Introduction
Ecosystem modification, climate change, and ongoing social injustices are major challenges for equitable and sustainable development (Sachs et al. Reference Sachs, Schmidt-Traub, Mazzucato, Messner, Nakicenovic and Rockström2019). Despite decades of action, recent global assessments show trends of rapidly declining biodiversity and difficulties in meeting globally agreed biodiversity targets (Díaz et al. Reference Díaz, Zafra-Calvo, Purvis, Verburg, Obura, Leadley and Chaplin-Kramer2020). Coupled with this biophysical loss, many rural and indigenous communities living with nature continue to be excluded from governing biodiversity (Artelle et al. Reference Artelle, Zurba, Bhattacharyya, Chan, Brown, Housty and Moola2019). At its core, the evolution of the biodiversity concept has been associated with the normative objective of ‘conserving’ (Soulé Reference Soulé1985). This has led to biodiversity becoming core to major international development targets, most recently the sustainable development goals (Griggs et al. Reference Griggs, Stafford Smith, Rockström, Öhman, Gaffney, Glaser and Kanie2014).
Philanthropic and corporate actors have embraced the practice of biodiversity conservation, often to fill the declining effectiveness of public agencies (Kumar Reference Kumar2012, Hamrick Reference Hamrick2016, Arlaud et al. Reference Arlaud, Cumming, Dickie, Flores, van den Heuvel, Meyers and Riva2018). Multiple human values influence how biodiversity is framed and conserved (Dedeurwaerdere et al. Reference Dedeurwaerdere, Admiraal, Beringer, Bonaiuto, Cicero, Fernandez-Wulff and Hagens2016), and a combination of modernist scientific thought and traditional indigenous knowledge interact to support holistic biodiversity outcomes (Hakkarainen et al. Reference Hakkarainen, Anderson, Eriksson, van Riper, Horcea-Milcu and Raymond2020). This makes biodiversity conservation an important concept for sustainable development, requiring critical insights from diverse knowledges and experiences to develop more impactful research and practice (Wyborn et al. Reference Wyborn, Kalas and Rust2019b).
A complex systems perspective has prompted increased recognition of the mutual interactions between humans and biodiversity. There is ample theory and empirical analysis of social-ecological systems (SES), and more recently the ‘relational turn’ has been offering new insights on the inseparability of human-environment connections (Fischer et al. Reference Fischer, Gardner, Bennett, Balvanera, Biggs, Carpenter and Daw2015, West et al. Reference West, Haider, Stålhammar and Woroniecki2020). Despite claims of this work to be focused on systems thinking, it omits the rather diverse epistemologies and methodologies from non-ecological systems thinking (Reynolds & Holwell Reference Reynolds and Holwell2020). This omission may pose limitations for revisiting how biodiversity is conceptualized and its conservation practised, as it constrains thinking to a specific way of understanding human-environment connections. Given the diverse and politically charged ways of influencing conservation, it is important to draw from existing interdisciplinary history of systems thinking.
We suggest that systems methodologies, as relevant to conservation, originate from two complementary histories, one focused on management and business sciences, and one focused on ecology and resilience. We posit that the two histories coalesce into ‘sustainability science’ and focus on systems leverage points as a heuristic for identifying interventions with different levels of transformative potential. Our contribution originated as a synthetic perspective, to stimulate the global Biodiversity Revisited initiative (Wyborn et al. Reference Wyborn, Kalas and Rust2019b). It complements other commissioned reviews on science, narratives (Louder & Wyborn Reference Louder and Wyborn2020) and futures (Wyborn et al. Reference Wyborn, Louder, Harfoot and Hillin press). The review was purposefully selective and designed to scope diversity and map available evidence from a topic in a synthetic way (Munn et al. Reference Munn, Peters, Stern, Tufanaru, McArthur and Aromataris2018), and was guided by our combined experience as researchers with backgrounds in agronomy, climate change, environmental economics and human ecology. Our selection of references is comprehensive and presents a historical context for the diversity of disciplines and epistemologies that have used the systems concept. The reader is invited to use this synthesis to reinterpret and further question linkages between systems concepts and biodiversity conservation.
We present a genealogy of systems thought from two major streams. The first is through a synthesis of systems thinking within a historical context of Western scholarship, outlining four historical ‘waves’ from the business and management sciences. The second is a parallel and complementary stream, evolving from ecology and resilience theories. At a point of convergence between the two, we focus on the seminal work of Meadow’s (1999) leverage points to discuss how focusing on different systems characteristics provides a guide for understanding the systemic dimensions of conservation. We conclude by suggesting how systems thinking can support future biodiversity conservation research, public policies, and corporate initiatives for sustainable and just futures.
