INTRODUCTION
In highly endemic countries, the largest part of morbidity control regarding soil-transmitted helminthiases (STHs) relies on chemotherapy using anthelmintics in mass drug administrations (MDAs), since access to health services and education, epidemiological monitoring and effective sanitation are often unavailable (Prichard et al. Reference Prichard, Basanez, Boatin, McCarthy, Garcia, Yang, Sripa and Lustigman2012; Ziegelbauer et al. Reference Ziegelbauer, Speich, Mausezahl, Bos, Keiser and Utzinger2012). Promising results in vaccination trials against Necator americanus suggest that less chemotherapy-dependent control strategies might be feasible, at least for hookworms (Hotez et al. Reference Hotez, Bethony, Diemert, Pearson and Loukas2010; Hotez, Reference Hotez2011). However, since no vaccine has successfully passed any phase 1 clinical trial, the use of anthelmintics remains the gold standard to control STHs in the foreseeable future.
Increased MDAs to highly parasite-exposed populations resulted in considerable declines as far as prevalence, intensity of infection and morbidity are concerned. However, long-term sustainability may be jeopardized, since only albendazole, levamisole, mebendazole and pyrantelpamoate are listed by the WHO as essential medicines to treat human STHs (World Health Organization, 2011) and strong selection pressures caused by the usage of four drugs belonging to only two drug classes may affect genetic diversity and favour spread of drug-resistant parasites (Prichard et al. Reference Prichard, Basanez, Boatin, McCarthy, Garcia, Yang, Sripa and Lustigman2012). Thus, the urgent need for the development of safe and efficacious new anthelmintics against STHs with new modes of action is apparent. Additionally, the conclusive identification of potent combinations of drugs to counteract selection pressure might ensure an extended long-term sustainability of already approved and putative new drugs (Geary et al. Reference Geary, Hosking, Skuce, von Samson-Himmelstjerna, Maeder, Holdsworth, Pomroy and Vercruysse2012).
Cyclooctadepsipeptides seem to be an interesting class for the development of drugs to treat human STHs, since they show a high degree of nematicidal efficacy in a broad range of hosts (Olliaro et al. Reference Olliaro, Seiler, Kuesel, Horton, Clark, Don and Keiser2011; Krücken et al. Reference Krücken, Harder, Jeschke, Holden-Dye, O'Connor, Welz and von Samson-Himmelstjerna2012). The cyclooctadepsipeptide emodepside, an essential component of the recently registered veterinary products Profender® (Bayer Animal Health, Leverkusen, Germany) and Procox® (Bayer Animal Health, Leverkusen, Germany), was shown to have a unique mode of action involving the large-conductance calcium-activated and voltage-gated potassium channel SLO-1 (Guest et al. Reference Guest, Bull, Walker, Amliwala, O'Connor, Harder, Holden-Dye and Hopper2007; Welz et al. Reference Welz, Krüger, Schniederjans, Miltsch, Krücken, Guest, Holden-Dye, Harder and von Samson-Himmelstjerna2011) and therefore has resistance breaking properties (von Samson-Himmelstjerna et al. Reference von Samson-Himmelstjerna, Harder, Sangster and Coles2005).
Another interesting class is represented by the aminophenylamidines – including tribendimidine, which was approved for the control of human STHs only in China (Xiao et al. Reference Xiao, Hui-Ming, Tanner, Utzinger and Chong2005; Olliaro et al. Reference Olliaro, Seiler, Kuesel, Horton, Clark, Don and Keiser2011). In the late 1970s the aminophenylamidine amidantel was reported to be efficacious against several nematodes and platyhelminths (Wollweber et al. Reference Wollweber, Niemers, Flucke, Andrews, Schulz and Thomas1979). While studying aminophenylamidine structure–activity relationship, tribendimidine was developed in 1983 (Xiao et al. Reference Xiao, Hui-Ming, Tanner, Utzinger and Chong2005). Requiring lower dosages than amidantel, tribendimidine has a broad spectrum of anthelmintic efficacy in a variety of hosts, including humans (Xiao et al. Reference Xiao, Hui-Ming, Tanner, Utzinger and Chong2005). Deacylated amidantel (dAMD, also known as BAY d 9216) is the major metabolite of amidantel and tribendimidine (Yuan et al. Reference Yuan, Xu, Qu, Wang, Zhang, Wei and Guo2010) and also exerts a strong anthelmintic efficacy in nematodes (Tritten et al. Reference Tritten, Nwosu, Vargas and Keiser2012), although apparently not in cestodes – at least not in Hymenolepis microstoma (Kulke et al. Reference Kulke, Krücken, Welz, von Samson-Himmelstjerna and Harder2012). In Caenorhabditis elegans, the mode of action of tribendimidine is very similar to that of levamisole since both drugs act as agonists through at least very similar nicotinic acetylcholine receptors (Hu et al. Reference Hu, Xiao and Aroian2009). Despite similar targets, synergistic effects of levamisole and tribendimidine against Ancylostoma ceylanicum in vitro and in vivo and antagonistic effects against Heligmosomoides bakeri in vitro and in vivo (Tritten et al. Reference Tritten, Nwosu, Vargas and Keiser2012) have been reported recently. We also observed antagonistic effects of both drug classes against Nippostrongylus brasiliensis and Trichinella spiralis in vitro (Kulke et al. Reference Kulke, Krücken, Demeler, Harder, Mehlhorn and von Samson-Himmelstjerna2013).
