Introduction
Fibronectin type III domain-containing protein 5 (FNDC5) was originally identified and characterised by two independent research teams in 2002, which is mainly expressed in the skeletal muscle along with the heart, adipose tissue, brain and liver but with relatively small amount (Ref. Reference Aydin1). The synthesis and secretion of FNDC5 is stimulated by peroxisome proliferator-activated receptor-γ co-activator 1α (PGC1α), which serves as a transcription cofactor in response to endurance exercise in muscle tissue (Ref. Reference Bostrom2). As a glycosylated type I transmembrane protein, FNDC5 is proteolytically cleaved and released into the circulation constituting a soluble polypeptide, namely irisin of 112 amino acids (aa). It is widely evidenced that FNDC5/irisin can potentiate energy expenditure by browning white adipose tissue and be responsible for glucose homeostasis by ameliorating insulin resistance, yet controversies also exist (Ref. Reference Perakakis3). In addition to its myokine activity, FNDC5/irisin can also serve as a type of adipokine. Reports have indicated that irisin suppresses lipids accumulation via modifying adipose triglyceride lipase and fatty acid synthase (Refs Reference Huh4, Reference Ma5). Furthermore, overexpressed FNDC5/irisin in obese mice led to reduced adipocyte size in subcutaneous adipose tissue and induced lipolysis (Ref. Reference Xiong6). All these results revealed that the main facet of FNDC5/irisin is governing fat size of the body and in consequence the metabolic rate of fat tissue. Taking into account the exercise-induced property of irisin (myokine) in the physiological context, it has extensively raised concerns of the scientific community to investigate its pathobiological role, clinical relevance and therapeutic potential in a wide spectrum of diseases. Since the inspiring discovery of irisin in 2012, approximately 1400 researches have been published with stable annual increment.
Liver disease accounts for a heavy burden on global healthcare and the economic system in line with increased prevalence and related morbidity/mortality (Ref. Reference Cui7). Accordingly, around 2 million yearly deaths have been attributed to predominant liver diseases including but not limited to viral hepatitis infection, liver cirrhosis and various hepatic malignancies (Ref. Reference Byass8). The development and widespread application of vaccines and antiviral drugs have remarkably reduced the incidence of hepatitis B virus (HBV) infection, but the resultant number of chronic HBV patients and carriers remains enormous (Ref. Reference Trepo, Chan and Lok9). Gloomily, 2.4 billion people are consuming alcohol with 950 million designated as highly addictive, giving rise to a lifetime prevalence of alcohol use disorder as being 5.1–8.6% (Ref. Reference Asrani10). On the other hand, non-alcoholic fatty liver disease (NAFLD) has been reported to be epidemic in approximately 25% of the adult population but geographic variability (Ref. Reference Younossi11). Of note, the burden of NAFLD may become the leading cause of hepatic disorders during the coming decades in some countries/regions currently undergoing rapid urbanisation (e.g. Asia) (Ref. Reference Kabarra, Golabi and Younossi12). The increasing and high rate of NAFLD is proportionate with the occurrence of obesity, diabetes mellitus (DM) and metabolic diseases. As a matter of fact, NAFLD refers to a pathological defect belonging to metabolic syndrome manifested in the liver, inspiring scientific endeavour to explore whether exercise-derived irisin may act as a possible therapeutic target as far back as 2014. Although lots of attempts have been made to characterise the clinical implication of irisin in the context of liver diseases, literature data appear to be irreproducible and enigmatic probably due to flawed testing assays by using dramatically discrepant reference range and methodology (Ref. Reference Maak13). However, it has been well documented with regard to the anti-inflammatory, antioxidative and antiapoptotic activities by administering recombinant irisin or employing genetic depletion mice within different pathological conditions (Ref. Reference Askari14).
In this article, we comprehensively mine and review the literature data with regard to the purported myokine irisin and its precursor FNDC5 in the pathogenesis of several hepatic pathologies, if available, as the therapeutic scale. The collected information and retrieved findings in relation to basic research and clinical studies of FNDC5/irisin are depicted in the separated section for more explicit statement and better understanding for our authors. Some basic knowledge and future research perspectives are also discussed in this article.
Structure–function basis of FNDC5/irisin
In rodents FNDC5 comprising six exons encodes a protein of 209 amino acids. FNDC5 protein consists of a 39-aa cytoplasmic tail (171–209), a 21-aa transmembrane domain (150–170), a 92-aa FNIII domain (33–124) and a 28-aa N-terminal signal peptide (1–28) (Ref. Reference Young, Valaris and Wrann15). The N-terminal sequence, responsible for transporting the protein across the membrane, is then cleaved (Ref. Reference Schumacher16). Irisin is proposed to result from proteolytically cleaved transmembrane protein FNIII plus a 19-aa linker peptide (29–140). Overall, FNDC5 appears to be a highly conserved gene except for a mutant start codon referring to canonical ATG to ATA (Ref. Reference Raschke17). The crystal structure of irisin has been deciphered with a continuous inter-subunit β-sheet dimer at the FNIII domain. The sequence of irisin exhibits 100% conserved between rodents and humans. The 112-aa irisin polypeptide possesses a theoretical molecular weight of 12 600 in response to deglycosylation. Accordingly, the glycosylated irisin at two N-linked sites is supposed to increase its expected molecular weight to a total of 28 000. Plenty of analytical metrics have been instigated to detect and quantify FNDC5/irisin, including antibody-based as well as label-free methods (e.g. western blot, enzyme-linked immunosorbent assay [ELISA], protein-lipid chip assay and mass spectrometry [MS]). However, we are far away from getting valid and reproducible measurements due to drastically methodological variability and heterogeneity. Furthermore, the reported contents/levels in the existing literature of irisin in the circulation represented a wide range from pg/ml to μg/ml, leading to more discordance and ambiguities.
Signalling pathways pertaining to cellular activities of FNDC5/irisin
A multitude of signalling pathways have been addressed to regulate or be activated by FNDC5/irisin with the purpose of delineating its pathobiological roles. More recently, Rabiee et al. have summarised and provided an outstanding article with respect to potentially intricate pathways facilitating intracellular functions of irisin circumstantially (Ref. Reference Rabiee18). In that paper, the authors denoted that FNDC5/irisin exerts multifaceted roles including anti-inflammation, anti-tumour, anti-depression, maintenance of metabolic homeostasis and bridging crosslink between the muscle and other organs/tissues. Notably, we intended to provide insight into signalling pathways specific to diverse liver diseases contextually in the following sections. Herein we briefly discussed the assumed receptor of irisin, since this ligand–receptor interaction takes responsibility for activating downstream pathways in charge of cellular processes. Although the receptor specific to irisin is yet to be identified until now, Kim et al. showed irisin could bind to integrins, a family of transmembrane cell adhesion molecules mediating cell-matrix and intercellular interactions (Ref. Reference Kim19). Using crosslinking and MS on the cultured cells, their observations implicated that αVβ5, known as the favoured receptor, represents the most strong response among screening determination, which is further verified by following research (Refs Reference Estell20–Reference Bi22). Further experiment indicated that CD81 serves as a partner in the integrins signalling by sensitizing the irisin–receptor interaction in HEK293T cells (Ref. Reference Oguri23). Intriguingly, accumulating evidence has proved αV integrins also play pivotal roles in the fibrogenic development, metastatic activity and anti-inflammatory response in a variety of liver diseases (Refs Reference Zhang24, Reference Sun25).
