1. Introduction
The Famennian (Late Devonian epoch) is a stage marked by important changes in the biosphere and a period of recurrent environmental perturbations. Seven global events occurred over an interval of c. 15 Ma. At its base, the Frasnian–Famennian (F/F) boundary is known as one of the five most drastic episodes of biological extinction of the Phanerozoic and has recently also been considered as a biodiversity crisis due to speciation rate drop (Racki, Reference Racki, Over, Morrow and Wignall2005; Stigall, Reference Stigall2012). This episode is called the Kellwasser event. At the top of the Famennian, the Devonian–Carboniferous boundary is also characterized by a worldwide extinction event called the Hangenberg event (Walliser, Reference Walliser1984). Other global events occurred during the Famennian such as Nehden (House et al. Reference House1985; Schülke, Reference Schülke2003; Schülke & Popp, Reference Schülke and Popp2005), Enkeberg (House, Reference House1985; Becker, Reference Becker1993), Condroz (Schülke & Popp, Reference Schülke and Popp2005), annulata (Becker, Reference Becker1993; Schülke & Popp, Reference Schülke and Popp2005) and Dasberg (Becker, Reference Becker1993; Hartenfels, Reference Hartenfels2010; Marynowski, Filipiak & Zaton, Reference Marynowski, Filipiak and Zaton2010). They are generally marked by palaeontological and/or environmental changes (Walliser, Reference Walliser1996; House, Reference House2002).
Among these events, the Kellwasser, annulata, Dasberg and Hangenberg are related to worldwide anoxic events. In most of the cases these anoxic events have been studied when easily identified based on facies deposits, i.e. when black shales are present (Becker, Reference Becker1993; Racka et al. Reference Racka, Marynowski, Filipiak, Sobstel, Pisarzowska and Bond2010). The Condroz event is not paired with anoxia spread, but with termination of black Cheiloceras shale due to regression (Walliser, Reference Walliser1996). Independently of any lithological signature, following the Late Devonian conodont zonation established by Ziegler & Sandberg (Reference Ziegler and Sandberg1990) all these events can be dated and globally correlated. Assumed to be synchronous at the global scale, these events are sometimes related to a glacioeustatic mechanism (Sandberg, Morrow & Ziegler, Reference Sandberg, Morrow and Ziegler2002; Racka et al. Reference Racka, Marynowski, Filipiak, Sobstel, Pisarzowska and Bond2010) and generally to sea-level fluctuations (Walliser, Reference Walliser1996).
Sea-level fluctuations through the Devonian are well known on the east European platform (Alekseev, Kononova & Nikishin, Reference Alekseev, Kononova and Nikishin1996) and at the scale of the Euramerica supercontinent (Johnson, Klapper & Sandberg, Reference Johnson, Klapper and Sandberg1985; Johnson & Sandberg, Reference Johnson, Sandberg, McMillan, Embry and Glass1989; Sandberg, Morrow & Ziegler, Reference Sandberg, Morrow and Ziegler2002; Haq & Schutter; Reference Haq and Schutter2008; Racka et al. Reference Racka, Marynowski, Filipiak, Sobstel, Pisarzowska and Bond2010). Because of the absence of continuous sections through the Famennian on the north Gondwana-related area (Galatian superterrane, Stampfli et al. Reference Stampfli, Hochard, Vérard, Wilhem and vonRaumer2013), sea-level fluctuations are poorly constrained.
The present study focuses on the tracing of Famennian sea-level fluctuations and related events in the Montagne Noire (France). Previous biostratigraphic works at Col des Tribes (CT) were principally based on goniatites (J.D. Price, unpubl. thesis, University of Hull, UK, 1982; Becker, Reference Becker1993; Becker & Weyer, Reference Becker and Weyer2004). The presence of variously abundant conodonts in a series of reconnaissance samples was discovered by G. Klapper in the early 1980s (unpublished results) but detailed conodont biostratigraphy of the entire Famennian sequence remained largely incomplete (Boyer et al. Reference Boyer, Krylatov, Fèvre and Stoppel1968). These works however suggested that there were no major biostratigraphical gaps or discontinuities allowing for both microfacies investigations and evaluation of conodont faunal changes to be made throughout the entire Famennian interval.
In this regard we aim to: (1) establish a detailed stratigraphy based on conodont biozonation; (2) establish a model of depositional environments using the evolution of microfacies; and (3) use conodont assemblages to obtain an insight into particular sea-level changes.
2. Geological setting
The Col des Tribes section is located in the Montagne Noire, France (Fig. 1). The Montagne Noire is part of the external tectonic zone of the Variscan belt in southern France (Matte, Reference Matte1991; Ballèvre et al. Reference Ballèvre, Bosse, Ducassou and Pitra2009; Faure, Lardeaux & Ledru, Reference Faure, Lardeaux and Ledru2009; Fig. 1a), composed of a south-facing pile of low-grade to non-metamorphic rocks (Arthaud, Reference Arthaud1970). Even if the palaeolocation of the Montagne Noire during Late Devonian time is still under debate, most authors consider it was on the northern-most margin of Gondwana or on an intermediate block rifted off Gondwana during Early Palaeozoic time and located between Laurussia and Gondwana (Tait, Bachtadse & Dinarès-Turell, Reference Tait, Bachtadse and Dinarès-Turell2000; Golonka, Reference Golonka, Kiessling, Flügel and Golonka2002; Nysaether et al. Reference Nysaether, Torsvik, Feist, Walderhaug and Eide2002). During Famennian time, the Montagne Noire should have been part of the Galatian superterrane which was bouded to the SE by the Palaeotethys Ocean and bounded to the NW by the Rheic Ocean (Stampfli et al. Reference Stampfli, Hochard, Vérard, Wilhem and vonRaumer2013). The studied section belongs to the inverse limb of the Montpeyroux nappe which suffered very low-grade metamorphism (anchizonal to non-metamorphic, Wiederer et al. Reference Wiederer, Königschof, Feist, Franke and Doublier2002). It is composed of oxidized sediments assumed to have been deposited on an outershelf submarine rise (Engel, Feist & Franke, Reference Engel, Feist and Franke1982).

Figure 1. Location of the Col des Tribes section (Montagne Noire, south France). Maps showing: (a) the position of the Montagne Noire in southeastern France; (b) the Col des Tribes section near Causses and Veyran; and (c) the position of the sampled levels (modified from House, Reference House1985; Becker, Reference Becker1993). F/F — Frasnian/Famennian boundary; D/C — Devonian/Carboniferous boundary.
In contrast to Palaeozoic rocks of the Pyrenees and southern Alps, those of the Montagne Noire did not suffer any alpine deformation which explains the comparatively good preservation of fossils and sedimentary fabrics. The investigated section near Col des Tribes is situated on the eastern slope of the Mont Peyroux summit (Fig. 1), IGN sheet 1:25000 St Chinian 2544E, between 43°29′26.65″N, 3°5’26.28″E and 43°29′23.94″N, 3°5′27.50″E.