Since the cyclooctadepsipeptides and aminophenylamidines showed a significant in vitro efficacy against third-stage larvae and adult worms of N. brasiliensis (Kulke et al. Reference Kulke, Krücken, Demeler, Harder, Mehlhorn and von Samson-Himmelstjerna2013), the present study aimed to evaluate and compare the in vivo potency of both drug classes in the N. brasiliensis model. The efficacy of combinations of cyclooctadepsipeptides with aminophenylamidines has not been investigated in vivo prior to this study. While the effects of levamisole/tribendimidine combinations were very heterogeneous when comparing different nematode species (Tritten et al. Reference Tritten, Nwosu, Vargas and Keiser2012), drug combinations of PF1022A and dAMD, PF1022A and tribendimidine, dAMD and levamisole, as well as tribendimidine and levamisole, were analysed against N. brasiliensis in vivo to investigate the absence or presence of combinatory effects in this model.
Furthermore, scanning electron microscopy (SEM) of in vivo treated N. brasiliensis was performed to further investigate the potential combinatory effects of the drug combinations on the morphology of the worms in comparison to the effects of the respective drugs alone. Finally, explorative plasma pharmacokinetics of dAMD and tribendimidine were analysed in rats, since the data in the literature are controversial in terms of the passage of tribendimidine into the blood after oral application in different mammalian hosts (Xiao et al. Reference Xiao, Hui-Ming, Tanner, Utzinger and Chong2005).
MATERIALS AND METHODS
Ethical statement
All animal studies were conducted at the laboratories of Bayer HealthCare AG, Global Drug Discovery Animal Health (Monheim, Germany). The experiments were approved by the State Office for Nature, Environment, Agriculture and Consumer Protection, North Rhine-Westphalia, Germany (LandesamtfürNatur-, Umwelt- und Verbraucherschutz Nordrhein-Westfalen) in accordance with §8a, Section 1 and 2, German Protection of Animals Act.
Drugs
Amidantel, dAMD and PF1022A were accessible at Bayer HealthCare AG, Global Drug Discovery Animal Health (Monheim, Germany). Levamisole was purchased from ABCR GmbH & Co. KG (Karlsruhe, Germany) and tribendimidine was obtained from Shandong Xinhua Pharmaceutical Company Limited (Zibo, People's Republic of China). All drugs were stored at 4 °C until further use. Drugs were always freshly prepared as dispersions in Cremophor EL (BASF, Ludwigshafen, Germany) and deionised water [1 : 3] on days of oral treatment.
Animals
Female laboratory outbred Wistar rats (HsdCpb:WU) were purchased from Harlan Netherlands at an age of 6–10 weeks with a body weight of 125–175 g. Rats were housed in Macrolon® cages under environmentally controlled conditions and kept in groups of 3–5 animals. Water and SNIFF® rodent food pellets were available ad libitum. Rats were allowed to acclimate for 7 days before starting any experiment.
Parasites and infections
The life cycle of N. brasiliensis was maintained as described previously (Kulke et al. Reference Kulke, Krücken, Demeler, Harder, Mehlhorn and von Samson-Himmelstjerna2013). Briefly, rats were subcutaneously infected with 0·5 mL Ringer's solution (118·4 mm NaCl, 4·7 mmKCl, 2·52 mm CaCl2, 1·18 mm MgSO4 1·18 mm KH2PO4, 25·01 mm NaHCO3, pH 7·4) containing routinely 2500 infective third-stage larvae (L3). On day 6 post infection (6 d.p.i.), feces were collected, mixed with charcoal and charcoal-filtered water and incubated at 37 °C and 95% relative humidity for 5 days. Larvae were collected and incubated for another 2 days at room temperature to ensure complete development to infective L3. Larvae were washed and then used for infection of rats.
In vivo efficacy of drugs against N. brasiliensis in rats
In a series of 24 consecutive experiments (blocks), a total of 500 rats were randomized into 100 groups, each consisting of five animals. The rats were infected orally with a gavage using 0·5 mL physiological sodium chloride solution containing infective L3 of N. brasiliensis. In initial experiments, different infection doses ranging between approximately 600 and 5000 L3 were used to analyse the relationship between the infective dose and recovered adult worms. Within each block all animals received the same number of L3. For each dose of a drug at least 5 rats were used. In each block, 5 vehicle-treated rats were used as infection controls. To achieve exactly the desired drug dosages, amounts of drugs were calculated for individual animals depending on body weights determined at 3 d.p.i. At 4–6 d.p.i., rats were orally treated with drugs in Cremophor EL/water dispersions. Dosages of 50, 75, 100, 125, 200, 250 and 500 mg kg−1 amidantel, 1·0, 2·5, 3·75, 4·25, 5·0, 10, 25, 50, 100 and 250 mg kg−1 dAMD, 0·5, 1·0, 1·75, 2·5, 3·75, 5·0, 10, 25, 50, 100 and 250 mg kg−1 tribendimidine, 0·5, 0·75, 1·0, 1·5, 2·0, 2·5, 5·0, 10, 25, 50, 100 and 250 mg kg−1 PF1022A, and 1·0, 2·5, 3·75, 5·0, 6·25, 7·5, 50, 100 and 250 mg kg−1 levamisole were used. The negative control groups received only Cremophor EL/water. Additionally, 12 combinations were administered (Table 1). At 7 d.p.i., rats were euthanized by carbon dioxide suffocation. Subsequently, necropsy was performed and worm burden in the duodenum was determined by counting the parasites under a dissecting microscope.
Table 1. Doses of drug combinations administered in the N. brasiliensis in vivo assay

Drug in row over drug in column.