Association between FNDC5/irisin and NAFLD (and relevant metabolic disorders)
NAFLD covers a broad spectrum of hepatic pathology entities ranging from simple fatty infiltration in hepatic parenchyma to the potentially progressive type designated as non-alcoholic steatohepatitis (NASH). In alignment with global obesity epidemic, NAFLD and NASH have approached alarming levels worldwide. In T2DM subjects, 55% of the population is suggested to be accompanied with NAFLD closely relevant to visceral obesity, hyperlipidaemia and hypertension (Ref. Reference Kabarra, Golabi and Younossi12). Notably, NASH is histologically evidenced by diffused steatosis, multi-lobular inflammation, hepatocellular ballooning, appearance of Mallory–Denk bodies along with fibrosis pericellularly. About 15–20% patients with NASH may proceed to cirrhosis when compared with 5–10% advancing to other end-stage liver diseases like hepatocellular carcinoma (HCC) requiring transplantation (Ref. Reference Younossi26). The incidence of HCC among NASH subjects with cirrhosis is estimated to be 0.5–2.6% (Ref. Reference Huang, El-Serag and Loomba27). Nonetheless, HCC has occasionally been diagnosed among patients with NAFLD and metabolic syndrome rather than those with obvious fibrosis or cirrhosis, giving rise to a challenge regarding a lack of regular and well-scheduled surveillance. Actually, this subset is prone to more advanced malignancies with dismal outcome compared with HCC on account of chronic viral hepatitis load (Ref. Reference Hester28). Until now, there are no specific and available pharmacological regimens to cure NAFLD/NASH approved by the FDA, although some natural components with anti-inflammation and antioxidant capabilities might be promising candidates (Refs Reference Soleimani29, Reference Soleimani30). The gold standard therapy recommended by the major scientific associations is to lose weight through calorie restriction in addition to physical exercise (Ref. Reference Chalasani31). Keeping in view the property of FNDC5/irisin in response to exercise, it has greatly inspired scholars' interest to deepen the potentials by targeting this novel and pleiotropic myokine as a biomarker or treatment of choice.
Basic researches
Soon after the discovery of irisin, mounting attempts have been made to reveal its pathogenic facet and therapeutic application in the context of NAFLD and relevant metabolic disorders (Table 1). Park et al. incubated mouse AML12 hepatic cells and primary hepatocytes with palmitic acid (PA) to mimic insulin resistance and steatosis, and found recombinant irisin markedly suppresses PA-induced lipogenesis and hepatocellular lipid accumulation (Ref. Reference Park32). Moreover, the nuclear localisation, lipogenic regulator expression/transcriptional activities, oxidative stress and inflammatory biomarkers were all markedly alleviated upon irisin treatment. These protective effects of irisin against hepatic steatosis may be accomplished by suppressing protein arginine methyltransferase-3, nuclear factor κB (NF-κB) or p38 mitogen-activated protein kinase signalling pathways. Liu et al. showed FNDC5 deficiency aggravates hyperlipidaemia, hepatic fatty acid oxidation/lipogenesis, lipid accumulation and autophagy flux blockage, which can be effectively reversed by exogenous prescription or FNDC5 overexpression through modulating adenosine monophosphate-activated protein kinase (AMPK)/mammalian target of rapamycin complex 1/peroxisome proliferator-activated receptor α (PPARα) axis (Ref. Reference Liu33). Similarly, liver-derived irisin functioned in both autocrine and paracrine modalities to surpass lipogenesis and reverse steatosis, responding to constitutive androstane receptor by activating the AMPK pathway with dose response in primary human hepatocytes (Ref. Reference Mo34). Relief of hepatic steatosis was also observed in irisin transgenic mice in correspondence with reduced lipogenic gene expression including sterol regulatory element binding protein-1c, fatty-acid synthase and stearoyl-CoA desaturase-1 (SCD-1). The connection between AMPK activation and administered irisin has been well documented in relation to lesser hepatic lipid accumulation and glucose output, increased fatty acid oxidation/glycolysis in addition to reduced lipogenesis/gluconeogenesis within different experimental models (Refs Reference So and Leung35, Reference Tang36). By conducting in vitro study, it has been confirmed that irisin reduces hepatic triglyceride/glucose content, but increases glycogen content and promotes HepG2 cells survival (Ref. Reference So and Leung35). In addition, subcutaneous infusion of irisin significantly reduced plasma and hepatic cholesterol in vivo, which proposes a promising therapeutic target of irisin for hypercholesterolaemia (Ref. Reference Tang36). In mice subjected to a fructose-rich diet, Motta et al. found liver steatosis develops with adipocyte hypertrophy, and these detrimental events were relevant to altered gene expressions including FNDC5, PGC1α and PGC1β, all of which were efficiently prevented upon high-intensity interval training (HIIT) (Ref. Reference Motta37). The authors concluded that the beneficial influences of HIIT, in parallel to the medicinal properties of metformin, may partially alleviate the liver injury and prevent progressive NAFLD from more advanced pathological stages. In contrast, the addition of recombinant irisin had no effect on lipid contents in HepG2 cells exposed to oleic acid and PA in line with unchanged mRNA expression