Introduction
In recent years, some modelling studies (e.g. Knorr & Lohmann Reference Knorr and Lohmann2003) and several palaeoclimate archives and reconstructions (e.g. Bianchi & Gersonde Reference Bianchi and Gersonde2004) suggested that the southern high latitudes (i.e. Antarctica and the Southern Ocean) might be much more important for the regulation of global climate than previously assumed. These studies proposed that abrupt climate changes that affected the Northern Hemisphere during the last glaciation and deglaciation were caused in response to more gradual changes in the southern high latitudes (Barker & Knorr Reference Barker and Knorr2007). Such a process implies the existence of teleconnections between the southern high latitudes and the rest of the world via oceanic circulation (EPICA Community Members 2006). In this context, Broecker (Reference Broecker1996) postulated that the southern Polar Front, rather than the Equator, could form the boundary between the “southern” (i.e. Antarctic) and “northern” climate modes. The southern Polar Front and its oceanographic manifestation (i.e. the Antarctic Polar Current), are closely associated with the southern Westerly winds. Reconstructing latitudinal shifts or changes in intensity of these southern Westerlies through time will thus help to better understand the role of the southern Polar Front in the regulation of global climate. The Kerguelen archipelago is located in a key position for such a study. It lies right in the middle of the Antarctic Polar Current (Barker & Thomas Reference Barker and Thomas2004) and in the core of the southern Westerlies (Kalnay et al. Reference Kalnay, Kanamitsu, Kistler, Collins, Deaven, Gandin, Iredell, Saha, White, Woollen, Zhu, Leetmaa, Reynolds, Chelliah, Ebisuzaki, Higgins, Janowiak, Mo, Ropelewski, Wang, Jenne and Joseph1996), whereas the Polar Front is situated just to the north of it (Belkin & Gordon Reference Belkin and Gordon1996). While the region around the archipelago (i.e. the Kerguelen Plateau) has been the focus of several palaeoceanographic studies (e.g. Whitehead & McMinn Reference Whitehead and McMinn2002), the islands themselves and their palaeoclimate records remained hitherto largely unexamined, except for a series of studies on peat bogs (e.g. Frenot et al. Reference Frenot, Gloaguen and Trehen1997a, Reference Frenot, Gloaguen, van De Vijver and Beyens1997b).
In this paper we present the results of an exploratory reflection seismic study of the sedimentary infill contained in Lac d'Armor, one of the large lakes on Grande Terre (unofficial name), the main island of the archipelago. These results show that the lake sediments have recorded the retreat of the glacier from the valley, as well as changes in the impact of the Westerly winds on the functioning of the lake. These results will further be used to select the optimal location for a long sediment core, to be taken in the future.
Study area
The Kerguelen archipelago is a sub-Antarctic group of islands, located in the southern Indian Ocean. The archipelago consists of one major island (‘Grande Terre’), several minor ones, about 300 islets and rocks, and outliers (Fig. 1). It is partly volcanic with a total area of 7215 km2 and a peak elevation of 1850 m (Mont Ross, unofficial name). ‘Mont Ross’ is also the youngest volcanic edifice (Fig. 1). The archipelago is the emergent part of a large igneous province, the Kerguelen Plateau. It was formed by large volume basalt eruptions c. 40 Ma ago. Since then, hot spot-type volcanism has remained important due to the persistence of a mantle plume.
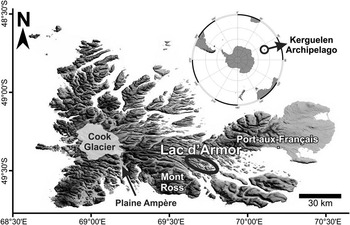
Fig. 1 Location of Lac d'Armor, Cook Glacier, ‘Mont Ross’ and Plaine Ampère on the ‘Grande Terre’ of the Kerguelen archipelago.