3. Methods
3.a. Palaeoenvironments
A total of 71 samples were collected (Figs 1b, 2a), marked in the field by pink numbers. Bed numbers mostly coincide with those formerly marked by House & Price (in Becker, Reference Becker1993, pp. 91–92). Many covered intervals were made accessible by hand digging. A total of 71 thin-sections were cut for microfacies analysis from the same samples used for conodont studies. Based on field and microscopic observations (semi-quantitative estimate of the relative abundance of bioclasts) and palaeontological qualitative results, four facies types are distinguished (Flügel, Reference Flügel2004). As facies are poorly diversified at Col des Tribes where the carbonates generally yield few bioclastic debris (only one sample provided up to 15% of bioclasts), we chose the following ratios of bioclast abundance to illustrate vertical changes along the section: very rare: <1%; rare: 1–2%; frequent: 2–6%; and abundant: 6–15%. In order to characterize the depositional environment, we introduced the ratio N/(N+B) where N is the abundance of debris of nektonik organisms, B is the abundance of benthic organisms and N+B represents the total abundance of bioclasts (nektonik + benthic) (see Flügel, Reference Flügel2004 for palaeoenvironmental setting of organisms). Even if such a ratio is dependent upon very different palaeoenvironmetal parametres (dysoxia, nature of bottom, currents, etc.), we consider it useful for discrimating large-scale proximal to distal trend changes. The most frequent pieces of benthic organisms are issued from thin-shelled and less than 500-μm-long ostracods, echinoids (crinoids), trilobites, brachiopods and, to a lesser extent, gastropods, bivalves, silicified foraminifers, sponges and possibly microbes. Nektonic organisms are represented by cephalopods (ammonoids), thin-shelled pelagic molluscs (‘filaments’ in thin-section), pelagic ostracods (entomozoans) and calcified radiolarians (<200 μm). Most foraminifers, conodont elements and fish teeth were also found from residues of washed samples. The lithological description is based on Dunham's (Reference Dunham and Ham1962) carbonate rock classification refined by Embry & Klovan (Reference Embry and Klovan1971). Each facies is related to a depositional environment according to the zonations proposed by Wright & Burchette (Reference Wright, Burchette and Reading1996) and Flügel (Reference Flügel2004). The pelagic carbonate systems of Devonian time are known to be large-extent platforms (Peterhänsel & Pratt, Reference Peterhänsel and Pratt2001; Morrow & Sandberg, Reference Morrow and Sandberg2008). The vertical change of facies during Famennian time suggests a low-angle ramp depositional system. Inner-ramp deposits correspond to marine sediments which were deposited above the base of the fair-weather wave action. Mid-ramp deposits were deposited between the lower limit of fair-weather wave action and the base of storm-wave action. Outer-ramp – basinal deposits were deposited under the lower limit of storm-wave action (Fig. 2b).

Figure 2. Col des Tribes section with microfacies interpretations and proposed relative sea-level changes. Conodont zones after Ziegler & Sandberg (Reference Ziegler1990). Estimated sea-level variations based on N/(N+B) ratio, where N represents nektonic faunas and B benthic faunas. Blackish argillaceous sediments are depicted in grey, shadings represent the tentative position of some Famennian events. lo – lower; up – upper; um – uppermost.
3.b. Faunas
A total of 71 samples were sampled for conodont studies. The weight of the samples analysed varied within the range 50–730 g depending on the abundance of conodonts (Table 1). The samples were taken at intervals of 0.1–1.0 m over the entire section in order to capture the changes in lithology. The samples were dissolved in dilute formic acid (10%). The insolute residues were separated through two sieves (100 μm and 1 mm). The two fractions were dried and picked up under a binocular microscope in order to collect the microfossils. All conodonts (Table 1) occurring in the residues were picked up. Some conodont elements were identified at the specific level for biostratigraphy (Fig. 3). For biodiversity analyses, the absolute and relative abundances of the different genera present in the samples have been investigated. When present, trilobites provided additional information about palaeoenvironment.
Table 1. Numbers of platform conodont genera per level. The samples in bold are not included in the analyses due to the very low abundance of conodonts. Pa – Palmatolepis; Po – Polygnathus; Ps – Pseudopolygnathus; Bi – Bispathodus; Br – Branmehla; Me – Mehlina; An – Ancyrodella; Ic – Icriodus; Sc – Scaphignathus; Pr – Protognathodus; Si – Siphonodella.


Figure 3. Famennian biostratigraphic species. (a) Palmatolepis triangularis Sannemann, Reference Sanneman1955; (b) Palmatolepis minuta minuta Branson & Mehl, Reference Branson and Mehl1934; (c) Palmatolepis termini Sannemann, Reference Sanneman1955; (d) Palmatolepis crepida Sannemann, Reference Sanneman1955; (e) Palmatolepis glabra prima Ziegler & Huddle, Reference Ziegler and Huddle1969; (f) Palmatolepis rhomboidea Sannemann, Reference Sanneman1955; (g) Palmatolepis gracilis gracilis Branson & Mehl, Reference Branson and Mehl1934; (h) Palmatolepis marginifera marginifera Helms, Reference Helms1959; (i) Polygnathus glaber bilobatus Ziegler, Reference Ziegler1962; (j) Scaphignathus velifer velifer Helms, Reference Helms1959; (k) Palmatolepis perlobata helmsi Ziegler, Reference Ziegler1962; (l) Palmatolepis gracilis sigmoidalis Ziegler, Reference Ziegler1962; (m). Palmatolepis perlobata postera Ziegler, Reference Ziegler1960; (n) Polygnathus styriacus Ziegler, Reference Ziegler, Flügel and Ziegler1957; (o) Palmatolepis gracilis expansa Sandberg & Ziegler, Reference Sandberg and Ziegler1979; (p) Bispathodus costatus Ziegler, Sandberg & Austin, Reference Ziegler, Sandberg and Austin1974; (q) Bispathodus ultimus Bischoff & Ziegler, Reference Ziegler, Flügel and Ziegler1957; (r) Siphonodella sulcata Huddle, Reference Huddle1934; (s) Protognathodus meischneri Ziegler, Reference Ziegler1962; (t) Siphonodella quadruplicata Branson & Mehl, Reference Branson and Mehl1934; (u). Pseudopolygnathus triangulus triangulus Voges, Reference Voges1959; (v) Siphonodella duplicata Branson & Mehl, Reference Branson and Mehl1934; (w) Siphonodella cooperi Hass, Reference Hass1959.
3.b.1. Conodont absolute and relative abundances
Absolute abundance was estimated as the number of platform elements (P1) per kilogram of rock. Since the differences in absolute abundances reach several orders of magnitude, these data have been log-transformed. Very different weight of rocks have been analysed in order to obtain a total close to 100 platform elements for each level, allowing for a reliable estimation of relative abundances. When the number of platform elements was <100, values were excluded from the analyses (see Table 1). Among these platform elements, several genera can be identified that have been related to different environmental preferences (Seddon & Sweet, Reference Seddon and Sweet1971; Sandberg, Reference Sandberg and Barnes1976). The variations of the relative proportion of the different genera are assumed to provide an indirect water depth proxy.
Such an approach is called biofacies analysis (Seddon & Sweet, Reference Seddon and Sweet1971; Sandberg, Reference Sandberg and Barnes1976). In this study the procedure established by Sandberg (Reference Sandberg and Barnes1976) has been followed. Each biofacies was named after the one or two most abundant genera among the conodont platform elements, usually constituting at least 75–80% of the total platform elements. During the investigated period, Icriodus and Protognathodus are considered as characteristic of shallow marine environments (Sandberg & Dreesen, Reference Sandberg, Dreesen and Clark1984), whereas Palmatolepis and Siphonodella have been interpreted as associated with deeper conditions (Sandberg, Reference Sandberg and Barnes1976). Bispathodus and morphologically related genera such as Branmehla and Mehlina are grouped under the term bispathodids (Ziegler & Sandberg, Reference Ziegler, Sandberg and Clark1984). Together with Polygnathus they are considered as ubiquitous, but bispathodids are considered as characteristic of the euphotic zone of deep environments (Sandberg, Reference Sandberg, Sandberg and Clark1979; Ziegler & Sandberg, Reference Ziegler, Sandberg and Clark1984). Scaphignathus and Alternognathus are characteristic of very shallow water, principally found in inner platforms, and are poorly represented on outer platforms (Sandberg, Reference Sandberg and Barnes1976). These biofacies models are consistent with those developed by Savoy & Harris (Reference Savoy and Harris1993) for a Famennian ramp deposited in North America.