of PPARα and SCD, while no significant hepatic expression of irisin was observed in high-fat diet (HFD) inducing NAFLD mice model (Ref. Reference Petta38). In two NAFLD models challenged by HFD or a methionine- and choline-deficient (MCD) diet, Canivet et al. implied hepatic FNDC5 expression increases with steatotic changes and liver damages, whereas circulating irisin did not proportionate to consequential complications in the liver (Ref. Reference Canivet39). Both hepatocytes and non-parenchymal cells incorporating innate immune cells and activated HSC dominated the production of FNDC5 in fatty liver, wherein hepatocellular source was stimulated by genotoxic stress and p53-PGC1α signalling pathway. Knockdown of FNDC5 in HepG2 cells and in mouse primary hepatocytes resulted in increased expression of glucogenesis gene (phosphoenolpyruvate carboxykinase) and decreased expression of very-low-density lipoprotein synthesis genes (such as apolipoprotein B). Using in vitro modalities, it has also been demonstrated that FNDC5 can suppress hepatocellular fat accumulation by enhancing the export of lipids, facilitating autophagic flux in addition to decreasing the cytokine-mediated apoptosis. Another research intended to delineate the therapeutic potential of nicotinamide riboside (NR) against NAFLD in similarly built models (i.e. mice fed an HFD or MCD) between wild-type and FNDC5−/− mice (Ref. Reference Li40). Their findings implicated the FNDC5 gene expression is dramatically up-regulated in the skeletal muscle, adipose and liver tissue along with plasma irisin values upon NR treatment. The medicinal properties were evidenced by alterations with respect to hepatic steatosis, steatohepatitis, insulin sensitivity, mitochondrial dysfunction and apoptotic cell death, which were all compromised in FNDC5 knockout mice. Mechanistically, NR treatment displayed its hepatoprotective action by regulating sirtuin 2-dependent FNDC5 deacetylation and deubiquitination to foster protein stability as well as contents expressed. Moreover, the exerkine nature of irisin has been explored and corroborated in another HFD-induced NAFLD (Ref. Reference Zhu41). Accordingly, exercise training markedly ameliorated hepatic steatosis and fibrosis with concurrent enhancement in circulating irisin levels and muscular irisin expression. By using PA-incubated AML12 cells in vitro, it has been suggested that irisin is capable of disturbing myeloid differentiation factor 2-Toll-like receptor 4 (TLR4) complex formation to exert anti-inflammatory activities dose-dependently. Moreover, irisin could improve lipid metabolism and fibrosis by attenuating expression of fatty acid-binding protein 4, collagen type 1 and transforming growth factor-β (TGF-β). As a matter of fact, there are concerns about the synchronizing impacts and reciprocal reactions between NAFLD and other entities. For instance, Medhat et al. determined the influence of irisin in an experimental model suffering from NAFLD due to metabolic syndrome induced by a high-fat high-carbohydrate diet (Ref. Reference Medhat42). Their findings unveiled that administered irisin can attenuate dysregulated hepatic architecture characterised by diminished foam cells, foster energy expenditure via PGC1α and repress hepatic cholesterol synthesis relying on AMPK-sterol regulatory element-binding transcription factor 2 axis. In a study exploring the pathogenic link between NAFLD and inflammatory bowel disease, Kwon et al. showed dextran sodium sulphate-induced colitis leads to a significant down-regulation of the skeletal muscle-derived irisin accompanied by perturbing impacts on overall metabolic processes, ultimately presented as hepatic steatosis- and dyslipidaemia-like phenotypes (Ref. Reference Kwon43). MicroRNAs (miRNAs) belong to a setting of single-stranded, small-sized non-coding RNAs negatively modifying gene expression at the post-transcriptional level bound to the 3ʹ-untranslated region (UTR) of target miRNAs (Ref. Reference Alamdari44). Diversely dysregulated miRNAs expressions have been linked to the pathological advancement in patients with NAFLD (Ref. Reference Gjorgjieva45). Most recently, Yu et al. unveiled miR-665-3p binds directly to the 3ʹ-UTR of FNDC5, in consequence down-regulates its expression, inactivates the downstream AMPKα signalling pathway, and thereafter mediates oxidative stress, inflammatory reaction and NAFLD progression (Ref. Reference Yu46). Correspondingly, increased hepatic irisin levels were found by antagonizing miR-665-3p. Knockdown of endogenous FNDC5 expression in the liver abrogated the miR-665-3p antagomir-mediated protection, which was evidenced by increased serum transaminase levels and hepatic hydroxyproline content. A schematic diagram was depicted in Figure 1.
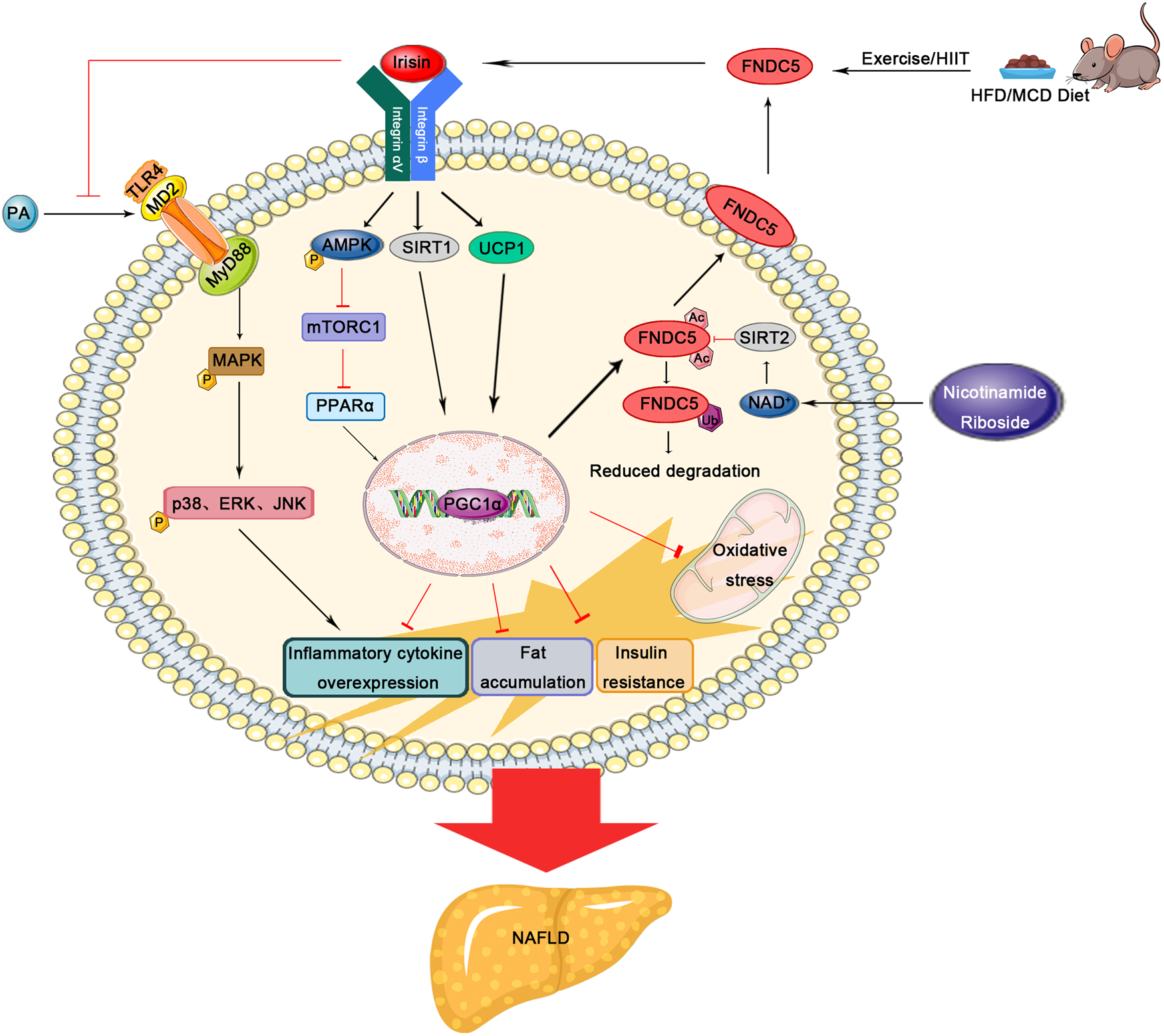
Fig. 1. Schematic diagram depicting the pathogenic role and therapeutic potential of FNDC5/irisin in experimental NAFLD models. FNDC5, fibronectin type III domain-containing protein 5; HFD, high-fat diet; MCD, methionine- and choline-deficient; SIRT, sirtuin; UCP1, uncoupling protein 1; PA, palmitic acid; AMPK, adenosine monophosphate-activated protein kinase; mTORC1, mammalian target of rapamycin complex 1; PPARα, peroxisome proliferator-activated receptor α; TLR4, Toll-like receptor 4; MD2, myeloid differentiation factor 2; PGC1α, proliferator-activated receptor-γ co-activator 1α; NAD+, nicotinamide adenine dinucleotide+; MyD88, myeloid differentiation factor 88; MAPK, mitogen-activated protein kinase.
