INTRODUCTION
One mechanism adopted by helminth parasites to resist the action of anthelmintics is the enhanced activity of drug efflux pumps, linked to transporter molecules such as P-glycoprotein (Pgp) (Kerboeuf et al. Reference Kerboeuf, Blackhall, Kaminsky and Von Samson-Himmelstjerna2003a ; Wolstenholme et al. Reference Wolstenholme, Fairweather, Prichard, Von Samson-Himmelstjerna and Sangster2004; Prichard and Roulet, Reference Prichard and Roulet2007; Lespine et al. Reference Lespine, Ménez, Bourguinat and Prichard2012). The phenomenon is well documented in nematodes (e.g. Xu et al. Reference Xu, Molento, Blackhall, Riberio, Beech and Prichard1998; Molento and Prichard, Reference Molento and Prichard1999; Stitt et al. Reference Stitt, Tompkins, Dooley and Ardelli2011; Tompkins et al. Reference Tompkins, Stitt, Morrissette and Ardelli2011). Among trematodes, over-expression of Pgp in schistosomes has been linked to resistance against praziquantel (James et al. Reference James, Hudson and Davey2009; Messerli et al. Reference Messerli, Kasinathan, Morgan, Spranger and Greenberg2009; Kasinathan et al. Reference Kasinathan, Goronga, Messerli, Webb and Greenberg2010a , Reference Kasinathan, Morgan and Greenberg b , Reference Kasinathan, Morgan and Greenberg2011; Kasinathan and Greenberg, Reference Kasinathan and Greenberg2012).
This study is on another trematode, Fasciola hepatica, and is part of a wider investigation examining the role of altered Pgp activity in the development of resistance to triclabendazole (TCBZ). It has been shown that the concentrations of TCBZ and its sulphoxide metabolite (TCBZ.SO) are lower in the TCBZ-resistant (TCBZ-R) Sligo isolate than in the TCBZ-susceptible (TCBZ-S) Cullompton isolate following incubation of flukes in the two compounds, a result which was attributed to an increased expression and action of Pgp-linked efflux pumps (Alvarez et al. Reference Alvarez, Solana, Mottier, Virkel, Fairweather and Lanusse2005; Mottier et al. Reference Mottier, Alvarez, Fairweather and Lanusse2006). The effect was reversed by co-incubation with Pgp inhibitors and the response was specific to TCBZ, as it was not evident with albendazole, a related benzimidazole drug (Mottier et al. Reference Mottier, Alvarez, Fairweather and Lanusse2006). In another TCBZ-R isolate, the Dutch isolate, an amino acid substitution in a Pgp gene has been identified and it may be linked to TCBZ resistance (Wilkinson et al. Reference Wilkinson, Law, Hoey, Fairweather, Brennan and Trudgett2012). Two previous scanning electron microscope (SEM) studies involving TCBZ-R flukes (the Oberon and Sligo isolates) have shown that co-incubation of flukes in TCBZ.SO + the Pgp inhibitor, R(+)-verapamil [R(+)-VPL] led to greater surface disruption of the flukes than treatment with TCBZ.SO alone (Meaney et al. Reference Meaney, Savage, Brennan, Hoey, Trudgett and Fairweather2013; Savage et al. Reference Savage, Meaney, Brennan, Hoey, Trudgett and Fairweather2013a ). A similar potentiation of drug activity was not seen with the Cullompton isolate. Moreover, the combination treatment significantly reduced the time taken for TCBZ.SO to immobilize the flukes, particularly in the TCBZ-R isolates (Meaney et al. Reference Meaney, Savage, Brennan, Hoey, Trudgett and Fairweather2013; Savage et al. Reference Savage, Meaney, Brennan, Hoey, Trudgett and Fairweather2013a ). A follow-up transmission electron microscope (TEM) study on the Sligo isolate determined the internal changes in the tegument (and gut) that caused the changes visible externally and the results supported the conclusion to the SEM study, in terms of the enhancement of drug action (Savage et al. Reference Savage, Meaney, Brennan, Hoey, Trudgett and Fairweather2013b ). The present study focuses on two key components of the reproductive system, namely, the testis and vitellaria. They are particularly sensitive to drug action and have often been used as models of drug action (see Toner et al. Reference Toner, Brennan, Hanna, Edgar and Fairweather2011a , Reference Toner, Brennan, Hanna, Edgar and Fairweather b for references). The results highlight the impact of Pgp inhibition on the reproductive potential of TCBZ-treated TCBZ-R flukes.
The Pgp inhibitor chosen was (R+)-VPL. VPL is an established inhibitor of Pgp-mediated drug efflux (Pereira et al. Reference Pereira, Teodori, Dei, Gualtieri and Garnier-Suillerot1995; Toffoli et al. Reference Toffoli, Simone, Corona, Raschack, Cappelletto, Gigante and Boiocchi1995; Tiberghien and Loor, Reference Tiberghien and Loor1996; Choi et al. Reference Choi, Lee, Kim, Choi, Park, Shin, Yoo, Jung and Lee1998; Biscardi et al. Reference Biscardi, Teodori, Caporale, Budriesi, Balestri, Scappini, Gavazzi and Grossi2006). The R(+) isomer was used rather than the S(−) isomer of VPL or racemic VPL [a mixture of (+) and (−) isomers]. This was because it is equally or more specific for Pgp than the other two forms of VPL but, more importantly, it is 10-fold less active as a calcium channel antagonist than S(−)-VPL (Echizen et al. Reference Echizen, Brecht, Niedergesass, Vogelgesang and Eichelbaum1985; Ye and Van Dyke, Reference Ye and Van Dyke1988; Höllt et al. Reference Höllt, Kouba, Dietel and Vogt1992; Varma et al. Reference Varma, Ashkraj, Dey and Panchagnula2003). In terms of avoiding changes to fluke motility (which, in turn, might impact on morphology), this was an important precaution to take, as racemic VPL at an equivalent concentration (100 μ m) has been shown to induce paralysis of the whole fluke (Holmes, Reference Holmes1983; Chapter 6, figs 6·5 and 6·7) and affect the contractility of isolated fluke muscle strips (Wells, Reference Wells2008; Chapter 5, fig. 5·4). In contrast, R(+)-VPL did not affect motility over a 24-h period (Savage et al. Reference Savage, Meaney, Brennan, Hoey, Trudgett and Fairweather2013a ).