‘Grande Terre’ features high mountains and plateaus with deep lakes formed by glacier erosion. The coast is characterized by deeply incised fjords. Cook Glacier, covering 10% of the island, is a remnant of the Last Glacial Maximum (LGM) ice sheet (Fig. 1). The extent of the Kerguelen ice cover during the LGM is heavily debated (Hall Reference Hall1990). Some authors claim that the LGM ice extent was very limited and that the fjords and glacial valleys are the result of earlier glacial events (Bellair Reference Bellair1965, Nougier Reference Nougier1972). Hall (Reference Hall1984) suggested they are LGM in age. The lack of tills in some areas (Bellair Reference Bellair1965) and the absence of isostatic rebound (Bellair Reference Bellair1965) argues for the case of incomplete ice cover. There may have been major centres of ice growth in the higher areas to the west with a number of locations of only cirque glacier growth (Hall Reference Hall2004). A key consideration is the fact that localized changes to topography, induced by volcanic and tectonic activity throughout the Quaternary, may have had a significant influence on both glaciation and preservation of earlier glacial imprints (Hall Reference Hall2004).
Current climate is in general cold (a mean annual temperature of 4.5°C), windy (average 66 km h-1 winds in all months), heavily clouded and rainy (more than 3200 mm yr-1 on the western side, less than 800 mm yr-1 on the eastern side) (Frenot et al. Reference Frenot, Gloaguen and Trehen1997a). Currently, the ocean around the islands is ice-free, but during the LGM Antarctic sea ice might have reached as far north as this location (Gersonde et al. Reference Gersonde, Crosta, Abelmann and Armand2005).
Lac d'Armor (49°27′S, 69°42′E) is a medium-sized, fjord-type lake. It is 3.5 km long and on average 0.5 km wide. It is located south-west of Port-aux-Français (the only village on ‘Grande Terre’) and of the Golfe du Morbihan (Fig. 1). A shallow sill of only a few metres high and a couple of hundred metres wide, separates the lake from the Fjord Henri Bossière. Lac d'Armor has two major inlets, one in the north-west (Rivière du Nord) and one in the south (rivière du Diable, unofficial name), and one minor, nameless, inlet in the north. The southern river connects four lakes (lac Parcival (unofficial name), Lac Lancelot, lac d'Enfer (unofficial name), and lac d'Argoat (unofficial name)) and several small ponds with Lac d'Armor, which is the ultimate connection of this freshwater system with the ocean (Fig. 2).
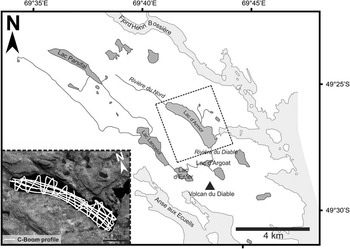
Fig. 2 Lac d'Armor and its catchment and the location of the seismic survey lines.
Methods
The seismic-reflection data on Lac d'Armor were collected in November–December 2006, during a challenging expedition that was organized in a collaborative effort between the Renard Centre of Marine Geology (RCMG) at Ghent University, the Université de Savoie and the Institut polaire français Paul-Emile Victor (IPEV).
The lake was surveyed with a C-Boom boomer, a lightweight, low-voltage seismic source that produces a signal with a dominant frequency of c. 1700 Hz (Fig. 2). A SIG single-channel streamer was used for the detection of the seismic reflections. It was towed as near as possible to the water surface to insure a high-resolution response. This streamer has an active length of 2.7 m, and consists of 10 hydrophones with 0.3 m spacing. The detected signal was pre-amplified in the streamer. During the expedition a Rockland band pass filter was used at 200 Hz high-pass and 60 kHz low-pass. The filtered signal was recorded in the Elics Delph-2 system on an ACME portable computer. Source, receiver and recording equipment were operated from a Zodiac inflatable boat. Navigation was undertaken by GPS.
Because of strong electrical noise, the data required significant post-processing. This was done using the ProMAX seismic data processing system at the RCMG, and involved the application of a frequency band-pass filter of 500–4000 Hz.
Seismic-stratigraphic interpretation was performed using KINGDOM Suite 7.5. Using the classic seismic interpretation criteria of Mitchum et al. (Reference Mitchum, Vai and Sangree1977), a seismic stratigraphy was established by identifying unconformity-bound seismic units and characterizing the seismic facies. The horizons representing the unconformities were picked and mapped in three dimensions throughout the lake basin, except in those areas where the seismic grid was not dense enough or where gas blanking (i.e. gas-rich sediment tends to absorb the energy from the acoustic wave - this leads to absence of reflections on seismic profiles and makes the identification of seismic horizons difficult to impossible) prevented mapping of the units. The interpreted horizon picks were subsequently exported from KINGDOM Suite 7.5 and used to create grids and maps with Golden Software Surfer 9, Global Mapper 8.3 and GMT 4.2. Using this mapped succession of infilling units, a reconstruction of the evolution of the dominant sedimentary processes in the lake through time can be made.