Genealogy of systems thinking for conservation
Systems are interconnected sets of things – for example cells, molecules, trees – that produce a particular pattern and behaviour (Meadows Reference Meadows2008). A system is more than just an assemblage of components; it is an entity that changes through time and can have elements added or removed as the system changes. Systems thinking is a diverse way of knowing, with contrasting epistemologies and ontologies and a history of applications in a range of contexts and disciplines (Jackson Reference Jackson1985, Checkland & Scholes Reference Checkland and Scholes1999). Historically, systems thinking has built from a critique of reductionist science, which sought to measure observable patterns in a system, ultimately aiming to model or predict an outcome while limiting the focus on the broader complexity of the problem. As a response to this narrowness, systems thinking evolved to articulate ways of synthesizing and understanding how systems interact and influence outcomes (Midgley Reference Midgley2000). We organize this genealogy around two major streams that have provided a diversity of theory and practice in systems thinking.
Stream One: evolving hard and soft systems methodologies
The changing epistemologies from over a century of Western traditions of systems thinking moved from the objectivist and positivist lenses of engineering to structuralist and constructivist notions from critical scholarship. Midgley (Reference Midgley2006) provides a historical overview, harking back to the origins of systems thinking in Greek philosophy. It was not until the end of the Second World War that there was exponential intellectual progression in systems thinking and practice. Since the mid-twentieth century, the engineering, management and business sciences have evolved systems thinking over what can be retrospectively termed a series of waves (Midgley Reference Midgley2006). We summarize the evolution of these waves in Table 1.
Table 1. Four waves of systems thinking.
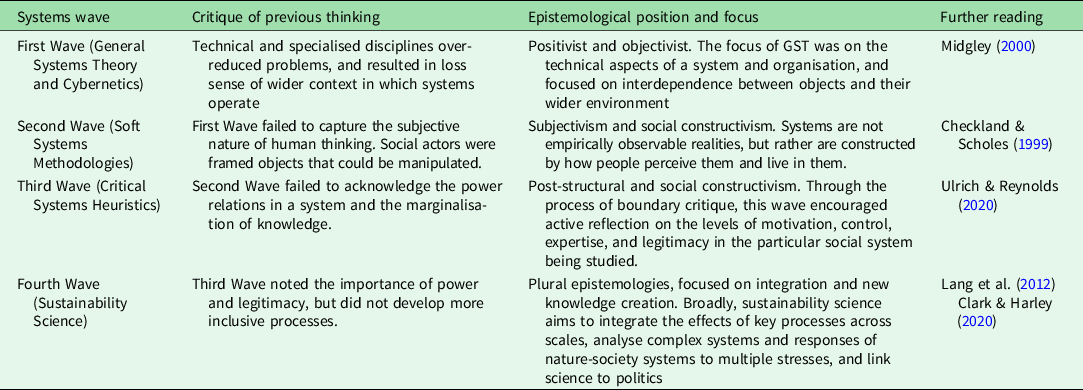
Core to the evolution were the notable ‘building blocks’ of theory. These emerged when a range of disciplines from organizational and management sciences identified gaps in the state of thinking and sought new methods and tools to advance systems thinking. Much of this theory was empirically tested in organizations (Checkland & Scholes Reference Checkland and Scholes1999), public health systems (Midgley Reference Midgley2000), and in the pedagogy of social learning in agricultural systems (Bawden & Packham Reference Bawden and Packham1993). The First Wave, with general systems theory and cybernetics as its building blocks, was highly influential in Europe during the 1970s–1980s (Midgley Reference Midgley2000). The focus here was to situate systems within their wider context, and prioritize quantification and reduction of systems towards entities that could be controlled, predicted and modelled. The computational modelling of feedbacks was supported by the engineering and mathematical fields of systems dynamics. While these efforts acknowledged the wider complexity of systems, methods and theories remained grounded in the positivist tradition. This led to increased critique of quantification as being assumed to represent reality and ignoring the subjective nature of people’s understanding and engagement with the systems around them (Midgley Reference Midgley2006).
The Second Wave built from this critique, and through the business and management research of Checkland & Scholes (Reference Checkland and Scholes1999), methodologies were developed to capture how people frame and understand systems differently, such as through participatory forecasting and rich-picturing (Bausch Reference Bausch2002). Despite actively engaging with stakeholders and their worldviews, the Second Wave failed to grapple with the power dynamics that condition social systems (Midgley Reference Midgley2006). This led to a further epistemological shift towards critical theory and post-structural thinking, creating a Third Wave, which focused on how power relations in systems can inhibit marginalized actors from being part of knowledge creation processes (Ulrich & Reynolds Reference Ulrich, Reynolds, Reynolds, Holwell and Milton Keynes2020). The Third Wave noted that though participation can lead to involvement of diverse groups, this does not mean marginalized actors can meaningfully participate due to fear of possible judgement (Ulrich & Reynolds Reference Ulrich, Reynolds, Reynolds, Holwell and Milton Keynes2020). This addition of a critical lens to systems thinking aims to understand the underlying norms, values and power structures that influence both the people doing the systems thinking and practice, and those impacted by the particular change (Jackson Reference Jackson1985). The focus on the underlying systems framings and outcomes encourages reflexivity on what the system is doing, but also on what it ought to be doing to deliver required changes.