MATERIALS AND METHODS
The protocol for this investigation was the same as that used for the TEM study that focused on the tegument and gut of F. hepatica following Pgp inhibition (Savage et al. Reference Savage, Meaney, Brennan, Hoey, Trudgett and Fairweather2013b ); the reader is referred to that publication for full details. Briefly, adult flukes of the Sligo TCBZ-R isolate were used for the experiments; for the provenance of the Sligo isolate, see the review by Fairweather (Reference Fairweather2011). Adult flukes were recovered from the bile duct of infected rats under sterile conditions in a laminar flow cabinet. Flukes were then washed in several changes of warm (37 °C) sterile NCTC 135 culture medium containing antibiotics (penicillin 50 IU mL−1; streptomycin, 50 μg mL−1) (NCTC 135 was obtained from Flow laboratories, Thame, Oxfordshire, UK; antibiotics were obtained from Sigma-Aldrich Co. Ltd., Poole, Dorset, UK). They were then subjected to one of three different drug treatments, as indicated below. Six flukes were incubated per treatment, with 4 being prepared for TEM and 2 for light microscopy.
In the first experiment, flukes were initially incubated for 2 h at 37 °C in R(+)-VPL (100 μ m) alone, then incubated in a combination of R(+)-VPL (100 μ m)+triclabendazole sulphoxide (TCBZ.SO) (at a concentration of 50 μg mL−1, or 133·1 μ m) until flukes ceased movement (at 9 h post-treatment: Savage et al. Reference Savage, Meaney, Brennan, Hoey, Trudgett and Fairweather2013a ). TCBZ.SO was initially prepared as a stock solution in dimethyl sulphoxide (DMSO) and added to the culture medium to give a final solvent concentration of 0·1% (v/v); a stock solution of R(+)-VPL was initially prepared at a concentration of 1×10−1 m in distilled water.
In the second experiment, flukes were incubated at 37 °C in NCTC containing TCBZ.SO on its own at a concentration of 50 μg mL−1 and then removed from the incubation medium following cessation of motility (after 15 h: Savage et al. Reference Savage, Meaney, Brennan, Hoey, Trudgett and Fairweather2013a ). A concentration of 50 μg mL−1 is the highest concentration that has been used in in vitro experiments and, at this concentration, the drug causes little disruption to tegumental morphology and no diminution of tubulin staining in the Sligo isolate over a 24-h period (Robinson et al. Reference Robinson, Trudgett, Hoey and Fairweather2002; see also Savage et al. Reference Savage, Meaney, Brennan, Hoey, Trudgett and Fairweather2013a , Reference Savage, Meaney, Brennan, Hoey, Trudgett and Fairweather b ).
In the third experiment, flukes were incubated at 37 °C for 24 h in NCTC containing 100 μ m R(+)-VPL on its own. At this concentration, there was no change in motility after 24 h incubation, nor any change in the fine structure of the tegument or gut (Savage et al. Reference Savage, Meaney, Brennan, Hoey, Trudgett and Fairweather2013a , Reference Savage, Meaney, Brennan, Hoey, Trudgett and Fairweather b ).
Untreated NCTC controls
Flukes were removed from the bile duct and briefly washed in warm (37 °C) sterile NCTC 135 culture medium (pH 7·4) containing antibiotics (penicillin, 50 IU mL−1; streptomycin, 50 μg mL−1). They were then incubated in NCTC culture medium containing 0·1% (v/v) DMSO at 37 °C for 24 h. 0 h controls were also prepared: the flukes were fixed immediately following their recovery from infected rats.
JB4 tissue preparation
Flukes were lightly flat-fixed for 30 min in 4% (w/v) paraformaldehyde (PFA) in 0·1 m phosphate buffered saline (PBS), pH 7·2, at room temperature. Each fluke was transected in three places to isolate the oral cone region, the anterior midbody region, the posterior midbody region and the tail region. Each section was free-fixed in 4% (w/v) PFA in 0·1 m PBS, pH 7·2, at room temperature for 4–6 h. The fluke sections were then washed overnight in 0·1 m PBS, pH 7·2, and dehydrated through an ascending series of alcohols. The sections were then infiltrated and embedded in JB4 resin. Sections 4 μm in thickness were cut on a pyramitome and mounted on multi-welled slides. The sections were stained with toluidine blue and examined under a light microscope.
Tissue preparation for transmission electron microscopy
Flukes were lightly flat-fixed for 1 h in 4% (w/v) glutaraldehyde in 0·1 m sodium cacodylate buffer (pH 7·4) containing 3% (w/v) sucrose at room temperature. Flukes were then sectioned into apical cone, midbody and tail regions. The apical cone region was divided into two parts, the midbody region was divided into the anterior and posterior midbody regions, and the tail region was sectioned into two parts. Each body section was further sub-divided into transverse sections approximately 2 mm in width. The sections were placed in fresh fixative for 3 h at room temperature and then washed in 0·1 m sodium cacodylate buffer (pH 7·4) containing 3% (w/v) sucrose and left overnight at 4 °C. Specimens were post-fixed in 1% osmium tetroxide for 1 h, washed in fresh buffer, dehydrated through an ascending series of ethanol and infiltrated and embedded in agar resin. Ultrathin sections, 60–70 nm in thickness, were cut on a Reichert Ultracut E ultramicrotome, placed onto sterile 200-mesh copper grids and stained with uranyl acetate (8 min) and lead citrate (5 min). Sections were examined in an FEI CM100 TEM which was operated at 100 keV.
RESULTS
Testis histology
For those readers not familiar with the characteristic features of the different stages in sperm formation, a brief description will be included here. There are three generations of spermatogonia, the tertiary spermatogonia forming a group of four cells, though they are not joined together. The spermatogonial cells typically lie at the periphery of the testis tubule, have a high nucleo-cytoplasmic ratio and are highly basophilic. Division of the tertiary spermatogonia produces a rosette of eight primary spermatocytes, joined together by a common cytoplasm. A distinctive feature of these cells at the EM level is the presence of synaptonemal complexes in the nucleus; they represent the pairing of homologous chromosomes at zygotene during meiotic prophase. The 16-cell secondary spermatocyte stage (also a rosette) is rarely seen. Once meiosis is complete, the 32-cell spermatid stage is reached. These cells undergo marked changes to form the spermatozoa: the changes include the elongation and differentiation of the nucleus and the formation of the axonemes that come to lie within the spermatozoa. Once formed, the latter break away from the residual cytoplasm and lie free within the lumen of the testis tubule. For a more detailed description of the various cell types, see Stitt and Fairweather (Reference Stitt and Fairweather1990).