Time-to-depth conversion was done using a mean acoustic velocity of 1500 m s-1 for the water column. Due to the absence of a velocity profile for the subsurface a velocity of 1750 m s-1 was chosen for the sedimentary infill. This velocity is used here as an average (Eyles & Mullins Reference Eyles and Mullins1997) and is based on seismic velocity studies carried out in several glacial lakes, showing that velocities in such environments generally vary between 1500 m s-1 in the uppermost strata and 2100 m s-1 deeper in the sedimentary sequence (Finckh et al. Reference Finckh, Kelts and Lambert1984).
Results
Seismic units and seismic facies
The sedimentary infill comprises a series of sedimentary units with distinctly different acoustic character and distribution. The Lac d'Armor lake basin consists of two sediment basins or sub-basins: a southern sub-basin and a northern sub-basin (Fig. 3). The two sub-basins are separated by a shallow sill, which culminates at about 20 m below the lake surface. This sill hosts a small perched, sediment filled sub-basin (Fig. 3). The total sediment infill has a thickness of about 60 ms two-way travel time (TWTT) (c. 52 m) in both the northern and southern sub-basins, and the thickest sedimentary sequence is located in the central part of both basins.
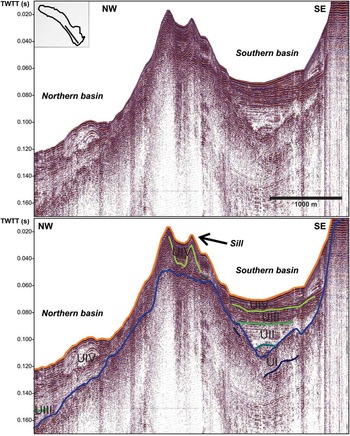
Fig. 3 Seismic profile of Lac d'Armor with the sill, which separates the northern and southern basin and the little basin on top of the sill. The top panel shows the original seismic line with no interpretation and has a small inset to indicate the location of the seismic profile in the lake. The bottom panel shows the interpretation.
In general, four units, each with a distinct seismic facies, can be identified in the lake basin infill. The acoustic basement (i.e. the facies below the deepest relatively continuous reflector visible on the seismic profiles) shows no acoustic stratification or internal structures and is excluded from this data description.
Unit I
The bottom Unit I directly overlies the acoustic basement and is characterized by a chaotic facies with discontinuous reflections (Figs 4 & 5). The reflection amplitudes are highly variable, ranging from very low, locally producing an almost transparent facies, to very high. The presence of these high-amplitude reflections distinguishes this unit from the quasi transparent acoustic basement. Unit I is separated from the acoustic basement and from the overlying Unit II by unconformities, the upper unconformity having a strongly undulating morphology and producing a very high-amplitude reflection. The bottom boundary was not imaged on all the profiles and could not be mapped throughout the lake basin. This also prevented the construction of an isopach map of the thickness of Unit I. In general, this unit is thicker in the deepest parts of the current basin. Here, this unit is about 10 ms TWTT thick (c. 8.5 m).
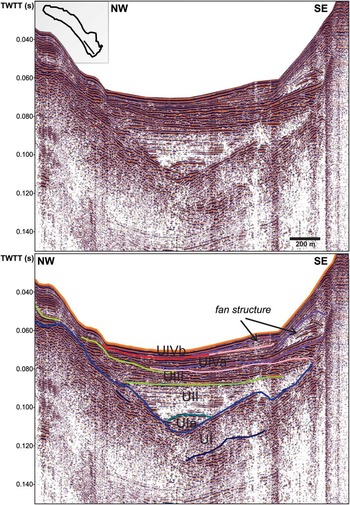
Fig. 4 Seismic profile of the southern basin of Lac d'Armor showing the entire basin infill with the fan structures at the southern edge of the sub-basin. The top panel shows the original seismic line with no interpretation and has a small inset to indicate the location of the seismic profile in the lake. The bottom panel shows the interpretation.