A Fourth Wave emerged from the increasing interest in integrative sustainable development, and has since evolved into the broad field of ‘sustainability science’ (Griggs et al. Reference Griggs, Stafford Smith, Rockström, Öhman, Gaffney, Glaser and Kanie2014). This wave went beyond the three previous waves and concentrated on process and methods for knowledge co-production within specific biophysical and socio-political contexts. It has the normative purpose of improving the state of systems. Sustainability science is, by design, a systems science focused on connections between scales and systems (Clark & Harley Reference Clark and Harley2020). The field is ultimately faced with the challenge of creating innovation, transforming unsustainable pathways, and shaping collective visions for sustainable futures. The Fourth Wave is particularly relevant in a time where conservation practice must acknowledge plural values and knowledges that influence biodiversity conservation (Hakkarainen et al. Reference Hakkarainen, Anderson, Eriksson, van Riper, Horcea-Milcu and Raymond2020).
Stream 2: resilience and social-ecological systems
In parallel to the management and business disciplines, the fields of mathematics and ecology also advanced systems theory and methods, which became dominant in conservation parlance. Holling (Reference Holling1973) used the term ‘resilience’ as a measure to understand an ecosystem’s resistance to a disturbance and the speed by which equilibrium can be regained. Resilience thinking aligned closely with the First Wave’s cybernetics approach, focused on mathematical tools for modelling feedbacks and systems change. To expand from this reductionist approach, Holling distinguished between the concepts of ‘ecological resilience’ and that of ‘engineering resilience’, signifying a concept that is open to change and transformation (Arora-Jonsson Reference Arora-Jonsson2016). Ecological resilience rapidly became contested and debated in the literature, and rather than being a unitary concept, it was applied in multi-faceted and diverse ways. As the concept grew in the 1980s–1990s, theorizing resilience was critiqued for assuming a separation between human and environmental systems (Walker & Salt Reference Walker and Salt2006). This led to the new term ‘social-ecological resilience’ as a systems approach emphasizing that humans are part of nature, rather than an external and dominant force (Berkes & Folke Reference Berkes and Folke1998). Within SES thinking, the concept of ‘panarchy’ was used to acknowledge that in a particular system of interest at a focal scale, its resilience and change cannot be understood without understanding the wider dynamics and changes in scales above and below it (Walker et al. Reference Walker, Gunderson, Kinzig, Folke, Carpenter and Schultz2006).
Throughout the 2000s, SES thinking started to align with analysis of social structures (e.g., Ostrom Reference Ostrom2009) and organized social units and institutions (Young Reference Young2002). This expansion of SES viewed social systems as manageable entities, notably formal and informal institutions (Fabinyi et al. Reference Fabinyi, Evans and Foale2014). However, issues of complexity, agency and power relations within this type of systems analysis were ignored once more (Davidson Reference Davidson2010). In focusing on local populations as the unit of analysis, internal diversity of these groups and the wide political structures, poverty and market forces influencing resilience were ignored (Fabinyi et al. Reference Fabinyi, Evans and Foale2014). This was a notable omission given that at the same time the Third Wave outlined earlier was asking salient questions around power and legitimacy (Ulrich & Reynolds Reference Ulrich and Reynolds2010). These limitations are now being addressed with increasing analysis of power within SES, and the unintended impacts of insights from potentially transforming these states in pursuits of resilience and sustainability (Blythe et al. Reference Blythe, Silver, Evans, Armitage, Bennett, Moore and Morrison2018).
The diversity of systems thinking outlined in this genealogy makes it relevant and transferable to a range of disciplines, and adaptable to multiple social-ecological challenges. Given that the practice of conserving biodiversity is inherently normative and has competing philosophical foundations (Soulé Reference Soulé1985, Moon & Blackman Reference Moon and Blackman2014), systems thinking is particularly useful for grappling with the cross-cultural and multi-scalar dimensions of conflict and opportunity.