4. Results and interpretations
4.a. Lithology and macrofauna
The Famennian deposits at Col des Tribes comprise a 84-m-thick carbonate succession (Fig. 2a). Above a 0.05-m-thick dark clayey level at the base, the lowest 17 m of the section are composed of poorly cropping out, yellowish mudstones to wackestones organized into decimetre-thick beds. Scarce fragments of blind trilobites were found (Nephranops, bed CT25). Beds can display a nodular aspect because of important post-depositional pressure-dissolution processes (stylolithes, pseudonodular texture). Between 17 and 31 m, typical griotte limestones (‘vrai griottes’, Boyer et al. Reference Boyer, Krylatov, Fèvre and Stoppel1968) are exposed (Fig. 4a, b). These are composed of massive, red mudstones to wackestones/floatstones organized into decimetre-thick beds. They display numerous centimetre-sized ammonoids concentrated in the uppermost part of the beds and a nodular texture. Carbonate nodules are composed of cephalopods or micrite coated by millimetre-thick ferruginous argilites (Fig. 4b). Ammonoids often display geopetal infillings without preferential orientation. Micritic nodules are millimetre- to centimetre-sized and are arranged parallel to bedding. Laterally they change into fragmented to intact micritic beds. At 20.5 m a bed with intraformational breccias is intercalated, characterized by angular, decimetre- to metre-wide tilted griotte blocks (Figs 2, 3a; Wendt & Aigner, Reference Wendt and Aigner1985; Becker, Reference Becker1993).

Figure 4. Famennian beds at Col des Tribes section. (a) General view of the Griotte limestones. On the left, arrows point to intraformational breccia; on the right, arrow points to intraformational sliding. Between are nodular limestones. (b) Detail of the nodular limestones. Arrows point to micritic nodules and streching of non-totally lithified micritic beds. (c) View of the massive micritic limestones. (d) View of the thin-bedded, pseudonodular limestones. Top – top of the beds.
Above the breccias is an irregular centimetre-thick ferruginous hardground with abundant ammonoids which were eroded in the upper part and contains patches of iron incrustations. The following beds (CT43, 44) yield blind phacopid trilobites (Tripholiops) and, in argillaceous interstices, reduced eyed Calybole proetids among mass occurrences of Guerichia bivalves. Between 31 and 52 m above the base of the section are massive, greyish mudstones (Fig. 4c) organized into decimetre-thick beds with locally planar laminations and sparse ammonoids. Between 52 and 63 m pseudonodular mudstones are exposed (Fig. 4d), composed of centimetre-thick beds which have experienced pressure-dissolution processes with irregular stylolites leading to their nodular aspect. Some ammonoids are present. Above are 7-m-thick pink massive wackestones with rare blind trilobites (Dianops, Chaunoproetus), followed by 12-m-thick pseudonodular wackestones with numerous ammonoids and trilobites (Haasia, Helioproetus). At 82 m above the base of the section is a 0.6-m-thick dark argillaceous interval. The uppermost part of the section, between 83 and 85 m above its base, comprises massive mudstones with discrete, plane laminations. Trilobites (Liobolina) of early Tournaisian age are abundant. Above the limestones, black radiolarian cherts (‘lydites’) of middle Tournaisian age occur.
4.b. Conodont biostratigraphy
In this paper, the Montagne Noire zonation by Klapper (Reference Klapper, McMillan, Embry and Glass1989) is followed for the Frasnian, the Ziegler & Sandberg (Reference Ziegler and Sandberg1990) zonation for the Famennian and the Sandberg et al. (Reference Sandberg, Ziegler, Leuteritz and Brill1978) zonation for the Tournaisian. Some variations on the Famennian scheme proposed by Corradini (Reference Corradini2008) and Kaiser et al. (Reference Kaiser, Becker, Spalletta and Steuber2009) have also been taken into account.
The youngest Frasnian, almost all the Famennian and two Tournaisian zones have been discriminated in the Col des Tribes section. The distribution of the determined species present all along the section is depicted in Figure 5.
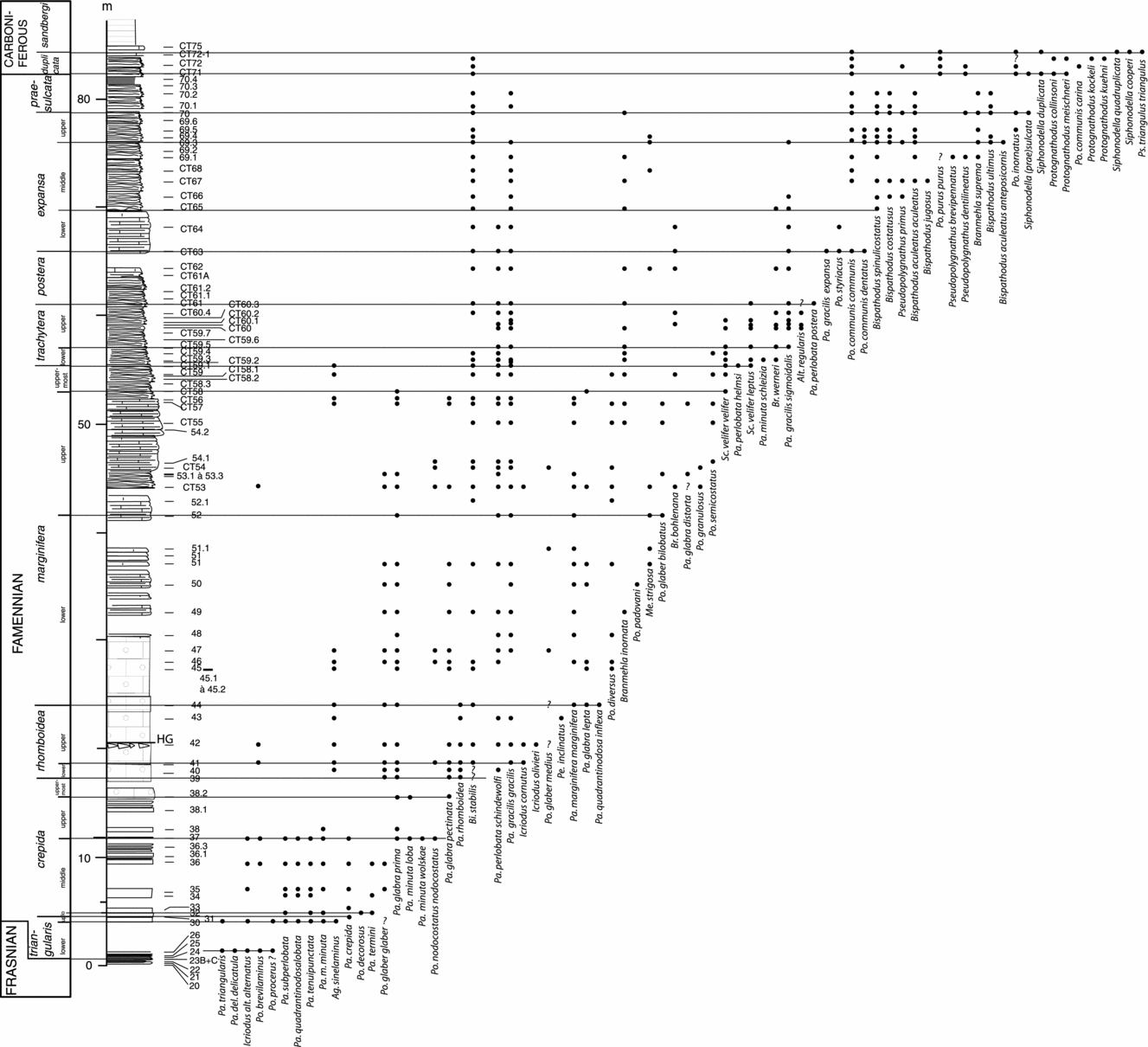
Figure 5. Distribution of major conodont species in the Famennian beds at the Col des Tribes section, Montagne Noire, France. Pa – Palmatolepis; Po – Polygnathus; Ps – Pseudopolygnathus; Bi – Bispathodus; Br – Branmehla; Me – Mehlina; An – Ancyrodella; Ic – Icriodus; Sc – Scaphignathus; Pr – Protognathodus; Si – Siphonodella.