Table 1. The pathogenic role and therapeutic potential of FNDC5/irisin in experimental NAFLD models

FNDC5, fibronectin type III domain-containing protein 5; HFD, high-fat diet; N.A., not applicable; T2DM, type 2 diabetes mellitus; PI3K, phosphoinositide 3-kinase; FOXO-1; forkhead box transcription factor O1; GSK3, glycogen synthase kinase-3; PEPCK, phosphoenolpyruvate carboxykinase; G6Pase, glucose-6-phosphatase; GS, glycogen synthase; PRMT3, protein arginine methyltransferase-3; AMPK, adenosine monophosphate-activated protein kinase; mTORC1, mammalian target of rapamycin complex 1; PPARα, peroxisome proliferator-activated receptor α; HSCs, hepatic stellated cells; MCDD, methionine- and choline-deficient diet; SIRT, sirtuin; NAD+, nicotinamide adenine dinucleotide+; MAPK, mitogen-activated protein kinase; NF-κB, nuclear factor-κB; IBD, inflammatory bowel disease; DSS, dextran sodium sulphate; miR, microRNA; UTR, untranslated region.
DM is a major metabolic disorder influencing multiple organs/tissues, and the generally acting mechanisms designated as insulin resistance and in consequence hyperglycaemia may give rise to NAFLD or NASH by interrupting carbohydrate, lipid and protein metabolisms and HCC in circumstances (Ref. Reference Chen47). In obese rats challenged by HFD, exercise and exogenous supplementation with potent antioxidant aged garlic extract exerted no influence on liver/gastrocnemius muscle masses in line with unchanged plasma irisin or skeletal muscle FNDC5 contents, counteracting its impact on homeostatic metabolism (Ref. Reference Seo48). In vitro study showed that irisin significantly dampens gluconeogenesis and enhances glycogenesis in HepG2 cells and mouse primary hepatocytes undergoing insulin resistance induced by glucosamine or palmitate via activation of the phosphoinositide 3-kinase (PI3K)/Akt pathway, which is mechanistically shared with insulin (Ref. Reference Liu49). Niranjan et al. also treated HFD-triggered obesity mice with recombinant irisin presented with significant up-regulation of uncoupling protein 1 (UCP1) in a dose-respondent manner (Ref. Reference Niranjan50). The pivotal role of UCP1 in dissipating energy in the form of heat rather than stored fat depots may link the pathophysiological facet regarding irisin to mitigate hepatic steatosis. Another metabolic and inflammatory regulation mechanism of irisin relied on its effect on brain-derived neurotrophic factor, fatty acid binding protein 4 and PPARγ in spleen. During the last 10 years, there has been a surge delineating the involvement of gut microbiota to the pathogenesis of obesity and its complications including DM, obesity, hyperlipidaemia and NAFLD (Refs Reference Cui7, Reference Canfora51). In this regard, Kwon et al. stated the Lactobacillus plantarum strain exerts favourable metabolic regulations including improvement in hepatic steatosis in HFD-induced obese mice along with up-regulated irisin mRNA expression in the skeletal muscle and subcutaneous fat tissue (Ref. Reference Kwon52). The protective effect of L. plantarum to improve lipid oxidation and alleviate adipose accumulation was implemented via SIRT1/PGC1α signalling pathway in addition to fighting against metabolic dysregulation and favouring hepatic cholesterol clearance (Ref. Reference Chang and Guarente53).
Two research teams used substances from natural products of choice, namely silymarin (belongs to flavonoid) and genistein (belongs to isoflavone) for treating metabolic disorders-related deleterious liver damages. Their findings demonstrated that these medicinal agents can significantly enhance serum irisin concentration and hepatic FNDC5 expression responsible for unobvious histopathological changes in the parenchyma and amended metabolism homeostasis (Refs Reference Kheiripour54, Reference Shen55). A plethora of hepatoprotective machinery is pointed out including antioxidant effects, anti-endoplasmic reticulum (ER) stress as well as anti-inflammatory activities. In this regard, garlic oil, a natural constituent, mitigated the diabetic liver injury by repressing oxidative stress-related culprits and modulating serum irisin values along with PGC1α, FNDC5, p38 in liver tissue to maintain insulin sensitivity and balance carbohydrate metabolism (Ref. Reference Eser56).
Clinical studies
As far back as 2013, Zhang et al. showed for the first time serum irisin levels are inversely associated with intrahepatic triglyceride contents and significantly decreased among NAFLD in 296 obese Chinese adults (Ref. Reference Zhang57). Furthermore, inverse correlation between circulating irisin levels and liver enzymes (i.e. ALT and AST) reflected a protective role of irisin against hepatic steatosis. In the same year, Polyzos et al. reported higher systemic irisin contents in lean controls when compared with obese subjects, biopsy-proven patients with simple steatosis and NASH participants (Ref. Reference Polyzos58). However, no difference was observed in patients with simple steatosis relative to NASH, despite higher irisin levels appeared to be independently associated with obvious portal inflammation (36.7 versus 30.8 ng/ml). Similarly, in a setting of patients with NAFLD, T2DM or their concurrence, the plasma irisin levels exhibited gradual reduction in that order (2.18 versus 1.96 versus 1.45 μg/ml) with highest values in healthy controls (Ref. Reference Shanaki59). It is suggested that irisin can predict liver stiffness independently, and elevated circulating irisin levels may reduce the risk of these metabolic disorders in addition to moderately diagnostic utility for NAFLD. Waluga and colleagues consistently addressed lower plasma irisin concentrations in the NAFLD group in comparison with healthy control and an inverse correlation with grading inflammation in NAFLD, which was contrary to the results by Polyzos (Ref. Reference Waluga60). The authors speculated the severity of liver histopathological lesion dominates these relationships.
Oppositely, another study indicated the serum irisin levels in NAFLD group are higher than those of control group, suggesting increased irisin as a defensive partner during the early stage of NAFLD. This compensatory action would be exhausted; simultaneously, the steatotic advancement is accompanied by a decrease in irisin contents (Ref. Reference Choi61). These observations were verified in patients with metabolic syndrome and concurrent fatty liver, whose systemic irisin concentrations were drastically up-regulated and represented a positive correlation with liver enzymes, homeostatic model assessment of insulin resistance index (HOMA-IR), anthropometry data as well as serum triglycerides (Ref. Reference Rizk, Elshweikh and Abd El-Naby62). Likewise, male HIVs without diabetes had higher irisin concentrations correlated to HOMA-IR and NAFLD identified by higher hepatic triglyceride contents via 1-H magnetic resonance spectroscopy (Ref. Reference Moreno-Perez63). Another study reported morbidly obese patients with hepatic steatosis and NASH have up-regulated FNDC5 mRNA expression in liver, closely relevant to the grade of steatosis, NAFLD activity score and ALT levels, rather than impact on the systemic levels of irisin (Ref. Reference Canivet39). Taken together, unlike the local involvement of FNDC5 to the onset and progression of NAFLD, increased and decreased systemic irisin have both been addressed in the existing literature. One justified interpretation refers to muscle-derived irisin constituting approximately 70% of the total circulating protein levels, while sarcopaenia (muscle wasting) has a reciprocal relationship with NAFLD as being a risk factor or a complication (Refs Reference Perakakis3, Reference Bhanji64). More recently, Armandi et al. denoted circulating irisin levels are increased (in the absence of sarcopaenia) in individuals with marked fibrosis and correlated with liver fibrogenesis, potentially identifying a more aggressive phenotype of liver disease with severe damage (Ref. Reference Armandi65). The authors speculated that a harmonic relationship between irisin and liver fibrogenesis, indicative of hepatic response to the inflammatory injury, may be attributed to the selection of a well-characterised cohort of biopsy-proven NAFLD for the absence of the major metabolic confounders. It is suggested that metabolic burdens such as DM and obesity could have affected the circulating irisin pool. Therefore, we believe future larger studies are merited to further uncover the association between circulating irisin levels and liver damages in patients with NAFLD.
