1. Introduction
Vertebrate remains have been recorded from several upper Silurian formations in Poland (e.g. Märss, Reference Märss1997; Kozłowski, unpub. thesis, 2006, University of Warsaw, 2008; Kozłowski & Munnecke, Reference Kozłowski and Munnecke2010), but only a few of these have been described or illustrated. Here, we report vertebrate microremains from two largely coeval sections in the upper Silurian stratigraphy of the Holy Cross Mountains. The samples come from palaeoenvironments of slightly different energy and are therefore important for understanding the palaeoecological distribution of vertebrate assemblages during this time. The assemblages are also compared to the vertebrate biozonation established for shallow-marine facies in the Silurian deposits of the Baltic Basin in an attempt to determine the detailed age of the host rock. This work functions as a preliminary study of Silurian vertebrate successions of the Łysogóry region and subsequently how the established Silurian vertebrate biozonation compares to this part of the Baltic Basin. The study supports previous suggestions of vertebrate facies dependence, which provides incentives for future investigations of environmental preferences among early vertebrates.
Institutional abbreviation. PMU – Palaeontological Collections, Museum of Evolution, Uppsala University, Uppsala, Sweden.
2. Geological setting
The Silurian succession in the subsurface of the northeastern Poland is reported from several deep drill cores towards the Caledonian and Variscan sutures, which limits its occurrence from the SW. The Holy Cross Mountains in central Poland is the only area in the southern part of the Trans-European Suture Zone with such Silurian outcrops (Kozłowski & Munnecke, Reference Kozłowski and Munnecke2010; Fig. 1). The upper Silurian stratigraphy of the northern (Łysogóry) region (Fig. 2) has been described and lithostratigraphically defined by Kozłowski (Reference Kozłowski2008). It represents the southwestern part of the Baltic Basin, which evolved into a Caledonian foreland during late Silurian time (Jaworowski, Reference Jaworowski1971). The fast infilling of the foredeep allowed for the formation of marginal-marine environments during and just after the first pronounced sea-level fall (Kozłowski, Reference Kozłowski2003), which is coincident with the mid-Ludfordian Lau oceanic event (Kozłowski & Munnecke, Reference Kozłowski and Munnecke2010). This shallow-water succession contains numerous carbonate intercalations that show similarities to Gotland (Sweden) in both facies development and fossil assemblages, but was deposited at the opposite side of the Baltic Basin (Kozłowski, Reference Kozłowski2003; Kozłowski & Munnecke, Reference Kozłowski and Munnecke2010). The carbonate intercalations contain numerous vertebrate microremains that were reported earlier by Kozłowski (e.g. unpub. thesis, 2006, University of Warsaw) and are described here for the first time.
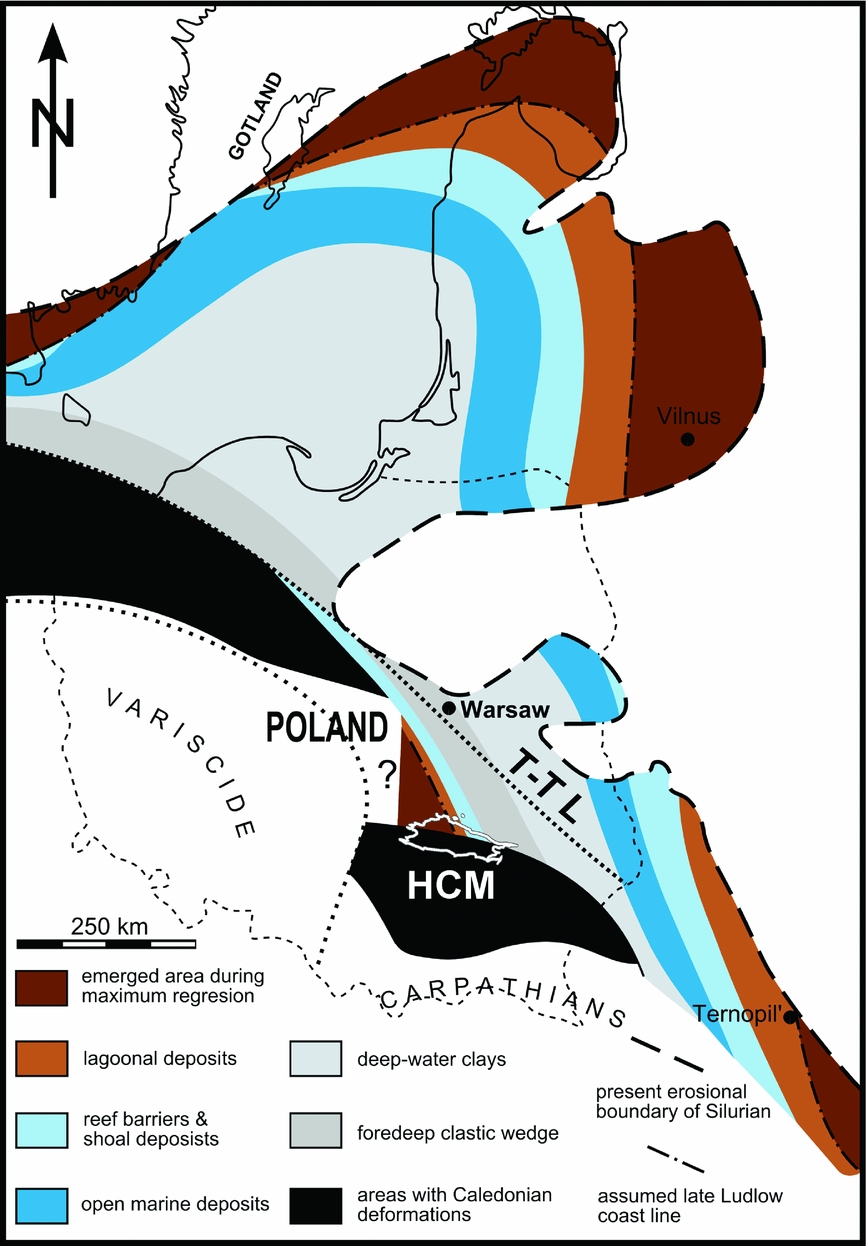
Figure 1. Palaeogeographical map of the Baltic region in the Ludfordian with the Palaeozoic deposits of the Holy Cross Mountains (HCM) highlighted in white (T-T L – Teisseyre-Tornquist Line). Modified from Kozłowski & Munnecke (Reference Kozłowski and Munnecke2010), based on Bassett, Kaljo & Teller, (Reference Bassett, Kaljo, Teller, Holland and Bassett1989), Jaworowski (Reference Jaworowski1971), Tomczykowa (Reference Tomczykowa1988), Paškevičius (Reference Paškevičius1997), Kozłowski (Reference Kozłowski2003) and Kaljo & Martma (Reference Kaljo and Martma2006).
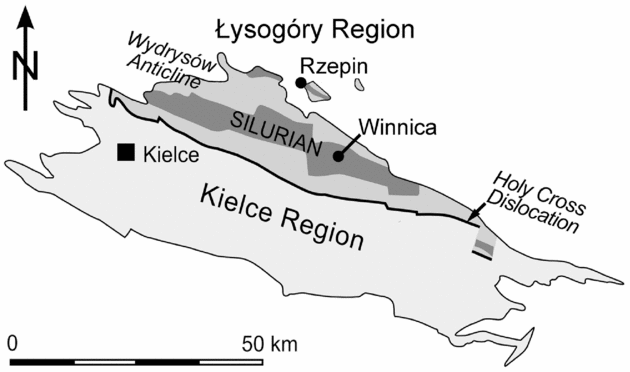
Figure 2. Geological sketch map of the Palaeozoic rocks of the Holy Cross Mountains with the geographical position of the Winnica and Rzepin sections. Modified from Kozłowski & Munnecke (Reference Kozłowski and Munnecke2010), based on Czarnocki (Reference Czarnocki1957).
2.a. Stratigraphical position and age
The sedimentary rocks exposed at both studied sites have been referred to the Lower and Upper Rzepin Beds (Filonowicz, Reference Filonowicz1968), Winnica Mudstone Complex by Bodzioch, Kozłowski & Popławska (Reference Bodzioch, Kozłowski and Popławska2003), and more recently as the Słupianka Member of the Winnica Formation (Kozłowski, Reference Kozłowski2008). The Winnica Formation consists of the Jadowniki, Bełcz and Słupianka members, and has time-equivalent clastic deposits in the Rachtanka Formation (see Kozłowski, Reference Kozłowski2008 and Kozłowski & Munnecke, Reference Kozłowski and Munnecke2010 for details). The studied samples are almost isochronous and represent the upper part of the Słupianka Member. The Winnica samples represent the lower band of the characteristic duple stromatoporoid-tabulate-bearing level, which also occurs 18 m below the sampled level in the Rzepin section.
Fossils useful for biostratigraphical correlations are rare in the Winnica Formation at the Winnica and Rzepin sections (see Kozłowski, Reference Kozłowski2008). The age of the studied interval is limited from the bottom by the occurrence of the mid-Ludfordian carbon isotope excursion (correlated with the Lau Event on Gotland), reported from the Rzepin section by Kozłowski & Munnecke (Reference Kozłowski and Munnecke2010), and has also been noted just below the sampled interval in Winnica (unpubl. data of W. Kozłowski). Based on chemostratigraphical and sequence stratigraphical correlations, the base of the Winnica Formation (Jadowniki oolite) may be correlated with the base of the Eke formation on Gotland, which is supported by an upper Ludlow trilobite assemblage (Kozłowski, Reference Kozłowski2003, Reference Kozłowski2008). According to this correlation, the lower part of the Winnica Formation (Jadowniki oolite member) may be referred to the lower Icriodontid Zone of Jeppsson, Eriksson & Calner (Reference Jeppsson, Eriksson and Calner2006) (= Neocucullograptus kozlowskii – Pseudoclimacis latilobus Interzone). Based on sequence stratigraphy and chemostratigraphy, Kozłowski & Munnecke (Reference Kozłowski and Munnecke2010) roughly correlated the lower 30 m of the Słupianka Member with the Hamra Formation on Gotland and the upper part of Słupianka with the Sundre Formation, as well as younger sediments represented by Beyrichienkalk off the south coast of Gotland. Subsequently, the upper Słupianka Member and the W1c and W1d samples could represent sediments equivalent to the topmost part of the Gotland stratigraphy, while the JB142 sample from the uppermost Słupianka Member may be slightly younger (see fig. 7 in Kozłowski & Munnecke, Reference Kozłowski and Munnecke2010).
The studied interval in both sections contains the first appearance of the trilobite Acastella prima (Kozłowski, Reference Kozłowski2003, Reference Kozłowski2008) as well as an ostracode assemblage (see Malec, Reference Malec2000) that suggest a local Neobeyrichia incerta – Acastella prima Zone (Tomczykowa & Witwicka, Reference Tomczykowa and Witwicka1974; Tomczykowa, Reference Tomczykowa1991). This level may be correlated with the latest Ludfordian, since Neobeyrichia incerta has its first appearance datum slightly above the minor twin peaks of the late Ludfordian carbon isotope excursion, and well above the major mid-Ludfordian carbon isotope excursion in the East Baltic (Kaljo et al. Reference Kaljo, Einasto, Martma, Märss, Nestor and Viira2015, fig. 3). The deposits occurring above the Winnica Formation (Bronkowice and Sarnia Zwola formations) contain faunas indicative of Pridoli deposits (Tomczykowa, Reference Tomczykowa1991; Bodzioch, Kozłowski & Popławska, Reference Bodzioch, Kozłowski and Popławska2003; Kozłowski, Reference Kozłowski2008). The stratigraphical data therefore delimit a latest Ludlow – earliest Pridoli age for the fish remains from the Winnica and Rzepin sites.