The lowest part of the section (CT20–23) delivered the species Palmatolepis bogartensis, Ancyrodella curvata late form and Ancyrognathus asymmetricus, indicating the Biozone ‘Montagne Noire Zone 13’ of latest Frasnian age (Klapper, Reference Klapper, McMillan, Embry and Glass1989).
Levels CT24, 25 can be dated to the first zone of the Famennian, the Lower triangularis Zone due to the occurrence of Palmatolepis praetriangularis, Pa. triangularis and Pa. delicatula delicatula.
The entry of the marker Pa. minuta minuta in sample CT30 allows the discrimination of the Upper triangularis Zone. The conodont association is dominated here by Palmatolepis triangularis, Pa. subperlobata and Pa. tenuipunctata, indicating the upper triangularis Zone.
The Lower crepida Zone is documented, and corresponds to sample CT31 which contains the first Palmatolepis crepida.
The occurrence of Pa. termini in sample CT32 allows the attribution of that level to the Middle crepida Zone. The Upper crepida Zone begins in level CT37 and the lower boundary is identified by the first occurrence of the marker Pa. glabra prima.
The presence of the Uppermost crepida Zone is attested in the level CT38-2 with the first appearance of the species Pa. glabra pectinata.
After this interval, level CT39 belongs to the Lower rhomboidea Zone due to the occurrence of the index species Pa. rhomboidea. The Upper rhomboidea Zone is identified here from the entry of Pa. gracilis gracilis in level CT41.
The Lower marginifera Zone starts 23 m above the base of the section and is recognized by the presence of the marker Pa. marginifera marginifera in sample CT44. The Upper marginifera Zone is discriminated in level CT52 by the first occurrence of Polygnathus glaber bilobatus. The entry of Scaphignathus velifer within the level CT58 allows the identification of the Uppermost marginifera Zone (equivalent to the velifer Zone of Corradini, Reference Corradini2008).
The base of the Lower trachytera Zone is identified in level CT59-1 due to the occurrence of Pa. perlobata helmsi. The appearance of Pa. gracilis sigmoidalis in sample CT59-5 allows the discrimination of the base of the Upper trachytera Zone.
An undifferentiated postera Zone is discriminated at 51–56 m from the base of the section. The base is recognized by the occurrence of the index, Pa. perlobata postera, in sample CT61.
The occurrence of Pa. gracilis expansa in sample CT63 marks the base of the Lower expansa Zone, whereas the entry of Bispathodus spinulicostatus in sample CT65 allows this level to be referred to the Middle expansa Zone. The Upper expansa Zone is discriminated by the presence of the marker Bispathodus ultimus in level CT66.
The base of the Lower praesulcata Zone is identified by the presence of the first representatives of Siphonodella in sample CT70, where both straight and curved forms occur. Following the suggestion of Corradini (Reference Corradini2008), the Middle praesulcata Zone is not considered here and has been included in the Lower praesulcata Zone due to the difficulty of discriminating this zone and its poor definition. The Lower praesulcata Zone extends up to level CT70-4, corresponding to the Hangenberg shales equivalent.
The first level above the Hangenberg shale equivalent belongs to the Lower duplicata Zone, due to the occurrence of the marker S. duplicata morphotype 1; the Upper praesulcata and the sulcata Zone are therefore not present. The Lower duplicata Zone extends up to level CT71. Above, the Upper duplicata Zone has not been discriminated and the top of the section (CT72-1) belongs to the upper part of the sandbergi Zone (equiavalent to the quadruplicata Zone of Kaiser et al. Reference Kaiser, Becker, Spalletta and Steuber2009) because of the occurrence of S. quadruplicata and Ps. triangulus triangulus.
4.c. Tentative location of the events
Except for the two major Upper Devonian events – the Kellwasser at the Frasnian–Famennian boundary and the Hangenberg event just before the Devonian–Carboniferous boundary – the other Famennian events are not represented by blackish argillaceous deposits or other particular deposits. Based on the detailed biostratigraphy, we propose a position of the events as follows from bottom to top (Fig. 2): the Condroz event occurs in the upper rhomboidea Zone (levels CT41–43); and the annulata event occurs in the upper trachytera Zone (Walliser, Reference Walliser1996) and can be located in successive CT59-5 to CT60-3 samples (Fig. 2).
4.d. Facies description and interpretation
At Col des Tribes a limitation to microfacies analysis is sometimes recrystallization of the fine-grained carbonates, probably due to late diagenesis during nappe setting up. In some cases, small-sized fragments cannot be easily identified and the original micritic cement is changed into microsparite. The most deformed samples show microshear zones and stylolites underlined by ferruginous surfaces, and the adjacent rock suffered intensive recrystallization into mosaic calcite. Nevertheless, it is possible to distinguish from macroscopic observations and microscopic investigations four main facies, namely F1–F4 (Fig. 2a). These facies are organized into a ramp depositional system (Fig. 2b).
4.d.1. Facies F1: pelagic mudstones
The limestones of F1 (Fig. 6) are greyish and display some parallel-bedding laminations. They are mudstones with widely dominant pelagic fauna composed of calcitized radiolarians (Fig. 6a), entomozoan ostracodes and filaments (bivalves). The benthic fauna is poor (very rare to <3%), mostly represented by ostracods, some echinoid fragments and rare benthic foraminifers and trilobites sclerites. Noticeable is the scarcity of cephalopods. Geopetal infillings of early cavities (some hundreds of micrometres long, microstromatactis or dessication figures) are frequent. The micritic matrix sometimes displays a peloidal texture (Fig. 6b), suggesting local microbial activity in some levels (Kazmierczak, Kremer & Racki, Reference Kazmierczak, Kremer and Racki2012). Burrowing is scarce and only plane lamination structures were observed. Plane lamination is defined by discrete changes in concentration of pelagic molluscs, entomozoans and radiolarians. No current structures have been evidenced in facies F1. It has been identified in the lower part of the section (samples CT30–32, 39, 40 and 46). It is widely developed in the central part of the section from samples CT48 to 60-4. The N/(N+B) ratio ranges from 0.7 to 1.0. Note that the most elevated values are grouped from samples CT 58-1 to 60-4, correlative with very scarce benthic foraminifers, trilobites and brachiopods (Fig. 2a). These features indicate that facies F1 was deposited in low-energy conditions under the storm-wave base, in an outer-ramp – basin setting (Fig. 2b). The scarce burrowings suggest some oxygenated bottoms (Droser & Bottjer, Reference Droser and Bottjer1986), at least during levels CT48, CT59-3 and CT61-1.