Emerging evidence suggests a variety of inherited factors, especially single-nucleotide polymorphisms (SNPs) may potentiate the susceptibility of individual regarding the development and progression as well as therapeutic response in the context of NAFLD (Ref. Reference Martinez-Montoro66). However, no association was observed between FNDC5 rs3480 and anthropometric, metabolic and biochemical indices on the basis of 593 liver biopsy-confirmed NAFLD participants compared to healthy controls (Ref. Reference Petta38). In contrast, according to another investigation enrolling 987 Caucasian NAFLD patients, Metwally et al. reported FNDC5 rs3480 minor (G) allele associates with advanced steatotic changes, an independent variant but additive to patatin-like phospholipase domain-containing 3 and the transmembrane 6 superfamily member 2 responsible for hepatic fat accumulation (Ref. Reference Metwally67). Alteration of the above-described three variants concomitantly with ≥1 copy at respective region increased hepatic steatosis risk by 1.4- to 6.8-fold in comparison with subjects without any risk allele. The underpinning mechanism unravels marked FNDC5 mRNA degradation embracing G allele (versus A allele), which was bound with miR-135a-5P at its SNP in the 3’UTR resulting in impaired FNDC5 expression. Accordingly, NAFLD patients represented higher hepatic contents of miR-135a-5P and lower FNDC5, but increased serum irisin levels closely relevant to improved steatosis and metabolic profiles in the circulation. Taken together, abovementioned discordance among studies with respect to inherited determinants facilitating NAFLD probably results from divergent recruited population, different disease stage/severity and discordant detecting approaches, which warrant further validation with solid evidence.
From therapeutic perspectives, a clinical trial indicated green cardamom supplementation exerts medicinal influences on fatty liver grading, serum glucose parameters and lipid components within obese NAFLD patients, which can be mediated by increased SIRT1 and irisin levels (Ref. Reference Daneshi-Maskooni68). However, another study conducted on NAFLD patients showed α-lipoic acid, a cofactor for mitochondrial enzymes with multifaceted properties, administered for 12 weeks leads to improved insulin resistance and serum adipokines without affecting anthropometry data, liver steatosis intensity and irisin levels (Ref. Reference Rahmanabadi69).
Fibrosis/cirrhosis
Hepatic fibrosis stands for a common pathology subsequent to a majority of chronic liver diseases, ultimately giving rise to cirrhosis, liver failure and in more aggressive type like HCC (Ref. Reference Trautwein70). The hallmark and cornerstone pertaining to the pathogenesis of hepatic fibrosis is the activation of hepatic stellate cell (HSC) and in consequence diffused deposition of extracellular matrix (ECM) proteins. Emerging evidence implicates the fibrotic ECM can undergo remodelling and convert into near-normal hepatic architecture by injury cessation (Ref. Reference Ellis and Mann71). Thus it is practical to terminate the fibrogenic process aimed at slowing progressive fibrosis and even cirrhosis (Ref. Reference Elpek72).
Basic researches
Petta et al. showed irisin expression increases in line with HSC activation, evident by collagen α1 and α-smooth muscle actin (α-SMA) mRNA expression (Ref. Reference Petta38). Furthermore, exogenous irisin triggered profibrogenic genes monocyte chemoattractant protein-1 (MCP-1) and tissue inhibitors of metalloproteinases-1 (TIMP-1) in activated HSCs, and its expression was up-regulated in experimental hepatic fibrosis exposed to carbon tetrachloride (CCl4). In a study conducted by Zhou et al., FNDC5 deficiency exacerbated hepatic fibrosis induced by HFD in mice (Ref. Reference Zhou73). The underpinning molecular basis was built on AMPK phosphorylation-mediated inhibition of HSCs activation and up-regulation of connective tissue growth factor/TGF-β. Using oxidised low-density lipoprotein triggered primary mouse HSCs and human LX-2 cells in vitro, exogenous FNDC5 treatment also effectively mitigated HSC activation and ECM deposition indicative of gradually down-regulated α-SMA and collagen expression. Additionally, the medicinal potency of irisin for improving fibrogenic process was corroborated in immortalised LX-2 cells via modifying HSC activation, proliferation, migration, contractility and production of interleukin-(IL-)1β/IL-6, given the fundamental participation of inflammation in activating and trans-differentiating resting HSC to myofibroblasts (Ref. Reference Dong74). More recently, Liao et al. used recurrent CCl4 exposure to establish in vivo fibrosis model and clarified that co-treatment with irisin remarkably attenuates collagen deposition and the expression of fibrogenic biomarkers in mice (Ref. Reference Liao75). This therapeutic approach was in part attributed to inhibitory ER stress via regulating PKR-like ER kinase mediated heterogeneous nuclear ribonucleoprotein A1 destabilisation. A schematic diagram was depicted in Figure 2.
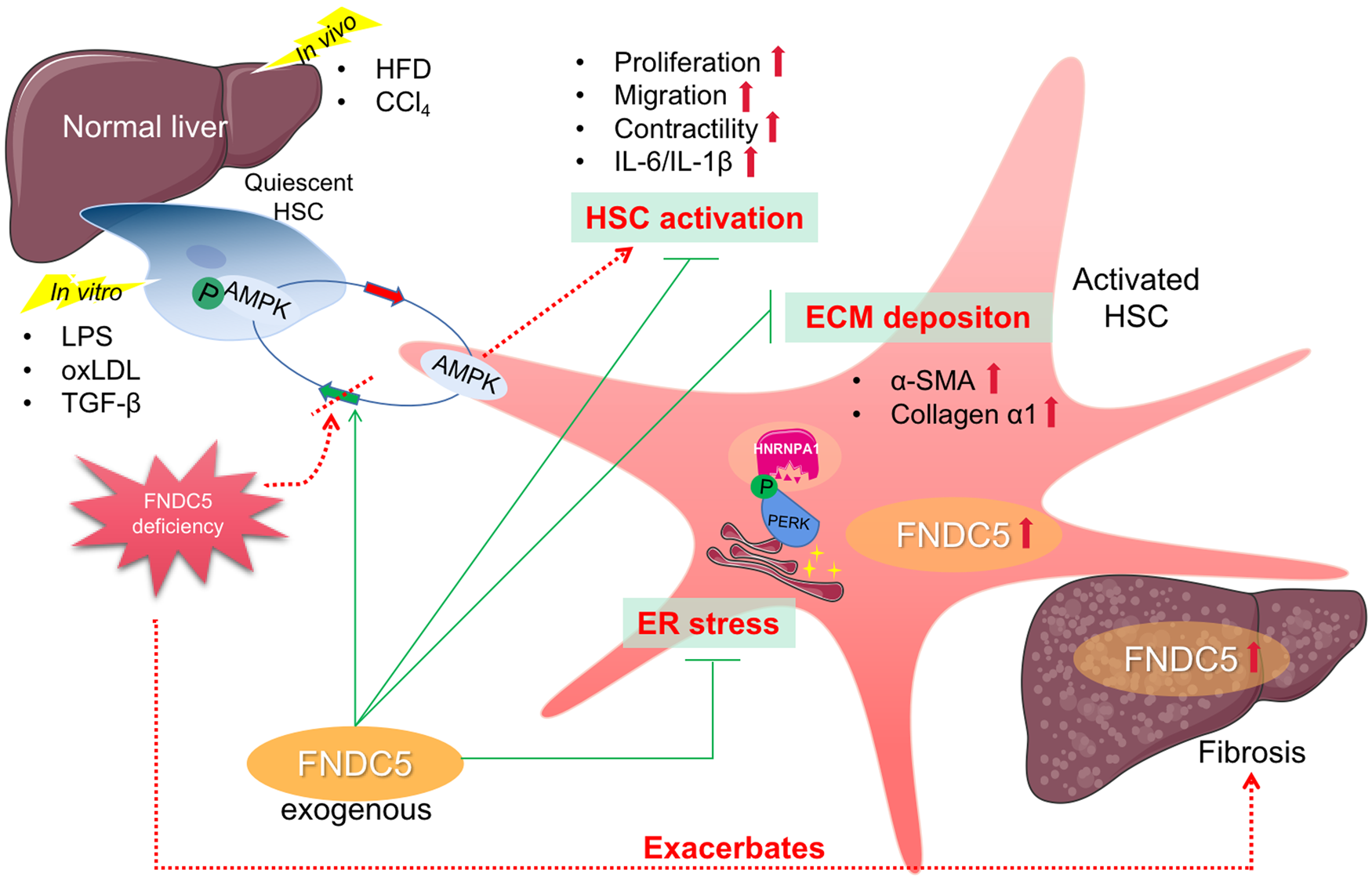
Fig. 2. Schematic diagram depicting the pathogenic role and therapeutic potential of FNDC5/irisin in experimental fibrosis models. FNDC5, fibronectin type III domain-containing protein 5; HSC, hepatic stellate cell; ECM, extracellular matrix; AMPK, adenosine monophosphate-activated protein kinase; α-SMA, α-smooth muscle actin; TGF-β, transforming growth factor-β; IL-, interleukin-; HFD, high-fat diet; CCl4, carbon tetrachloride; LPS, lipopolysaccharide; PERK, PKR-like ER kinase; HNRNPA1, heterogeneous nuclear ribonucleoprotein A1; oxLDL, oxidised low density lipoprotein.