2.b. Depositional setting of the Winnica Formation and Słupianka Member
The facies zones of the Łysogóry region (see Fig. 1) generally run in a NNW–SSE direction with more proximal facies occurring to the SW (Kozłowski, Reference Kozłowski2008). The deposition during this period was sensitive to small-scale alterations in sediment input and sea level, which is reflected by the lateral facies migrations and the different members of the Winnica Formation (Kozłowski & Munnecke, Reference Kozłowski and Munnecke2010). The Winnica and Rzepin sections overlap temporally, but represent slightly different environments in the shallow-marine facies belt of the Winnica Formation (Kozłowski, Reference Kozłowski2008).
The Winnica Formation is underlain by fine-grained sediments of the Trochowiny Formation that represent deposition in a low-energy, open-shelf environment (Kozłowski, unpub. thesis, 2006, University of Warsaw; Kozłowski & Munnecke, Reference Kozłowski and Munnecke2010). The wide geographical distribution of the Trochowiny Formation indicates a uniform sedimentary environment corresponding to the outer shelf (Kozłowski, Reference Kozłowski2008), but the top of the formation is characterized by a rapid loss of accommodation space (Kozłowski & Munnecke, Reference Kozłowski and Munnecke2010) due to the pronounced sea-level fall. The Winnica Formation starts with regressive to lowstand deposits of the shallow-water Jadowniki oolites in its lower part (Kozłowski & Munnecke, Reference Kozłowski and Munnecke2010). This is followed by transgressive pulses represented by the Bełcz Member, which records slowly rising sea level balanced by high rates of deposition (Kozłowski & Munnecke, Reference Kozłowski and Munnecke2010).
The Słupianka Member (upper part of the Winnica Formation) consists of green clayey shales, mudstone, siltstones, marls and argillaceous limestones that occur in coarsening-upwards cyclothems of thickness several metres, with the shales forming the base (Kozłowski, Reference Kozłowski2008; Kozłowski & Munnecke, Reference Kozłowski and Munnecke2010). These shallowing-upwards sequences contain open-marine faunal assemblages such as nuculoid bivalves, brachiopods, trilobites, numerous crinoid ossicles, bryozoans and beyrichiid ostracodes in their lower parts and marginal marine assemblages (eurypterid fragments, worm tubes, gastropods, leperditicopid ostracodes and fragments of terrestrial flora) in their upper parts (Bodzioch, Kozłowski & Popławska, Reference Bodzioch, Kozłowski and Popławska2003; Kozłowski, Reference Kozłowski2008; Kozłowski & Munnecke, Reference Kozłowski and Munnecke2010). The cycles most likely represent progradation of delta lobes into a marine environment with limited accommodation space (Kozłowski, Reference Kozłowski2008; Kozłowski & Munnecke, Reference Kozłowski and Munnecke2010), and the lack of larger fractions in both the Winnica and Rzepin sections has been explained by sediment bypassing (Kozłowski & Munnecke, Reference Kozłowski and Munnecke2010). The cyclothems increase in thickness towards the top of the member, recording subtle and gradual transgression and deepening. There is a maximal flooding interval towards the upper part of the Słupianka Member represented by two 10 m thick parasequences (Kozłowski & Munnecke, Reference Kozłowski and Munnecke2010). Additionally, the carbonate intercalations record more offshore facies towards the top, with stromatoporoids and tabulates forming small biostromes at the sample level in the Winnica section.
The uppermost part of the member (only visible in the Rzepin section) and the successive Bronkowice Formation contain abundant coarse-grained (silty to sandy) clastic material with intercalations of oolitic limestones that indicate a loss or limitation of accommodation space, and a resume of reworking and winnowing processes (Kozłowski & Munnecke, Reference Kozłowski and Munnecke2010). These sediments probably represent a stillstand deposit according to Kozłowski & Munnecke (Reference Kozłowski and Munnecke2010). The Bronkowice Formation is in turn followed by the Sarnia Zwola Formation, which is interpreted as a transgressive deposit with clayey shales and pelagic fossils that mark restitution to open-shelf sedimentation (Kozłowski & Munnecke, Reference Kozłowski and Munnecke2010).
The Słupianka Member as a whole is interpreted as representing a shallow-shelf environment with intermittent, limited communication with an open basin (Kozłowski, unpub. thesis, 2006, University of Warsaw), but local differences between the Winnica and Rzepin sites can be observed. The Winnica section is more proximal than the Rzepin section, but is interpreted by Kozłowski (Reference Kozłowski and Belka2000) as representing a relatively calm, back-barrier and periodically brackish environment. The carbonate layers at the Winnica site reveal a fully marine fauna, that is, brachiopods, crinoids, trilobites, rugose corals, tabulates and numerous stromatoporoids (Bodzioch, Kozłowski & Popławska, Reference Bodzioch, Kozłowski and Popławska2003), and could record periods of more open-marine conditions. However, most of the fauna (except stromatoporoids and tabulates) is strongly crushed, which might suggest a strong reworking for most of the bioclasts (Bodzioch, Kozłowski & Popławska, Reference Bodzioch, Kozłowski and Popławska2003). The sediments of the uppermost Winnica Formation in the Rzepin area, and the oolite layer where the JB142 sample is from, probably represent the final highstand in this formation (Kozłowski & Munnecke, Reference Kozłowski and Munnecke2010). Despite being more distal compared to the Winnica section, it therefore records an extremely shallow bathymetry and high-energy water with winnowing and reworking of oolite bars on a silty substrate (Kozłowski & Munnecke, Reference Kozłowski and Munnecke2010).
3. Materials and methods
The Winnica site samples were taken from a limestone bed in the upper part of the Słupianka Member, while the JB142 sample was taken from an oolite-bed with bioclasts close to the defined, upper boundary of the whole Słupianka Member in the Rzepin section (see Fig. 3 and Kozłowski, Reference Kozłowski2008). The JB142 sample therefore not only represents somewhat shallower marine conditions, but it is also from slightly younger sediments than the Winnica samples. The material is housed in the palaeontological collections of the Museum of Evolution (Uppsala University) in Uppsala, Sweden.
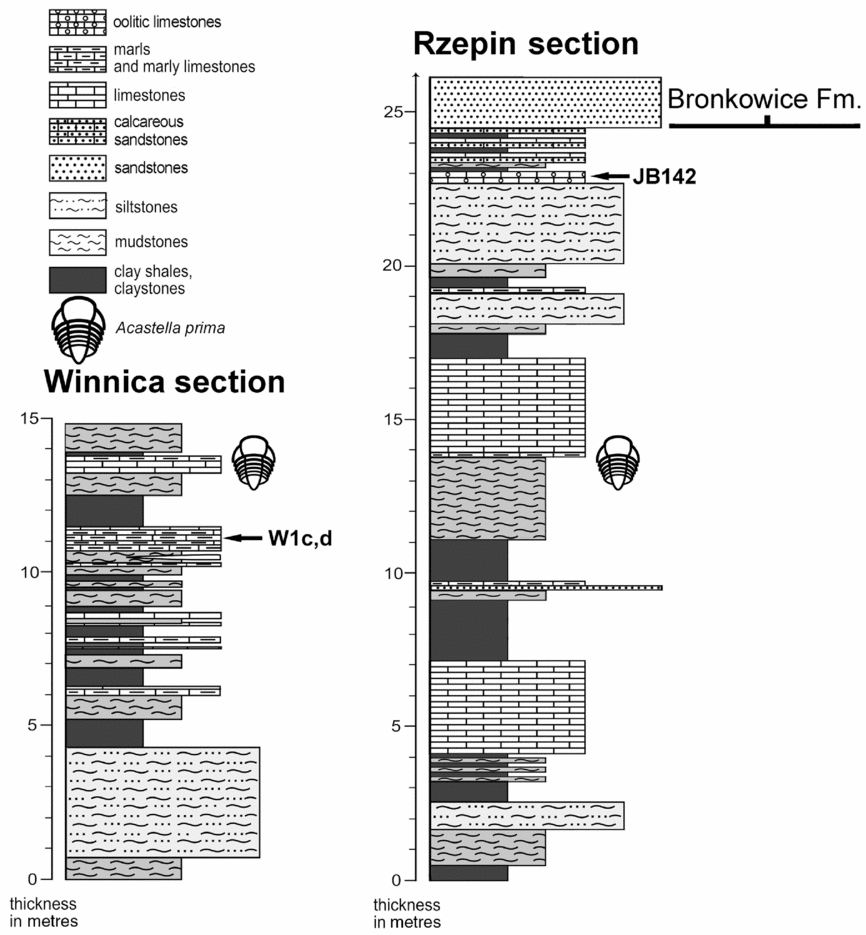
Figure 3. Lithological columns with information about positions of collected samples and the approximate level of the trilobite index fossil Acastella prima. Left: the top part of the section exposed at the Winnica locality (after Bodzioch, Kozłowski & Popławska, Reference Bodzioch, Kozłowski and Popławska2003). Right: uppermost part of the Słupianka Member in the Rzepin section (based on Kozłowski & Munnecke, Reference Kozłowski and Munnecke2010). The sections are only roughly correlated.
The Winnica locality is located at the western part of the Winnica village, about 1.6 km NE of the centre of Nowa Słupia (50°52′34″N, 21°06′25″E; for detailed maps see Kozłowski, Reference Kozłowski2008, fig. 6). In the Winnica site, olive to green clay shales, mudstones and siltstones intercalated with lenses of the marly carbonaceous rocks of the Słupianka Member (Fig. 3) are exposed along the Słupianka River (Bodzioch, Kozłowski & Popławska, Reference Bodzioch, Kozłowski and Popławska2003; Kozłowski, Reference Kozłowski2008).
The Rzepin section crops out in the eastern valley escarpment of an unnamed right tributary of the Świslina River (Rzepin area) close to the Jadowniki Dolne village (50°58′16″N, 21°04′39″E). This escarpment was cut by a research ditch in 2002 that was measured, described and sampled (Kozłowski & Munnecke, Reference Kozłowski and Munnecke2010). The uppermost part of the Słupianka Member is present in this section, and Kozłowski & Munnecke (Reference Kozłowski and Munnecke2010) described it as containing muscovite-bearing siltstones intercalated with mostly oncolitic, dark-coloured and bioclastic wackestones to packstones (Fig. 3).
The rock material from the Winnica and Rzepin sections was processed in the chemical laboratory of the Institute of Paleobiology, Polish Academy of Sciences. The W1c and W1d samples (each weighing c. 2 kg) were dissolved in dilute formic acid (5–10%). There was less acid added than necessary to dissolve the carbonates, which provided some buffering. In total 16 samples were dissolved from the middle (4) and upper (12) carbonate-rich part of the Winnica section, but only two contained phosphatic remains of vertebrates and microproblematica (carbon structures, possibly plant remains). After dissolution, the residue was carefully viewed under a magnifier and washed by warm water. The largest fraction in the samples was screened for large specimens after being dried. Only the six most promising samples were passed through a 1 mm sieve in order to remove acid-resistant elements and undissolved carbonitic pieces. The remaining dry residues of the W1c and W1d samples were separated in an electromagnetic mineralogical separator at the Institute of Paleobiology, Polish Academy of Sciences. The angle and amperage of separation were similar for all six samples. The residue was later screened using water and passed through 1 mm and 0.1 mm sieves.