Figure 6. Characteristic microfacies types from thin-sections, Col des Tribes (France). A – ammonoid; B – brachiopod; E – entomozoan; F – filament (pelagic bivalve); T – trilobite; R – radiolarian. (a) Microfacies F1: pelagic mudstone with entomozoans, filaments and radiolarians (CT59). (b) Microfacies F2: pelagic mudstones to wackestones (CT32). (c) Microfacies F3: cephalopod mudstone to wackestone. Note geopetal infilling (arrow) oblique on bedding, indicating post-depositional rotation (CT41). (d) Microfacies F4: diversified fossiliferous wackestone (CT66). (e) Calcitized radiolarians (CT61-1). (f) Fenestral fabric: amalgamated peloids (dark) leave space for spar-filled cavities (light) (CT69-2).
4.d.2. F2: Pelagic mudstone to wackestone
Facies F2 (Fig. 6) is poorly represented in the section (samples CT34, 35, 59-7). It is composed of decimetre-thick greyish to yellowish limestones. Pelagic organisms are dominated by radiolarians, entomozoan ostracodes, pelagic molluscs (‘filaments’) and cephalopods. Benthic organisms (3–5%) are often preserved as small-sized well-sorted fragments of echinoids, ostracods and trilobites. They are more abundant and more diversified than compared to facies F1. Geotrope infillings of early cavities or fossils are present. No current structure has been evidenced. The N/(N+B) ratio falls within the range 0.6–0.8.
Facies F2 is interpreted as deposited under the storm-wave base in an outer-ramp setting (Fig. 2b). A part of the bioclasts probably originated in the innermost parts of the ramp.
4.d.3. F3: Cephalopod mudstone to wackestone (typical griotte limestones)
Facies F3 is composed of reddish, massive nodular or wavey laminated mudstones to wackestones (Fig. 4a, b). Pelagic organisms are dominant with abundant centimetre-sized goniatites (Fig. 6) and entomozoan ostracodes, pelagic bivalves and some radiolarians. Benthic organisms are present (1–5%) and diversified with trilobites, brachiopods, echinoids, benthic ostracods and foraminifers. The N/(N+B) ratio has values 0.5–0.8. The micritic matrix suffered early diagenetic processes (D. Michel, unpubl. thesis, University of Paris, Paris Sud, 1981) leading to cracks and micritic nodules embedded by iron-clay coatings or sedimentary deposits. Micritic geopetal infillings are frequent in ammonoid shells.
The goniatites are well sorted, suggesting current activity prior to their deposition. Iron- and manganese-oxide-encrusted hardgrounds and numerous crypto-hardgrounds indicate condensation episodes during times of low sedimentation rates and bacterial activity (Préat et al. Reference Préat, Mamet, Bernard and Gillanc1999). Intraformational breccias with tilted consolidated slabs of rock (centimetres to a metre wide), and micritic nodules with cephalopods exhibiting variously inclined geopetal structures pointing to different degrees of rotation, indicate unstable conditions with repeated slidings of not completely lithified sediments. These features suggest the griotte limestones were deposited on a slope under current activity, probably under the fair-weather wave action (middle–outer ramp, as also suggested by Préat et al. Reference Préat, Mamet, Bernard and Gillanc1999 at Coumiac; Fig. 2b).
The deposition of ‘griottes limestones’ often occurred through Devonian time. It has been a matter of much debate, but most authors suggest that these limestones were deposited in a low-energy environment on platforms or ramps, but under tectonically instable conditions (e.g. Bourrouilh, Reference Bourrouilh, Farinacci and Elmi1981; Coudray & Michel, Reference Coudray and Michel1981; D. Michel, unpubl. thesis, University of Paris, Paris Sud, 1981; Franke & Walliser, Reference Franke, Walliser, Martin and Eder1983; Dreesen, Reference Dreesen1989) as for the Rosso Ammonitico deposits of the Tethys Ocean during Mesozoic time.
4.d.4. F4: Diversified fossiliferous wackestones
Facies F4 (Fig. 6) is composed of greyish massive to pseudonodular, centimetre- to decimetre-thick beds. Pelagic organisms comprise undetermined rare radiolarians, entomozoan crustaceans, pelagic bivalves and abundant goniatites. The benthic fauna is well represented (5–15%) and diversified with trilobites and benthic ostracods, sparse echinoids, brachiopods, gastropods, bivalves and foraminifers. The proportions of pelagic organisms and benthic organisms are rather similar with a slight domination of pelagic organisms, the N/(N+B) ratio falling within the range 0.4–0.6. Geopetal infillings of cavities and burrows are present. Only local plane laminations have been found, but the intensive post-depositional pressure/dissolution responsible for the pseudonodular texture probably hides most of the sedimentary structures.
The presence of diversified benthic fauna and of pelagic fauna, associated with poorly sorted goniatites, indicates a depositional environment shallower than the previous environments. Some organisms are well preserved, such as trilobites, while others are broken. Noticeable is the absence of current structures, and between bioclasts the matrix is micritic. All these features indicate a middle–uppermost outer-ramp depositional system (Fig. 2b).
4.e. Conodont genera and abundances
4.e.1. Abundance
After complete processing of the sampled limestones, almost 7200 platform conodont elements were obtained for abundance evaluation (Table 1). The abundances are very variable through the section with an estimated mean of more than 600 platform elements per kilogram (Fig. 7). Some levels are very poor however and have been excluded from the analyses (Table 1). Autochtony of conodont assemblages is indicated by the rather high percentage of juveniles as well as ramiform elements among the conodont assemblages; additionally, conodonts are well preserved. These support the exclusion of sorting processes such as current activity or downslope gravity flow, as recorded in other slope settings (e.g. Bàbek & Kalvoda, Reference Bàbek and Kalvoda2001; Vierek & Racki, Reference Vierek and Racki2011). Among the genera occurring during the Famennian, Palmatolepis is always present and is the major representative of the conodont fauna in the first 50 m (Fig. 7). After the annulata event, the Bispathodus specimens replace Palmatolepis. The abundances of Icriodus and Protognathodus are noticable in a few levels: at the base of the section for the former (samples CT30–42) and at the top for the latter (H71–72).

Figure 7. Relative and absolute abundances of conodonts at the Col des Tribes (France) during Famennian time. (a) Percentage of conodont genera. (b) Abundance of platform genera conodonts per kilogram (in log). Black line: total number of platform conodont specimens; red line: total number of Palmatolepis specimens; and yellow dotted line: Bispathodus specimens. Grey: identified Famennian events; shading: tentative position of some Famennian events. Pa-Ic – Palmatolepis–Icriodus biofacies; Pa – Palmatolepis biofacies; Pa-Bi – Palmatolepis–Bispathodus biofacies; Po-Bi – Polygnathus–Bispathodus biofacies; Pr-Po – Protognathodus–Polygnathus biofacies; Si-Po – Siphonodella–Polygnathus biofacies.
Although always present, Polygnathus is never dominant except at the top of the section just after the Hangenberg event (Fig. 7).
The last sampled level dated as Carboniferous (CT72-1) is dominated by the genus Siphonodella (Table 1). When present, other genera (including Pseudopolygnathus, Scaphignathus and Alternognathus) are always rare.
4.e.2. Conodont biofacies (Fig. 7)
Six biofacies can be distinguished, from bottom to top of the sampled section: (1) palmatolepid-icriodid; (2) palmatolepid; (3) palmatolepid-bispathodid; (4) polygnathid-bispathodid; (5) polygnathid-protognathodid; and (6) polygnathid-siphonodellid.