Systems leverage points and characteristics
To situate these historical streams of systems thinking, we now turn to a seminal framework for bridging the positivist and social constructivist ways of understanding systems. We draw from the systems leverage points framework proposed by Meadows (Reference Meadows1999) and its subsequent adaptation by Abson et al. (Reference Abson, Fischer, Leventon, Newig, Schomerus, Vilsmaier and von Wehrden2017) into four major system characteristics. A leverage point is defined as a place in the system where a small change could lead to a large shift in system behaviour (Meadows Reference Meadows2008). The broad concept grew out the 1970s Limits to Growth work (Meadows et al. Reference Meadows, Meadows, Randers and Berhrens1972), and provided much foundational thinking about the Earth systems’ interaction with social systems. This work was taking place at a time when cybernetics and resilience thinking were also evolving. In the leverage points framework, Meadows (Reference Meadows2008) distinguishes: interventions that are ‘shallow’ but have potential of creating change, and interventions that are ‘deep’ and have great potential of enabling a transformation. This spectrum of shallow to deep leverage points was organized around a scale of 12 leverage points, which increase in impact as they progress. In reorganizing the leverage points concept to suit sustainability science and transdisciplinary research more broadly, Abson et al. (Reference Abson, Fischer, Leventon, Newig, Schomerus, Vilsmaier and von Wehrden2017) synthesized the 12 leverage points into four major system characteristics: parameters, feedbacks, design and intent.
Fischer & Riechers (Reference Fischer and Riechers2019) argue that the value of leverage points to sustainability challenges lies in their ability to: link different modes of thinking, identify combinations of shallow and deep interventions; guide enquiry into the interactions among leverage points, and offer a transferable boundary object useful for problem analysis. The leverage points concept is transferable across contexts, and has been used to identify intervention points for such diverse contexts as global biodiversity targets and indicators, gender outcomes (Manlosa et al. Reference Manlosa, Schultner, Dorresteijn and Fischer2019), urban dynamics in a changing climate (Proust et al. Reference Proust, Newell, Brown, Capon, Browne, Burton and Dixon2012), and conservation-development policies (Scullion et al. Reference Scullion, Vogt, Winkler-Schor, Sienkiewicz, Peña and Hajek2016). Dorninger et al. (Reference Dorninger, Abson, Apetrei, Derwort, Ives, Klaniecki and Lam2020) found that in food-energy research, the deeper – and arguably more powerful – interventions remain understudied, indicating an ongoing opportunity for applying the concept to complex sustainable challenges.
The concept of leverage points is important for biodiversity conservation, as it provides a way of bringing together diverging framings, interventions, and interpretations of outcomes of a particular biodiversity problem. Efforts to bridge divides between Western, traditional and Indigenous knowledges can draw from the leverage points concept to help create new ways of thinking and practice (Huntington Reference Huntington2000). To demonstrate the application of the four characteristics of leverage points to biodiversity, we use interdisciplinary examples. These examples illustrate the opportunities for systems thinking to advance biodiversity conservation.
System characteristic 1: parameters and conservation
Parameters can represent system characteristics such as constants, system stocks (e.g., buffer size), flows (e.g., ecosystem services), and feedbacks (Meadows Reference Meadows2008). Quantification through measurement and modelling provide an initial – but necessarily simplified – understanding of the measurable dynamics of a system (e.g., population size, financial flows or rates of change). Parameter-based interventions have always been core to biodiversity conservation, given its foundations in the positivist scientific disciplines (Soulé Reference Soulé1985). One example of parameter-based systems analysis is the ‘fishbanks’ resource management simulation game (Meadows et al. Reference Meadows, Sterman and King2017). In this computer simulation, participants play the role of fishers seeking to maximize their net economic worth as they compete against other fishing fleets and deal with variations in fish stocks and their catch. Participants can buy, sell and order vessels, decide in which waters to fish, and negotiate with one another. Policy options, for example fishing quotas, auctions of new vessels, and fishing permits, are available to simulation instructors. The actions and interactions of fishermen and the policymaker with the dynamic resource base (the fish populations) trigger new system parameter configurations in real time, which in turn prompt the players of the game to respond. Over time, the system’s feedback loops are navigated, or ‘gamed’ by the players. The brief for players is to create ‘win-win’ situations (i.e., maximize net worth while keeping the fish stocks in good shape). The ultimate outcome of the game is either a sustained or a collapsed resource base, and insight into player’s behaviour. This simulation provides the opportunity to learn about the challenges of managing resources sustainably in a common pool resource setting, using measurable variables to understand systems change.
A limitation of parameter-based systems thinking is that it offers a necessarily simplified understanding of real systems (Anderies Reference Anderies2005). Choosing parameters involves abstraction from reality and pragmatic selection of system elements of immediate interest. When quantification and simulation are the key objectives, parameters that are difficult to quantify are often ignored by necessity, for example due to data paucity or when measurement costs are prohibitive (Hornberger & Cosby Reference Hornberger and Cosby1985). While parameter-based systems thinking and analysis helps understand biological processes and feedbacks, other types of system characteristics can get closer to the social and ecological interactions in systems (Meadows Reference Meadows1999). We emphasize that the intention or perspective with which a parameter-based tool is used is not necessarily a reflection of its efficacy – were the tool to be used with a more appropriate perspective, it might demonstrate its true power.