The histological profile of the testis of untreated 0 and 24 h control flukes from the TCBZ-resistant Sligo isolate showed a normal morphology, corresponding to that previously described by Hanna et al. (Reference Hanna, Edgar, Moffett, McConnell, Fairweather, Brennan, Trudgett, Hoey, Cromie, Taylor and Daniel2008, Figs 3d and e; see also McConville et al. Reference McConville, Hanna, Brennan, McCoy, Edgar, McConnell, Castillo, Hernández-Campos and Fairweather2010; Hanna et al. Reference Hanna, Forster, Brennan and Fairweather2013). All cell types (from spermatogonia to spermatozoa) were present within the tubule. Primary and secondary spermatogonia were present at the periphery of the tubule, and tertiary spermatogonia remained in close proximity. The later stages of spermatogenesis, namely, the primary spermatocytes, spermatids and spermatozoa were more centrally located in the tubule (Fig. 1A). Therefore, no abnormalities were observed following in vitro incubation. After 24 h incubation, R(+)-VPL (100 μ m)-treated flukes appeared to have a normal testis profile, with all stages of spermatogenesis present, including mature spermatozoa and an abundance of primary and secondary spermatogonia at the periphery of the tubule (Fig. 1B). After treatment with TCBZ.SO (50 μg mL−1) until inactivity at 15 h, flukes showed a normal testis profile, with all stages of spermatogenesis present in the tubules. An abundance of primary and secondary spermatogonia were located at the periphery of the tubule, and many mature spermatozoa were seen throughout the tubules (Fig. 1C and D). After treatment with a combination of R(+)-VPL (100 μ m)+TCBZ.SO (50 μg mL−1) until inactivity at 9 h, the testis tubules showed a marked reduction in the number of cells generally, resulting in an increase in ‘empty’ space within the tubule. There were few spermatogonia present. The intermediate stages of sperm development, viz. primary and secondary spermatocytes, were present. Apoptotic bodies containing irregular clumps of dense chromatin were present in the tubules; these bodies are due to missegregation during meiotic division in primary spermatocytes, which leads to apoptosis and ultimately cell death (Kumar et al. Reference Kumar, Abbas and Fausto2005). No spermatids or mature spermatozoa were visible in the testis tubule after combination treatment (Fig. 1E and F).
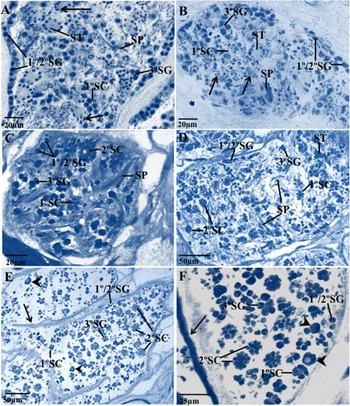
Fig. 1. Light micrographs of Fasciola hepatica (Sligo isolate) showing profiles of testis tubules of an untreated specimen (A); a fluke following in vitro treatment for 24 h with R(+)-verapamil (100 μ m) (B); flukes following incubation in vitro for 15 h in TCBZ.SO (50 μg mL−1) (C and D); and specimens treated in vitro with a combination of R(+)-verapamil (100 μ m)+TCBZ.SO (50 μg mL−1) until inactivity at 9 h (E and F). (A) Control fluke image. Primary (1°SG) and secondary (2°SG) spermatogonia are located at the periphery of the testis tubule, and numerous tertiary (3°SG) spermatogonia are present. Few primary (1°SC) spermatocytes are evident. Spermatids (ST) are numerous, with evidence of nuclear elongation (arrows). SP, mature spermatozoa. (B) R(+)-verapamil-treated fluke. Testis tubule showing peripherally located primary (1°SG) and secondary (2°SG) spermatogonia, also tertiary (3°SG) spermatogonia. Spermatid clusters (ST) are present and spermatids at various stages of maturation and showing elongation of the nuclei (arrows) can also be seen. 1°SC, primary spermatocytes; SP, mature spermatozoa. (C) TCBZ.SO-treated fluke. Primary (1°SG) and secondary (2°SG) spermatogonia are located at the periphery of the tubule. Primary (1°SC) and secondary (2°SC) spermatocytes are more centrally located. Mature spermatozoa (SP) are present in close parallel bundles throughout the tubule. 3°SG, tertiary spermatogonia. (D) TCBZ.SO-treated fluke. Primary (1°SG) and secondary (2°SG) spermatogonia are present in close proximity to the peripheral wall. Tertiary spermatogonia (3°SG) are numerous and located in the central tubule region. Mature spermatozoa (SP) are also numerous and are located throughout the tubule. 1°SC, primary spermatocytes; 2°SC, secondary spermatocytes. (E) Combination-treated fluke. Few primary (1°SG) and secondary (2°SG) spermatogonia are visible along the tubule wall (arrow) and only a small number of tertiary spermatogonia (3°SG) can be seen. Large apoptotic-like bodies can be seen in the tubule (arrowheads). No mature spermatozoa were visible in the tubule. 1°SC, primary spermatocytes; 2°SC, secondary spermatocytes. (F) Combination-treated fluke. Few primary (1°SG) and secondary (2°SG) spermatogonia are visible along the tubule wall (arrow). Tertiary (3°SG) spermatogonia are present in the central region of the tubule. Primary (1°SC) and secondary (2°SC) spermatocytes are present, but no mature spermatozoa or spermatids are visible. Large apoptotic-like bodies are present in the tubule (arrowheads).
Testis ultrastructure
The ultrastructure of the testis in the 24 h control specimens retained a normal morphology (and was similar to the 0 h controls): it corresponded to the images presented by Stitt and Fairweather (Reference Stitt and Fairweather1990) (Figs 1–6) and by McConville et al. (Reference McConville, Hanna, Brennan, McCoy, Edgar, McConnell, Castillo, Hernández-Campos and Fairweather2010, Figs 5–7). Thus, no abnormalities in the spermatogenic cells of F. hepatica were observed following incubation in vitro.
Treatment for 24 h with R(+)-VPL (100 μM)
All stages of sperm development were present. Primary and secondary spermatogonia were abundant and situated in layers beneath the peripheral wall of the tubule (Fig. 2A). Primary spermatocytes retained a normal morphology, and synaptonemal complexes were present, indicating synapsis of meiosis at early prophase (Fig. 2B and C). Clusters of secondary spermatocyte cells were present, showing the typical reduction in the nucleo-cytoplasmic ratio, and the cells were connected via cytoplasmic bridges (Fig. 2D). The spermatid cells contained elongated nuclei with chromatin forming lamellar-like sheets. The accompanying zone of differentiation appeared normal, with a typical layer of microtubules beneath the plasma membrane (PM) (Fig. 2E). Sections of axonemes lying free in the lumen of the tubule showed that they possessed an arrangement of nine microtubules surrounding a single central element, which is typical of the spermatozoa in parasitic platyhelminths (Fig. 2F).