Morphological investigation of adult N. brasiliensis after drug exposure
For determination of drug effects on parasite morphology, suboptimal dosages of 100 mg kg−1 amidantel, 2·5 mg kg−1 dAMD, 2·5 mg kg−1 tribendimidine, 2·5 mg kg−1 levamisole, and 1·0 mg kg−1 PF1022A, as well as combinations of 1·0 mg kg−1 PF1022A plus 2·5 mg kg−1 dAMD, 1·0 mg kg−1 PF1022A plus 2·5 mg kg−1 tribendimidine and 2·5 mg kg−1 levamisole plus 2·5 mg kg−1 tribendimidine were used. The negative control group received only the vehicle (Cremophor EL/water). A total of 27 rats were randomized into nine groups, consisting of 3 rats each. Otherwise, the infection- and treatment-protocol was identical to that used for drug efficacy trials.
Immediately after necropsy and resection of the guts, small intestines were opened longitudinally and worms in the intestinal mucosa were carefully isolated using fine surgical forceps. For each treatment group, worms were collected and prefixed in snap-cap vials containing 5% glutaraldehyde (SERVA Electrophoresis GmbH, Heidelberg, Germany) in 0·1 m cacodylate buffer (pH 7·3) at 4 °C overnight. Then, worms were washed 6 times using 0·1 m cacodylate buffer, followed by dehydration of the samples using an ascending ethanol series. Worms were incubated in 70, 80, 90, 96 and 100% ethanol for 15 min each followed by incubation in fresh 100% ethanol at 4 °C for 24 h. Critical point drying was performed with the CPD 030 Critical Point Dryer (BAL-TEC GmbH, Schalksmühle, Germany) and carbon dioxide as transition fluid. Dried samples were gold-sputtered for 2 min at 15 mA using the sputter coater Emitech K550 (Emitech Ltd., Ashford, England). SEM analyses were performed using a Supra 40VP (Carl Zeiss SMT AG, Oberkochen, Germany).
Plasma pharmacokinetics
Orally applied dose rates of 10 mg kg−1 dAMD or 10 mg kg−1 tribendimidine led to an almost complete elimination of the worm burden in the N. brasiliensis in vivo assays (96·2 and 99·5%, respectively). Since these dose rates did not cause any clinical side effects, they were chosen for explorative plasma pharmacokinetic analyses. To derive plasma concentration–time curves, dAMD and tribendimidine plasma levels were recorded before treatment and between 0·5 and 504 h past treatment.
Two groups of 18 rats each were used for explorative plasma pharmacokinetic analyses. After seven days of acclimatization, the pre-treatment blood samples were drawn from all rats to ensure drug absence prior to treatment. The body weight of all rats was determined 3 days prior to treatment to ensure exact dosing. Administrations were performed by gavage at single oral dose rates of 10 mg kg−1 body weight using 0·1% oral suspensions. Dose volume applied was 1 mL 100 g−1 body weight. Following a sparse sampling schedule, each animal was used to draw 3–4 blood samples by sublingual bleeding or heart puncture (only for final samples). Samples were drawn in triplicates.
Concentrations of dAMD and tribendimidine were determined by turbulent flow chromatography coupled with a tandem mass spectrometry detector. Plasma was injected directly into the turbulent flow chromatography system while it was in the loading position. Matrix components contained in the injected sample were separated from the retained analytes on an extraction column suited for pre-treatment of samples at high flow rates. After switching to the eluting position, the sample was transferred to an analytical column. The quantitative determination was performed with a tandem mass spectrometric detector. The limit of quantitation was 25 μg L−1.
Pharmacokinetic evaluation was performed using Phoenix®, version 6.3 (Pharsight Corp., Mountain View, USA). Non-compartmental analysis of extravascular dosing and sparse sampling was used to derive the pharmacokinetic profiles of dAMD in plasma.
Calculation of dose–response curves and statistical analysis
For single drug treatments, reduction of worm burden in comparison to the mean worm burden of the respective placebo-treated control (expressed in per cent) was plotted against the log10 of the drug dosages. Then, four-parameter-logistic curves were fit using GraphPad Prism 5.03. ED50 values were compared using the extra sum of squares F test. In cases where multiple tests were performed, P-values were corrected using the Bonferroni–Holmes procedure.
The combinations were compared to respective single drug treatments using the non-parametric Kruskal–Wallis test with Dunn's post hoc test for identification of significant differences between individual groups. In order to identify any additive or synergistic drug effects, efficacy of the combinations was compared in a Wilcoxon signed rank-sum test to a theoretical expected median, which was set to the sum of the median efficacies of the individual drugs. These calculations were also performed using GraphPad Prism software version 5.03.
RESULTS
Reproducibility of worm recovery for different infective doses
Since the 24 no-drug control groups (each consisting of 5 animals) varied in the infective doses (600–5000 larvae per rat), preliminary statistical analyses were performed. A positive correlation between the absolute number of larvae used for the oral infection and the absolute number of adult worms recovered from the small intestines after necropsy was identified by linear regression (Fig. 1A). There was a significant positive correlation between the number of adult worms recovered from the intestines and the infective dose (P < 0·0001). The slope of the regression curve was 0·3097±0·01849 and the coefficient of determination was R 2 = 0·7056.

Fig. 1. Reproducibility of worm recovery in placebo-treated rats. (A) Using infective doses of between 600 and 5000 Nippostrongylus brasiliensis third-stage larvae, recovery of adult worms showed significant correlation between infective dose and worm burden (P < 0·0001). (B) When recovery was calculated in percentage of the infective dose, comparison between eight experiments with rats infected with 2000 larvae revealed high variability between individual animals but no significant differences between experiments as determined in a Kruskal–Wallis test. (C) Significant differences in recovery rates were observed between experimental groups infected with different numbers of larvae. However, when recovery rates were compared between infective doses ranging from 1000 to 2500 larvae, no significant differences were detectable.