In the southern sub-basin, a sub-unit (Ia) can be distinguished, on top of Unit I (Fig. 4). This sub-unit is only present in the deepest part of the southern sub-basin. Unit I and sub-unit Ia are separated by an unconformity associated with a high reflection amplitude. The top boundary of sub-unit Ia is also an unconformity, which is associated with a low-amplitude reflection. The seismic facies of sub-unit Ia also comprises discontinuous reflections that are in general of higher amplitude than those of Unit I.
Unit II
The overlying Unit II is characterized by a facies with slightly undulating or (sub)-parallel and sometimes discontinuous, low-amplitude reflections, sometimes appearing as almost transparent. Overall, the reflection amplitudes of this unit are much lower than those in Unit I (Figs 4 & 5). This unit only occurs in the deepest parts of both sub-basins and smoothes the initial U-shaped morphology of the lake basin. The unit is thickest in the southern corner of the southern sub-basin and along the north-eastern edge of the northern sub-basin (Fig. 6). In those areas the unit is about 30 ms TWTT (c. 25 m) thick.
Unit III
The depocentre of Unit III is no longer focused in the deepest part of the basin. This unit mostly consists of sub-parallel, high-amplitude reflections (Figs 4 & 5). The boundary between Unit II and III is marked by a continuous, high-amplitude reflection. The boundary between Unit III and IV is also continuous and marked by a high-amplitude reflection. Unit III has generally a lower reflection amplitude in the northern sub-basin, than in the southern. An almost transparent wedge with low-amplitude chaotic reflections is present inside this unit (Fig. 5).
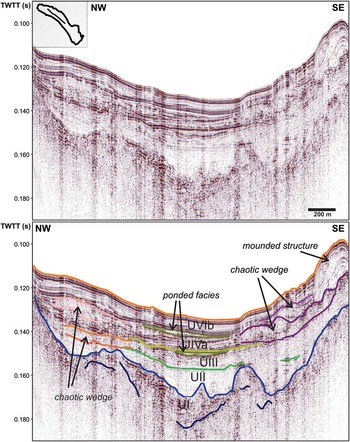
Fig. 5 Seismic profile of the northern basin of Lac d'Armor showing the entire basin infill with the mounded structure and the associated chaotic wedges at the southern edge of the sub-basin and the chaotic wedges and the associated ponded facies at the northern edge of the sub-basin. The top panel shows the original seismic line with no interpretation and has a small inset to indicate the location of the seismic profile in the lake. The bottom panel shows the interpretation.
The unit is rather thin in the southern sub-basin (on average 4 ms TWTT or c. 3.5 m). In this sub-basin the unit is the thickest at the southern edge (8 ms TWTT or c. 7 m) and it thins towards the north (Fig. 6). In the northern sub-basin the unit is thickest at the northern edge (12 ms TWTT or c. 10.5 m) and there is another thick depocentre in the south. The thickest northern depocentre thins towards the south, while the southern one thins towards the north (Fig. 6).
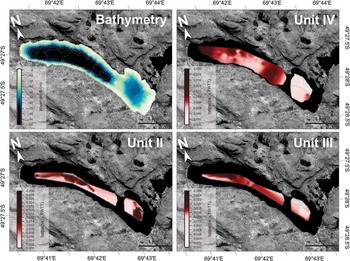
Fig. 6 Bathymetric map of Lac d'Armor based on the seismic profiles and single-beam echosounding data and isopach maps of unit II, III and IV illustrating the variations in thickness of each unit.
Unit IV
Unit IV is characterized by a facies with strong, continuous reflections (Figs 4 & 5) and by a series of mounded structures that are located in the southern corner of each sub-basin. The acoustic layering of Unit IV is often disrupted by prograding features from the southern or northern shore, where the river inlets are located (Fig. 4). These features have internally a more chaotic character, but there is some internal structure, mostly consisting of downlapping reflections. There is quite a sudden shift in how far these features reach down into the basin. This shift marks the boundary between two sub-units (IVa and IVb). In sub-unit IVb the prograding features reach deeper into the lake basin. This boundary is also marked by a difference in the height of the mounded structures in the southern corners of the sub-basins (Fig. 7). In sub-unit IVa, they are not as high and steep as in sub-unit IVb. In sub-unit IVb, the slopes of these mounded structures get much steeper and the structures are higher.