Clinical studies
In a cohort of 593 patients prone to NASH, FNDC5 rs3480 A > G was in relation to protective impact against clinically significant fibrosis (Ref. Reference Petta38). Mechanistically, the authors proposed FNDC5 rs3480 may influence fibrosis by regulating irisin expression mainly derived from HSCs and adipocytes, in other words, irisin from distinct sites/origins may enter into plasma to elicit proinflammatory (e.g. MCP-1) and fibrogenic (e.g. TIMP-1) factors and consequently hepatic fibrogenesis.
Like elusive role of serum irisin in the context of NAFLD, the association between circulating irisin and liver cirrhosis remains unclear. A clinical study recruited 43 virus-related liver cirrhosis of whom 36 participants had HCC (Ref. Reference Pazgan-Simon76). The authors showed cirrhotics with concomitant HCC exhibit lower irisin concentrations than do healthy controls, although non-significantly lower irisin levels were observed in patients at more severe stage stratified by Child–Pugh classification. Kukla et al. intended to analyse the relationship between serum irisin levels and sarcopaenia in a total of 88 patients with decompensated cirrhosis with median model for end-stage liver disease (MELD) scores of 15 points (Ref. Reference Kukla77). Their findings suggested there is no marked difference regarding serum irisin levels among cirrhotic patients stratified by Child–Pugh classification and MELD score. Moreover, despite irisin primarily standing for a myokine, it did not correlate with sarcopaenia defined by low handgrip strength or central body muscle loss in foresaid work. On the other hand, existing insulin resistance may explain less relevance between cirrhosis and complication/comorbidity. On the contrary, Zhao et al. performed a cross-sectional study on 187 patients with cirrhosis, among whom a total of 39% presented sarcopaenia, and described plasma irisin as an independent biomarker relevant to sarcopaenia (Ref. Reference Zhao78). Other novel findings included positive correlation between skeletal muscle index at the third lumbar vertebra, namely an index of sarcopaenia, as well as correspondingly decreased irisin levels with worsening hepatic function (Ref. Reference Hou79). Collectively, it must be noted that all described studies were on the basis of relatively small sample size and their retrospective nature in design. The extremely wide reference values of retrieved irisin levels in serum (from pg/ml to μg/ml) and methodological heterogeneity may give rise to inconsistent results.
Various liver injury and relevant illness
Liver injury provides a general idea of hepatocytes damage resulting from a wealth of detrimental insults, including but not limited to toxicants, chemical agents, drugs, viral infection as well as binge. Additionally, it can be presented as accompanying symptom under deleterious attack to other organ/tissue. Within persistent and intensive pathobiological stress, hepatic damage proceeds to advanced or end-stage disease such as liver failure (Ref. Reference Wang80). Of note, acute liver failure brings high mortality rates due to insufficient transplant organ allocation and relatively limited treatment of choice.
Inadequate coronary blood supply against normal myocardial demand results in the decease of myocardial tissue designated as myocardial infarction (MI), which also leads to significant liver function abnormality (Refs Reference Barbagelata81, Reference Thirupurasundari, Jayalakshmi and Niranjali Devaraj82). Kuloglu et al. demonstrated that liver irisin levels, generated from both hepatocytes and sinusoidal cells, drastically decrease in the presence of MI induced by isoproterenol and considered decreased irisin as an uncoupling agent in the circulation to surpass ATP consumption and maintain cell viability by inhibiting necrotic process (Ref. Reference Kuloglu83). Intriguingly, a similar study conducted by Aydin et al. on myocardial ischaemic rats implied both iloprost and sildenafil can reverse down-regulated irisin immunoreactivity in the hepatocytes of liver tissue (Ref. Reference Aydin84). These tissue ischaemia-eliminating effects to some extent accelerated the blood supply through vasodilatation, cellular reoxygenation and enhanced irisin synthesis. The same research team clarified formaldehyde, a normal metabolite conditionally deleterious to liver tissue, increases free radical and poses oxidative stress on hepatocytes manifested by cell death in dose-dependent way (Ref. Reference Aydin85). The authors hypothesised the damaged cells may circumvent irisin synthesis aimed at preventing energy waste, evident from the reduced irisin in liver and the circulation. Furthermore, the histopathological and biochemical signs due to formaldehyde exposure were restored upon carnosine supplementation, a potent antioxidant capable of scavenging reactive oxygen species, which was usually found in the muscle and brain tissues. Accordingly, the antioxidant activity of irisin was validated. Further research intended to explore the relationship between irisin activity and methotrexate, known as a chemotherapeutic and immunosuppressive agent, triggered hepatotoxicity in rats (Ref. Reference Erdogan and Yalcin86). It was found that an increase in irisin activity is helpful for diminishing oxidative stress against hepatic toxicity and compensating for the increased energy demand, evident from reduced immunoreactivity of irisin in liver responding to benfotiamine (a thiamine pyrophosphate metabolite with antioxidant effect). CCl4 has been widely used to elicit hepatotoxicity experimentally and characterised by multiple biological facets including lipid peroxidation, oxidative stress, autophagy and ferroptotic cell death (Refs Reference Unsal, Cicek and Sabancilar87, Reference Zhao88). Rabey et al. reported CCl4 toxicity promotes inflammatory reaction at aggravated site of liver damage and potentiates oxidative stress (Ref. Reference Rabey89). However, green coffee methanolic extract exerted hepatoprotection by improving irisin and other antioxidants to reverse liver dysfunction, and converting inflammatory cytokines/oxidative stress indices to their normal values in the exposed rats. These medicinal effects are in alignment with other natural components such as propolis in the context of liver injury (Ref. Reference Pahlavani90).