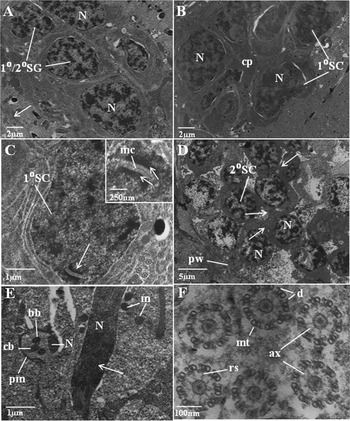
Fig. 2. Transmission electron micrographs (TEMs) showing profiles of testis tubules of Fasciola hepatica (Sligo isolate) following in vitro treatment for 24 h with R(+)-verapamil (100 μ m). (A) A TEM showing a group of spermatogonia (1°/2°SG) in close proximity to the wall (arrowed) of the testis tubule. N, nucleus. (B) A TEM of a group of primary spermatocytes (1°SC), which are joined together by the central cytophore (cp). N, nucleus. (C) TEM of the nucleus of an individual primary spermatocyte (1°SC) containing a visible synaptonemal complex (arrow). Inset shows a high magnification image of the synaptonemal complex, which consists of two homologous chromosomes separated by a medial complex (mc) and with heterochromatin (arrows) visible on either side of the complex. (D) A TEM showing a cluster of secondary spermatocyte (2°SC) cells, with cytoplasmic bridges (arrows) connecting the cells. Pw, peripheral wall of the tubule; N, nucleus. (E) A TEM of a spermatid cell showing the elongation of the nucleus (N), and the chromatin within the nucleus which is organized into lamellar-like sheets (arrow). Basal bodies (bb), a central body (cb) and mitochondria (m) are also evident. A single row of peripheral microtubules lies beneath the plasma membrane (PM). (F) A TEM of a cross-section of six axonemes (ax), each possessing 9 sets of outer doublet microtubules (mt) arranged around a central element. Dynein side-arms (d) are visible on the outer microtubules, and radial spokes (rs) connect the microtubules to the central element.
Treatment until inactivity at 15 h with TCBZ.SO (50 μg mL−1)
The spermatogonia located beneath the peripheral wall of the tubule appeared normal and showed a typically high nucleo-cytoplasmic ratio (Fig. 3A). Primary spermatocytes were present in the tubule and mitochondria appeared to be congregated at the edge of the cell cluster (Fig. 3B). Secondary spermatocytes were typically located at the centre of the tubule and numerous cytoplasmic bridges connecting the cells were visible (Fig. 3C). Differentiation of the spermatid cells was evident, the cells containing elongated nuclei which were located at the periphery of the cell mass and whose content appeared to be condensed. However, in some regions the nuclei were clustered together and spermatid elongation was disrupted. The mitochondria appeared normal and were congregated at the edge of the spermatid cell cluster (Fig. 3D). Mature spermatozoa were abundant within the tubule (Fig. 3D–F). Cross-sections of the mature spermatozoa showed that the nucleus and axonemes were present, but that mitochondria were not visible (Fig. 3E). In a different region of the tubule, the axonemes and cortical microtubules were evident in the mature spermatozoa, but their mitochondria were disrupted (Fig. 3F).

Fig. 3. Transmission electron micrographs (TEMs) showing profiles of testis tubules of Fasciola hepatica (Sligo isolate) following in vitro treatment with TCBZ.SO (50 μg mL−1) until inactivity at 15 h. (A) TEM of a group of secondary spermatogonia (2°SG) located near to the wall (arrow) of the testis tubule, showing the typically high nucleocytoplasmic ratio. N, nucleus. (B) TEM showing a group of primary spermatocytes (1°SC). Mitochondria (m) are present in the cytoplasm of the cells at the edge of the cell cluster and appear to be normal. (C) TEM showing a cluster of secondary spermatocyte (2°SC) cells, with numerous cytoplasmic bridges (arrows) connecting them. N, nucleus. (D) TEM showing a section through a rosette of spermatid (ST) cells. Elongated nuclei (N) are visible and elongation of individual spermatid cells has occurred. Mature spermatozoa (SP) are visible at the edge of the spermatid rosette. The mitochondria (m) appear normal. (E) TEM showing transverse sections of mature spermatozoa (SP), containing nuclei (N), axonemes (ax) and cortical microtubules (arrow). Free axonemes are also visible in the lumen of the tubule. (F) TEM showing transverse sections of mature spermatozoa (SP). Axonemes (ax) and cortical microtubules (arrows) are visible within the spermatozoa. The mitochondria (m) in the spermatozoa appear to be disrupted and no nuclei are visible.
Treatment until inactivity at 9 h with a combination of TCBZ.SO (50 μg mL−1) and R(+)-VPL (100 μM)
Disruption was observed at all stages of spermatogenesis and discrete cell stages were often difficult to distinguish. The number of primary and secondary spermatogonia close to the peripheral wall of the tubule was low and disruption was evident to the nuclear and cell membranes. The membranes had little definition and the cell membrane was ruptured in places (Fig. 4A). The breakdown of the nuclear membrane and of the chromatin within the nucleus suggest that the cells were undergoing karyorrhexis. The primary spermatocytes were irregular in appearance, with disrupted nuclei, which were misshapen and the chromatin clumped, exemplifying pyknosis. The cell membrane of a primary spermatocyte was ruptured in one region. Electron-lucent areas were present in the cytoplasm and the mitochondria were swollen (Fig. 4B). Spermatid development appeared to be disrupted, in that the chromatin in the nucleus was loosely arranged into lamellar-like sheets, but elongation of the cell body was not apparent. Formation of the zone of differentiation was observed, which was separated from the main body of the spermatid cluster by a distinct collar (Fig. 4C). Axonemes were visible and seen to be extending away from the basal bodies in this zone (Fig. 4C). Late spermatid development was evident in the tubule. Within the median processes of the developing spermatozoa, the arrangement of microtubules appeared to be normal, although patches of electron-lucent material were present, suggesting possible nuclear or mitochondrial breakdown. The median processes and the axonemes appeared to be distinct from each other (Fig. 4D). Mature spermatozoa were severely disrupted throughout the follicle. The normal doublet arrangement of axonemes within a mature spermatozoon was not the general rule, but rather the presence of multi-axonemal structures, which were widespread throughout the follicle; these multi-axonemal structures often contained electron-lucent mitochondria and nuclei with a very granular appearance (Fig. 4E). In some regions of the tubule, mature spermatozoa appeared to be extensively swollen, with electron-lucent nuclei, although the axonemes within the spermatozoa appeared to have the normal ‘9+1’ arrangement of microtubules. The mitochondria were abnormally large and electron-lucent. The nucleus of the spermatozoon was visible between the two axonemes and appeared to be irregular in shape (Fig. 4F). A multi-axonemal structure was observed in close proximity to the mature spermatozoon (Fig. 4F).