Fig. 7 Seismic profiles of Lac d'Armor showing the mounded structures at the southern edge of each sub-basin. The right hand panels show the original seismic lines with no interpretation and have small insets to indicate the location of the seismic profiles in the lake. The left hand panels show the interpretations.
In the lower part of Unit IV in the northern sub-basin there are also some chaotic wedges which thicken towards the sill and towards the northern shore. These wedges are most probably associated with thin ponded, transparent facies in the deepest parts of the northern sub-basin since they are located at the same stratigraphic level.
The unit is thickest at the southern edge of both sub-basins, i.e. at the location of the mounded structures (Fig. 6). In the southern sub-basin the unit is 5 ms TWTT (c. 4 m) thick, while it is up to 10 ms TWTT (c. 8.5 m) thick in the northern sub-basin. In this latter sub-basin the unit is also thick close to the inlet of the Rivière du Nord and along the northern edge of the sub-basin.
Unit IV is also present in the small basin located on top of the sill, which separates the two sub-basins. Here the reflections of this unit are always continuous and never disrupted. This basin has a continuous draping infill, which is about 10 ms TWTT (c. 8.5 m) thick.
Interpretation: bathymetry, seismic units and seismic facies
Bathymetry
Prior to this study the depths of the lakes on this archipelago were unknown. By combining the information obtained from the seismic profiles and from echosounding data, a bathymetric map was constructed for Lac d'Armor (Fig. 6).
Morphologically, Lac d'Armor can be defined as a fjord-like or perialpine lake (Cohen Reference Cohen2003). The lake has a rather complex bathymetry and structure, with two sub-basins: a southern sub-basin with a maximum water depth of 50 m and a northern sub-basin with a maximum water depth of 98 m (Fig. 6). These two bathymetric sub-basins are the same as the two sedimentary sub-basins. The two sub-basins are separated by a shallow sill (Figs 6 & 3). Remarkably, the lake is much deeper than the nearby Fjord Henri Bossière (Fig. 2), which has a depth of about 15–20 m (SHOM 1978).
Acoustic basement
The acoustic basement, lacking any internal structure, most probably consists of the basaltic rock of the Kerguelen Plateau (Nougier Reference Nougier1970a, Reference Nougier1970b, Giret Reference Giret1980). The unconformity between the acoustic basement and Unit I was most probably created by glacial action during previous glacial events (Bellair Reference Bellair1965, Nougier Reference Nougier1972). Consequently all units above this unconformity can be considered to represent different phases during and after deglaciation.
Unit I
Unit I is the oldest seismic facies that can be identified in the lake basin. This chaotic layer covers the acoustic basement with irregular patches of varying thickness. The chaotic character and irregular morphology of Unit I closely resembles the description of bottom facies from many glacigenic lakes in Europe and North America (Syvitski & Lee Reference Syvitski and Lee1997, Van Rensbergen et al. Reference Van Rensbergen, De Batist and Manalt1998, Eyles et al. Reference Eyles, Boyce, Halfman and Koseoglu2000). In glacigenic lakes such facies is interpreted as an ice-contact deposit. Since no continuous structures are present, Unit I will most probably be a diamictic deposit.
Sub-unit Ia is only present in the deepest part of the southern sub-basin. It has a elongated shape and although the facies is still rather chaotic, some internal reflections can be distinguished. All these characteristics are indications that sub-unit Ia might be the relic of a meltwater tunnel (Heirman et al. Reference Heirman, De Batist, Charlet, Moernaut, Chapron, Brümmer, Pino and Urrutia2011).
Unit II
This unit does not drape on top of Unit I, but is confined to the deepest parts of the basin, hereby smoothing all previously existing morphology on the lake floor. The reflections, however, can be traced over large areas of the sub-basins. Based on its stratigraphic position, low-amplitude facies and similarity with seismic facies in other glacigenic lakes (Syvitski & Lee Reference Syvitski and Lee1997, Van Rensbergen et al. Reference Van Rensbergen, De Batist and Manalt1998), this unit is interpreted to represent glaciolacustrine sediments deposited in a proglacial lake. A combination of density currents (underflows and turbidity currents) involving glacier meltwater can be invoked as a possible depositional mechanism. Such mechanism causes the sediment to focus in the deepest parts of the basin (Eyles et al. Reference Eyles, Boyce, Halfman and Koseoglu2000) as can be observed for Unit II.