The Rzepin sample (JB142) weighed c. 2 kg and was dissolved in 10% acetic acid at the chemical laboratory of the Institute of Paleobiology, Polish Academy of Sciences. The residue was ‘cooked’ in water with acid for a few minutes, which is a good technique for extracting phosphatic material from clay-rich carbonates. The JB142 residue was separated using a sodium polytungstate (SPT) solution with a specific density of 2.8 g cm–3 at the Department of Organismal Biology, Uppsala University, largely following the procedure described in Schiøler (Reference Schiøler1989).
The scanning electron microscopy (SEM) of microremains coated in a gold-palladium alloy was performed at the Evolutionary Biology Centre, Uppsala University with a Zeiss Supra 35VP, and at the Institute of Paleobiology, Polish Academy of Sciences with a Phillips XL-20. Some scales (indicated in the figure captions) were photographed in low vacuum with a variable-pressure SE detector at 3.3 mm working distance and an extra-high tension of 15.00 kV.
4. Vertebrate remains
The vertebrate faunas of the Winnica and Rzepin sections are similar, but there are some striking differences as a few taxa exclusively occur in the Rzepin sample. These vertebrate remains are described in more detail below, but their occurrences and relative abundance at the two sites are summarized in Table 1.
Table 1. Number of identified scales and other microremains in the Winnica and Rzepin samples.

The scale-taxonomy of acanthodians from the Baltic Basin is currently in a state of flux, which is further complicated by the increased understanding about variations in the squamation of acanthodians and other early gnathostomes (e.g. Trinajstic, Reference Trinajstic2001; Brazeau, Reference Brazeau2012; Burrow, Reference Burrow2013; Burrow et al. Reference Burrow, Newman, Davidson and den Blaauwen2013, Reference Burrow, Davidson, den Blaauwen and Newman2015, Reference Burrow, den Blaauwen, Newman and Davidson2016; Burrow & Rudkin, Reference Burrow and Rudkin2014; Chevrinais, Sire & Cloutier, Reference Chevrinais, Sire and Cloutier2017). A thorough review of the scale-based taxonomy of Baltic acanthodians is beyond the scope of this paper, and a detailed systematic description of these remains may therefore be superfluous. The scales will, however, be compared to largely coeval, scale-based taxa from the Baltic Basin as presented by Vergoossen (Reference Vergoossen, Ivanov, Wilson and Zhuravlev1997, Reference Vergoossen1999a, Reference Vergoossenb, Reference Vergoossenc, Reference Vergoossen, Blieck and Turner2000, Reference Vergoossen2002a, Reference Vergoossenb, Reference Vergoossenc, Reference Vergoossen2003, unpub. thesis, University of Groningen, Reference Vergoossen2003; Reference Vergoossen2004) and Valiukevičius (Reference Valiukevičius1998, Reference Valiukevičius2003a, Reference Valiukevičiusb, Reference Valiukevičius2004a, Reference Valiukevičiusb, Reference Valiukevičius2005, Reference Valiukevičius2006), albeit solely based on morphological similarities since no histological studies have been performed for this work. Valiukevičius & Burrow (Reference Valiukevičius and Burrow2005) demonstrated the importance of histology in scale-based taxonomy among ‘nostolepid’ acanthodians; the comparisons to different Nostolepis taxa solely based on morphological similarities here should therefore be regarded as tentative. The word ‘morphotype’ is used here to define different morphologies within an assemblage for both thelodonts and acanthodians. The different acanthodian morphotypes will be discussed separately and compared to other scale-based taxa in order to facilitate future reviews and revisions of acanthodians from the Baltic Basin.
4.a. Winnica site samples
The two Winnica site samples come from the same bed and have identical faunal components, and will therefore be treated collectively in the remaining text. The fauna consists of thelodont, acanthodian and anaspid scales and fragments. Larger pieces show signs of wear and breakage but many of the smaller vertebrate remains are relatively well preserved, enabling the majority to be readily identified.
4.a.1. Thelodonts
The bulk of the Winnica samples are represented by thelodont scales, and the majority of the thelodont scales conform within the scale-sets of Thelodus parvidens Agassiz Reference Agassiz and Murchisson1839 and Thelodus trilobatus Hoppe, Reference Hoppe1931, as presented in Märss, Turner & Karatajūte-Talima (Reference Märss, Turner, Karatajūte-Talima and Schultze2007), with the latter often being considered a scale variety of the former. There are also rare occurrences of traquairiform, bicostatiform and pugniform scales, again often considered as scale varieties within the squamation of T. parvidens (see Märss, Turner & Karatajūte-Talima, Reference Märss, Turner, Karatajūte-Talima and Schultze2007).
The scales identified as T. parvidens (Fig. 4a–h) constitute the majority of the larger thelodont scales in the samples, but they also range down to the smallest sizes. The least common morphotype in the samples is the traquairiform scale type (Fig. 4a, b). These scales are small and have shallow, trapezoid bases hosting a small central pulp cavity. The crown is high with a distinct neck (often with riblets) and has three or more ridges that unite in a single apex that is either central or slightly offset posteriorly. These ridges often have a knee-like bend halfway up the crown (Fig. 4b). The scales are similar to those described as Thelodus traquairi Gross, Reference Gross1967, but it has previously been suggested that they represent a specialized form of scales in the squamation of T. parvidens (Märss, Turner & Karatajūte-Talima, Reference Märss, Turner, Karatajūte-Talima and Schultze2007). Morphologically similar scales were figured as costatiform scales by Vergoossen (Reference Vergoossen2002c, pl. 1, fig. 1–6), who described them as oral scales of T. parvidens. The morphotype indeed fits nicely at the extreme, traquairiform end in a transition of scale morphologies where typical T. parvidens body scales with slightly crenulated crown rims grade into scales with only a small patch of smooth, flat crown in the centre (Fig. 4c). The latter is figured as head scales in Märss, Turner & Karatajūte-Talima (Reference Märss, Turner, Karatajūte-Talima and Schultze2007). Another scale morphotype (Fig. 4d) occurring in the samples has a diamond-shaped base with a small to moderately large pulp cavity opening. It has a distinct incurved, short and smooth neck and a slightly bulging crown which ends in one posterior apex, but does not overhang the base. The anterior part of the crown is composed of four distinct ridges: two medial ridges separated by a median furrow, and two lateral ridges accompanied by two lateral troughs. The most numerous scale morphotype (Fig. 4e) has a shallow to moderately deep base hosting a small, central pulp cavity opening. The base is mostly of the same dimensions as the crown and lacks a prominent anterior process. These scales have distinct, gently incurved necks, often hosting a number of vertical riblets. The crown surface is flat and smooth, and the anterior crown outline is angular or rounded while the posterior end has an acute posterior point. This results in crown shapes ranging from smooth-edged trapezoids to a water-drop-like morphology, with the posterior apex slightly overhanging the base. These probably represent body scales of T. parvidens (Märss, Turner & Karatajūte-Talima, Reference Märss, Turner, Karatajūte-Talima and Schultze2007). Some of the trunk scales have two longitudinal ridges along their crowns (Fig. 4f), effectively forming an intermediate between the typical trunk scale crown morphologies and those of the bicostatiform scales. The more typical bicostatiform scales (Fig. 4g, h) have two parallel medial ridges and one or two lateral longitudinal ridges, all with concave areas in between. These are similar to scales described as belonging to T. parvidens by Märss & Miller (Reference Märss and Miller2004, text-fig. 12B) and Märss, Turner & Karatajūte-Talima (Reference Märss, Turner, Karatajūte-Talima and Schultze2007, fig. 95N–Q).
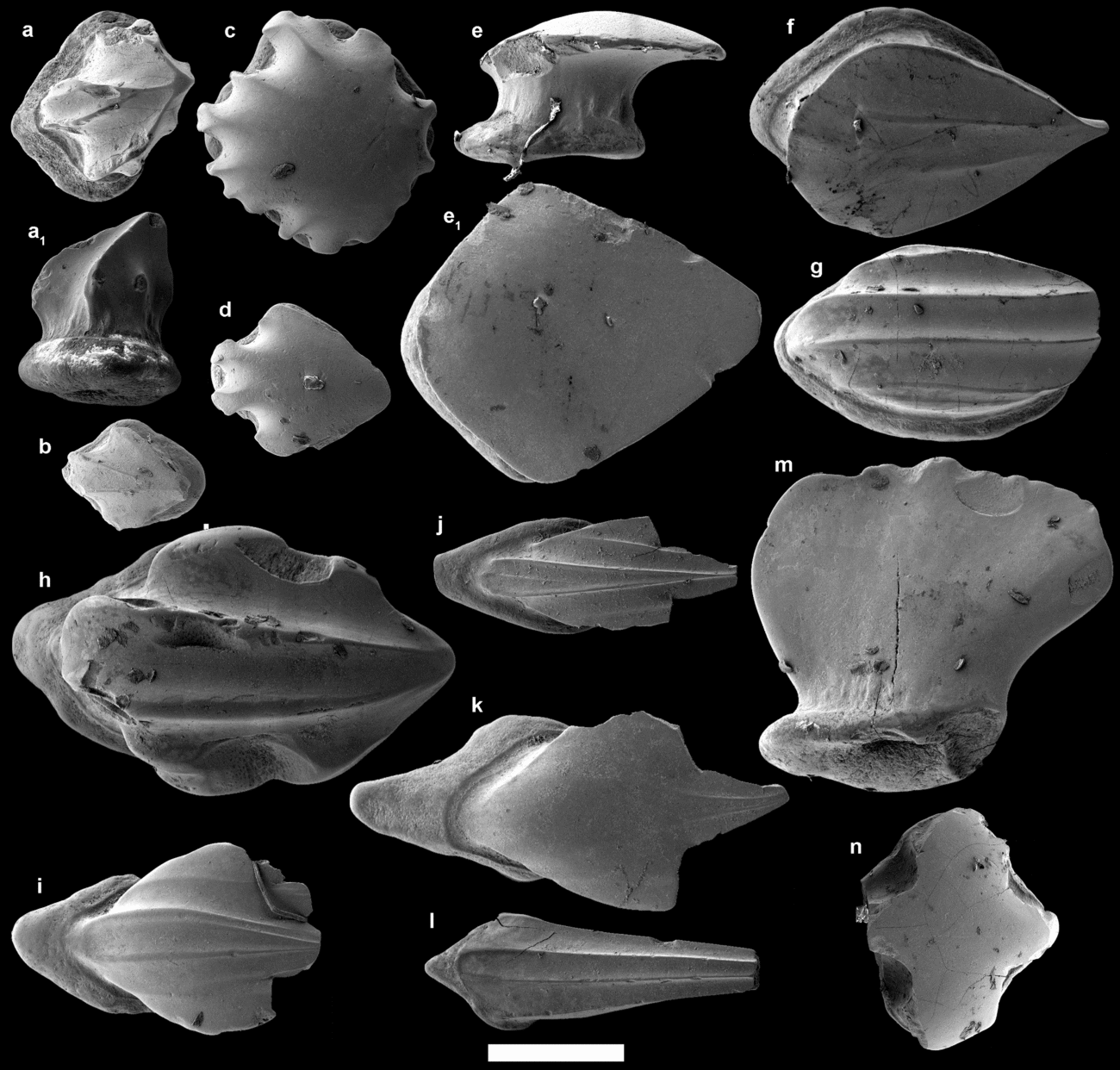
Figure 4. Thelodont scales from the Winnica samples identified as (a–h) Thelodus parvidens PMU 30019–30028 and (i–k) Thelodus trilobatus PMU 30029–30031, as well as the (l) fin scale PMU 30032, (m) pugniform scale PMU 30033, and (n) one of the two special scales (PMU 23151) of unclear thelodont affinity. All scales are depicted with anterior towards the left and in crown view, except for a1, e and m which that are in lateral view. Scale bar is equivalent to 200 µm.