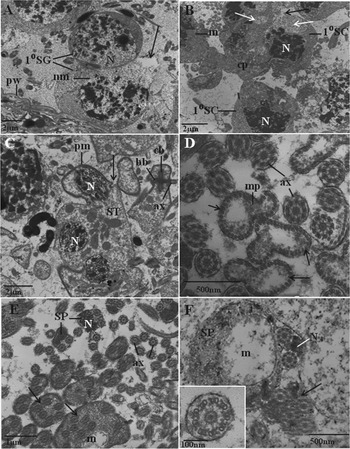
Fig. 4. Transmission electron micrographs (TEMs) showing profiles of testis tubules of Fasciola hepatica (Sligo isolate) following in vitro treatment with a combination of R(+)-verapamil (100 μ m)+TCBZ.SO (50 μg mL−1) until inactivity at 9 h. (A) TEM showing a cross-section of two primary spermatogonial (1°SG) cells in close proximity to the peripheral wall (pw) of the testis tubule. Disruption to the nucleus (N) is evident, with loss of definition of the nuclear membrane (nm). The cell membrane has ruptured (arrow) and the cytoplasm is electron-lucent in this region. (B) TEM showing a cluster of primary spermatocytes (1°SC) containing irregular nuclei (N) with dense chromatin. The cell membrane appears to have ruptured (black arrow) in one region, and large electron-lucent areas (white arrows) are present within the cytoplasm. The mitochondria (m) appear rounded and swollen. Cp, cytophore. (C) TEM of a cross-section of an abnormal cluster of spermatid (ST) cells. The chromatin within the nucleus (N) appears to be loosely arranged into lamellar-like sheets, but elongation of the nucleus is not evident. The plasma membrane (pm) appears thickened and invaginated to form a groove-like collar (arrow). The zone of differentiation of a spermatid cell is visible, with one axoneme (ax) extending away from its basal body (bb); the two basal bodies are situated on either side of the central body (cb). (D) TEM showing a cross-section through the median process (mp) and axonemes (ax) which are separate from one another. The median process (mp) is lined with cortical microtubules (arrows). The centre of the median process appears to be very electron-lucent. A large number of free axonemes is present in close proximity to the median processes. (E) TEM showing a cluster of spermatozoa in various abnormal states. Multi-axonemal spermatozoa (arrows) are present, some of which contain disrupted, electron-lucent mitochondria (m). Multiple free axonemes (ax) are also visible. Other spermatozoa (SP) contain a nucleus (N) which is irregular in shape and its content is abnormally granular. (F) A cross-section of a mature spermatozoon (SP) which appears to be extremely swollen and contains an abnormally large electron-lucent mitochondrion (m). A multi-axonemal spermatozoon (arrow) is also present. Inset shows a high-power micrograph of an individual axoneme, which possesses the normal 9+1 arrangement of microtubules. N, nucleus.
Vitelline ultrastructure
The ultrastructure of the vitellaria in the 24 h control specimens was normal (and similar to the 0 h controls), as described by Irwin and Threadgold (Reference Irwin and Threadgold1970) and Colhoun et al. (Reference Colhoun, Fairweather and Brennan1998). Thus, no abnormalities were noted following incubation in vitro.
Treatment for 24 h with R(+)-VPL (100 μM)
The morphology of the vitelline follicles appeared to be normal after treatment, as they contained a normal heterogeneous population of stem cells, nurse cells, intermediate type 1 (It1) and type 2 (It2) cells and mature cells. Nurse cells and stem cells were present at the periphery of the follicle, whilst mature stages were more centrally located within the follicle (Fig. 5A and B).
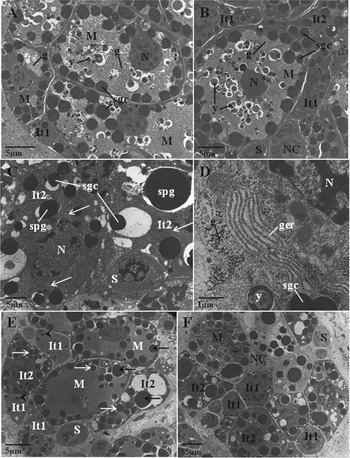
Fig. 5. Transmission electron micrographs (TEMs) showing the vitelline follicles of Fasciola hepatica (Sligo isolate) following in vitro treatment with R(+)-verapamil (100 μ m) for 24 h (A and B), and TCBZ.SO (50 μg mL−1) until inactivity at 15 h (C-F). (A) R(+)-verapamil-treated fluke. A low-power TEM of a vitelline follicle containing mature vitelline cells (M) and an intermediate type 1 cell (It1). Shell globule clusters (sgc), glycogen (g) and yolk globules (y) are present in the mature cells, and eggshell globule clusters are present in the It1 cell. N, nucleus. (B) R(+)-verapamil-treated fluke. A TEM showing a vitelline follicle containing all stages of cell development. A mature cell (M) and a number of intermediate type 1 (It1) and type 2 (It2) vitelline cells are present, along with a stem cell (S) and a nurse cell (NC). Shell globule clusters (sgc), glycogen granules (g), a nucleus (N) and yolk globules (y) are all visible in the mature cell. Shell globule clusters are also present in the It1 and It2 cells. (C) TCBZ.SO-treated fluke. A TEM showing intermediate type 2 (It2) vitelline cells containing large single shell protein globules (spg) and small shell globule clusters (sgc). Swelling (arrows) of the cisternae of granular endoplasmic reticulum is evident in the It2 cells. S, stem cell. (D) TCBZ.SO-treated fluke. A high-power TEM showing swelling of the cisternae of granular endoplasmic reticulum (ger) in a mature vitelline cell. A shell globule cluster (sgc) is present, as are glycogen granules (g) and yolk globules (y). N, nucleus. (E) TCBZ.SO-treated fluke. A TEM of a vitelline follicle containing mature cells (M) at the centre of the follicle, and intermediate type 1 (It1) and type 2 (It2) cells and a stem cell (S) at the periphery of the follicle. Large shell protein globules (black arrows) and small shell globule clusters (white arrows) are present in mature and It2 cells, while only small, single shell protein globules (arrowheads) are present in It1 cells. (F) TCBZ.SO-treated fluke. A low-power TEM showing an abnormal distribution of cells within a vitelline follicle. A nurse cell (NC) and It1 (It1) cells are situated in the centre of the follicle, whilst It2 (It2) and mature (M) cells are situated at the periphery. S, stem cell.