Ischaemia-reperfusion (I/R) injury is subsequent to inflammatory processes in the case of target organ undergoing a cessation of blood flow or transient reduction, followed by perfusion re-establishment. Following liver surgery, haemorrhagic shock and severe sepsis, liver I/R injury results in organ dysfunction, liver failure and vice versa. Importantly, it represents a predisposing factor to the onset of graft rejection during liver transplantation (Ref. Reference Lu91). There is currently no clinically applicable therapeutics specific to I/R injury with the exception of supportive care medicine. Bi et al. demonstrated exogenous irisin markedly improves hepatic function, inhibits cell death and relieves inflammatory response in the setting of liver I/R (Ref. Reference Bi92). It is suggested that mitochondria constantly undergoes fission and fusion, which is biologically essential to maintain internal homeostasis (Ref. Reference Youle and van der Bliek93), and irisin significantly restrains mitochondrial fission-related protein, dynamin-related protein 1 and fission 1 for normally functioning. On the contrary, the mitochondrial content/biogenesis was fostered along with quenched oxidative stress by regulating PGC1α and uncoupling proteins 2 expression. In one study performed on hepatic I/R in HFD-fed mice, administered irisin ameliorated hepatic injury, eradicated oxidative/ER stress and restored mitochondrial function in steatotic liver (Ref. Reference Zhang94). However, inhibition of kindlin-2, an important regulator of αVβ5 integrin function, by RNA interference offsets irisin's influence on aforementioned cellular mechanisms in cultured hepatocytes. Intestinal I/R can give rise to local and remote tissue/organ necrosis, rendering it to be vital in the progressively deteriorated state of an illness (Ref. Reference Jing95). Notably, liver is among the most vulnerable organs after intestinal I/R, since these two organs share a common anatomical passage (Ref. Reference Saidi96). In rats with concurrent intestine and liver injuries, Fan et al. unravelled decreased circulating irisin levels in contrast to increased hepatic irisin and inflammasome expressions after intestinal ischaemia perfusion (Ref. Reference Fan97). In addition, plasma irisin contents inversely correlated with hepatic damage severity and proinflammatory cytokines such as IL-6/tumour necrosis factor-(TNF-) α. Looking into the therapeutic potential, an analgesic dexmedetomidine can promote irisin expression to alleviate intestinal I/R-mediated liver injury by dampening NOD-like receptor protein 3 (NLRP3) inflammasome activation. Intriguingly, irisin-treated hepatic I/R old rats exhibited restored reparative capacity (Ref. Reference Bi98). This conspicuous benefit of irisin may be attributed to the activation of autophagy and in consequence telomerase activity via regulating phosphorylated JNK during hepatic I/R advancement.
Infection-associated liver injury
It has been well documented that severe acute pancreatitis can lead to the development of systemic inflammatory response syndrome, which imposes additional burden on multiple organs incorporating liver and the intestine (Refs Reference Cheng99, Reference Tan100). In L-arginine-induced AP mice, intraperitoneal injection of irisin can effectively mitigate intestinal cell injury by blocking ER stress and diminishing oxidative stress in combination with attenuated hepatic injury (Ref. Reference Ren101). Sepsis exhibits a leading cause of in-hospital mortality and morbidity, and early liver dysfunction serves as an independent risk factor for dismal outcomes and deterioration of sepsis (Refs Reference Rhee102, Reference Kobashi, Toshimori and Yamamoto103). A multitude of regulated cell death (RCD) has been linked to the development and progression of sepsis, including ferroptosis and pyroptosis. Ferroptosis is a type of RCD sensitive to iron accumulation which is then executed by glutathione peroxidase 4 (GPx4) deletion and subsequently overwhelming lipid peroxidation, while pyroptosis a lytic form triggered by invasive pathogens/tissue injury to amplify inflammation. Both described RCD modalities are involved in the pathogenesis of various liver diseases (Refs Reference Mao104, Reference Knorr, Wree and Feldstein105). In this context, the interplay between RCD and septic liver injury has been concerns of scientific endeavour. Wei et al. stated serum levels of irisin decrease in septic patients (12.85 versus 16.35 ng/ml), inversely correlated with disease severity, and in septic mice subjected to caecal ligation and puncture (CLP) (Ref. Reference Wei106). Overall, these observations indicated that there may be an irisin deficiency in sepsis. Moreover, ferroptosis were instigated in septic mice liver indicative of decreased GPx4 expression, Fe2+ accumulation and increased malondialdehyde content, whereas exogenous irisin treatment remarkably reversed their expression and mitigated mitochondrial impairment in morphology. These protective effects of irisin against ferroptosis were eliminated by inhibiting GPx4 or blocking αV integrin in lipopolysaccharide- (LPS-) treated hepatocytes and CLP-induced septic mice. Li et al. denoted that irisin intervention counters LPS-mediated septic liver injury by eradicating NF-κB signalling and downstream components responsible for inflammasome assembly and the activation of pyroptosis, such as caspase-1, NLRP3 and gasdermin D at both animal and cell levels (Ref. Reference Li107).
Hepatocellular carcinoma
HCC is one of the most common liver cancers worldwide. It carries a variety of risk factors, including alcohol addiction, concurrent NAFLD, obesity, chronic viral hepatitis infection, iron accumulation and family history (Ref. Reference Erstad and Tanabe108). A variety of multifunctional proteins have been proposed to contribute to HCC development, progression, metastasis and recurrence such as midkine, forkhead box O and osteopontin (Refs Reference Gowhari Shabgah109, Reference Yang110, Reference Wen111). Although great efforts have been carried out with respect to therapeutic strategy and supportive management for patients with HCC, such as surgery, chemotherapy, interventional therapy, immunotherapy and liver transplantation, the survival rate remains approximately 3–5% in developed countries. Thus, it is crucial to figure out more effective treatment by concentrating on novel molecular targets and underpinning mechanisms.
Basic researches
Shi et al. showed irisin enhances two HCC cell lines, namely HepG2 and SMCC7721, proliferation, migration and invasiveness in a dose-dependent manner through activating PI3K/Akt signalling pathway as evidenced by opposing impact after LY294002 (a PI3K inhibitor) administration (Ref. Reference Shi112). Mounting evidence has proved the PI3K/Akt pathway is involved in antiapoptotic activity during HCC development (Ref. Reference Sun113). Accordingly, treatment with irisin rendered protective impact on HepG2 apoptotic cells evoked by doxorubicin, which indicates the detrimental role of irisin pertaining to hepatic malignancy progression and decreased sensitivity to chemotherapy. Given the hallmark of tumour microenvironment on biological actions within HCC, it is appealing to modulate this peri-cancerous tissue as a novel therapeutic scale (Ref. Reference Pathria, Louis and Varner114). Notably, inflammation has become the hallmark as featured malignancy, and it is ascertained that tumour-associated inflammation facilitates the contribution of monocyte in HCC growth and metastasis. Tumour-associated macrophages (TAMs) play a pivotal role in crosstalk between tumour and stromal cells, actively participating in tumour-related inflammation (Ref. Reference Arvanitakis115). By using co-culture system embracing both HCC cells and TAMs, the same research group denoted HepG2/SMCC7721-derived FNDC5 overproduction results in increased M2 phenotype and decreased M1 phenotype in THP-1 induced macrophages (Ref. Reference Liu116). Further mechanistic investigation implied that high FNDC5-expressed HCC cells may influence the PPARγ/NF-κB/NLRP3 pathway in TAMs, in consequence, promoting M2 polarisation.