Comparison of control groups from eight independent experiments with a consistent infective dose of 2000 larvae revealed considerable variation in recovery rates (as a percentage of the infective dose) between individual rats but no significant differences in the recovery rates of adult worms between experiments (Fig. 1B). When recovery rates between different infective doses were compared using a Kruskal–Wallis followed by a Dunn's post hoc test, the lowest dose of 600 larvae and the two highest doses of 3000 and 5000 larvae resulted in significantly lower or higher worm recovery rates, respectively (Fig. 1C). No significant difference in the relative recovery rate of adult worms was identified between any of the infective doses between 1000 and 2500 larvae (Fig. 1C). Therefore, only experiments with an infective dose between 1000 and 2500 larvae were included in the analyses of the in vivo efficacy of the test compounds against N. brasiliensis (n = 400 rats).
In vivo efficacy of drugs against N. brasiliensis in rats
Levamisole, PF1022A and the aminophenylamidines amidantel, dAMD and tribendimidine diminished the worm burden in the small intestines in a dose-dependent manner. The calculated dose–response curves of all tested drugsare shown in Fig. 2, whereas Table 2 summarizes the ED50 values with 95% CI and R 2 values. Furthermore, P-values for comparisons between ED50 values of different aminophenylamidines are indicated (Table 2). Due to the large variability in worm recovery between individual rats as described above, R 2 for these in vivo dose–response curves are relatively low (0·45–0·87).

Fig. 2. In vivo dose-response curves for efficacy of levamisole (LEV), deacylated amidantel (dAMD), tribendimidine (TBD), amidantel (AMD) and PF1022A against adult Nippostrongylus brasiliensis. Efficacy was calculated as relative number of worms recovered from the intestines compared with the vehicle control in percentage. Data shown are means±s.e.m. The drug dosages in the negative controls were set to 10−4 mg kg−1 to allow log10 transformation of dosages. Logistic regressions were calculated with top and bottom values constrained between 0 and 100%.
Table 2. Comparison of tested drugs in the N. brasiliensis in vivo assay. Presented are the ED50 values with the 95% confidence intervals (CI) and coefficients of determination (R 2) as well as P values, for determination of significant differences

a Significant difference in ED50 to drug in parentheses. P values were corrected for multiple testing using the Bonferroni–Holmes method.
The ED50 value for amidantel was approximately 30 times higher than the ED50 values of the other aminophenylamidine derivatives dAMD and tribendimidine (Table 2). In contrast to the highly significant differences between amidantel and its derivatives dAMD and tribendimidine, the ED50 values of dAMD and tribendimidine were not significantly different, illustrated by their very similar dose–response curves (Fig. 2B). The cyclooctadepsipeptide PF1022A and the reference drug levamisole also showed high in vivo efficacy against N. brasiliensis. The ED50 value for PF1022A (1·2 mg kg−1) was approximately three to four times lower than that of levamisole (4·8 mg kg−1), dAMD (3·8 mg kg−1) and tribendimidine (3·4 mg kg−1).
In vivo effects of drug combinations
Combinations of PF1022A and dAMD, PF1022A and tribendimidine, dAMD and levamisole as well as tribendimidine and levamisole were investigated against N. brasiliensis in vivo at suboptimal dosages (⩽50% reduction in worm burden) of the single drugs (Fig. 3). In a series of preliminary statistics all drug combinations and single drug dosages were compared with the no-drug control by using the non-parametric Kruskal–Wallis test with Dunn's post hoc test for identification of significant differences between individual groups. Significant differences were identified only for the drug combinations but not for the single drugs. Combinations of 5·0 mg kg−1 levamisole plus 3·75 mg kg−1 dAMD and 5·0 mg kg−1 levamisole plus 2·5 mg kg−1 dAMD, but not 2·5 mg kg−1 levamisole plus 2·5 mg kg−1 dAMD led to a significant reduction of the worm burden (P < 0·0001). The same combined dosages of levamisole plus tribendimidine reduced the worm burden in a highly significant manner, but also the combination of 2·5 mg kg−1 levamisole plus 2·5 mg kg−1 tribendimidine was significantly more efficacious against N. brasiliensis compared with the effects of the respective single drugs (P < 0·0001).

Fig. 3. Effects of drug combinations in comparison to treatments with individual drugs at the same concentrations. Combinations of levamisole and deacylated amidantel (dAMD) (A), levamisole and tribendimidine (B), dAMD and PF1022A (C), and tribendimidine and PF1022A (D) were administered. Combinations were compared with single drug treatments with the same dosages using the Kruskal–Wallis test followed by the Dunn's post hoc test. Arithmetic means are indicated in the box plots by a cross. Whiskers range from minimal to maximal values. Indices at the combinations indicate: d, P < 0·05 vs dAMD; dd, P < 0·01 vs dAMD; l, P < 0·05 vs levamisole; ll, P < 0·01 vs levamisole; p, P < 0·05 vs PF1022A; t, P < 0·05 vs tribendimidine.
While the three tested dAMD/PF1022A combinations (1·5 mg kg−1 PF1022A plus 3·75 mg kg−1 dAMD, 1·5 mg kg−1 PF1022A plus 2·5 mg kg−1 dAMD and 1·0 mg kg−1 PF1022A plus 2·5 mg kg−1 dAMD) led to a significant reduction of the worm burden, only two PF1022A-tribendimidine combinations were significantly efficacious (1·5 mg kg−1 PF1022A plus 3·75 mg kg−1 tribendimidine and 1·5 mg kg−1 PF1022A plus 2·5 mg kg−1 tribendimidine) (P < 0·0001). The third combination (1·0 mg kg−1 PF1022A plus 2·5 mg kg−1 tribendimidine) was not significantly better than the single drugs.