Treatment until inactivity at 15 h with TCBZ.SO (50 μg mL−1)
The morphology of the vitelline follicle had changed slightly after treatment. Swelling of the cisternae of the granular endoplasmic reticulum was evident in the It2 cells and large single shell protein globules were present. The stem cells appeared normal (Fig. 5C). The mature vitelline cells contained glycogen deposits, yolk globules and small shell globule clusters, which appeared normal, although the cisternae of the granular endoplasmic reticulum were swollen (Fig. 5D). The organization of the vitelline follicles generally appeared normal, with mature cells located in the central region, whilst nurse cells, stem cells and It1 and It2 cells were located more peripherally within the follicle (Fig. 5E). Only small, single shell protein globules were present in It1 cells (Fig. 5E) In some instances, the organization of cells in the follicle appeared abnormal, as nurse cells and It1 cells were unusually located in the centre of the follicle, with mature and It2 cells visible at the periphery of the follicle (Fig. 5F).
Treatment until inactivity at 9 h with a combination of TCBZ.SO (50 μg mL−1) and R(+)-VPL (100 μM)
Following combination drug treatment, the vitelline follicles were disrupted at all stages of development. However, not all stages were obviously disrupted in all follicles, as differences in disruption were observed both between and within follicles in the same cell stages. Large spaces were evident between cells, due to the separation of cells from the nurse cell cytoplasm. Large single shell protein globules were present in It1 cells and swelling of the cisternae of the granular endoplasmic reticulum was evident. The It1 cells also appeared to have a rounded, rather than elongated, shape (Fig. 6A). Similar features were observed in It2 cells, with swelling of the cisternae of the granular endoplasmic reticulum and large single shell protein globules present. The stem cells were round in shape (Fig. 6B). The mature cells were often highly disrupted, with extensive cytoplasmic breakdown evident throughout the cell, although the shell protein globules appeared to be normal and the shell globule clusters remained tightly packed; the yolk globules also appeared to be normal (Fig. 6C). Extreme swelling of the cisternae of the granular endoplasmic reticulum was evident in some It2 cells, although It1 cells in the same follicle appeared undisrupted. Large single shell protein globules were present in the It2 cells (Fig. 6D). There appeared to be relatively more It2 and mature cells in the follicles than usual.

Fig. 6. Transmission electron micrographs (TEMs) showing the vitelline follicles of Fasciola hepatica (Sligo isolate) following in vitro treatment with a combination of R(+)-verapamil (100 μ m)+TCBZ.SO (50 μg mL−1) until inactivity at 9 h. (A) TEM showing separation of the vitelline cells from the nurse cell processes (black arrows) which has resulted in large open spaces (arrowheads) within the follicle. Intermediate type 1 (It1) cells appear rounded and swelling of the cisternae of granular endoplasmic reticulum (white arrows) is evident within the It2 (It2) cell. Yolk globules (y) and shell protein globules (spg) are present within the mature cell (M). (B) A TEM showing separation of the vitelline cells from the nurse cell processes (black arrows) which has resulted in large open spaces (arrowheads) between the vitelline cells. Swelling of the cisternae of granular endoplasmic reticulum (white arrows) is visible in an intermediate type 2 (It2) cell. Large shell protein globules (spg) and shell globule clusters (sgc) are present in the mature (M) and It2 cells. It1, intermediate type 1 cell; S, stem cell; y, yolk globules. (C) TEM showing breakdown of the cytoplasm (black arrows) within a mature vitelline cell (M). Shell protein globules (spg), shell globule clusters (sgc) and yolk globules (y) remain intact. Slight swelling of the cisternae of the granular endoplasmic reticulum (white arrows) can be seen in intermediate type 2 (It2) cells. (D) A high-power TEM showing discrete differences in disruption within intermediate-type vitelline cells. There is extreme swelling of the cisternae of granular endoplasmic reticulum (arrows) in the It2 (It2) cell, whilst the granular endoplasmic reticulum appears normal in the adjacent It1 (It1) cell. N, nucleus.
Note
The changes seen in the spermatogenic and vitelline cells following the various treatments were consistent between specimens.
DISCUSSION
From the results of this investigation, it is evident that co-incubation of TCBZ.SO with the Pgp inhibitor, R(+)-VPL greatly increases the impact of TCBZ.SO on the reproductive tissues of the TCBZ-R Sligo isolate of F. hepatica. The combination also reduced the time taken for flukes to cease movement (Savage et al. Reference Savage, Meaney, Brennan, Hoey, Trudgett and Fairweather2013a ). The implications of the results for the mechanism of resistance to TCBZ and on the reproductive output potential of the fluke will be discussed below.
Taking the testis first, incubation in R(+)-VPL alone did not alter the morphology or relative numbers of spermatogenic cells. Treatment with TCBZ.SO on its own induced few changes to the cells and no diminution of the cell population within the tubule. Sperm formation continued, although on occasion spermatid elongation was affected, as were the mitochondria within the spermatozoa. However, there was a sharp drop in the number of cells in the testis tubule following co-incubation in TCBZ.SO+R(+)-VPL, with few spermatogonia and apoptotic spermatocytes; the latter are equivalent to the eosinophilic, multinucleate cells initially identified by Hanna et al. (Reference Hanna, Edgar, Moffett, McConnell, Fairweather, Brennan, Trudgett, Hoey, Cromie, Taylor and Daniel2008) and also described by Hanna et al. (Reference Hanna, Edgar, McConnell, Toner, McConville, Brennan, Devine, Flanagan, Halferty, Meaney, Shaw, Moffett, McCoy and Fairweather2010, Reference Hanna, Scarcella, Solana, McConnell and Fairweather2012a , Reference Hanna, Forster, Brennan and Fairweather2013), McConville et al. (Reference McConville, Hanna, Brennan, McCoy, Edgar, McConnell, Castillo, Hernández-Campos and Fairweather2010), Scarcella et al. (Reference Scarcella, Fiel, Guzman, Alzola, Felipe, Hanna, Fairweather, McConnell and Solana2011) and Toner et al. (Reference Toner, Brennan, Hanna, Edgar and Fairweather2011b ). What spermatids and spermatozoa were present were abnormal. Typically, the sperm were multi-axonemal, often swollen and their nuclei and mitochondria were electron-lucent.