Unit III
The sediments are no longer focused in the deepest part of the basin, but a sediment drape is slowly developing. This indicates a change in the sedimentation pattern and points towards a decrease in the concentration of suspended sediment in the inflowing water. The density of the inflowing sediment-loaded water is not large enough to continue as an underflow. Consequently, a suspended sediment plume is moved around the lake as an inter- or overflow (Van Rensbergen et al. Reference Van Rensbergen, De Batist and Manalt1998) from which particles settle down, forming a sediment drape on the bottom of the lake basin. This transition in the sedimentation pattern can be interpreted as the conversion from a glacier-fed lake to an alluvial-fed lake (Van Rensbergen et al. Reference Van Rensbergen, De Batist and Manalt1998). Consequently, the complete retreat of the glacier from the Lac d'Armor basin during the deposition of Unit III can be assumed. Based on the isopach map of Unit III (Fig. 6) the sediment-laden river water entering the lake was deflected to the southern edge of the lake in the southern sub-basin. The opposite is true for the northern sub-basin.
The chaotic wedges that occur in this unit have the typical characteristics of sedimentary mass-wasting deposits: a chaotic to transparent seismic facies and a lens-shaped intercalating geometry (Schnellmann et al. Reference Schnellmann, Anselmetti, Giardini, McKenzie and Ward2002). They might be the result of the collapse of over-steepened slopes. Although soft, water-saturated sediments on a steep slope will collapse easily on their own, here the deposits might be earthquake triggered. Several mass-wasting deposits occur on the same stratigraphic level. This implies that sediment accumulated on different slopes within the lake basin must have failed simultaneously. This points towards an external trigger, an earthquake (Schnellmann et al. Reference Schnellmann, Anselmetti, Giardini, McKenzie and Ward2002). Very little is known about the seismicity in the Kerguelen area. The archipelago is located far away from tectonic plate boundaries, but earthquakes (probably volcanic or hot spot related) have been reported during the last century (http://neic.usgs.gov/neis/epic/epic.html, accessed March 2007).
Unit IV
The prograding structures at the river mouths indicate a higher input of terrigenous material by the inflowing rivers than during the deposition of Unit III or that the inflowing sediment plume contains more coarse sediment, which will deposit more rapidly leading to the progradation of the river deltas. The mounded depocentres resemble drift deposits and suggest the presence of strong bottom currents, as has been observed in other lakes (Gilli et al. Reference Gilli, Ariztegui, Anselmetti, McKenzie, Markgraf, Hajdas and McCulloch2005, Anselmetti et al. Reference Anselmetti, Ariztegui, De Batist, Gebhardt, Haberzettl, Niessen, Ohlendorf and Zolitschka2009). Indirect observations in the field also support this interpretation: strong bottom current caused difficulty in coring this depocentre, drifting the corer before it reached the bottom. The increase in size (both in height and volume) of these mounds from sub-unit IVa to IVb might indicate an increase in the strength of the bottom currents. The river derived sediments appear to have been concentrated in fan-like depocentres, as opposed to the more uniform thickness distribution across the entire basin floor in Unit III.
The reflection amplitudes in this unit are much lower than those of similar deposits in the glacigenic lakes in Chile (Heirman et al. Reference Heirman, De Batist, Charlet, Moernaut, Chapron, Brümmer, Pino and Urrutia2011), and resemble the amplitudes encountered in lakes in the European Alps (Van Rensbergen et al. Reference Van Rensbergen, De Batist and Manalt1998) and Canada (Eyles & Mullins Reference Eyles and Mullins1997, Eyles et al. Reference Eyles, Boyce, Halfman and Koseoglu2000). In the Chilean lakes, the most recent sediments in the lacustrine infill are almost entirely autochthonous in origin, consisting predominantly of lacustrine algae (Bertrand et al. Reference Bertrand, Charlet, Charlier, Renson and Fagel2008), whereas the most recent sediment of the lakes in the European Alps and in this lake still have an important terrigenous component (Arnaud et al. unpublished).