Comparisons of the three different dosages tested for each combination detected no significant differences. There were also no significant differences between combinations of tribendimidine and levamisole and the equivalent dosages of dAMD and levamisole. In addition, combinations of PF1022A with either tribendimidine or dAMD showed no significant differences in efficacy.
Next, all drug combinations were compared with the respective single drug dosages by using the Kruskal–Wallis test with Dunn's post hoc test. Combinations of dAMD plus levamisole and of dAMD plus PF1022A were only significantly better than dAMD alone but not significantly more efficacious than levamisole (Fig. 3A) and PF1022A (Fig. 3C), respectively. However, combinations of tribendimidine plus levamisole and of tribendimidine plus PF1022A led to a significantly higher elimination of the worm burden than tribendimidine, levamisole or PF1022A alone (Fig. 3B and D).
Since a number of combinations were significantly more effective than the single drugs, a Wilcoxon rank-sum test against the expected values of the sum of the single drug medians was performed to differentiate additive and synergistic effects. All drug combinations were not significantly different from the expected sum of the single drug efficacies, suggesting that drug combinations acted additively.
Morphological effects of drug treatments on N. brasiliensis by SEM
SEM studies of N. brasiliensis treated in vivo with (i) 1·0 mg kg−1 PF1022A, (ii) 100 mg kg−1 amidantel, (iii) 2·5 mg kg−1 dAMD, (iv) 2·5 mg kg−1 tribendimidine, (v) 2·5 mg kg−1 levamisole or (vi) placebo (Cremophor EL/water) were performed to investigate the effects of the different drug classes on the overall morphology of the worms. Furthermore, treatments with combinations of (i) 1·0 mg kg−1 PF1022A and 2·5 mg kg−1 dAMD, (ii) 1·0 mg kg−1 PF1022A and 2·5 mg kg−1 tribendimidine, (iii) as well as 2·5 mg kg−1 tribendimidine and 2·5 mg kg−1 levamisole were performed to detect any potential combinatory effects of the drug combinations on the morphology of the worms in comparison to the respective drugs alone.
The typical morphology of vehicle-treated N. brasiliensis is shown in Fig. 4. The overall condition of the untreated worms was very homogeneous as far as the body surface, attributed to the high hydrostatic pressure in the fluid-filled pseudocoel, acting as a hydroskeleton against the rigid cuticule (Fig. 4A), the typical amphids located in the apical region (Fig. 4B), the occurrence of longitudinal ridges of the cuticule along the whole body (Fig. 4C and D) as well as the well-developed cephalic cap with the triradiate mouth opening (Fig. 4D) are concerned. This indicates that the worms were intact and in an unscathed condition before fixation. Gender-specific characteristics such as the copulatory bursa at the posterior end of male worms (Fig. 4A), as well as the vulva aperture surrounded by thick lips and the anus at the base (Fig. 4E) and the pair of phasmids at the tip of the tail of females (Fig. 4F) are illustrated. The wrinkle formation of the cuticula in Fig. 4F is due to the fixation.
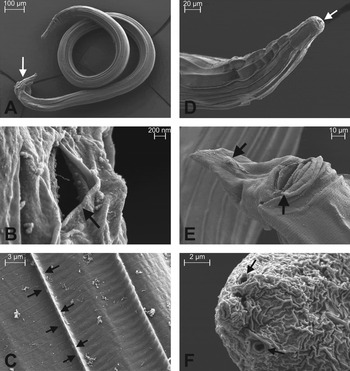
Fig. 4. Overall appearance of untreated adult Nippostrongylus brasiliensis by scanning electron microscopy. (A) Male worm with the copulatory bursa at the posterior end of the tail (arrow). The worm is approximately 2400 μm long and 85 μm wide at midbody (widest part). (B) Detailed view of an amphid (see arrow) of a male worm, a secretory organ secreting e.g. acetylcholine esterase. (C) Detailed view of the cuticule of the midbody of a male worm. Two longitudinal ridges (one is marked by arrows) and unspecified debris are present. (D) Anterior region of a female worm. The well-developed cephalic cap with the triradiate mouth opening (arrow) does not show any longitudinal ridges. (E) Ventral view of the caudal end of a female worm showing the vulva aperture (arrow) surrounded by thick lips and the anus (arrow) at the base of the tail. (F) Detailed view of a pair of phasmids (see arrows) at the tip of the tail of an adult female worm.
The overall shape of the PF1022A-treated worms was indistinguishable from the placebo-treated N. brasiliensis (Fig. 5A and B). Only at a very high magnification, several small cuticular knobs were observed next to the longitudinal ridges after three treatments with 1 mg PF1022A kg−1 body weight (Fig. 5C). However, treatments with levamisole had significant effects on the total body shape of both male and female worms (Fig. 5D and E). Whereas the whole body of male worms was severely contracted (Fig. 5D), the morphological changes of the females were limited to the posterior part (Fig. 5E), which appeared to be strongly swollen, presumably due to the completely retracted caudal end (Fig. 5E and F). Similar effects were observed when N. brasiliensis were treated with aminophenylamidines (Fig. 6). Since treatments with amidantel, dAMD and tribendimidine had identical effects on N. brasiliensis morphology, only exemplary specimens are shown. Whereas male worms looked indistinguishable from the levamisole treated specimens (Fig. 6D), females were also swollen and contracted along the whole body (Fig. 6A) except for the cephalic cap, which corresponded to the phenotype of vehicle-treated specimens (Fig. 6B). Just like levamisole, the aminophenylamidines induced a complete retraction of the tail region, so that the vulva aperture was no longer visible (Fig. 6C). Only tribendimidine caused extrusion of the two twisted spicules (Fig. 6E and F).