The block in mitotic division of the spermatogenic cells and formation of aberrant sperm are changes typical of microtubule inhibition and compatible with the presumed anti-microtubule action of TCBZ. Similar changes have been observed in TCBZ-S flukes following incubation in TCBZ.SO in vitro (Stitt and Fairweather, Reference Stitt and Fairweather1992) and following treatment with TCBZ in vivo (Toner et al. Reference Toner, Brennan, Hanna, Edgar and Fairweather2011b ). The changes have also been seen in experiments with microtubule inhibitors and the TCBZ analogue, compound alpha (Stitt and Fairweather, Reference Stitt and Fairweather1992; McConville et al. Reference McConville, Hanna, Brennan, McCoy, Edgar, McConnell, Castillo, Hernández-Campos and Fairweather2010). However, equivalent changes have not been seen in TCBZ-R flukes (Hanna et al. Reference Hanna, Edgar, McConnell, Toner, McConville, Brennan, Devine, Flanagan, Halferty, Meaney, Shaw, Moffett, McCoy and Fairweather2010, Reference Hanna, Forster, Brennan and Fairweather2013). This suggests that Pgp inhibition has allowed the normal action of TCBZ to become apparent, leading to the apoptotic breakdown of the spermatogenic cells. Other flukicides and drugs induce different changes to the spermatogenic cells, a point discussed in more detail by McConville et al. (Reference McConville, Hanna, Brennan, McCoy, Edgar, McConnell, Castillo, Hernández-Campos and Fairweather2010) and Toner et al. (Reference Toner, Brennan, Hanna, Edgar and Fairweather2011b ).
Turning to the vitellaria, incubation in R(+)-VPL on its own did not alter the morphology of the vitelline cells, the cell composition of the follicle or the overall ratio of different cell stages within the follicle. Few changes were seen after treatment with TCBZ.SO alone: e.g. a swelling of the cisternae of the granular endoplasmic reticulum and abnormal packaging of the shell protein globule. Far more severe changes were seen in the combination-treated flukes, involving all cell types and large spaces were evident between the cells. There was a shift in the cell population towards the later developmental stages and the mature cells were undergoing cytoplasmic breakdown.
The changes seen in the vitelline follicles of combination-treated TCBZ-R flukes were similar to those evident following treatment of TCBZ-S isolates with TCBZ.SO in vitro (Stitt and Fairweather, Reference Stitt and Fairweather1996) and TCBZ in vivo (Toner et al. Reference Toner, Brennan, Hanna, Edgar and Fairweather2011a ). They are representative of microtubule disruption and, as with the changes in the testis, the drug sensitivity of the Sligo isolate has been changed to a more susceptible state by co-incubation with a Pgp inhibitor. Other drugs induce different changes to the vitellaria (Fairweather et al. Reference Fairweather, Anderson and Threadgold1988; Skuce and Fairweather, Reference Skuce and Fairweather1988; Stitt and Fairweather, Reference Stitt and Fairweather1991; Colhoun et al. Reference Colhoun, Fairweather and Brennan1998; Toner et al. Reference Toner, Brennan, Wells, McGeown and Fairweather2008; Halferty et al. Reference Halferty, O'Neill, Brennan, Keiser and Fairweather2009). So, the changes seen in the current study with respect to the testis and vitellaria are specific to TCBZ.SO and do not simply represent a general response to any drug. It is known that disruption of the vitelline cells and spermatozoa following TCBZ treatment in vivo leads to abnormalities in egg formation, culminating in the complete cessation of egg production (Hanna et al. Reference Hanna, Edgar, McConnell, Toner, McConville, Brennan, Devine, Flanagan, Halferty, Meaney, Shaw, Moffett, McCoy and Fairweather2010, Reference Hanna, Scarcella, Solana, McConnell and Fairweather2012a ; Toner et al. Reference Toner, Brennan, Hanna, Edgar and Fairweather2011a ). So, the changes seen in the two tissues following R(+)-VPL co-treatment with TCBZ.SO would have a serious impact on the reproductive capacity of TCBZ-R flukes. Knockdown of the SMDR2 and SmMRP1 transporter genes in Schistosoma mansoni has been shown to cause a reduction in egg production (Kasinathan et al. Reference Kasinathan, Morgan and Greenberg2011). In the same study, incubation of worms in vitro with R(+)-VPL alone led to a drop in egg production; this was attributed to a disruption of oocyte development in the ovary. Whilst the ovary was not examined in the present study on F. hepatica, it is interesting that R(+)-VPL alone had no effect on the testis or vitelline follicle. Egg hatch data have shown that eggs of TCBZ-R fluke isolates are more resistant to the action of TCBZ than those of TCBZ-S isolates (Fairweather et al. Reference Fairweather, McShane, Shaw, Ellison, O'Hagan, York, Trudgett and Brennan2012). Pgp has been localized in the multi-layered eggshell of nematode parasites and the level of staining is greater in anthelmintic-resistant than -susceptible isolates (Kerboeuf et al. Reference Kerboeuf, Chambrier, Le Vern and Aycardi1999, Reference Kerboeuf, Guénard and Le Vern2003b ; Riou et al. Reference Riou, Koch, Delaleu, Berthon and Kerboeuf2005). It was suggested that the role of Pgp in the eggshell is to protect the egg from the toxic effects of xenobiotics such as anthelmintics. The eggshell of F. hepatica is a less complex structure, formed by the coalescence of shell protein material contained in the shell globule clusters in the vitelline cells, and it is possible that Pgp is associated with these clusters.
The existence of large spaces between the cells within the vitelline follicle indicates that the nurse cell network has been disrupted. As well as providing a support(ing) framework for the cells, the nurse cells serve to transfer precursor molecules from the parenchyma to the vitelline cells, which is essential for their development (Irwin and Threadgold, Reference Irwin and Threadgold1970). The loss of contact between the cells and the nurse cell processes prevents that exchange and supply of nutrients to the cells from taking place. A similar network exists in the testis tubules, emanating from the sustentacular cells, and these cells have a similar function to the nurse cells (Hanna et al. Reference Hanna, Moffett, Brennan and Fairweather2012b ). The sustentacular cells are analogous to the Sertoli cells in the mammalian testis, which support, protect and nourish the germ cells (Griswold and McLean, Reference Griswold, McLean and Neill2006). The Sertoli cells express a diverse array of drug transporters, including Pgp, which serve to protect the germ cells from the harmful effects of xenobiotics by preventing their entry into the testis (Melaine et al. Reference Melaine, Liénard, Dorval, Le Goascogne, Lejeune and Jégou2002; Su et al. Reference Su, Mruk and Cheng2011; Robillard et al. Reference Robillard, Hoque and Bendayan2012). Disruption to the sustentacular tissue following TCBZ treatment in vivo has been described by Toner et al. (Reference Toner, Brennan, Hanna, Edgar and Fairweather2011b ). Pgp has been located in the cytoplasmic networks within the vitelline follicles and testis tubules of F. hepatica (Wells, Reference Wells2008). It is possible that Pgp-linked efflux pumps are involved in the exchange of materials between the parenchyma and the cells within these two organs. The level of Pgp activity in TCBZ-S flukes may not be sufficient to protect the vitelline and spermatogenic cells from the effects of TCBZ. In TCBZ-R flukes, however, a greater expression of Pgp would normally shield the cells, but inhibition by R(+)-VPL would allow TCBZ to gain access to the cells, leading to the disruption seen.