In the SEM, many of the T. parvidens scales displayed the typical ultrasculpture described for this taxon (Märss, Reference Märss2006a). However, one scale with an overall morphology similar to T. parvidens had an ultrasculpture consisting of an irregular, polygonal network, which is typical of Oeselia mosaica Märss Reference Märss2005 (Fig. 5a, b). This taxon was described from the middle and upper Homerian Viita and Vesiku beds of Saaremaa, Estonia (Märss, Reference Märss2005), and also occurs in the largely coeval Halla and Klinteberg formations on Gotland (Jarochowska et al. Reference Jarochowska, Bremer, Heidlas, Pröpster, Vandenbroucke and Munnecke2016). However, the ultrasculpture on the scale from Winnica is not as prominent as that presented in Märss (Reference Märss2005), and it is unclear whether the typical ultrasculpture could represent a functional rather than taxonomical trait in these scales (T. Märss, pers. comm. 2016).
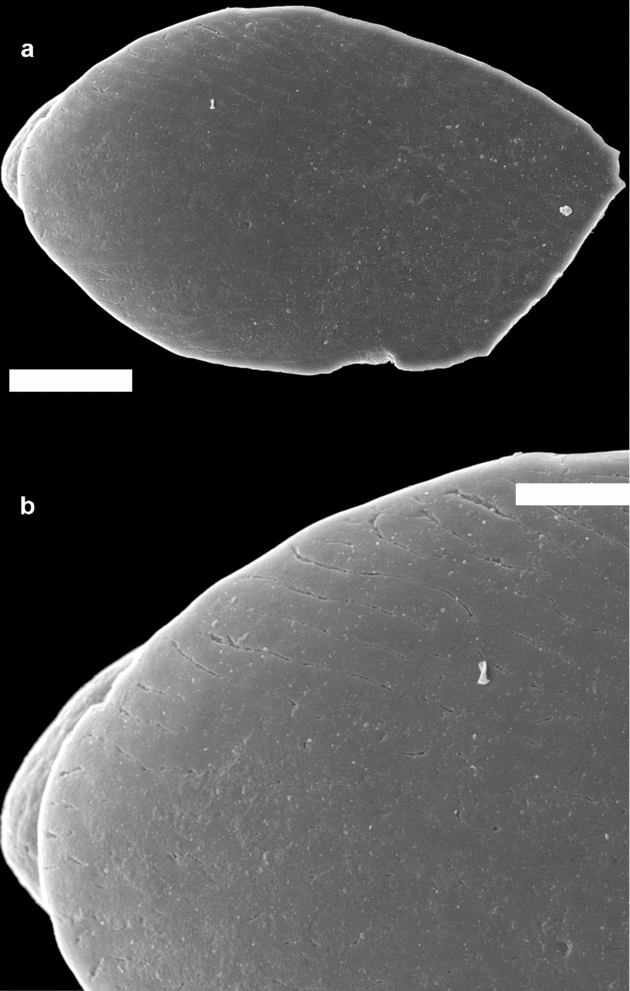
Figure 5. (a) Crown view and (b) close-up of the ultrasculpture identified on one of the thelodont scales from the Winnica section (PMU 30034), which is similar to that described for Oeselia mosaica. Scale bar (a) 50 µm and (b) 20 µm.
Scales identified as T. trilobatus (Fig. 4i–k) is the second most common scale form in the samples. They have a slightly more restricted range of sizes compared to the scales identified as T. parvidens, and mainly show two types of morphologies. The first morphotype (Fig. 4i, j) has a shallow to moderately deep base with a short to long anterior process and a posteriorly offset, small pulp cavity opening. The crown is flat and hosts two medial longitudinal ridges with a median trough, as well as two lateral longitudinal ridges. The crowns slant downwards at the anterior end and often ends posteriorly in three apices that overhang the base. The second morphotype (Fig. 4k) has a similar base morphology as the previous but the crown is flat and often smoother with a leaf-like morphology, although the anterior end is often ridged and slants downwards towards the anterior margin. The posterior part of the crown in these scales is often fragile and thin, and ends in three or more points beyond the base. Both these morphotypes have a short but distinct neck. Thelodus trilobatus was considered a separate species by Gross (Reference Gross1947, Reference Gross1967), Turner (Reference Turner1973) and Karatajute-Talimaa (Reference Karatajute-Talimaa1978), but has also been considered a scale variety of T. parvidens (Märss, Reference Märss1986; Turner, Reference Turner1986; Vergoossen, Reference Vergoossen2002a, Reference Vergoossenb, Reference Vergoossenc) because of their coeval occurrences and the presence of both types on an articulated specimen (Turner, Reference Turner1986) later synonymized (by some) with T. parvidens (see Märss, Turner & Karatajūte-Talima, Reference Märss, Turner, Karatajūte-Talima and Schultze2007 for details). However, Turner (Reference Turner, Boucout and Lawson1999, Reference Turner, Blieck and Turner2000) and Märss & Miller (Reference Märss and Miller2004) treated T. parvidens and T. trilobatus as separate taxa (see Märss & Miller, Reference Märss and Miller2004 for a more thorough discussion). Märss, Turner & Karatajūte-Talima (Reference Märss, Turner, Karatajūte-Talima and Schultze2007) presented T. trilobatus as a separate set of scales, but with the notion that it could be part of the squamation of T. parvidens as well as several other taxa (although with different histologies).
Four kinds of rare, special thelodont scales have been found in the Winnica samples. The first is represented by eight scales in the samples (Fig. 4l). These have small and spine-like, elongated crowns that extend far beyond the base posteriorly and host one median and two lateral longitudinal troughs separated by narrow ridges. The base of these scales is shallow, wider than the crown and hosts a large pulp cavity opening and a small anterior spur. These scales most likely represent the fin scales in a thelodont squamation. The second kind (Fig. 4m), which is represented by six scales, have an antero-posteriorly elongated, narrow and shallow base that hosts a small to moderately large pulp cavity. The crown is extremely compressed laterally, being thinner than the base, and expands in its antero-posterior direction which gives it an anvil-like appearance in lateral view with a slight posterior overhang over the base. The neck is short and smooth, but distinct. A couple of these scales have laterally thicker bases and crowns. These look like the pugniform scales (=Thelodus pugniformis Gross, Reference Gross1967) that have been interpreted as oral, pharyngeal or branchial scales (see Vergoossen, Reference Vergoossen2002c), and similar scales have been interpreted as belonging to T. parvidens (Märss, Reference Märss1986, pl. 21, fig. 5). The third scale with a special morphology (Fig. 4n) is only represented by two scales. These are similar to head scales of loganellids, but their affinities are considered as uncertain given their rarity and the general un-diagnostic features of head scales. Finally, two scales (see online Supplementary Fig. S1, available at http://journals.cambridge.org/geo) found in the Winnica sample is similar to one of the palmatilobate scales figured in Märss (Reference Märss2006b: pl. 4, fig. 18); however, the affinities of these scales remain unclear.
4.a.2. Anaspids
Anaspid remains (Fig. 6) are rare in the Winnica samples, only represented by six fragments. These are dense pieces with flat visceral surfaces and smooth external surfaces, with the exception of a few raised ridges. One fragment (Fig. 6b) is divided into a lower and a higher part by a distinct line running across it. This is reminiscent of the posterior external rib found in the scales of birkeniid anaspids (Blom, Märss & Miller, Reference Blom, Märss and Miller2002). When present, the slightly curved ridges on the external surface point in the same general direction, at an approximately 45° angle relative to the posterior external rib. The ridges are of different sizes (compare Fig. 6b and c), but have similar outlines and a flattened surface. The fragmentary nature of these pieces makes identification difficult, and they are reminiscent of both genera Tahulalepis Blom, Märss & Miller, Reference Blom, Märss and Miller2002 and Liivilepis Blom, Märss & Miller, Reference Blom, Märss and Miller2002 in their overall morphology (see Blom, Märss & Miller, Reference Blom, Märss and Miller2002: figs 40, 48). However, the curvature, height and flattened surface of the ridges makes them more similar to the latter and the fragments are therefore treated as cf. Liivilepis.
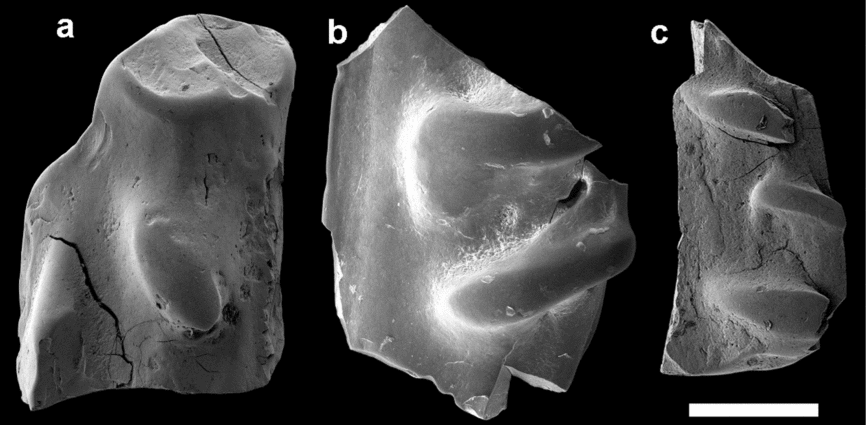
Figure 6. Anaspid fragments from the Winnica samples referred to as cf. Liivilepis, all in external view: (a) PMU 30055; (b) PMU 30056; and (c) PMU 31614. Low-vacuum SEM was used to acquire (b). Scale bar represents 300 µm.
4.a.3. Acanthodians
The acanthodian scales are common in the Winnica samples, although not as numerous as the T. parvidens scales. Many of them show signs of breakage, but most are still relatively well preserved. The largest pieces have been identified as a variety of acanthodian fragments, such as broken ischnacanthiform fin spines and teeth, as well as larger tesserae. However, these remains are difficult to determine taxonomically and will not be discussed further.