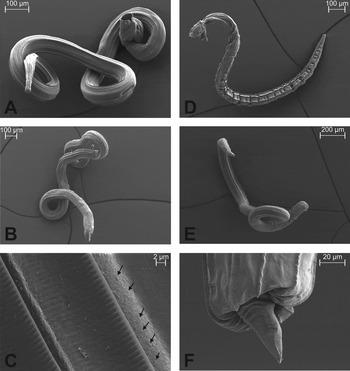
Fig. 5. Overall appearance of anthelmintic-treated adult Nippostrongylus brasiliensis by scanning electron microscopy. Worms in (A–C) were treated with PF1022A and in (D–F) with levamisole. (A) Isolated adult male worm after three treatments with 1 mg PF1022A kg−1 body weight. (B) Female worm after three PF1022A-treatments. The overall appearance is indistinguishable from untreated males and females. (C) Detailed view of the cuticule after treatments with PF1022A. Next to the longitudinal ridges, several small tegumental knobs (see arrows) are present. (D) Male worm after three treatments with 2·5 mg levamisole kg−1 body weight. The whole body is strongly contracted and swollen. (E) Adult female worm after treatments with levamisole. Similar to the male worm in (D), the posterior end appears to be contracted and swollen. (F) Posterior end of a female worm after three levamisole-treatments. The caudal end is almost completely retracted.

Fig. 6. Morphology of adult Nippostrongylus brasiliensis treated with aminophenylamidines by scanning electron microscopy. (A) Adult female worm after three doses of 100 mg amidantel kg−1 body, showing a strongly contracted and swollen body. (B) The cephalic cap of a dAMD treated female worm corresponds with the phenotype of untreated specimens. The anterior part of the cap consists of eight wider annuli, followed by 17 narrower annuli and the cephalic boundary. (C) Tail region of a female worm after 3 treatments with 2·5 mg tribendimidine kg−1 body weight. The caudal end is retracted up to the anus aperture. The vulva aperture became completely invisible. (D) Male worm after three dAMD-treatments. The whole body is strongly contracted and swollen. (E) Copulatory bursa from a male worm treated with tribendimidine. Two twisted spicules (see arrow) are extruded from the cloaca (not shown). A small dorsal and two large lateral lobes surround the cloaca. (F) Detailed view of extruded copulatory spicules of a tribendimidine-treated adult male worm.
Since none of the combinations altered the effects of the aminophenylamidines alone, these images are not shown.
Plasma concentration-time-profiles and derived pharmacokinetics
In contrast to its metabolite dAMD, tribendimidine was not detectable above its limit of quantification in plasma after single oral dosing at a rate of 10 mg kg−1 body weight to rats. Tribendimidine rapidly degraded to dAMD in an aqueous environment and was not detectable even in plasma samples spiked with tribendimidine. Plasma pharmacokinetic profiles of dAMD derived after dosing dAMD or tribendimidine were comparable (Fig. 7). Since only a small number of data points were available during the elimination phase, half-life could not be calculated reliably. Means (±s.e.m.) of plasma pharmacokinetic parameters of dAMD are presented in Table 3.

Fig. 7. Plasma pharmacokinetic analyses of deacylated amidantel (dAMD) after oral treatment with dAMD. Mean plasma concentrations (± s.d.) of dAMD after single oral administration of dAMD (circles) or of tribendimidine (rectangles) were recorded over time. For all time points where no dAMD was detected, the value was set to 25 μg mL−1, i.e. the limit of quantification. No dAMD was detected at time points more than 24 h after drug application.
Table 3. Plasma pharmacokinetic parameters of deacylated amidantel after oral treatment with deacylated amidantel or tribendimidine

a After treatment with dAMD.
b After treatment with tribendimidine.
C max, maximal plasma concentration; AUClast, area under the curve to the last measurement time point;±s.e.m., standard error of the mean.
No significant differences were observed between treatment with tribendimidine and dAMD.
DISCUSSION
Within the initial experiments presented in this article, the reproducibility of inoculum size and worm recovery was investigated. Data show relatively high variability between individual animals within each block but differences between blocks were not statistically significant. This underlines the requirement of relatively high numbers of animals when testing anthelmintic drugs to obtain meaningful data. High variability was also observed for intermediate efficacy rates in the drug-treated groups, highlighting the fact that the number of animals essentially contribute to the goodness of fit (dose–response curve) and the related determination of the ED50. If only intermediate efficacy rates are available, interpretation of data will remain vague and therefore increase the risk of misinterpretation.
The study presented here aimed at the evaluation and comparison of the in vivo nematocidal efficacy of the cyclooctadepsipeptide PF1022A, the aminophenylamidines amidantel, dAMD and tribendimidine and the reference drug levamisole in rats experimentally infected with the rat hookworm N. brasiliensis. The utilization of drug combinations is considered to be a powerful tool in the field of veterinary parasitology (Leathwick et al. Reference Leathwick, Hosking, Bisset and McKay2009; Geary et al. Reference Geary, Hosking, Skuce, von Samson-Himmelstjerna, Maeder, Holdsworth, Pomroy and Vercruysse2012; Prichard et al. Reference Prichard, Basanez, Boatin, McCarthy, Garcia, Yang, Sripa and Lustigman2012). In principle, drug combinations can be used for three purposes: (i) to increase the spectrum (e.g. emodepside and praziquantel in Profender®); (ii) to improve the efficacy of drugs (e.g. abamectin and derquantel in Startect®); and (iii) to decelerate the development of drug resistance (e.g. abamectin and derquantel in Startect®) (Geary et al. Reference Geary, Hosking, Skuce, von Samson-Himmelstjerna, Maeder, Holdsworth, Pomroy and Vercruysse2012). Since Beach et al. (Reference Beach, Streit, Addiss, Prospere, Roberts and Lammie1999) found in a randomized, placebo-controlled trial that albendazole plus ivermectin treatments had a significantly higher efficacy against human Wuchereria bancrofti infections and soil-transmitted helminthiases including Trichuris trichiura in comparison to the respective single drug efficacies, investigations regarding drug combinations to treat human helminth infections were also intensified (e.g. Knopp et al. Reference Knopp, Mohammed, Speich, Hattendorf, Khamis, Khamis, Stothard, Rollinson, Marti and Utzinger2010; Speich et al. Reference Speich, Ame, Ali, Alles, Hattendorf, Utzinger, Albonico and Keiser2012). Accordingly, in the present study combinations of PF1022A plus dAMD, PF1022A plus tribendimidine, levamisole plus dAMD and levamisole plus tribendimidine were evaluated in a N. brasiliensis in vivo assay to detect any potential combinatory effects in comparison to the effects of the individual drugs alone.