System characteristic 2: feedbacks and conservation
Feedbacks are ‘looping’ processes in a system, where change in one element will change another part of the system, impacting the originating element (Blythe et al. Reference Blythe, Nash, Yates and Cumming2017). An example of a feedback loop is the reduction of perennial plants due to herbicide application, which allows annual ragweeds to thrive. Ragweed is a fast-growing plant that can cause hayfever, and thrives in poor soils and patchy perennial cover. As herbicides are applied, perennial vegetation is also killed, reinforcing the ability of ragweed to grow. This is an example of a positive reinforcing feedback loop with an amplifying effect. Negative feedback loops, on the other hand, result in a damping effect (Dyball & Newell Reference Dyball and Newell2015).
Feedback loops may perpetuate in systems that continue to erode social and environmental well-being. Such perpetuation can be halted by an improved understanding of feedbacks, for example those between conservation initiatives and SES (Miller et al. Reference Miller, Caplow and Leslie2012). Institutional rigidity and inability to change historical practices can perpetuate a feedback processes, and erode social-ecological well-being. One example of this historical rigidity is the case of smallholder coconut production systems in the Philippines (Davila Reference Davila2020). A small group of affluent, post-colonial elites continues to own rural landscapes in the Philippines, and smallholder families lease land established in feudal colonial systems. Global trade policies have created policy incentives for converting smallholders’ land towards cash commodity production, notably sugar and coconut production (Davidson Reference Davidson2016). Despite farmers’ interest in planting trees, diversifying their crops, and reducing dependency on coconut incomes, they fail to see alternative support options (Davila Reference Davila2020). This traps them into a positive feedback loop, where the ongoing institutional support for cash commodities leads to smallholders’ belief that coconuts are a means for achieving food security (Figure 1, Table 2). This erodes opportunities for diversifying production to improve degraded landscapes and habitat in one of the world’s most biodiverse countries. Feedback loops can be easy to miss, and the interactions between scales of feedbacks can be hard to understand, creating a risk of developing maladaptive interventions that are blinded by the actual behaviours of a system (Proust et al. Reference Proust, Newell, Brown, Capon, Browne, Burton and Dixon2012). In resilience thinking, this is also called a ‘rigidity trap’, where the suppression of innovation and change perpetuates a statis state, resulting in an undesired regime shift in a system (Olsson et al. Reference Olsson, Galaz and Boonstra2014).

Fig. 1. An example of feedback processes in the Philippines coconut production system. R = reinforcing loop, B = balancing loop. See Table 2 for explanation of numbers and links.
Table 2. Feedback processes in Figure 1 explained.

System characteristic 3: design and conservation
This characteristic relates to the rules of the system, power of different groups to influence the change, and information flows that shape institutions (Meadows Reference Meadows2008). Design of interventions for social and ecological outcomes requires consideration of the various biophysical and socio-economic dimensions of the sustainability problem at hand. One example of designing social-ecological interventions is community-based fisheries management. In the South Pacific ocean, coastal fisheries provide up to 90% of animal source protein and 50% of incomes (SPC 2015). With a growing population and increasing pressures on fishing resources, there is an urgent need to support communities in the sustainable use of their resource. In 2015, with substantial efforts from governments, donors, and scientists, coastal fisheries management was re-envisioned to incorporate community-based ecosystem approaches and scalable ways of managing fisheries. A series of institutional structures and incentives are now in place to support this type of fisheries management (SPC 2015), for example through ‘report cards’ of multiple coastal fisheries that track management impacts.
As a system characteristic, design in a system has stronger potential of increased impact on the behaviour of a system than the parametric and feedback characteristics discussed above. Focusing on the design of a system often requires negotiation and deliberation among groups, a crucial element of conservation where there are frequent contested values and normative interests about the need for conservation (Colloff et al. Reference Colloff, Lavorel, Kerkhoff, Wyborn, Fazey, Gorddard and Mace2017).
System characteristic 4: intent and conservation
Meadows (Reference Meadows2008) argues that actors’ transcending paradigms and worldviews represent the most powerful opportunities to intervene in a system. As a system characteristic, intent is concerned with the underlying values and cognitive processes that set the direction of the design of a system. Shifting the intent of a system is ultimately concerned with the ontological and epistemological dimensions of systems that can enable a meaningful transformation. Intent is inherently normative, and helps us ask: what biodiversity exists to be understood, why is biodiversity to be conserved, what knowledge is used to make decisions, and how to interpret the available knowledge? While theoretical approaches to system transformation abound (Pelling et al. Reference Pelling, O’Brien and Matyas2015) and transformative change is identifiable in hindsight (Tanner & Bahadur Reference Tanner and Bahadur2012), there are fewer examples of the practice of enabling processes that facilitate multi-stakeholder consideration of the intent of the transformation in a system.