A number of different scale types have been identified in the Winnica samples and can generally be divided into ‘nostolepid’, poracanthodid and ‘gomphonchid’ types. The most numerous acanthodian scales are those belonging to the ‘nostolepid’ type. These form a range of more or less overlapping morphologies, but seven different morphotypes can be distinguished. All of them fit within the morphological and temporal range of scales that Vergoossen (Reference Vergoossen1999a, Reference Vergoossen2002a, Reference Vergoossenb, Reference Vergoossenc, Reference Vergoossen2003, Reference Vergoossen2004) treated as Nostolepis striata Pander, Reference Pander1856, largely following the definition by Gross (Reference Gross1947, Reference Gross1971). However, Valiukevičius (Reference Valiukevičius2003a, Reference Valiukevičius2004a) viewed the morphological range of N. striata as too wide and erected several separate taxa from it. Vergoossen (Reference Vergoossen, Blieck and Turner2000) also viewed the clean sweep of N. striata performed by Gross (Reference Gross1947, Reference Gross1971) as problematic because Gross disregarded both stratigraphical and geographical differences and synonymized several taxa from Devonian material to older variants described by Lehman (Reference Lehman1937), and included them all in N. striata. The lack of articulated specimens makes it difficult to determine whether the different scale morphologies described below belong to different taxa, or if they merely represent variation within the squamation of one taxon. All of the ‘nostolepid’ scales presented in this work are tentatively referred to as N. striata, but the different morphotypes and their counts are described separately.
Type 1 scales (Fig. 7a–e) display the widest range of morphologies and are represented by more than 100 scales. These have irregular to rhomboidal, shallow to moderately tumid bases that are often larger than the crown. The crowns are symmetrical or slightly curved, and wider anteriorly where they host a differing number of pronounced but short riblets (compare Fig. 7a and b) that are often irregularly arranged and bend downwards towards the base. Two of these riblets develop into prominent lateral rims that form a median area above two oblique ridges. This median area hosts a shallow and short trough in some scales (Fig. 7b), or a deep trough that runs along a large part of the crown length in some scales (Fig. 7d). The crown is moderately inclined and tapers into a single posterior apex that overhangs the base posteriorly in most scales. Many of the type 1 scales may not be directly comparable to the original description of N. striata by Pander (Reference Pander1856), but a majority of them fit the features listed for the taxon in Gross (Reference Gross1947). Furthermore, Gross (Reference Gross1947) described (but did not figure) asymmetrical scale variants where one lateral half of the crown was more pronounced, which created a shallow to deep trough running along the midline of the crown (e.g. Fig. 7b, d). The scales in Figure 7a–c are also similar to the N. striata scales presented by Valiukevičius (Reference Valiukevičius2005, fig. 3L, M). A few of the type 1 scales show similarities to Nostolepis amplifica Valiukevičius Reference Valiukevičius2003a and especially the Nostolepis cf. amplifica scales presented in Valiukevičius (Reference Valiukevičius2004a, fig. 4B), but they often lack the pores at the base of the neck typical for this taxon. Valiukevičius (Reference Valiukevičius2003a) claimed that typical N. striata scales did not have a pronounced, longitudinal trough on the crown (contra Gross, Reference Gross1947; Vergoossen, Reference Vergoossen2002b), and synonymized similar scales as ‘morph 2’ of the new taxon Nostolepis musca Valiukevičius Reference Valiukevičius2003a, which also display a similar range of morphologies as some of the scales described here. The Winnica samples contain a number of scales with type 1 crown structure, but with flat and sometimes concave bases. These scales have steep and pointed crowns that host prominent ridges (Fig. 7e). Burrow et al. (Reference Burrow, Vergoossen, Turner, Uyeno and Thorsteinsson1999) mentioned that scales with such morphology could represent pinnal scales of climatiids, and similar scales were described as part of the squamation in N. amplifica by Valiukevičius (Reference Valiukevičius2003a, fig. 2H).
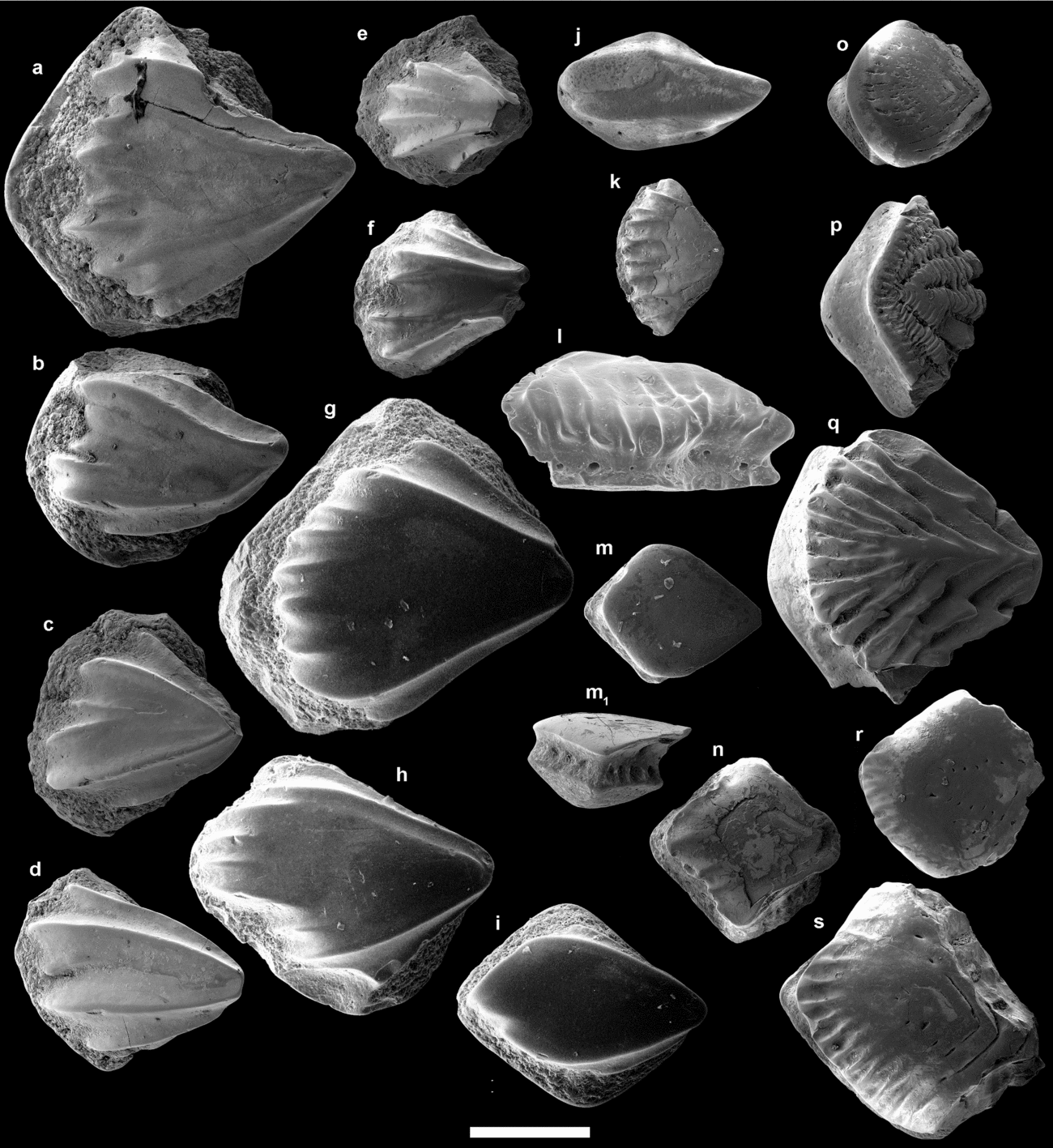
Figure 7. Acanthodian scales from the Winnica samples. ‘Nostolepid’ type 1: (a–e) PMU 30035–30039; type 2: (f) PMU 30040; type 3: (g) PMU 30041; type 4: (h) PMU 30042; type 5: (i) PMU 30043; type 6: (j) PMU 30044; and Nostolepis sp. indet.: (k--l) PMU 30045–30046. ‘Gomphonchid’ type scales: (m, m1) Gomphonchus sandelensis PMU 30047–30048 and (n) Gomphonchus volborthi PMU 30049. Poracanthodid type scales: (o) Radioporacanthodes biblicus PMU 30050; (p) ‘forma bifurcata’ PMU 30051; (q) ‘conicus variant’ PMU 30052; and (r, s) Radioporacanthodes sp. indet. PMU 30053–30054. All scales in crown view with anterior towards the left, except l (anterior view) and m1 (lateral view). Low-vacuum SEM was used to acquire (g–i) and (m). Scale bar equals 300 µm.
The ‘morph 2’ described for N. musca by Valiukevičius (Reference Valiukevičius2003a, fig. 5O), that is, pronounced ridges on the crown and a concave median area, is similar to scale type 2 (Fig. 7g) in our samples. The crowns are asymmetric and the two most pronounced ribs divide towards the anterior end. Since the posterior part is often broken, the strongly concave median area creates a seemingly two-pointed posterior crown. However, unlike the scales described as N. musca by Valiukevičius (Reference Valiukevičius2003a), these scales have a flat or only slightly convex base and lack a pronounced neck. Only seven of these scales have been recovered in the Winnica samples, and Vergoossen (Reference Vergoossen2004, pl.7, fig. 62) described similar scales as ‘modified’ N. striata scales.
Type 3 (Fig. 7d) are represented by 23 scales in the samples. It is similar to the first scale type, but the base is deeper and the more triangular crown has a pronounced and flat median area. The crown hosts several small and parallel riblets at its anterior end, and it is only slightly inclined or almost flat. These scales are superficially similar to N. amplifica scales (compare Valiukevičius, Reference Valiukevičius2003a, fig. 2D), but most of them lack the neck-pores typical for this taxon.
Fourteen type 4 scales (Fig. 7h) have been recovered. They have elongate, horizontal crowns that host pronounced, parallel riblets at the anterior end. The anterior ends meet in an anterior point but one of them is markedly longer than the other posteriorly, which makes the crown asymmetric. Two of the riblets form lateral rims on the sides of the flat crown that converge posteriorly to a single point. Below the lateral rims on each side are oblique ridges. The base is moderately deep and rhomboidal in outline. This scale type is similar to scales figured as transitional scales of N. amplifica or Nostolepis elegans Brotzen Reference Brotzen1934 in Valiukevičius (Reference Valiukevičius2005, fig. 6l). Vergoossen (Reference Vergoossen2004, pl. 5, fig. 56) figured scales with similar crowns (but larger bases) as N. striata flank scales.
The fifth scale type (Fig. 7i) is represented by 27 scales. This morphotype has a horizontal and smooth crown with narrow oblique ridges. These ridges originate approximately halfway along the crown and gently slant upwards towards the posterior crown tip. In the largest type 5 scales, there are up to five smaller oblique ribs anterior to the main oblique ridge on the ‘shorter’ side of the crown (the side facing down in Fig. 7i). The crown has an anterior point with weakly developed ribs that also converge anteriorly. The base is deep, elongated rhomboidal and larger than the crown, except in some scales where the crown slightly overhangs the base posteriorly. Morphologically similar scales were referred to the Devonian Nostolepis arctica Vieth Reference Vieth1980 by Vergoossen (Reference Vergoossen1999b, fig. 4), who thought that some of the Devonian N. striata scale morphologies indeed represent a different taxon. However, the same scales were later revised as N. elegans by Valiukevičius (Reference Valiukevičius2004a), who also reinstated N. elegans as a separate species on the basis that N. arctica scales have more steeply inclined crowns with more prominent ornamentation. He also referred several other reports to N. elegans (see synonymy list in Valiukevičius, Reference Valiukevičius2004a). This was partly in agreement with Vergoossen's (Reference Vergoossen1999a, Reference Vergoossenb, Reference Vergoossen2002a, Reference Vergoossenb, Reference Vergoossenc) views in that he did not consider the older, Silurian scales as belonging to N. arctica. However, Vergoossen (Reference Vergoossen1999a, Reference Vergoossenb, Reference Vergoossen2002a, Reference Vergoossenb, Reference Vergoossenc) considered them as part of the N. striata squamation, but recognized them as distinguishable and specialized scales, which he called the ‘elegans form group’.