The mass-wasting deposits in this unit, represented by the chaotic wedges, might again be the result of the possibly earthquake-triggered failure of over-steepened slopes. The ponded, transparent deposit that occurs in the deepest parts of the sub-basin at the same stratigraphic level as these mass-wasting deposits, are most probably homogenites, as has been observed in other lakes (Chapron et al. Reference Chapron, Beck, Pourchet and Deconinck1999). This argues in favour of a non-negligible seismic activity on Iles Kerguelen in the last millennia.
Discussion: environmental implications
The seismic stratigraphy of the sedimentary infill of Lac d'Armor depicts the evolution of the sedimentary environment in the lake basin during and after the last retreat from a glacier out of the basin. Very little is known about the timing of deglaciation in the Kerguelen archipelago. A study by Frenot et al. (Reference Frenot, Gloaguen, van De Vijver and Beyens1997b) of a peat bog in the Plaine Ampère in front of the current Ampère Glacier (Fig. 1), which is an outlet glacier of Cook Glacier, is the only ‘long’, dated record currently available. Currently, Lac d'Armor is about 35 km away from the limit of Cook Glacier (Fig. 1). The record of Frenot et al. (Reference Frenot, Gloaguen, van De Vijver and Beyens1997b) implies that the peat bog site was ice-free between 10 000 and 5000 cal yr bp. Most probably, the glacier had left the Armor basin prior to 10 000 cal yr bp. A study of sea ice extent in the South Atlantic indicates the first retreat of the Antarctic sea ice around 18 000–17 000 cal yr bp (Bianchi & Gersonde Reference Bianchi and Gersonde2004).
Evolutionary history of the Lac d'Armor basin
The extent of Antarctic sea ice is related to the position of the Polar Front (Gersonde et al. Reference Gersonde, Crosta, Abelmann and Armand2005), which currently passes virtually across the Kerguelen archipelago. A retreat of sea ice and consequently of the Polar Front towards the south (Gersonde et al. Reference Gersonde, Crosta, Abelmann and Armand2005), would have initiated warming in the region of the Kerguelen archipelago. Sometime between 18 000 and 10 000 cal yr bp, the Kerguelen ice sheet should have started to retreat. Initially, the retreating glacier was still present in the lake basin, resulting in the pro- or subglacial deposition of Unit II. After the glacier had retreated further, terrigenous material was delivered to the basin by meltwater streams. During the deposition of Unit III, the coarsest fraction of this terrigenous material was deposited closest to the inlet, while the finer fraction reached much further into the basin. The amount of sediment brought by these meltwater streams was probably lower than during deposition of the overlying Unit IV. The fan structures of Unit IV indicate a higher runoff and a larger supply of sediment to the Lac d'Armor basin.
Influence of the southern Westerlies
The onset of the construction of the drift structures indicates the formation of strong bottom currents. The observed pattern is reminiscent of sediment geometries found in lakes in Argentina (Gilli et al. Reference Gilli, Ariztegui, Anselmetti, McKenzie, Markgraf, Hajdas and McCulloch2005, Anselmetti et al. Reference Anselmetti, Ariztegui, De Batist, Gebhardt, Haberzettl, Niessen, Ohlendorf and Zolitschka2009). In these Argentinean lakes, these deposits have been interpreted to be the result of increasing wind stress. Considering the current extreme wind regime on the Kerguelen archipelago, which is dominated by the southern Westerlies, and with monthly averages of 66 km h-1 during the period of 1973–2007 at Port-aux-Français (http://www.ncdc.noaa.gov/, accessed May 2007), the wind seems a very likely culpret. Wind-induced waves have a great influence on sediment (re)distribution in lake systems, especially in shallow waters (Håkanson & Jansson Reference Håkanson and Jansson1983). A uniform wind stress, in this case imposed by the southern Westerlies, may also result in a large-scale steady-state lake circulation pattern. This is probably the case in Lac d'Armor, where a homogeneous surface temperature of 4°C has been observed during the field campaign (summer season) whereas the average air temperature was above 10°C. This surface temperature pattern can be interpreted as the absence of stratification due to permanent mixing of lake waters. Such a circulation pattern generally consists of a pair of counter-rotating gyres with downwind flow near the shores of the lake and upwind