosts continues.
FINAL REMARKS
The economic, social and human impact of toxoplasmosis is higher than previously realized. Meat contamination, a major source of T. gondii infection for humans and the cause of great losses in farming, could be eliminated in closed and well-organized animal production systems (factory farms e.g. piggeries) in highly developed countries. By contrast, oocyst contamination of soil, water and food is difficult to control (Kijlstra and Jongert, Reference Kijlstra and Jongert2009).
Widely distributed latency of T. gondii infections in both human and animal populations is a persistent threat for public health because even transient immune depression of the host, induced by many exogenous and endogenous factors (including the still increasing use of immunosuppressive drugs), may result in a reactivation of the latent infection. Taking into account potential distant consequences of T. gondii infection in both animals and humans (e.g. threat of latent infection reactivation, behavioural changes) and unsatisfactory treatment, the development of effective immunoprophylaxis is the optimal solution. Many efforts in the last 20 years have focused on the development of effective immunoprevention against toxoplasmosis. The opportunity of direct synergistic action of a vaccine and chemotherapeutics in the control of toxoplasmosis, as well as other parasitoses, could help to reduce the medicine use (Vercruysse et al. Reference Vercruysse, Schetters, Knox, Willadsen and Clarebout2007).
Thus high prevalence of T. gondii latency is a justified premise for the development of a therapeutic vaccine, aside from a prophylactic one. These attempts require explanation as to why specific T. gondii immunity is not sufficient to eliminate the parasite completely and where the weakest element of the parasite-induced immunity can be found. Specific memory CD8+ lymphocytes are critical for long-term protection against all intracellular pathogens including T. gondii. Whereas the acute phase of toxoplasmosis is characterized by a robust CD8+ response, late chronic toxoplasmosis is associated with a CD8+ exhaustion phenomenon (Bhadra and Khan, Reference Bhadra and Khan2013). CD8+ cells in C57BL/6 mice, which are innately susceptible to toxoplasmosis, exhibit polyfunctionality loss (i.e. single cells progressively fail to produce optimal IFN-γ and TNF-α as well as mediate cytotoxicity), elevated apoptosis accompanied by increased expression of PD-1 marker (Programmed Death) and decreased expression of T-bet and Eomes transcription factors. Restoration of the full activity of CD8+ cells could be a promising supplementary or independent therapeutic method to fight toxoplasmosis (Bhadra et al. Reference Bhadra, Gigley and Khan2011).
The majority of previously licensed, successful vaccines involve microbes that cause acute rather than chronic infections and induce protective humoral immunity, whereas in infections caused by intracellular pathogens such as T. gondii, cell-mediated immune responses, involving NK cells, CD4+ and CD8+ as well as proinflammatory and regulatory cytokines, are major effector mechanisms. They are triggered by live attenuated vaccines which are unsatisfactory even for farm animals (short shelf life, unstable, infectious for non-vaccinated animals and humans). Previous T. gondii vaccine trials on farm animals are not numerous. Most studies have been performed on laboratory mice (Mus musculus) which as natural hosts for Toxoplasma, commonly available and not expensive, are undoubtedly a proper basic model to investigate post vaccination immunity. Unfortunately, immune mechanisms are not only species but also strain-, sex- and individuum-specific (Letscher-Bru et al. Reference Letscher-Bru, Pfaff, Abou-Bacar, Filisetti, Antoni, Villard, Klein and Candolfi2003). Recent studies on T. gondii vaccine construction clearly suggest that single or a few antigens (epitopes) elicit only partial protection against toxoplasmosis. Thus, future trials should take into consideration as many CTL (cytotoxic T lymphocytes) epitopes as express live attenuated vaccine preparations (Zhang et al. Reference Zhang, Chen, Wang, Petersen and Zhu2013). A successful outcome of an effective anti-T. gondii vaccine seems a very difficult challenge. To date, aside from limited Toxovax™ vaccine use, the most effective measures in protecting humans from meat-borne infection comprise treatment of meat products with salt solutions, low or high temperature and gamma irradiation (Dubey, Reference Dubey1996, Reference Dubey2009; Kijlstra and Jongert, Reference Kijlstra and Jongert2009; Hill and Dubey, Reference Hill and Dubey2013), and the prevention of infection with toxoplasmosis of meat-producing animals, involving indoor breeding, hygiene procedures and stray cats control.
FINANCIAL SUPPORT
The article was supported by the Polish Ministry of Science and Higher Education and Polish National Science Centre and prepared during implementation of the projects on T. gondii immunity and experimental vaccines (J.G., grant number N N302 636340; M.G., grant number UMO-2011/03/N/NZ6/04655).
COMPETING INTERESTS
The authors have declared that no competing interests exist.