A sixth scale type (Fig. 7j) from the Winnica samples is only represented by two scales. These are similar to type 5, but the crowns are more elongated and some of them preserve pores around the neck. The scales in the Winnica samples are not as highly inclined as N. arctica (Valiukevičius, Reference Valiukevičius2004a) scales, and the crown overhang is not as long as in those described for N. elegans by Valiukevičius (Reference Valiukevičius2004a). Furthermore, the pores around the neck in some scales strengthen potential affinities to N. amplifica (Valiukevičius, Reference Valiukevičius2004a).
Another morphotype with ‘nostolepid’ affinity is only represented by five scales in the Winnica samples. These scales (Fig. 7k, l) have slightly bulging or flat bases that are wider laterally than antero-posteriorly, and exceed the crown slightly at the anterior margin. The concave neck is narrow, high and prominent on the posterior side of the scale, but restricted at the anterior end where the crown bends down towards the base. The neck also hosts a series of pores (Fig. 7l). The strongly inclined, rhomboidal crown is wider than long, rounded anteriorly, and hosts longitudinal ridges of varying sizes that extend roughly halfway across the crown surface before fading out to differing degrees. Given their rarity, these scales could represent a specialized position on the body, and similar scales were identified as N. striata by Gross (Reference Gross1947, pl. 7, fig. 13). Furthermore, Vergoossen (Reference Vergoossen1999c) suggested that scales with similar morphologies could have been part of the same squamation as N. striata. These scales are here referred to as Nostolepis sp. indet.
The second most common acanthodian scales in the Winnica samples are those of ‘gomphonchid’ type (Table 1), but the group is only represented by two main morphotypes. The first is similar to Gomphonchus sandelensis Pander, Reference Pander1856 (Fig. 7m, m1). These scales have prominent necks and bulging bases that are of similar dimensions as the crown or slightly smaller. The crown is flat and smooth on both its surface and along the circumference, but the anterior edge can be slightly ribbed in some scales. The anterior outline of the crown is rounded, but it is abutted by lateral sides from where the bulging posterolateral edges originate and converge towards a posterior point that extends beyond the base to differing degrees. The often high necks display differing degrees of ribbing on the anterior and posterior side (Fig. 7m1). The other morphotype (Fig. 7n) is comparable to Gomphonchus volborthi Rohon, Reference Rohon1893. These scales have convex bases with rounded rhomboidal outlines and low but prominent necks. The base is larger than the crown, except at the posterior end where the crown can overhang it to differing degrees. In some scales the base is enlarged anteriorly and downwards. Similar to G. sandelensis the crown in this morphotype is flat, but it has a more angular outline and often hosts larger, more prominent ribs anteriorly. Furthermore, the posterolateral sides of the crown are more sharply angled to the anterior edge, and they often acquire a concave outline and taper quickly towards the posterior point. Vergoossen (Reference Vergoossen1999a, Reference Vergoossenb) considered G. volborthi as a valid taxon (contra Gross, Reference Gross1947) and viewed previous reports of G. sandelensis from rocks older than late Pridoli age (e.g. Fredholm, Reference Fredholm1988) as belonging to G. volborthi. However, this was not agreed upon by Valiukevičius (Reference Valiukevičius2003b). Some of the ‘gomphonchid’ scales in the Winnica samples have anterior edges that acquire concave outlines, and some crowns show down-stepped lateral rims. This is more reminiscent of Gomphonchoporus (previously Gomphonchus) hoppei Gross, Reference Gross1947, but histology is needed to confirm a potential affinity to this taxon. Furthermore, Gom. hoppei has not been reported from rocks older than of Pridoli age (Vergoossen, Reference Vergoossen1999c). It must be emphasized that the ‘gomphonchid’ types of scales described above overlap in morphology to differing degrees, and it is therefore uncertain if they truly represent different taxa or merely variation within one squamation.
Poracanthodid acanthodian scales are almost as numerous as the ‘gomphonchid’ scales in the Winnica samples (Table 1). Scales identified as Radioporacanthodes biblicus Lehman Reference Lehman1937 (Fig. 7o) have high and restricted necks, swollen bases and flat, teardrop-shaped crowns with a smooth anterior border. Posterior to this border arise a number of arcade slits that run over the remaining crown surface, forming the typical menorah-like pattern described by Vergoossen (Reference Vergoossen2002a). The crown overhangs the base posteriorly and the posterior neck hosts a number of vertical slits. Other, possibly specialized, porosiform scales found in the samples are referred to as the ‘forma bifurcata’ (Fig. 7p) and the ‘conicus variant’ (Fig. 7q), both defined by Vergoossen (Reference Vergoossen, Ivanov, Wilson and Zhuravlev1997, Reference Vergoossen1999b, Reference Vergoossen, Blieck and Turner2000). Another porosiform scale type (Fig. 7r, s) has rhomboidal, flat crowns that are anteriorly ribbed and host radial rows of pores on its surface. No successful comparison has been found and they are here referred to as Radioporacanthodes sp. indet. One of these scales (Fig. 7s) shows tendencies towards having arcade slits, which makes it somewhat reminiscent of Poracanthodes? lehmani Vergoossen Reference Vergoossen1999a.
4.b. Rzepin site sample
The state of preservation in the JB142 sample from the Rzepin site is not as good as in the Winnica samples, with many scales showing signs of abrasion and some even being rounded, which probably resulted from the higher energy of this deposit. The vertebrate fauna is similar to the Winnica samples, but not the same (see Table 1). As well as thelodonts, acanthodians and anaspids, rare remains of osteostracans have also been found in this sample.
4.b.1. Thelodonts
Thelodus parvidens (including traquairiform and bicostatiform variants) and T. trilobatus have also been found in the JB142 sample (online supplementary Fig. S2a–e, available at http://journals.cambridge.org/geo). These generally display the same range of morphologies as those from the Winnica samples. Thelodus parvidens is also very common in this sample but T. trilobatus is exceedingly rare (Table 1), which is surprising since they are often considered to represent the same species.
Scales identified as Paralogania ludlowiensis Gross, Reference Gross1967 are as numerous as T. parvidens in the Rzepin sample. Most of the scale variants presented for this taxon in the re-description by Miller & Märss (Reference Miller and Märss1999) have been found (Fig. 8), but they lack the complete lateral ribs otherwise only seen in exceptionally well-preserved specimens (see Miller & Märss, Reference Miller and Märss1999). Scales similar to head scales figured for this taxon by Miller & Märss (Reference Miller and Märss1999, pl. 2, fig. 4) have been found in the sample (Fig. 8a), but the crown is not as steeply inclined. Other scales only have a small, knob-like crown (Fig. 8b) which is unlike anything figured for P. ludlowiensis before; however, their overall morphology is otherwise similar to the other P. ludlowienis scales. There are also scales similar to those which Turner (Reference Turner1973, fig. 4c) described as head scales (Fig. 8c), but which Miller & Märss (Reference Miller and Märss1999) later interpreted as transitional scales of Paralogania. These have an oval to quadrangular base that is wider than the crown, a high neck without vertical ribs and a slightly elevated to flat crown that often host knobs along its margin. Other transitional scales have similar bases and necks, but the crowns can display a range of morphologies: some have a more irregular crown outline and a couple of longitudinal ridges along its surface (Fig. 8d, f), while others have a smoother anterior margin and a flattened crown hosting a number of spines along its posterolateral margins (Fig. 8e). The trunk scales (Fig. 8g, h) have elongated rhomboidal or quadrangular crowns with a flat surface or sometimes a shallow median furrow. A prominent oblique ridge runs on both sides of the crown (Fig. 8g), originating anteriorly at the contact with the base and slanting upwards and backwards until they meet below the posterior crown apex. These ridges are sometimes more prominent on one side of the crown. The presence of lateral spines is evident by their preserved attachments, usually five of them, underneath the previously mentioned ridges (Fig. 8h). A few scales attain morphologies approaching that of fin scales (Fig. 8i, j), but extreme forms such as those figured in Miller & Märss (Reference Miller and Märss1999, pl. 2, fig. 25) have not been recovered.

Figure 8. Paralogania ludlowiensis from the Rzepin sample: (a) head scale (PMU 30057); (b) knob-like scale (PMU 30058); (c–e) transitional scales (PMU 30059–30061); (f) PMU (31649); (g, h) trunk scales (PMU 30062–30063), and (i, j) possible fin scales (PMU 30064–30065). All scales in crown view and facing left, except h (lateral view). Scale bar equals 200 µm.
4.b.2. Anaspids
Only one anaspid fragment has been found in the JB142 sample, but it broke in the mounting process for SEM. It was morphologically similar to those referred to as cf. Liivilepis in the Winnica samples, and most likely represents the same taxon.
4.b.3. Osteostracans
As previously stated, the Rzepin sample contains rare osteostracan remains. Eight osteostracan fragments displaying different morphologies were found in the sample. Some hosted a regular network of pores divided by ridges (Fig. 9a), while others consisted of larger, detached and smooth tubercles with pores visible at their bases (Fig. 9b). One larger fragment (Fig. 9c) hosts a number of long ridges running in the same direction with a faint ribbed pattern and pores discernible toward their bases. All of the fragments described above are reminiscent of the sculpture developed in the largely coeval East Baltic taxon Tahulaspis ordinata Märss, Afanassieva & Blom, Reference Märss, Afanassieva and Blom2014 but, because of their fragmentary state, the few remains described here are referred to as Tahulaspis cf. ordinata.

Figure 9. Osteostracan fragments referred to as Tahulaspis cf. ordinata from the Rzepin sample. Fragment (a) PMU 30071 and (c) PMU 30073 in external view; and (b) PMU 30072 in lateral view. Low-vacuum SEM was used to acquire (c). Scale bar represents 300 µm.
4.b.4. Acanthodians
Acanthodian scales are also the second most common group of scales in the Rzepin sample, but they are generally smaller than those from the Winnica section and often less well preserved. The largest fragments in the sample are identified as broken fin spines and teeth, an ischnacanthiform jaw fragment (Fig. 10a) and a few tesserae.

Figure 10. An ischnacanthiform jaw fragment (a) PMU 30067 and acanthodian scales only found in the Rzepin sample: Nostolepis gracilis (b, c) PMU 30068–30069 and cf. Gomphonchus (d) PMU 30070. The jaw fragment is in lingual view with anterior to the right, (b) is in anterior view, (c) is in crown view with anterior facing downwards and (d) is in crown view with anterior towards the left (based on swelling of base). Scale bar equals 200 µm.