In Australia, a multi-stakeholder process to articulate transformed intent of systems was facilitated over a 10-year period in New South Wales (NSW) (Jacobs et al. Reference Jacobs, Lee, Watson, Dunford and Coutts-Smith2016, Sebastian & Jacobs Reference Sebastian, Jacobs and Brears2020). NSW is home to some of Australia’s unique biodiversity, which continues to be threatened by increased extreme weather events such as fire and prolonged droughts. The Enabling Regional Adaptation program (ERA) sought to provide information for strategic regional planning for adaptation of government service delivery to climate change (NSW Government Reference Government2018). Through a range of participatory processes, ERA linked local knowledge of climate change impacts and adaptation actions with the centralized planning processes of the NSW Government at regional scale. This was done because the impacts of climate change are predicted to vary greatly across regions and vulnerable groups, and the capacity to adapt is distributed unequally (Biesbroek & Lesnikowski 2018). The multi-stakeholder process led to an envisioning of possible transformations in a system, organized in the form of qualitative transition models (e.g., Figure 2) that seek to coordinate adaptation actors (in this case, public sector decision-makers) that are geographically and institutionally dispersed. These transition models present a vision for a ‘transformed system’. Each model focuses on a regional ‘system’ that, in the opinion of participants, needs to transform to maintain regional resilience. The transformed system becomes a guiding vision (Kooiman & Jentoft Reference Kooiman and Jentoft2009) rather than a prescription that emerges from continuous incremental change (Termeer et al. Reference Termeer, Dewulf and Biesbroek2017).

Fig. 2. Model of system transformation co-designed with public sector stakeholders at regional scales (NSW Government, 2008).
The process sought to help articulate a novel system’s intent to support healthy, adaptive and resilient ecosystems that contribute to regional liveability. However, many aspects of the transformation rely on the resolution of existing tensions within regional communities. Looking at this as a system identifies the tensions across the regional change models, which include agriculture, land use planning, tourism, and settlements and infrastructure (NSW Government Reference Government2019). The ERA models identify a nexus of conflict over the need to address regional biodiversity loss, the lack of co-ordinated land use planning, the drivers of economic development (manifesting as expansion of agriculture and exploitation of the environment for tourism) and the enhancement of infrastructure to support regional population growth (NSW Government Reference Government2018, NSW Government Reference Government2019).
For biodiversity in the NSW context, extensive engagement with stakeholders is needed to implement pathways of change that lead to new forms of governance supporting place-based and community-led actions (at the micro-scale) and challenge dominant paradigms about the value of nature (at the macro-level) (Sievers-Glotzbach & Tschersich Reference Sievers-Glotzbach and Tschersich2019). To operationalize the transition, systems need to be designed, and if necessary, adapted to fulfil the transformed vision. The intent component of the leverage point’s framework can guide analysis of how governance structures, community-led activities, and a range of institutions are set up to enable the implementation of alternate visions for a system.
Discussion
We have synthesized two major genealogies of systems thinking, one emerging from business and management sciences, and one from ecology and resilience thinking. The systems concept of leverage points, and its four major characteristics, offers a heuristic for drawing from the diversity of methods that emerged from the two major genealogies of systems thinking covered in this paper. Biodiversity conservation is practised by multiple actors in interacting sub-systems and across contexts, making systems thinking useful for managing conflicting perspectives and navigating the biodiversity problem.
Here, we discuss the ‘performance’ of conservation by three major actor groups that work across the different characteristics of the biodiversity problem. These groups, rather than being defined by neatly bounded paradigms and epistemologies, each represent diverse ranges of views, ways of knowing, and normative intent. Systems thinking as presented in this paper can be harnessed to address diversity and manage conflict both between and within these groups.
Revisiting biodiversity research
Universities, non-government organizations and government natural resource agencies all contribute to biodiversity research, making conservation science a boundary discipline spanning a range of sectors (Cook et al. Reference Cook, Mascia, Schwartz, Possingham and Fuller2013). Additional knowledges, notably Indigenous and traditional knowledges, also have specific contributions to make to the traditional field of conservation research. The high levels of uncertainty in complex problems like biodiversity require tools to help grasp competing epistemologies and practices (Scoones & Stirling Reference Scoones and Stirling2020). We presented sustainability science as a transdiscipline that combines different systems thinking tools, offering opportunities for revisiting how conservation research is done (Lang et al. Reference Lang, Wiek, Bergmann, Stauffacher, Martens, Moll and Swilling2012).