PF1022A showed a high degree of efficacy against N. brasiliensis in vivo, illustrated by an ED50 value of 1·213 mg kg−1 and by complete elimination of worm burdens using three dosages of at least 2·5 mg kg−1 PF1022A each. This corroborates a number of previously published in vitro and in vivo studies showing that PF1022A is highly effective against gastrointestinal nematodes including the rat hookworm (for review see Krücken et al. Reference Krücken, Harder, Jeschke, Holden-Dye, O'Connor, Welz and von Samson-Himmelstjerna2012). In in vitro assays it has been shown that PF1022A is anthelmintically active against the infective L3 as well as the adult stage of N. brasiliensis (Kulke et al. Reference Kulke, Krücken, Demeler, Harder, Mehlhorn and von Samson-Himmelstjerna2013). The in vivo results presented here agree with a previous study, which employed oral treatments on 3 consecutive days starting at 5 d.p.i. Dosages of 2·5 mg kg−1 PF1022A resulted in an almost complete elimination of N. brasiliensis (Wang et al. Reference Wang, Watanabe, Shomura and Ohtomo1995). Therefore, the efficacy of PF1022A against N. brasiliensis appears to be in the same dose range as the required emodepside dosage (2·5 mg kg−1, but in a single oral treatment regime) (Harder and von Samson-Himmelstjerna, Reference Harder and von Samson-Himmelstjerna2002).
The morphology of N. brasiliensis after PF1022A treatments was largely unchanged as shown by SEM, except for several small cuticular knobs next to the longitudinal ridges. Since the cyclooctadepsipeptides are known to cause flaccid paralysis, it was not unexpected to find only minimal changes due to PF1022A treatment. However, the cause of the cuticular knobs remains unknown.
In the present study, the in vivo EC50 for amidantel was about 30-fold higher than that of tribendimidine and dAMD. The in vivo efficacies of the aminophenylamidines against N. brasiliensis have also been investigated previously. Using the same protocol as in the present publication, three treatments using 250 mg kg−1 amidantel resulted in complete elimination of the worm burden (Wollweber et al. Reference Wollweber, Niemers, Flucke, Andrews, Schulz and Thomas1979). This result corresponds to the present findings of an ED50 value of 109·5 mg kg−1 and an almost complete elimination of the worm burden starting at 200 mg kg−1. Regarding the in vivo efficacy of dAMD and tribendimidine against N. brasiliensis using a single-treatment regimen against adult stages, ED90 values of 5·104 and 8·435 mg kg−1 were reported, respectively (Xue et al. Reference Xue, Xiao, Xu and Qiang2010). In contrast, the ED50 values of the present study were 3·8 and 3·4 mg kg−1 for dAMD and tribendimidine, respectively and an almost complete elimination of worm burden was achieved using three dosages of 10 mg kg−1 dAMD or 5 mg kg−1 tribendimidine, which is in agreement with the results published by Xiao et al. (Reference Xiao, Hui-Ming, Tanner, Utzinger and Chong2005). It has been shown, that tribendimidine is rapidly metabolized to dAMD (Yuan et al. Reference Yuan, Xu, Qu, Wang, Zhang, Wei and Guo2010), which by itself exerts a strong anthelmintic activity against nematodes (Tomlinson et al. Reference Tomlinson, Albuquerque and Woods1985). Indeed, our pharmacokinetic analysis could not detect any tribendimidine in the plasma and even in plasma samples spiked with tribendimidine only dAMD was detectable. This strongly supports the report that tribendimidine spontaneously disintegrates in an aqueous environment to two molecules dAMD plus one molecule terephthalaldehyde (Xue et al. Reference Xue, Xiao, Xu and Qiang2010). However, our results are in marked contrast to Xiao et al. (Reference Xiao, Hui-Ming, Tanner, Utzinger and Chong2005), summarizing in their review unpublished data with e.g. peak plasma concentrations of 318 ng mL−1 tribendimidine at 1·78 h after treatment and AUC of 1674 ng×h mL−1 after oral dosage of 150 mg kg−1 tribendimidine to rats. The reason for these striking differences remains unclear, in particular since Xiao et al. (Reference Xiao, Hui-Ming, Tanner, Utzinger and Chong2005) did not provide any experimental details. The very similar time course of dAMD plasma levels observed in the present study after treatment with dAMD or tribendimidine presumably corresponds to the very similar anthelmintic activity observed for both drugs in terms of ED50 values. Since 1 mg dAMD contains approximately 25% more dAMD than what could be released from 1 mg tribendimidine (approximately 0·8 mg dAMD) and tribendimidine is spontaneously and rapidly converted to dAMD, this high similarity is slightly unexpected. This relatively small increase in available dAMD drug might either be too small to cause a significant difference or the availability of tribendimidine in the Cremophor/water dispersion might be higher than that of dAMD.