Clinical studies
Initially, Aydin et al. determined irisin immunoreactivity in various gastrointestinal cancers, and showed strong staining in all tissues except for liver (Ref. Reference Aydin117). No significant histoscores of irisin were observed between HCC and normal liver tissue. On the contrast, another two investigations reported discordant findings. Gaggini et al. found the highest expression of hepatic FNDC5 mRNA in patients with HCC relative to the transplantation donors (Ref. Reference Gaggini118). Moreover, hepatic FNDC5/irisin was transcriptional associated with sterol regulatory element-binding factor 1, SCD-1, proinflammatory cytokines (i.e. TNF-α/IL-6) and neurogenic locus notch homolog protein 1, all of which accounted for lipogenesis, inflammation and tumourigenesis. Mechanistically, the overexpressed FNDC5/irisin may exert compensatory effect and protective role to limit de novo lipogenesis during HCC progression as a paracrine hormone. Consistent with prior results, Shi et al. also indicated FNDC5 levels increase in the hepatic tissue of HCC patients (Ref. Reference Shi112). Intriguingly, no correlation was found between circulating irisin levels and hepatic FNDC5/irisin according to described studies, probably due to multiple origins of this myokine via distinct regulation mechanisms (Ref. Reference Varela-Rodriguez119). Nevertheless, a study recruiting 117 HCC participants and 102 healthy controls measured preoperative irisin contents by ELISA (Ref. Reference Zhang120). The authors demonstrated irisin levels (at μg/ml) decrease in patients with HCC undergoing hepatectomy on the basis of enrolled population as well as the Cancer Genome Atlas cohort, and supposed lower irisin expression may prevent patients from cachexia through restricting energy consumption. Another study further corroborated reduced serum irisin levels in HCC patients compared to controls (2.52 versus 4.46 μg/ml, P = 0.02) and clarified its negative correlation with more advanced HCC grade (Barcelona clinic liver cancer classification) and impaired live function reserve (Child–Pugh classification) (Ref. Reference Pazgan-Simon121). Supposable, a decrease of systemic irisin may promote invasive and metastatic behaviours of HCC by suppressing epithelial-to-mesenchymal transition and resultant fibrosis. Taken together, we are currently as its infancy to regard irisin as a surrogate associated with poor outcomes in the context of HCC taken into consideration of existing literature data (Ref. Reference Kim122).
Intrahepatic cholestasis of pregnancy
Intrahepatic cholestasis of pregnancy (ICP) is the most common pregnancy-specific liver disorder typically commencing in the late second and third trimester, which can dissolve within 48 h subsequent to delivery of the foetus (Refs Reference Williamson and Geenes123, Reference Geenes and Williamson124). It is characterised by unexplained mild to severe itching, increased serum bile acids and abnormal liver functional tests. Emerging evidence has suggested that more prevalent ICP is associated with abnormal metabolic profiles, including glucose intolerance and dyslipidaemia secondary to maternal aberrant bile acid homeostasis (Ref. Reference Menzyk125). Kirbas et al. conducted a cross-sectional study on 59 consecutive pregnant ICP women, and stated maternal systemic irisin levels are markedly higher in ICP patients relative to healthy controls (Ref. Reference Kirbas126). By using an established cut-off, that is 908.875 pg/ml with 72.5% sensitivity and 86.8% specificity, the risk of cholestasis appeared to be approximately 16 times higher (odds ratio = 16.972, P < 0.001). One interpretation built on these changes referred to irisin's adaptive and compensatory response to attenuate metabolic disturbances within ICP progression. Similarly, Chen et al. also demonstrated the values of irisin in the serum and umbilical vein blood increases with more aggressive ICP severity (Ref. Reference Chen, Li and Ma127). Serum irisin levels may serve as a proxy for diagnosing and indexing ICP subjects along with its role in diminishing oxidative stress and improving lipid metabolism in the pathogenesis of ICP.
Conclusions and future perspectives
Accumulating data have implicated liver diseases are on the steep rise and account for a large amount of deaths worldwide. Understanding the precise role of a target protein at the cellular, tissue and systemic levels is of upmost significance to dissect its potentials as therapeutic approach or prognostic/diagnostic surrogate. After a decade of extensive investigation and research on FNDC5/irisin, we are still surrounded by a gamut of open questions. FNDC5, as an overlooked transmembrane protein with a sleeping beauty-like fate in 2012, was kissed awake to be the precursor of irisin, known as a systemic exerkine/myokine with multiple origins. Since then, this hormone-like polypeptide has rapidly evolved into a component significantly involved in amazingly diverse diseases and metabolic dysregulations (Refs Reference Perakakis3, Reference Cao128, Reference Zhao129). In this article, we first concentrated on the structure–function relationship of FNDC5/irisin. Next, we comprehensively summarised the current knowledge and findings regarding pathogenic roles/therapeutic applications of FNDC5/irisin in the context of NAFLD, fibrosis, liver injury due to multiple detrimental insults and hepatic malignancy. Furthermore, the prominent molecules involved in underlying mechanisms and signalling pathways were highlighted. As a result, emerging evidence reveals FNDC5/irisin may act as a surrogate for diagnosing liver pathology, a sensitive biomarker for assessing damage severity, a predisposing factor for surveilling illness progression and a treatment option with protective/preventive effect, all of which are highly dependent on disease grading and contextually pathological features.
Although much progress has been made in our understanding of the contribution of FNDC5/irisin to the onset and development of liver diseases, several critical issues are supposed to be addressed for directing future investigation. First, there is a substantial gap between basic researches and clinical studies according to existing literature data, probably on account of flawed methods for detection and quantification. Additionally, the dosage of recombinant irisin applied to cells or injected to animals appears to exceed normal range among human beings in physiological context, hindering the smooth translation of available findings to clinical practice. Second, the medicinal impact of irisin to treat liver diseases is likely specific to cell types, with distinct effect on parenchymal versus non-parenchymal cells within liver tissue. More precise manipulation of hepatocytes subroutine is merited. Last, it is more practical and beneficial to target irisin in certain hepatic pathological condition and disease stage aimed at maximizing therapeutic efficacies and avoiding adverse events. Our capability to achieve selectivity when targeting this protein will be challenging in this direction.
Acknowledgements
None.
Author contributions
Xiaoyu Wang: writing – original draft, visualisation. Lihong Mao: writing – original draft, visualisation. Chaoqun Li: writing – original draft, visualisation. Yangyang Hui: conceptualisation. Zihan Yu: conceptualisation. Mingyu Sun: conceptualisation. Yifan Li: conceptualisation. Gaoyue Guo: conceptualisation. Wanting Yang: conceptualisation. Binxin Cui: conceptualisation. Xiaofei Fan: conceptualisation. Chao Sun: conceptualisation, writing – review and editing, supervision.
Financial support
This research received no specific grant from any funding agency, commercial or not-for-profit sectors.
Conflict of interest
None.