Conservation research has underlying, sometimes competing intents, depending on funding sources and researcher interests. Global mapping and modelling offers a description of the parameters and feedback processes in conservation, whereas social science conservation research helps clarify the root causes of problems and possible interventions (Bennett et al. Reference Bennett, Roth, Klain, Chan, Christie, Clark and Cullman2016). Insights from the different streams of systems thinking can help advance conservation as a sustainability science – for example through the participatory systems methods developed by Checkland & Scholes (Reference Checkland and Scholes1999), or through participatory identification of resilience and adaptive capacity of a SES.
One tool that can leverage systems thinking to expose underlying paradigms is the co-production process embedded in sustainability science (Lang et al. Reference Lang, Wiek, Bergmann, Stauffacher, Martens, Moll and Swilling2012; Wyborn et al. Reference Wyborn, Datta, Montana, Ryan, Leith, Chaffin and Miller2019a). This process engages multiple stakeholders in producing multiple outcomes, including knowledge, decisions, and impact-oriented actions (Wyborn et al. Reference Wyborn, Datta, Montana, Ryan, Leith, Chaffin and Miller2019a; Norström et al. Reference Norström, Cvitanovic, Löf, West, Wyborn, Balvanera and Bednarek2020). For example, in the Biodiversity Revisited initiative, co-production enabled a challenging reflection process for identifying how competing values and paradigms influence the ultimate intent of biodiversity conservation research (Contestabile Reference Contestabile2020). The emergent research agenda and process from the project proposes that a focus on plurality of paradigms is important for equitable and just futures (Wyborn et al. Reference Wyborn, Montana, Kalas, Davila, Clements, Izquierdo and Knowles2020, Wyborn et al. Reference Wyborn, Louder, Harfoot and Hillin press), making systems methodologies salient for conservation research.
Revisiting biodiversity policy
Policy and decision-making for future biodiversity is done under inherent uncertainty, following non-linear pathways influenced by a mix of institutional settings, political systems and available knowledge (Evans et al. Reference Evans, Davila, Toomey and Wyborn2017). In an extensive analysis across six languages, Rose et al. (Reference Rose, Sutherland, Amano, González-Varo, Robertson, Simmons and Wauchope2018) found that the use of biodiversity knowledge by decision-makers is hindered by short-termism, whereas conservation is not a short-term priority, poor engagement between decision-makers and scientists, and the high levels of uncertainty in conservation problems. The entire question on how and whether biodiversity should be conserved at all is continuously contested in research, posing major challenges for decision-makers with political mandates, budgetary cycles and specific priorities (Dovers Reference Dovers2005). Biodiversity policies are formalized in institutions that establish the formal binding rules and laws that set the boundaries of the socio-political conservation system, and tend to reinforce and resist change to the dominant intent they set for this system (Abson et al. Reference Abson, Fischer, Leventon, Newig, Schomerus, Vilsmaier and von Wehrden2017).
A systems thinking lens to biodiversity problems can support working across uncertain contexts with different time horizons. In the ERA example, multiple sub-systems were identified by policymakers as one-way incremental interventions that could help guide an overall transformation in the system (Jacobs et al. Reference Jacobs, Lee, Watson, Dunford and Coutts-Smith2016). Addressing combinations of systems characteristics helps to identify decisions on the immediate requirements of a policy action. Such combinations can also help navigate the often-perverse nature of institutions that are path dependent and perpetuate paradigms (Abson et al. Reference Abson, Fischer, Leventon, Newig, Schomerus, Vilsmaier and von Wehrden2017). Biodiversity conservation policies frequently include trade-offs between interest groups and jurisdictions (Leventon et al. Reference Leventon, Schaal, Velten, Loos, Fischer and Newig2019). The policy process often requires negotiation and decisions of the ‘winners and losers’ in a particular situation, and has inevitable dimensions of politics and conflict within it. Analysis of policy settings and decisions based on leverage points and systems characteristics can help identify and reframe the ultimate institutional paradigm influencing the policy instruments used to conserve biodiversity (Dovers Reference Dovers2005). The aforementioned systems concepts provide both analytical and practical ways of identifying conflict and commonalities in the groups involved in public policy setting. Systems practice from the management sciences can include different stakeholders to enable shared understandings of different envisioned systems outcomes (Davila et al. Reference Davila, Dyball and Amparo2018, Jacobs et al. Reference Jacobs, Boronyak and Mitchell2019).