All of the ‘nostolepid’-type scales found in the Winnica samples are also present in the sample from the Rzepin section (online supplementary Fig. S2f–j). Type 1 scales are again represented by more than 100 scales, but they are generally smaller and have flatter bases. Nineteen type 2 scales, only one small scale comparable to type 3 (not figured), 15 type 4 scales, 24 type 5 scales and 24 type 6 scales have been recovered in the Rzepin sample. The type 1 scale in supplementary Figure S2f is superficially similar to the N. amplifica scale figured by Valiukevičius (Reference Valiukevičius2003a, fig. 2A), but no pores are visible around its neck. Many of the ‘nostolepid’ scales from the Rzepin sample that are included in morphotype 6 have more restricted bases and subsequently larger posterior overhangs (supplementary Fig. S1j). The posterior part is often not well preserved, which gives the crown in some of these scales an elongate, rectangular outline. In addition to this, the Rzepin sample contains 46 scales identified as Nostolepis gracilis Gross, Reference Gross1947. These scales (Fig. 10b, c) have flat to slightly bulging bases that are wider laterally than antero-posteriorly. The neck is prominent, high and concave all around. The crown is slightly elevated or flat and hosts differing numbers of more or less pronounced, straight ribs that run from the anterior edge towards the back of the crown. In some scales these ribs converge slightly as in the original descriptions by Gross (Reference Gross1947). Unlike the original description, however, the ribs on many scales fade out roughly half-way across the crown and the number of ribs is sometimes fewer. Pores on the crown surface, which is a common feature in this taxon (see Valiukevičius, Reference Valiukevičius1998), are difficult to discern with certainty as a result of the poor preservation. It should be mentioned that the sample also contains a few scales more similar to the two Nostolepis sp. indet. scales described from the Winnica samples (Fig. 7k, l), and could likewise represent specialized scales.
Similar to the Winnica samples, the second most common acanthodian group in JB142 is the ‘gomphonchid’ type. These are also represented by the two morphotypes showing affinities to G. sandelensis and G. volborthi (supplementary Fig. S1o, p). One additional morphotype present in the sample (Fig. 10d) is similar to Gomphonchus? minutus Valiukevičius Reference Valiukevičius2004a, but is treated here as cf. Gomphonchus since no histological data are available. It has a flat crown with a curved, rhomboidal outline, smooth edges and a low but prominent neck. The base has a similar outline and dimensions as the crown, and is moderately tumid. These scales are considered as ‘gomphonchid’ because the swelling of the base is slightly offset, and because the neck is well marked around the entire crown.
Poracanthodids are collectively only represented by ten scales in the JB142 sample. These are not well preserved but R. biblicus, the ‘forma bifurcata’, the ‘conicus variant’ and cf. R. sp. indet have been identified (supplementary Fig. S1k–n).
5. Discussion
Polish vertebrate microremains were described from the Miastko-1 core by Märss (Reference Märss1997), who reported T. parvidens, T. traquairi, Loganellia cuneata Gross, Reference Gross1947, Gomphonchus? hoppei, G. sandelensis, N. striata, N. gracilis and redeposited Thelodus sculptilis Gross, Reference Gross1967 scales from one level. Consequently, Märss (Reference Märss1997) assigned this level to the Nostolepis gracilis Zone Märss Reference Märss, Kaljo and Klaasmann1982a and equalized its fauna to the upper Kaugatuma and lower Ohesaare stages of Pridoli age in the East Baltic. The faunas reported here are of a similar composition, but contain P. ludlowiensis and lack L. cuneata as well as definite Gom. hoppei scales. The sampled levels at the Winnica and Rzepin sites are probably older than that reported from the Miastko-1 core, but the precise placement within the vertebrate biozonation scheme established for the Silurian (see Märss & Männik, Reference Märss and Männik2013) has proven difficult (see below).
The faunal composition of the two sites investigated here are similar (Table 1), but only the Rzepin sample contains the thelodont P. ludlowiensis, acanthodians N. gracilis and cf. Gomphonchus as well as remains of osteostracans. Notable numerical differences between the samples are the high abundance of poracanthodid-type scales and hundreds of T. trilobatus scales in the Winnica samples, while the Rzepin sample only contained a few scales of each.
Combined sequence stratigraphy and chemostratigraphy place the Słupianka Member as largely equivalent to the Hamra and Sundre formations on Gotland (Kozłowski & Munnecke, Reference Kozłowski and Munnecke2010), the latter being placed within the Ozarkodina crispa Conodont Zone (Jeppsson, Eriksson & Calner, Reference Jeppsson, Eriksson and Calner2006). According to Märss & Männik (Reference Märss and Männik2013), this conodont zone (sensu Walliser, Reference Walliser1964) likely equals a hiatus between the Paadla and Kuressaare regional stages in the East Baltic, which was also noticed by Märss (Reference Märss1992). However, O. crispa seems to have both a wider morphological variation and a longer stratigraphical range than previously thought, and the taxon shows some striking ecostratigraphical trends with earlier forms only occurring in shallow-water settings (Kaljo et al. Reference Kaljo, Einasto, Martma, Märss, Nestor and Viira2015). In the East Baltic the latest Ludfordian is represented by the Kuressaare Stage, which includes the Oz. remsheidensis baccata – Oz. snajdri parasnajdri conodont subzones in the Oz. remscheidensis superzone (Märss & Männik, Reference Märss and Männik2013). This is slightly complicated by Oz. snajdri parasnajdri showing similar ecostratigraphical trends to O. crispa (Kaljo et al. Reference Kaljo, Einasto, Martma, Märss, Nestor and Viira2015). In both Estonia and Latvia, the thelodont index taxon T. sculptilis first appears at the same level as Oz. remsheidensis baccata, and its biozone is correlated with the Oz. remsheidensis baccata – Oz. snajdri parasnajdri Zone in these areas (Märss & Männik, Reference Märss and Männik2013). In Lithuania, T. sculptilis first appears within the Pagegiai Regional Stage at a level corresponding to the Oz. crispa Conodont Zone (see Märss & Männik, Reference Märss and Männik2013). Märss & Männik (Reference Märss and Männik2013) therefore equated the Thelodus sculptilis Zone Märss Reference Märss, Kaljo and Klaasmann1982a to both the Oz. crispa and Oz. remsheidensis baccata – Oz. snajdri parasnajdri conodont zones. However, T. sculptilis has been reported from the Burgsvik and Sundre formations on Gotland (Fredholm, Reference Fredholm1989; Märss, Reference Märss, Holland and Bassett1989, Reference Märss1992; Eriksson, Nilsson & Jeppsson, Reference Eriksson, Nilsson and Jeppsson2009), the former being placed within the older O. snajdri Conodont Zone (Jeppsson, Eriksson & Calner, Reference Jeppsson, Eriksson and Calner2006). The subsequent Thelodus admirabilis vertebrate Zone Märss Reference Märss, Kaljo and Nestor1990 starts within the Oz. remsheidensis baccata – Oz. snajdri parasnajdri Conodont Subzone, prior to the first appearance of Oz. remsheidensis eosteinhornensis, and covers most of the Oz. remsheidensis eosteinhornensis Conodont Subzone, hence spanning latest Ludfordian – earliest Pridoli (Märss & Männik, Reference Märss and Männik2013). Neither T. sculptilis nor Thelodus admirabilis Märss Reference Märss1982b have been found in the samples treated here. The lack of the latter is surprising considering previous correlations and since it has been reported from lagoon to slope facies in the Baltic Basin (Märss, Reference Märss1982b). The lack of T. sculptilis in the Winnica samples despite the postulated late Ludfordian age of this section (Kozłowski, Reference Kozłowski2008) could be attributed to facies, since the Poracanthodes porosus Zone Märss & Männik Reference Märss and Männik2013 has been suggested as a deeper shelf equivalent to the T. sculptilis, T. admirabilis and N. gracilis zones (Märss, Reference Märss1997; Märss & Männik, Reference Märss and Männik2013). Indeed, the higher abundance of poracanthodid-type scales at the sample level in the Winnica site, which contains an invertebrate fauna characteristic for more distal parts of the shelf (Bodzioch, Kozłowski & Popławska, Reference Bodzioch, Kozłowski and Popławska2003), does corroborate this suggestion. However, this is not entirely in agreement with the suggested depositional environment proposed for the Winnica section (see Section 2.b. above). Furthermore, it is unclear why T. sculptilis and T. admirabilis are also missing in the shallow-water facies of the Rzepin sample if previous correlations are correct.
The acanthodian N. gracilis is the index taxon for the upper Äigu Bed and most of Lõo Bed of Kaugatuma Regional Stage in the East Baltic, which is early Pridoli in age (Märss & Männik, Reference Märss and Männik2013). The lower boundary of this vertebrate zone is defined where N. gracilis starts to dominate the fauna, but it is also present in older beds in the northern Baltic (Märss & Männik, Reference Märss and Männik2013). Several scales of N. gracilis have been recovered from the Rzepin sample, but it is in no regards dominating the fauna (see Table 1). Valiukevičius (Reference Valiukevičius2005, Reference Valiukevičius2006) reported N. gracilis from the Pagegiai Regional Stage (i.e. Oz. crispa Conodont Zone) from eastern Lithuania, together with the acanthodians N. striata, N. amplifica, N. musca, Nostolepis consueta, G. sandelensis, G. sp. cf. G. hoppei, Gomphonchus sp., Arenaceacanthus arcuatacanalis, Acanthoides? sp. and Cheiracanthoides sp. in the Sudervé Beds of the Neris Formation. Thelodonts T. sculptilis and T. admirabilis have also been reported from the upper Pagegiai Regional Stage (Karatajūte-Talimaa & Brazauskas, Reference Karatajūte-Talimaa and Brazauskas1994). In the western parts of Lithuania, which represents deeper-water facies according to Valiukevičius (Reference Valiukevičius2006), N. gracilis does not occur before the Pridoli. Märss & Männik (Reference Märss and Männik2013) considered the late Ludfordian N. gracilis scales from both eastern Lithuania and northern Baltic as atypical because of their smaller size and fewer ribs on the crown. It is unclear how the scales presented here compare to these, but they are generally small (0.3 mm long) and sometimes only host three ribs on the crown (Fig. 10b). As previously stated, the occurrence of the trilobite A. prima (Kozłowski, Reference Kozłowski2003, Reference Kozłowski2008; Kaljo et al. Reference Kaljo, Einasto, Martma, Märss, Nestor and Viira2015) and the ostracod faunas (Tomczykowa & Witwicka, Reference Tomczykowa and Witwicka1974; Tomczykowa, Reference Tomczykowa1991), as well as sequence stratigraphy and chemostratigraphy at both sample sites, indicate a latest Ludfordian age for the upper Słupianka Member (Kozłowski & Munnecke, Reference Kozłowski and Munnecke2010; Kaljo et al. Reference Kaljo, Einasto, Martma, Märss, Nestor and Viira2015). Acastella prima has been found above the sample bed in the Winnica section (Bodzioch, Kozłowski & Popławska, Reference Bodzioch, Kozłowski and Popławska2003), and some metres below the sample level in the Rzepin section (Kozłowski, Reference Kozłowski2008). Carbon isotope values also indicate a slightly lower stratigraphical position of the Winnica samples compared to the Rzepin sample. The lack of N. gracilis scales in the Winnica samples could then be the result of a slightly lower stratigraphical position, or the difference in facies as observed by Valiukevičius (Reference Valiukevičius2006). Nostolepis gracilis have not been reported from the partially coeval shallow-marine beds on Gotland, which could further indicate a difference in occurrence of this taxon between northern and southern parts of the Baltic Basin. It could also indicate a slightly younger age of the JB142 sample from the uppermost Słupianka Member in the Rzepin section compared to Gotland, which was suggested by Kozłowski & Munnecke (Reference Kozłowski and Munnecke2010). Indeed, the uppermost Sundre Formation only seem to reach the equivalent level of lowermost Kuressaare Formation (Kaljo & Martma Reference Kaljo and Martma2006; Kaljo et al. Reference Kaljo, Einasto, Martma, Märss, Nestor and Viira2015) and therefore could not represent the latest Ludfordian. This is further supported by the lack of ostracods indicative of the latest Ludfordian Neobeyrichia incerta Zone on Gotland (Perrier & Siveter, Reference Perrier, Siveter, Harper and Servais2013).