4.e.2.a. Biofacies 1
At the base of the section (Lower triangularis through rhomboidea zones), the faunas are dominated by palmatolepids but the percentage of icriodids can reach 25%. The co-occurrence of the deep-water Palmatolepis with a significant contribution of Icriodus, considered a shallow-water indicator, coincides with the pseudo-nodular limestone deposits, facies F2. This high proportion of Icriodus can reflect the telescoping of normally shallower-water faunas related to eustatic sea-level fall and increased downslope sediment transport, often associated with the aftermath of the F/F crisis (Sandberg et al. Reference Sandberg, Ziegler, Dreesen and Butler1988). The largest Icriodus peak (level CT38) is often regarded as coinciding with the Nehden event, which is interpreted to be triggered by a short sea-level fall (Schülke, Reference Schülke2003). This increase has also been interpreted to be due to oceanic factors such as the overturn of oxygen-poor water masses that may have selectively reduced ‘offshore pelagic’ conodont taxa (Sandberg et al. Reference Sandberg, Ziegler, Dreesen and Butler1988; Schindler, Reference Schindler1990; Buggisch, Reference Buggisch1991). This could also be the result of biological factors such as the opportunistic occupation of niche space vacated by other conodont groups, as suggested by Corradini (Reference Corradini and Bagnoli1998, Reference Corradini2003), in typical pelagic sections where the large amount of new Famennian species of icriodids led to the introduction of a palmatolepid-icriodid biofacies regarded as pelagic.
4.e.2.b. Biofacies 2
This biofacies starts with the Condroz event. The percentages of the conodont genera Polygnathus, Icriodus and Bispathodus are rather low (<15%) in the sampling interval CT42–50 (Fig. 2), allowing a palmatolepid biofacies to be defined in that interval (Lower marginifera Zone). This genus is known to be confined to deep-water zones, and is clearly dominant in facies deposited below the storm-wave action (Facies F1). The proportion of bispathodids slightly increases afterwards but remains low (<10%) from level CT51 to CT58 (uppermost marginifera).
4.e.2.c. Biofacies 3
Bispathodids and polygnathids increase simultaneously (trachytera Zone) with the annulata event deposits. The contribution of Palmatolepis drops from 80% (triangularis Zone to uppermost marginifera Zone) to 30–40% (postera and expansa zones) concomitantly to the deposition of the shallowest facies (Facies 4 dominant). In this bispathodid-palmatolepid biofacies there is still a high percentage of Bispathodus which presumably lived in the euphotic zone of pelagic seas (Sandberg, Reference Sandberg and Barnes1976; Ziegler & Sandberg, Reference Ziegler, Sandberg and Clark1984). Palmatolepis is considered as a genus living in deep water (Sandberg, Reference Sandberg and Barnes1976). A co-occurrence of these two genera has been observed in association with the annulata black shale deposits in Poland (Kowala section), and was interpreted as a position of the section close to pelagic ridge (Racka et al. Reference Racka, Marynowski, Filipiak, Sobstel, Pisarzowska and Bond2010). The change from a palmatolepid biofacies to a palmatolepid-bispathodid biofacies was already observed for two sections in Sardinia (Corradini, Reference Corradini2003) after the trachytera zone, and may emphasize sea-level fall.
The short occurrence of Scaphignathus (CT59-3–60-4) during this palmatolepid-bispathodid biofacies seems to contradict a shallow-water preference for this genus (Sandberg, Reference Sandberg and Barnes1976), but may indicate that the genus was transported during fluctuating water depth changes allowing the presence of organisms into the waters above submarine rises (Sandberg & Ziegler, Reference Sandberg and Ziegler1979). Another explanation could be better water oxygenation, permitting the introduction and mixing of this shallow-water genus into the pelagic palmatolepid-bispathodid biofacies.
4.e.2.d. Biofacies 4
Bispathodids become increasingly abundant during the expansa Zone and lower praesulcata Zone, reaching 60–80% of the total number of specimens. During the 2 m preceeding the Hangenberg event, biofacies 4 is characterized by the relative increase of Polygnathus at the expense of Palmatolepis, suggesting a slight shallowing. This unusual biofacies has been related to a shallow environment in an extensive flat sea bottom in Spain by García-Alcalde & Menéndez-Alvarez (Reference García-Alcalde, Menéndez-Alvarez, Flajs, Feist and Ziegler1988).
4.e.2.e. Biofacies 5
The onset of biofacies 5, composed of Polygnathus-Protognathodus at the top of the section below the Devonian–Carboniferous boundary and above the Hangenberg event, confirms a shallowing-upwards trend which is observed at numerous geographic locations (Ziegler, Ji & Wang, Reference Ziegler, Ji, Wang, Flajs, Feist and Ziegler1988; Girard, Reference Girard, Königshof and Werner1994).
4.e.2.f. Biofacies 6
At the end of the sampled interval, biofacies 6 is composed of a high proportion of siphonodellids which are assumed to be pelagic–hemipelagic genus (Lane, Sandberg & Ziegler, Reference Lane, Sandberg and Ziegler1980). They increase in abundance towards deeper water, thus indicating a deepening-upwards trend as proposed in other places (Girard, Reference Girard, Königshof and Werner1994; Sanz Lopez, García López & Montesinos, Reference Sanz Lopez, García López and Montesinos1999).
4.e.3. Correlation between microfacies and conodont data
Synchronous and similar trends emerge from the comparison of sea-level proxies (Fig. 8). Here only the percentage of Palmatolepis is considered as the more relevant index to estimate sea-level changes, as this genus is assumed to live in deeper environments than the other genera. It is compared to the N/(N+B) ratio through Famennian time. The results of both these sea-level estimators are concordant.

Figure 8. Comparison between different Late Devonian sea-level indicators. (a) From left to right: sea-level curve proposed in this paper based on the N/(N+B) ratio and percentage of the Palmatolepis genus through the Col des Tribes (MN, France). Long-term sea-level curve at Col des Tribes by combining microfacies and conodont informations. (b) Sea-level curve according to Johnson, Klapper & Sandberg (Reference Johnson, Klapper and Sandberg1985) and Sandberg et al. (Reference Sandberg, Morrow and Ziegler2002) with the five transgressive starts recognized through the Famennian. Conodont zones are figured according to the depth of the Col des Tribes. Grey: identified Famennian events; shading: tentative position of some Famennian events.
Linear regression analysis of microfacies estimated by the N/(N+B) ratios and biofacies estimated with the percentage of Palmatolepis reveals a significant correlation (N = 43; R = 0.50; P = 0.000). The correlation is confirmed (P<0.002) using the non-parametric Kendall test, used to measure the congruent relation between two measured proxies. Eight remarkable periods of sea-level rise are evident, highlighted by an increase in Palmatolepis percentage (black arrows in Fig. 8) and an increase in the N/(N+B) ratio (red arrows in Fig. 8; see online colour version).
5. Discussion
The Col des Tribes section contains relatively abundant and diversified conodont assemblages. This section is one of the most fossiliferous Famennian sections for conodonts worldwide. Consequently, different proxies indicative of environmental changes (sea level) and hypotheses influencing the onset of anoxic sediments can be discussed.
5.a. Relative sea-level changes
The agreement between the proxies used in this study allows to the proposal of a complete curve for sea-level changes through Famennian time for the Col des Tribes section (Fig. 8a).
The long-term trend indicates a progressive sea-level rise from the Upper triangularis Zone to the uppermost marginifera Zone, followed by a gradual sea-level fall from the Lower trachytera Zone to the uppermost praesulcata Zone.