Revisiting private conservation
Private actors and philanthropic organizations are increasingly working towards conservation outcomes, setting specific paradigms on conservation (Vatn Reference Vatn2018). Private conservation efforts are underpinned by paradigms and design interventions, which likely differ from those employed by public agencies or research organizations. This, however, does not make them less legitimate in the biodiversity knowledge system. Philanthropic investments are now common, supporting a wide range of conservation initiatives (Commonwealth of Australia 2007), and private land conservation is increasing as a way of conserving terrestrial biodiversity on private properties (Raymond & Brown Reference Raymond and Brown2011). A review of private land conservation literature has found that there is a strong emphasis on conflicting values and a need to improve the overall governance effectiveness to support equitable and just conservation outcomes (Gooden Reference Gooden2019). A systems thinking approach to improving the role of private actors can help navigate how greater inclusion of conflicting values can form part of conservation decisions, ultimately helping shape new shared paradigms on the role of corporate actions on conservation. Private land managers have their own motivations for protecting biodiversity on their private property, and these influence how conservation programmes are designed and implemented on private lands (Moon & Cocklin Reference Moon and Cocklin2011). The participatory tools from the Second Wave of systems thinking provide an approach for understanding the power dimension of who has been included and excluded from such systems. Conservation practice has strong colonial implications, where Indigenous knowledge has been systematically excluded from conservation practice (Domínguez & Luoma Reference Domínguez and Luoma2020). Privatizing land has clear influence on power dynamics where larger powerful landholders can exclude less agile or able actors from private conservation efforts. Ulrich’s and Reynolds’s (2010) critical system heuristics approach, focused on examining power relations, supports analysis of the systemic power dimensions of these types of issues. Furthermore, understanding the paradigms and worldviews that motivate private landholders is critical when designing interventions that are to foster different outcomes. At a corporation level, impact investment is growing to support conservation, where companies use funds with the intention of generating measurable social and environmental impact alongside a financial return. Between 2010 and 2015, global impact investments increased from US $2.5 billion to US $15.2 billion (Watts & Scales Reference Watts and Scales2020). Impact investment uses instruments from the financial sector, and thereby embeds the underlying neoliberal paradigm within it. Critically applying thinking from the systems genealogies helps identify how the dominant paradigms might include and exclude different actors in the conservation context in which investments take place.
Conservation systems thinking in practice
Earlier, we stated that systems thinking can be harnessed to address diversity and manage conflict both between and within these groups. While our argument has emphasized the ability of systems thinking to flexibly offer ‘tools’ for predefined situations, we do not merely recommend a ‘best fit’ contingency approach. When it comes to the practice of systems thinking for biodiversity conservation, best practice should always be given priority over best fit: where ‘best practice’ privileges tools irrespective of context, ‘best fit’ privileges particular tools for particular contexts (Reynolds Reference Reynolds and Patton2015). Conservation practice has an opportunity to unfold a continually adaptive and innovative use of systems thinking tools as conceptual constructs among all of its users in relation to changing conservation contexts, or ‘conservation thinking-in-practice’. In the face of the current biodiversity crisis, such thinking-in-practice would be timely and relevant both within and across the three actor groups that we have discussed above. Biodiversity researchers, policymakers and private sector practitioners each embody not one monolithic paradigm, but rather a range of different paradigms. The power and value of systems thinking across the boundaries between research, policy, NGOs and the private sector can help us surface such paradigms and associated worldviews, ways of knowing, and normative intent, and ensure stronger alignment for concerted action towards biodiversity conservation.
Conclusion
Systems thinking has much to offer to the science, policy and practice of biodiversity conservation. Systems thinking draws from a rich diversity of ontologies, epistemologies, theories and methods. There is a diverse genealogy of systems theories and methods, and some continue to evolve today. This diversity has potential to equip the biodiversity conservation community with a spectrum of conceptual and practical tools for better understanding and managing biodiversity. The complementarity in thinking between management and business science systems thinking and resilience thinking provides avenues for improving research, policy and corporate practices in conservation. We have reviewed this systems genealogy and applied a leverage points perspective to selected examples and case studies to guide future use of systems thinking in conservation. While these systems characteristics represent broad categories, they provide a workable framework for organizing the various pathways that exist to conserve biodiversity. Embracing systems thinking and its associated tools and methods is an urgent priority for the competing interests working for conservation outcomes. With mounting pressures on the world’s biodiversity, systems thinking can help to overcome both epistemic and practical barriers, and moreover inspire those practicing biodiversity to enable transformative change in the human and ecological systems that depend on biodiversity.
Acknowledgements
Thank you to the Luc Hoffman Institute for the opportunity to contribute to the Biodiversity Revisited initiative and for the comments and review of this work. Thank you to Megan C. Evans for the thoughtful and considered review of the working draft and the subsequent discussions.
Financial support
This work was supported by the Luc Hoffman Institute.
Conflict of interest
None.