To our knowledge, this is the first study describing the effects of nicotonic acetylcholine receptor agonists on the overall surface of a parasitic nematode using SEM techniques. At least in C. elegans the aminophenylamidines share their mode of action with levamisole, since all drugs are agonists of nicotinic acetylcholine receptors (Hu et al. 2009). Therefore, the homogeneous effects on the morphology of N. brasiliensis are comprehensive. Since activation of nicotinic acetylcholine receptors results in spastic paralysis, significant effects on the total body shape of the worms are to be expected. Differences in the intensity of contractions and swellings of the worms might be due to different intrinsic drug concentrations/availability. The reason for extrusion of the two twisted spicules only after tribendimidine but not dAMD exposure remains unclear.
The efficacy of all tested combinations was consistently improved in comparison to the individual drugs. Since a Wilcoxon rank sum test against the expected values of the sum of the single drug medians was not significantly different from the efficacy of the combinations, additive effects can be presumed.
As far as combinations of levamisole and tribendimidine are concerned, the literature is somewhat controversial. Whereas synergistic effects of tribendimidine and levamisole have been reported against A. ceylanicum L3 in vitro and against adult A. ceylanicum in vivo, significantly antagonistic effects have been observed against L3 of H. bakeri in vitro and adult H. bakeri in vivo (Tritten et al. Reference Tritten, Nwosu, Vargas and Keiser2012). In a previous study, we did not find significantly higher efficacies of the levamisole plus tribendimidine combinations in comparison to at least one drug against N. brasiliensis L3 and adult worms as well as against L3 of T. spiralis (Kulke et al. Reference Kulke, Krücken, Demeler, Harder, Mehlhorn and von Samson-Himmelstjerna2013). As mentioned above, tribendimidine apparently shares its mode of action with levamisole and therefore strong changes in overall efficacy can be expected if drug concentrations are in the range where the dose–response curve is steep and synergistic effects of such doses might be expected. Since tribendimidine is unstable in water and not present in human plasma (Yuan et al. Reference Yuan, Xu, Qu, Wang, Zhang, Wei and Guo2010), the general combinatory effects of either dAMD and levamisole or tribendimidine and levamisole should be identical. However, there was a slight trend that combinations containing tribendimidine were more efficacious than combinations containing dAMD, although this was not significant. Since tribendimidine directly disintegrates into two molecules dAMD and one molecule terephthalaldehyde in aqueous environments and terephthalaldehyde exerts no efficacy against nematodes (Xue et al. Reference Xue, Xiao, Xu and Qiang2010), the reason for higher efficacies using combinations of tribendimidine/levamisole in comparison to dAMD/levamisole remains unclear as outlined above. However, synergistic effects were observed for none of the drug combinations tested. In addition to the shared mode of action, possible reasons for synergistic effects of levamisole/tribendimidine combinations, as observed in A. ceylanicum (Tritten et al. Reference Tritten, Nwosu, Vargas and Keiser2012), might be allosteric interactions of both drugs on the same receptor. Differences in the composition and sets of the acetylcholine receptor subunits between nematode species as well as between life cycle stages might result in different sensitivities to individual cholinergic anthelmintics (Kotze et al. Reference Kotze, Lowe, O'Grady, Kopp and Behnke2009; Martin et al. Reference Martin, Robertson, Buxton, Beech, Charvet and Neveu2012) but could also influence the interaction of drugs.
The effects of combinations of cyclooctadepsipeptides with aminophenylamidines have only been investigated in vitro against N. brasiliensis and T. spiralis and they were not significantly superior in comparison to either of the respective single drugs (Kulke et al. Reference Kulke, Krücken, Demeler, Harder, Mehlhorn and von Samson-Himmelstjerna2013). Cyclooctadepsipeptides boost the inhibitory system and lead to hyperpolarization of cells whereas aminophenylamidines activate the excitatory system, leading to membrane depolarization. Since both drug classes have opposite effects on the membrane potential of excitable cells, it was unclear whether the combinations might result in additive/synergistic or even antagonistic effects. The results presented here show that additive effects are possible although both drug classes act on neurological systems that antagonise each other.
In conclusion, cyclooctadepsipeptides might be an interesting class for the treatment of human STHs, since they might achieve a complete elimination of the worm burden in a single-treatment regimen (Olliaro et al. Reference Olliaro, Seiler, Kuesel, Horton, Clark, Don and Keiser2011). Due to a significant reduction of costs and management efforts, single dosages should be the preferred treatment-scheme, independently of the question: single drug or combination?
Because of their increased efficacy, broader activity spectrum and decreased risk to select for drug resistance, combinations of cholinergic drugs with cyclooctadepsipeptides should be further investigated. However, before further testing of potential drug combinations including the aminophenylamidines, there is an urgent need to obtain more data on the safety of this drug class (Epe and Kaminsky, Reference Epe and Kaminsky2013).
ACKNOWLEDGEMENTS
The authors would like to thank Professor Dr Rolf Entzeroth and Thomas Günther (Dresden, Germany) for their help with SEM.
FINANCIAL SUPPORT
The Bayer HealthCare AG funded the presented study. The Bayer HealthCare AG sells anthelmintics for use in veterinary medicine.
CONFLICT OF INTEREST
D. Kulke and K. Fraatz are employees of Bayer HealthCare AG. R. Krebber is an employee of Bayer CropScience AG and A. Harder was an employee of Bayer HealthCare AG, when the study was conducted.