These results are in accordance with the results of Johnson, Klapper & Sandberg (Reference Johnson, Klapper and Sandberg1985), Haq & Schutter (Reference Haq and Schutter2008) and Sandberg, Morrow & Ziegler (Reference Sandberg, Morrow and Ziegler2002) for the trachytera-praesulcata interval (Fig. 8b). This regressive trend has also been observed in other areas from north Gondwana, such as in Algeria (Wendt et al. Reference Wendt, Kaufmann, Belka, Klug and Lubeseder2006). This long-term trend is punctuated by cycles of minor changes in sea level. Johnson, Klapper & Sandberg (Reference Johnson, Klapper and Sandberg1985) and Sandberg, Morrow & Ziegler (Reference Sandberg, Morrow and Ziegler2002) reported six transgressive cycles. The detailed study of the Col des Tribes section allows the proposal of a curve for the Galatian superterrane. From the Upper triangularis Zone to the Lower marginifera Zone three cycles are identified based on simultaneous and similar trends in both proxies. Two transgressive events are in accordance with the previous results (Lower–Middle crepida and Lower marginifera zones), and a new event has been identified (Lower rhomboidea zones). In the Col des Tribes section as in Euramerica, minor cycles have not been found in the Upper marginifera Zone. Later, the three transgressive events of Johnson, Klapper & Sandberg (Reference Johnson, Klapper and Sandberg1985) and Sandberg, Morrow & Ziegler (Reference Sandberg, Morrow and Ziegler2002) are identified (Lower trachytera and Lower and Middle expansa zones), and a third event is now depicted for the north Gondwana-related area in the Upper trachytera Zone.
As a whole, the main discrepancies between our curve and the previous curves are: (1) the absence of a transgressive trend at the Frasnian–Famennian boundary as well as a regressive trend between the Upper crepida and the Lower marginifera zones; and (2) the presence of a transgressive trend during the marginifera Zone. The differences between our curve and that of Johnson, Klapper & Sandberg (Reference Johnson, Klapper and Sandberg1985) or Wendt et al. (Reference Wendt, Kaufmann, Belka, Klug and Lubeseder2006) could be a result of the following factors: (1) our outcrop data provide lower resolution because some parts of the section are not exposed, especially during the lower Famennian (triangularis and crepida zones), or because parts of the section suffered recrystallization and the results of microfacies analysis are not accurate enough (marginifera Zone); (2) transgressive trends proposed by Johnson, Klapper & Sandberg (Reference Johnson, Klapper and Sandberg1985) are based on data from facies shifts in basinal deposits whereas our samples were collected on a ramp, an environment which is more sensitive to sea-level changes; or (3) local or regional tectonic events, even if moderate, can hide low-amplitude sea-level changes. Such tectonic events can be expected in a flexural basin on continental crust which characterizes the Devonian of the Montagne Noire (Raymond, Reference Raymond1987); this concerns the Famennian deposits at the transition between the Devonian ramp and the Early Carboniferous flysch basin in particular (Engel, Feist & Franke, Reference Engel, Feist and Franke1982).
5.b. Anoxic events
The Col des Tribes section is characterized by a continuous succession of limestones devoid of anoxic (organic-matter-rich) deposits, except for two levels with weathered blackish deposits. The lower level is located at the top of the MN13 zone and corresponds to deposits of the Upper Kellwasser event. The upper deposit is located in the uppermost part of the Lower praesulcata Zone and corresponds to deposits of the Hangenberg event. Two other widely known events of the Famennian, Condroz and annulata, cannot be identified by facies changes. Contrary to some other Devonian events, which are difficult to place due to the imprecision of the current stratigraphy when they are not lithologically defined (i.e. the Lower Kellwasser event; Girard & Renaud, Reference Girard and Renaud2007), the Condroz and the annulata events can be easily positioned. The new biostratigraphic frame allows the positioning of the Condroz in the Upper rhomboidea and annulata in the Upper trachytera zones (Fig. 2a). Moreover, the Condroz and annulata events are related to major environmental changes at the global scale (Walliser, Reference Walliser1996; Becker, Ashouri & Zyazdi, Reference Becker and Weyer2004; Racka et al. Reference Racka, Marynowski, Filipiak, Sobstel, Pisarzowska and Bond2010). Note that no bioturbation was recorded in any of the intervals where anoxic events are assumed to occur (Fig. 2). At Col des Tribes, important changes in the conodont assemblages are recorded at levels assumed to have been deposited during these events. That indicates that their location in the carbonate succession is robust.
The Upper Kellwasser event is lithologically represented by dark grey limestones (sample CT23, Fig. 2a). It occurs just before the first occurrence of Famennian conodont and trilobite faunas (Nephranops). This event is associated with a major crisis, recognized at the global scale (McGhee, Reference McGhee1996; Walliser, Reference Walliser1996). The crisis is marked, among other features, by a drastic turnover of conodonts, the disappearance of the genus Ancyrodella and a total replacement of Palmatolepis species. Accompagnying the reduction in conodont diversity, changes in the generic composition of conodont faunas were observed at a global scale (Sandberg et al. Reference Sandberg, Ziegler, Dreesen and Butler1988; Ji & Ziegler, Reference Ji and Ziegler1993; Girard & Feist, Reference Girard and Feist1996; Lazreq, Reference Lazreq1999; Morrow, Reference Morrow2000). The biotic changes were surimposed to numerous abrupt environmental changes (Gerecke & Schindler, Reference Gerecke and Schindler2012) that are evidenced in the geological record by sea-level fluctuations, intervals of anoxic deposits (Bond & Wignall, Reference Bond and Wignall2008) and major worldwide excursions in the stable carbon and oxygen isotopes (Buggisch, Reference Buggisch1991; Joachimski & Buggisch, Reference Joachimski and Buggisch2002; Joachimski et al. Reference Joachimski, Breisig, Buggisch, Talent, Mawson, Gereke, Morrow, Day and Weddige2009).
The Condroz event is represented by the Upper rhomboidea Zone (samples CT42–43), and is considered to have been triggered by a regressive phase (Walliser, Reference Walliser1996; Schülke & Popp, Reference Schülke and Popp2005). The sea-level curve of Johnson, Klapper & Sandberg (Reference Johnson, Klapper and Sandberg1985) shows a sharp sea-level fall during this time interval. At Col des Tribes, the Condroz event began during the deposition of the lower half of the griotte limestones during a short-term regressive event. A short-term transgressive event (Fig. 8a) began after this. This part of the griotte limestones is characterized by abundant ferruginous clays and at least one hardground, compatible with condensation processes on a slope during sea-level rise. This situation has been described elsewhere in the Montagne Noire (D. Michel, unpubl. thesis, University of Paris, Paris Sud, 1981; Feist, Reference Feist2002) or in Germany (Schülke & Popp, Reference Schülke and Popp2005).
The annulata event is not associated with extinction of faunas but rather with originations (House, Reference House1985; Becker, Reference Becker1993; Hartenfels, Reference Hartenfels2010). The annulata event is generally lithologically marked by dark to black sediments. The woldwide occurrence of black shale sediments supports the hypothesis that this event was caused by short-term flooding on the continent by oxygen-depleted waters due to a rise of the anoxic layers (Wilde & Berry, Reference Wilde and Berry1984). These deposits are often coincident with a significant contribution of assumed shallow-water conodonts such as Scaphignathus, observed in numerous parts of the world. As a consequence, the definition of the uppermost marginifera zone is based on the first occurrence of one species of this genus (Scaphignathus velifer) in pelagic sequences (Ziegler, Reference Ziegler1962, Reference Ziegler1971). To explain the presence of Scaphignathus in pelagic sequences, Sandberg & Ziegler (Reference Sandberg, Sandberg and Clark1979) suggested that this genus was transported from shallow-depth submarine rises into the deep-sea environment.