The difference in facies between the two sites seems to be the most plausible explanation for the high abundance of P. ludlowiensis in the Rzepin sample and its complete absence in the Winnica samples. The slight difference in stratigraphical level between the samples does not suffice as an explanation, since this taxon has been reported from older strata of the Eke and Burgsvik formations on Gotland (Eriksson, Nilsson & Jeppsson, Reference Eriksson, Nilsson and Jeppsson2009) and the Tahula Beds of the Kuressaare Regional Stage in Estonia (Märss & Miller, Reference Märss and Miller2004). Instead, it seems like P. ludlowiensis has a rather long temporal range but a restricted environmental distribution, as proposed by Kaljo et al. (Reference Kaljo, Einasto, Martma, Märss, Nestor and Viira2015). On Gotland, the taxon is most numerous in the Burgsvik Sandstone and overlying Burgsvik Oolite members, where it occurs together with T. sculptilis according to Eriksson, Nilsson & Jeppsson (Reference Eriksson, Nilsson and Jeppsson2009). Only one scale of P. ludlowiensis has been found in the Tahula Beds, which mainly consists of pure carbonates and where T. sculptilis is more abundant (Märss & Miller, Reference Märss and Miller2004; Märss & Männik, Reference Märss and Männik2013). Karatajūte-Talimaa & Brazauskas (Reference Karatajūte-Talimaa and Brazauskas1994) only found P. ludlowiensis at one level in the Virbalis-5 drill core from Lithuania, together with T. sculptilis and T. parvidens. Vergoossen (Reference Vergoossen2002c) reported two scales of P. ludlowiensis together with T. sculptilis from Ramsåsa D, southern Sweden, and six scales from a sandy limestone sample from the uppermost Öved Sandstone Formation at Ramsåsa C (Vergoossen Reference Vergoossen2003). Vergoossen (unpub. thesis, University of Groningen, Reference Vergoossen2003) claimed that it was more common in another sample (Ramsåsa SMNH-Q 607) from the Öved Sandstone Formation in the same area of Sweden, but the exact locality and lithology of this sample is not entirely clear. Vergoossen (unpub. thesis, University of Groningen, Reference Vergoossen2003) further noted that T. sculptilis was becoming less common as P. ludlowiensis increased in abundance, which might relate to the complete lack of the former in the Rzepin sample where P. ludlowiensis is so abundant. The youngest P. ludlowiensis scales were reported by Miller & Märss (Reference Miller and Märss1999) from lowermost Pridoli in the Welsh Borderlands, where both T. sculptilis and T. admirabilis are missing (see also Märss, Turner & Karatajūte-Talima, Reference Märss, Turner, Karatajūte-Talima and Schultze2007). According to Miller & Märss (Reference Miller and Märss1999), P. ludlowiensis dominated the microvertebrate faunas in two samples taken from calcareous, very fine sandstones. Märss & Miller (Reference Märss and Miller2004) only found P. ludlowiensis in samples from the Downtown Castle Sandstone Formation at the Ludlow–Pridoli boundary, but Miller & Märss (Reference Miller and Märss1999) reported its occurrence in unpublished data from the Upper Whitcliffe Formation (Ludlow). They explained the lack of P. ludlowiensis in the Pridoli of the Baltic Basin by a hiatus corresponding to the earliest Pridoli in this area. Turner (Reference Turner1973) reported P. ludlowiensis from the Wenlock Lower Woolhope Limestone (later questioned by Märss & Miller Reference Miller and Märss1999), as well as the top Sedgley Limestone bonebed (Leintwardine Formation) and Whitcliffe Beds of Ludlow age. The two latter occurrences were reported from bone beds that Turner (Reference Turner1973) described as ‘thin, calcareous, siltstone or sandstone bands’. The fact that P. ludlowiensis only occurs in the JB142 sample, taken from an oolite bed towards the top of the Rzepin section where clastic input increases in importance (subsequently leading to the deposition of the clastic Bronkowice Formation), indicates a similar environmental control on its occurrence here. This confirms the seemingly restricted environmental distribution of P. ludlowiensis suggested by Kaljo et al. (Reference Kaljo, Einasto, Martma, Märss, Nestor and Viira2015), largely confining it to mostly high-energy, very shallow-marine environments often with clastic input.
Thelodus parvidens can be used as a contrasting example to that of P. ludlowiensis. Scales belonging to this taxon are abundant at both sampled sites, and have been reported from a wide range of lithologies from the Lower Whitcliffe Formation (Märss & Miller, Reference Märss and Miller2004) and the uppermost Paadla Regional Stage of middle Ludlow age in Estonia (Märss, Reference Märss1986), to at least the upper Ohesaare Regional Stage of late Pridoli age (Märss & Männik, Reference Märss and Männik2013). The wide distribution of this taxon is also discussed in Kaljo et al. (Reference Kaljo, Einasto, Martma, Märss, Nestor and Viira2015).
The occurrences of the anaspid cf. Liivilepis and fragments similar to the osteostracan Tahulaspis ordinata agrees well with a placement of the sampled levels in the late Ludfordian (compare to stratigraphical charts in Blom, Märss & Miller, Reference Blom, Märss and Miller2002 and Märss, Afanassieva & Blom, Reference Märss, Afanassieva and Blom2014). The presence of osteostracan fragments only in the JB142 sample may also be the result of environmental control, as suggested in previous work (e.g. Sansom, Reference Sansom2008; Märss, Afanassieva & Blom, Reference Märss, Afanassieva and Blom2014; Sansom, Randle & Donoghue, Reference Sansom, Randle and Donoghue2015), but the overall rarity of these remains makes this less conclusive in the present study.
6. Conclusions
The Słupianka Member has previously been proposed as late Ludfordian in age based on sequence stratigraphy and chemostratigraphy (Kozłowski & Munnecke, Reference Kozłowski and Munnecke2010), and the Winnica section is viewed as largely coeval to the upper part of the Rzepin section (Kozłowski, Reference Kozłowski2008). The presence of trilobite A. prima (Kozłowski, Reference Kozłowski2003, Reference Kozłowski2008) and the ostracod faunas (Tomczykowa & Witwicka, Reference Tomczykowa and Witwicka1974; Tomczykowa, Reference Tomczykowa1991) at both sites seems to support this (Kaljo et al. Reference Kaljo, Einasto, Martma, Märss, Nestor and Viira2015). The uppermost part of the member has been correlated with the Sundre Formation on Gotland and the younger Beyrichienkalk (Kozłowski & Munnecke Reference Kozłowski and Munnecke2010). Thelodus sculptilis has been reported from Sundre Formation (Fredholm, Reference Fredholm1989), and the T. sculptilis biozone is considered as largely coeval with the late Ludfordian O. crispa and Oz. remsheidensis baccata – Oz. snajdri parasnajdri conodont zones (Märss & Männik, Reference Märss and Männik2013). However, this thelodont has not been found in the samples treated here. On the other hand, the acanthodian N. gracilis is present in the Rzepin sample, while this taxon has not been reported from Gotland. Nostolepis gracilis is an index taxon of early Pridoli age, but according to Märss & Männik (Reference Märss and Männik2013) the base of the N. gracilis Zone is defined as where this taxon starts to dominate the fauna, which is not the case in our sample. Furthermore, this taxon or a variant thereof (Märss & Männik, Reference Märss and Männik2013) has been reported from uppermost Ludfordian strata in eastern Lithuania (Valiukevičius, Reference Valiukevičius2005, Reference Valiukevičius2006) as well as in the northern Baltic (Märss & Männik, Reference Märss and Männik2013). The vertebrate faunas therefore do not impede previous suggestions of a late Ludfordian age for the uppermost Słupianka Member. However, there are some uncertainties regarding how the established vertebrate zones (largely based on East Baltic occurrences) corroborate to this part of the Baltic Basin and its depositional setting. Märss & Männik (Reference Märss and Männik2013) noted that the faunas of the late Ludlow – early Pridoli T. sculptilis and T. admirabilis zones are similar, but the scale frequencies tend to be lower in the latter (end Ludfordian) fauna. It also seems like faunas containing P. ludlowiensis are often dominated by this taxon (Miller & Märss, Reference Miller and Märss1999), which was also implied by Vergoossen (unpub. thesis, University of Groningen, Reference Vergoossen2003; Reference Vergoossen2003). This could explain the absence of these Thelodus taxa in the Rzepin sample, but additional sampling is needed to resolve this.
The presence of N. gracilis only at one site could be explained by the Winnica samples being slightly older or by the differences in depositional environments between the two sites, since Valiukevičius (Reference Valiukevičius2006) only found Ludfordian N. gracilis scales in shallower-water facies. The difference in facies seems like the most plausible explanation for the lower poracanthodid abundance and the comparably unique occurrence of abundant P. ludlowiensis scales in the JB142 sample from the Rzepin area. This lends support to the suggestion that the P. porosus Zone represents a deeper-water equivalent to the T. sculptilis, T. admirabilis and N. gracilis zones by Märss & Männik (Reference Märss and Männik2013). It also confirms the previous suggestion by Kaljo et al. (Reference Kaljo, Einasto, Martma, Märss, Nestor and Viira2015) that P. ludlowiensis has a rather long temporal range but restricted occurrence as a result of specific environmental preferences.
Additional material from both the Winnica and Rzepin sections is needed before the stratigraphical distribution of vertebrates discussed here can be confirmed. This preliminary study does not suffice to definitely disprove or confirm the proposed late Ludfordian age of the Słupianka Member, but it does lend some support to previous correlations. Further sampling may also enable investigations of conodonts, which could help elucidate some of the biostratigraphical concerns flagged here. However, it is clear that some Silurian vertebrates show tendencies towards specific environmental preferences. An increased understanding of this may prove important for understanding early vertebrate distribution patterns and to further test the vertebrate biozonation of the Silurian.
Acknowledgements
The authors would like to thank Helena Malmikumpu (Uppsala University) for help with setting up a heavy liquid separation laboratory at the Department of Organismal Biology in Uppsala, and Miloš Bartol for aiding us with the SEM in Uppsala. We would also like to thank Tiiu Märss for her help with vertebrate identifications in this work, and the reviewers for helpful comments that improved this paper. Part of this work was funded by a travelling grant from C. F. Liljewalchs stipendiestiftelse.
Supplementary material
To view supplementary material for this article, please visit https://doi.org/10.1017/S0016756817000681.