The changes to the vitelline and spermatogenic cells induced by the drug combination occurred very quickly in vitro (after only 9 h incubation). They are consistent with the changes to the tegument and gut described previously (Savage et al. Reference Savage, Meaney, Brennan, Hoey, Trudgett and Fairweather2013a , Reference Savage, Meaney, Brennan, Hoey, Trudgett and Fairweather b ). Translating the morphological disruption and inactivity data to the in vivo situation, they would undoubtedly hasten the ‘death’ and elimination of the fluke and lead to greater efficacy of TCBZ against TCBZ-R flukes. Enhanced efficacy of anthelmintic+VPL combinations against nematode parasites has been demonstrated by Molento and Prichard (Reference Molento and Prichard1999), Bartley et al. (Reference Bartley, McAllister, Bartley, Dupuy, Menez, Alvinerie, Jackson and Lespine2009, Reference Bartley, Morrison, Dupuy, Bartley, Sutra, Menez, Alvineire, Jackson, Devin and Lespine2012) and Lifschitz et al. (Reference Lifschitz, Entrocasso, Alvarez, Lloberas, Ballent, Manazza, Virkel, Borda and Lanusse2010a , Reference Lifschitz, Suarez, Sallovitz, Cristel, Imperiale, Ahoussou, Schiavi and Lanusse b ). Based on this evidence and data from other resistance reversal experiments carried out in vitro, the use of anthelmintics plus Pgp inhibitors has been proposed as a strategy to deal with drug resistance (e.g. Lespine et al. Reference Lespine, Alvinerie, Vercruysse, Prichard and Geldhof2008, Reference Lespine, Ménez, Bourguinat and Prichard2012; Kasinathan et al. Reference Kasinathan, Goronga, Messerli, Webb and Greenberg2010a , Reference Kasinathan, Morgan and Greenberg2011; Lifschitz et al. Reference Lifschitz, Entrocasso, Alvarez, Lloberas, Ballent, Manazza, Virkel, Borda and Lanusse2010a , Reference Lifschitz, Suarez, Sallovitz, Cristel, Imperiale, Ahoussou, Schiavi and Lanusse b ; Kerboeuf and Riou, Reference Kerboeuf and Riou2011; Kasinathan and Greenberg, Reference Kasinathan and Greenberg2012).
In conclusion, inhibition of Pgp activity in a TCBZ-R isolate of F. hepatica has altered (increased) the response of the testis and vitellaria to treatment with TCBZ.SO. That is, it has reversed the TCBZ-resistant phenotype of the fluke. The changes are representative of those normally seen in TCBZ-S flukes following exposure to TCBZ and are not simply non-specific drug-induced changes. Such potentiation of TCBZ activity may be due to the increased expression of Pgp-linked drug efflux pumps in TCBZ-R flukes, as previously suggested (Alvarez et al. Reference Alvarez, Solana, Mottier, Virkel, Fairweather and Lanusse2005; Mottier et al. Reference Mottier, Alvarez, Fairweather and Lanusse2006), although this may not be the case (Fuchs et al. Reference Fuchs, Ryan, Brennan, Trudgett, Fairweather and Hoey2008). Alternatively, blocking of Pgp may simply increase the effective concentration of TCBZ/TCBZ.SO within the fluke, thereby overcoming a separate mechanism of resistance (see below). It is known that TCBZ-R flukes possess a variant allele for Pgp (Wilkinson et al. Reference Wilkinson, Law, Hoey, Fairweather, Brennan and Trudgett2012); this may be more efficient at pumping out TCBZ metabolites. The results support other morphological, pharmacological and molecular data for the role of Pgp in resistance to TCBZ, as referred to in the Introduction. The same mechanism appears to be displayed by TCBZ-R fluke isolates from three different countries (Australia, Ireland and the Netherlands), suggesting that it has arisen independently in each case. It may not be the only resistance mechanism in operation, because greater drug metabolizing activity has been demonstrated in both the Sligo isolate (Robinson et al. Reference Robinson, Lawson, Trudgett, Hoey and Fairweather2004; Alvarez et al. Reference Alvarez, Solana, Mottier, Virkel, Fairweather and Lanusse2005) and the Oberon isolate. In the case of the latter, drug sensitivity can be reversed by the use of inhibitors of detoxifying enzyme pathways (e.g. Devine et al. Reference Devine, Brennan, Lanusse, Alvarez, Trudgett, Hoey and Fairweather2011, Reference Devine, Brennan, Lanusse, Alvarez, Trudgett, Hoey and Fairweather2012). These two non-specific mechanisms seem to be more important than any specific changes in the presumed target molecule for TCBZ, namely, β-tubulin (Ryan et al. Reference Ryan, Hoey, Trudgett, Fairweather, Fuchs, Robinson, Chambers, Timson, Ryan, Feltwell, Ivens, Bentley and Johnston2008). Their combined effect would serve to block TCBZ and its metabolites from reaching their target. While the present results point towards a role for drug transporters in resistance to TCBZ, more needs to learnt about their identity and localization in F. hepatica, their expression in TCBZ-S and -R isolates and the interaction between the transporters and Pgp inhibitors. Future work could also involve the use of other Pgp inhibitors, to confirm the phenomenon, and application of some form of statistical analysis to the morphological data. As far as the authors are aware, the latter has not been attempted in other drug studies on F. hepatica (or other helminth parasites). Semi-quantitative analyses have been carried out (e.g. Hanna et al. Reference Hanna, Edgar, McConnell, Toner, McConville, Brennan, Devine, Flanagan, Halferty, Meaney, Shaw, Moffett, McCoy and Fairweather2010, Reference Hanna, Forster, Brennan and Fairweather2013; Devine et al. Reference Devine, Brennan, Lanusse, Alvarez, Trudgett, Hoey and Fairweather2011, Reference Devine, Brennan, Lanusse, Alvarez, Trudgett, Hoey and Fairweather2012; Meaney et al. Reference Meaney, Savage, Brennan, Hoey, Trudgett and Fairweather2013; Savage et al. Reference Savage, Meaney, Brennan, Hoey, Trudgett and Fairweather2013a ); they might have been useful in underpinning the results, although in this case the differences in responses between drug treatments were clear-cut.
ACKNOWLEDGEMENTS
This work was supported by a DARDNI Postgraduate Studentship to Joanne Savage. It was also partially supported by a grant from the European Union (DELIVER grant, no. FOOD-CT-200x-023025) and by a BBSRC/Defra grant (C00082X/1).