At Col des Tribes, despite the absence of significant lithological changes, the annulata event can be biostratigraphically located at the top of the Upper trachytera Zone (Walliser, Reference Walliser1996; Racka et al. Reference Racka, Marynowski, Filipiak, Sobstel, Pisarzowska and Bond2010). Facies analyses indicate that annulata occurred during the deposition of the deepest sediments of the section during a short-term transgressive context. The same results have been found by Racka et al. (Reference Racka, Marynowski, Filipiak, Sobstel, Pisarzowska and Bond2010) in Poland where they observed an episodic sea-level rise interrupting an overall regressive trend, and in Germany where Schülke & Popp (Reference Schülke and Popp2005) assumed that the anoxic levels represented transgressive conditions. This is in concordance with the sea-level curves established by Johnson, Klapper & Sandberg (Reference Johnson, Klapper and Sandberg1985) at a global scale or by Sandberg, Morrow & Ziegler (Reference Sandberg, Morrow and Ziegler2002) for the North American area (Fig. 8b). If organic-matter-rich sediments had not been found at Col des Tribes, the period of the annulata event would be characterized by rare to absent benthic fauna limited to some benthic ostracods and pieces of echinoids. The occurrence of peloids and microdessication vugs suggest local microbial activity, as proposed in Poland in the Hangenberg shale (Kazmierczak, Kremer & Racki, Reference Kazmierczak, Kremer and Racki2012). Some pelagic organisms are present, except for ammonoids. The scarcity and poorly diversified fauna suggest that some dysoxic conditions could exist, especially for bottom sea waters, but carbonates could still be deposited in an outer-ramp setting.
This event coincides with a radiation of pelagic faunas, mainly goniatites (House, Reference House1985; Becker, Reference Becker1993). At Col des Tribes, abundant cephalopods suddenly appear a few metres above the end of the annulata (Fig. 2a). This shows that for this event the influence of global environment on the organisms exceeds the influence of local conditions, which determine whether anoxic sediments are deposited. Similar results have already been observed for the Lower Kellwasser event at the end of the Frasnian (Girard & Renaud, Reference Girard and Renaud2007).
The Dasberg event coincides with an eustatic rise marked by transgressive pulses at the base of the Lower expansa Zone (Hartenfels & Becker, Reference Hartenfels and Becker2009; Hartenfels, Reference Hartenfels2011). It is known as a worldwide hypoxic event (Hartenfels & Becker, Reference Hartenfels and Becker2009), sometimes marked by two discrete anoxic events interpreted to be the result of the major transgressions (Marynowski, Filipiak & Zaton, Reference Marynowski, Filipiak and Zaton2010). At Col des Tribes no anoxic sediments occur around the Lower expansa Zone. A slight deepening is observed which could correspond to this crisis, but the absence of any changes in the conodont faunas or microfacies composition does not allow the confirmation of this hypothesis.
By constrast, the Hangenberg event is typified by a lithological change (shale deposit) and by a ‘turnover’ in the conodont fauna (disappearance of Palmatolepis and development of a Protognathodus biofacies). Similar changes of the environment (Hangenberg shale deposit) as well as of the conodont assemblages (development of a protognathodid biofacies) are remarkable at the global scale (e.g. Girard, Reference Girard, Königshof and Werner1994). At Col des Tribes the Hangenberg occurred during a regressive trend on a global scale as proposed by Johnson, Klapper & Sandberg (Reference Johnson, Klapper and Sandberg1985). Opposing results have also been proposed, however. On Baltica, Marynowski et al. (Reference Marynowski, Zatoń, Rakocinski, Filipiak, Kurkiewicz and Pearce2012) found Hangenberg shale deposit beginning in transgression. The same trends were also observed in another section from the Montagne Noire (France), where Girard (Reference Girard, Königshof and Werner1994) and Kaiser, Steuber & Becker (Reference Kaiser, Steuber and Becker2008) suggested a transgressive trend associated with the beginning of the Hangenberg shales.
Global-scale anoxic events are frequently recorded through Phanerozoic time (e.g. Wilson & Norris, Reference Wilson and Norris2001; Caswell & Coe, Reference Caswell and Coe2012; McLaughlin, Emsbo & Brett, Reference McLaughlin, Emsbo and Brett2012), with concomitant extinction and faunal turnover. Anoxic deposits require particular conditions for their formation such as high organic productivity (Murphy et al. Reference Murphy, Sageman, Hollander, Lyons and Brett2000; Riquier et al. Reference Riquier, Tribovillard, Averbuch, Devleeschouwer and Riboulleau2006), poor seawater circulation or installation of oxygen-impoverished bottom water (Murphy et al. Reference Murphy, Sageman, Hollander, Lyons and Brett2000; Buggisch & Joachimski, Reference Buggisch and Joachimski2006). They can be deposited during a fall or rise in sea level, in relation to ocean temperature change (e.g. Joachimski & Buggisch, Reference Joachimski and Buggisch2002; Dopieralska, Reference Dopieralska2003; Arthur & Sageman, Reference Arthur, Sageman and Harris2005). The globally known anoxic events are not lithologically represented by black deposits at Col des Tribes, except for the Upper Kellwasser and the Hangenberg. Depositional environments are located in an open-sea, low-energy, mid- to outer-carbonate muddy ramp setting marked by deepening trends and transgressives pulses.
In this part of the Montagne Noire, despite the deepening trend associated with the period where anoxic deposits could be deposited, the depositional environment was perhaps not deep enough to induce the deposition of typical black shales; micritic limestones could still be deposited, as recorded elsewhere in the world (Playford, Reference Playford1980; Schülke & Popp, Reference Schülke and Popp2005; Königshof et al. Reference Königshof, Savage, Lutat, Sardsud, Dopieralska, Belka and Racki2012). Such a scenario has already been proposed for a Moroccan section just before Turonian (Cretaceous) time, when anoxic conditions characteristic of the OAE2 (oceanic anoxic event 2) did not reach the local shallow-water environments (Gertsch et al. Reference Gertsch, Adatte, Keller, Tantawy, Berner, Mort and Fleitmann2010). The shallow-water fore-reef and shoal limestone successions in the Holy Cross Mountains are also denoted by an obscured Kellwasser record (Casier et al. Reference Casier, Devleeschouwer, Lethiers, Préat and Racki2000; Bond, Wignall & Racki, Reference Bond, Wignall and Racki2004).
6. Conclusions
The Col de Tribes section displays an almost uninterrupted fine-grained carbonate succession through the whole Famennian in the Montagne Noire. The study of conodonts allows the proposal of a detailed biostratigraphical frame, with all of the conodont zones represented along the 84-m-thick section. Facies analysis indicates a depositional setting on a middle–outer, carbonate and muddy ramp. The combination of faunal and facies analyses provides insight into the variations of sea level on the northern Gondwana margin during the Famennian. The sea-level rose slightly from the uppermost triangularis Zone to the Upper trachytera Zone, then dropped from the trachytera Zone to the uppermost praesulcata Zone. This long-term trend was punctuated by at least seven short-term cycles. The main discrepancies with Laurussia or Gondwana curves may be related to the initiation of a south-facing flexural basin in the Montagne Noire.
At Col des Tribes the Upper Kellwasser and Hangenberg events are recorded by blackish organic-matter-enriched deposits associated with changes in conodont assemblages, which occurred during sea-level falls. In contrast, major intra-Famennian events such as the Condroz and annulata events are not materialized by specific lithological changes such as the display of black shales for the latter, though their time-equivalents can be positioned in the section. The Condroz event is represented by iron-argillaceous deposits and hardgrounds which accumulated during a sea-level rise. The annulata is typified by deposits mostly barren of fauna and by a strong decrease in Palmatolepis content which were laid down during a major sea-level rise.
Acknowledgements
This work was supported by a BQR (Bonus Qualité Recherche) of the University of Lyon. This paper is a contribution to IGCP Project n. 596 ‘Mid Palaeozoic climate and biodiversity’ and a contribution to ISEM (UMR5554) no. 2013-161.