return flow in the deeper parts of the lake basin (Rao & Murty Reference Rao and Murty1970, Schwab & Beletsky Reference Schwab and Beletsky2003). The pattern of these upwind return flow bottom currents is strongly controlled by bottom topography (Håkanson & Jansson Reference Håkanson and Jansson1983). Consequently, it is not unlikely that the onset of the formation of these drift deposits is an indication of a strengthening of the southern Westerlies and the installation of a wind-driven steady-state circulation pattern. In Lago Cardiel, Argentina (49°S), the onset of southern Westerly intensification has been dated at 6800 cal yr bp (Gilli et al. Reference Gilli, Ariztegui, Anselmetti, McKenzie, Markgraf, Hajdas and McCulloch2005) and in Laguna Potrok Aike (52°S) around 6000 cal yr bp (Anselmetti et al. Reference Anselmetti, Ariztegui, De Batist, Gebhardt, Haberzettl, Niessen, Ohlendorf and Zolitschka2009). Since no age-depth model is as yet available for the sedimentary infill of Lac d'Armor, we cannot ascertain that the onset of the drift here was simultaneous with the onset of the formation of drift deposits in South American lakes. A strengthening of the southern Westerlies would also imply an increase in precipitation, which would explain the higher runoff indicated by the development of the river-fan structures in Unit IV. The boundary between sub-units IVa and IVb, might indicate that the southern Westerlies’ strength increased even more, leading to even stronger bottom currents and consequently higher and larger drift structures.
These wind-induced, strong bottom currents are responsible for the redistribution of the sediment brought in by the rivers. The sediment load from the rivière du Diable seems to be smaller than that of the Rivière du Nord (Fig. 2). This rivière du Diable is also much shorter than the Rivière du Nord. The rivière du Diable connects Lac d'Armor with four other lakes. Most of the terrigenous material of the catchment of these lakes will already have been deposited in one of these lake basins before it reaches the rivière du Diable (Fig. 2).
Conclusions
The seismic stratigraphy of Lac d'Armor illustrates that the lakes of the Kerguelen archipelago potentially hold an important sedimentological record of palaeoclimatic and palaeoenvironmental changes. The lake infill consists of a succession of sedimentary units that is strikingly similar to that found in other glacigenic lakes all over the world, depicting an evolution from ice-contact deposits (Unit I), to glaciolacustrine deposits in a sub- or proglacial lake (Unit II), lacustrine deposits predominantly fed by sediment-laden meltwater streams (Unit III) to open-lacustrine deposits (Unit IV). Units III and IV also comprise a number of mass-wasting deposits, which may have been triggered by seismic activity. Unit IV is characterized by the presence of fan-like deposits off the river mouths and of mounded features, which are here interpreted as drift deposits. These drift deposits imply the presence of strong, probably wind-driven bottom currents and are interpreted to result from a strengthening of the southern Westerlies some time during the Holocene. Similar drift deposits have been observed in Lago Cardiel and in Laguna Potrok Aike, both located in South America, at roughly the same latitude as Lac d'Armor. The onset of southern Westerly intensification was dated at 6800 cal yr bp in Lago Cardiel and at c. 6000 cal yr bp in Laguna Potrok Aike. A future long sediment core to be retrieved from a key location in Lac d'Armor should allow establishing the exact timing of the onset of southern Westerly intensification and reconstructing the behaviour of the southern Polar Front.
Acknowledgements
We thank Dries Boone and Roland Pagni for logistic and technical support, Wim Versteeg for expert assistance with the processing of the seismic data, and Dr Jasper Moernaut for stimulating discussions on earthquakes and lake sedimentation processes. We also kindly acknowledge the reviewers, Dr Michele Rubesco and Dr Brenda Hall, and the editor, Dr Alan Vaughan, for their useful comments and suggestions which significantly improved the manuscript. This study was carried out in the framework of the French-Belgian collaborative PEISACG program, supported by the Institut polaire français Paul-Emile Victor (IPEV), and of the Belgian-UK HOLANT project, funded by the Belgian Science Policy Office (BELSPO). Katrien Heirman was supported by a grant from the Fund for Scientific Research - Flanders (FWO - Vlaanderen).