INTRODUCTION
Several authors have proposed a scenario that involves climate as a causal factor in the replacement of Neanderthals by anatomically modern humans (AMH) (Leroyer and Leroi-Gourhan, Reference Leroyer and Leroi-Gourhan1983; Leroyer, Reference Leroyer and Otte1988; Mellars, Reference Mellars1992; Djindjian, Reference Djindjian, Bánesz and Kozlowski1993; Zilhão, Reference Zilhão and Cabrera1993; d'Errico and Sánchez-Goñi, Reference d'Errico and Sánchez-Goñi2003; Stewart, Reference Stewart2005). However, there is still a lack of empirical data to confirm or reject the hypotheses on this issue. Nevertheless, elucidating the paleoenvironment in which this bioanthropological transition took place appears as a requisite to reliably advance towards answering this question. In this sense, taphonomic analyses should be relevant to reach solid interpretations about the conditions of the site formation.
Fossil sites constitute open windows into past ecosystems, but the taphonomic agents have an important influence on the site formation, and therefore, on deciphering the original biological community that lived in the area and the preservation of these fossils (Denys, Reference Denys1985, Reference Denys1986; Andrews, Reference Andrews1990). To use small mammals for biochronologic, biostratigraphic, paleoenvironmental, paleoclimatic, and paleoecologic studies, taphonomic analyses are essential to establish to what degree a fossil assemblage may be considered representative of the past ecosystems (Andrews Reference Andrews1990; Fernández-Jalvo, Reference Fernández-Jalvo1992; Denys and Patou-Mathis, Reference Denys, Patou-Mathis, Denys and Patou-Mathis2004; Fernández-Jalvo et al., Reference Fernández-Jalvo, Scott and Andrews2011, Reference Fernández-Jalvo, Andrews, Sevilla and Requejo2014).
Taphonomy, as the study of the transition from the biosphere to the lithosphere (Efremov, Reference Efremov1940), retrieves meaningful paleobiological information coded on the fossils that otherwise cannot be known (Fernández-López, Reference Fernández-López1981, Reference Fernández-López1991; Behrensmeyer and Kidwell, Reference Behrensmeyer and Kidwell1985; Soligo and Andrews, Reference Soligo and Andrews2005). Numerous studies have revealed the role of raptors and/or carnivores as the principal agents that bring micromammals to paleontological and archaeological sites (Gawne, Reference Gawne1975; Andrews, Reference Andrews1990; Fernández-Jalvo, Reference Fernández-Jalvo1995; Fernández-Jalvo et al., Reference Fernández-Jalvo, Denys, Andrews, Williams, Dauphin and Humphrey1998; Williams, Reference Williams2001; Matthews et al., Reference Matthews, Denys and Parkington2005; Montalvo et al., Reference Montalvo, Pessino and Bagatto2008; Stoetzel et al., Reference Stoetzel, Marion, Nespoulet, El Hajraoui and Denys2011). Thus, the relevant paleoecological value of microfaunal species recorded in a fossil site is compromised by the eventual involvement of predators, whose bone remains may or may not have been preserved. The current fossil site most likely constitutes the original living, nesting, or roosting site of the predator (or a place where it accumulated large piles of prey remains in order to mark its territory). Thus, the microfaunal assemblages in those fossil sites reflect the predator's dietary preferences. This must be taken into account when inferring paleoecological conditions. If the predator involved is a specialist predator that favors certain hunting areas or feeds upon favorite species, the microfaunal assemblages cannot be considered representatives of the past environments because they are biased by the selective choice of the predator.
Taphonomic modifications by predation of small mammals are recognized mainly by digestion damage, breakage, and skeletal representation (Mellet, Reference Mellett1974; Mayhew, Reference Mayhew1977; Dodson and Wexlar, Reference Dodson and Wexlar1979; Andrews, Reference Andrews1990; Fernández-Jalvo, Reference Fernández-Jalvo1995). From these taphonomic variables, Andrews (Reference Andrews1990) established a systematic methodology in order to distinguish between a selective predator, which can strongly affect species representation by selecting for their favorite prey, and a generalist predator, which yields a representative spectrum of the nearby ecosystems. Therefore correct identification of the predator is essential to obtain accurate paleoenvironmental and paleoecological interpretations based on small mammal assemblages. Post-depositional processes may also disturb the original assemblage and yield incongruent interpretations of the site if they are unnoticed. Trampling, weathering, plant activity, soil corrosion, microbial attack, mineralization, sediment compression, and compaction must also be identified in order to improve environmental interpretations and potentially reveal distorting processes leading to mixtures before and/or after burial. Therefore, taphonomic studies may also add information that can influence climatic interpretations—and climate has always been considered as an influential factor involved in biogeographical reordering.
Preliminary works by Fagoaga et al. (Reference Fagoaga, Ruiz-Sánchez, Laplana, Blain, Marquina, Marin-Monfort and Galván2018, Reference Fagoaga, Laplana, Marquina, Machado, Marin-Monfort, Crespo, Hernández, Mallol, Galván and Ruiz-Sánchez2019a) showed that small mammal accumulations from the El Salt Middle Paleolithic site (Alcoi, Spain) were related to predation. To corroborate this information, we carried out a detailed and systematic taphonomic analysis of the small mammal assemblages of the El Salt site, with the objective to decipher the formation of the association. In the present study, we analyze two different stratigraphic units that were systematically excavated in the El Salt Middle Paleolithic site: one near the base of the sequence (Unit X), associated with recurrent Neanderthal occupations; and the other one near the top (Unit V), overlying the last Neanderthal occupations documented at this site. Our goal is to identify the origin of the small mammals, characterize their post-depositional alterations, and determine their associated paleoecological contexts.
BACKGROUND TO THE EL SALT MIDDLE PALEOLITHIC SITE
The Middle Paleolithic site of El Salt is located in the municipality of Alcoi (Alicante, eastern Spain) at 680 m above sea level (Fig. 1A, B). It constitutes an open-air sedimentary sequence resting against a 38 m high Paleocene limestone wall, formed at a reverse fault and covered with tufa and travertine deposits (Fumanal, Reference Fumanal1994). The stratified deposit is 6.3 m thick and was divided into 13 lithostratigraphic units (XIII-I) (Fumanal, Reference Fumanal1994). These units were then grouped into five different segments according to their macroscopic textural appearance and archaeological content (Galván et al., Reference Galván, Hernández, Mallol, Machado, Sistiaga, Molina, Pérez-Luis and Sala2014b) (Fig. 2).
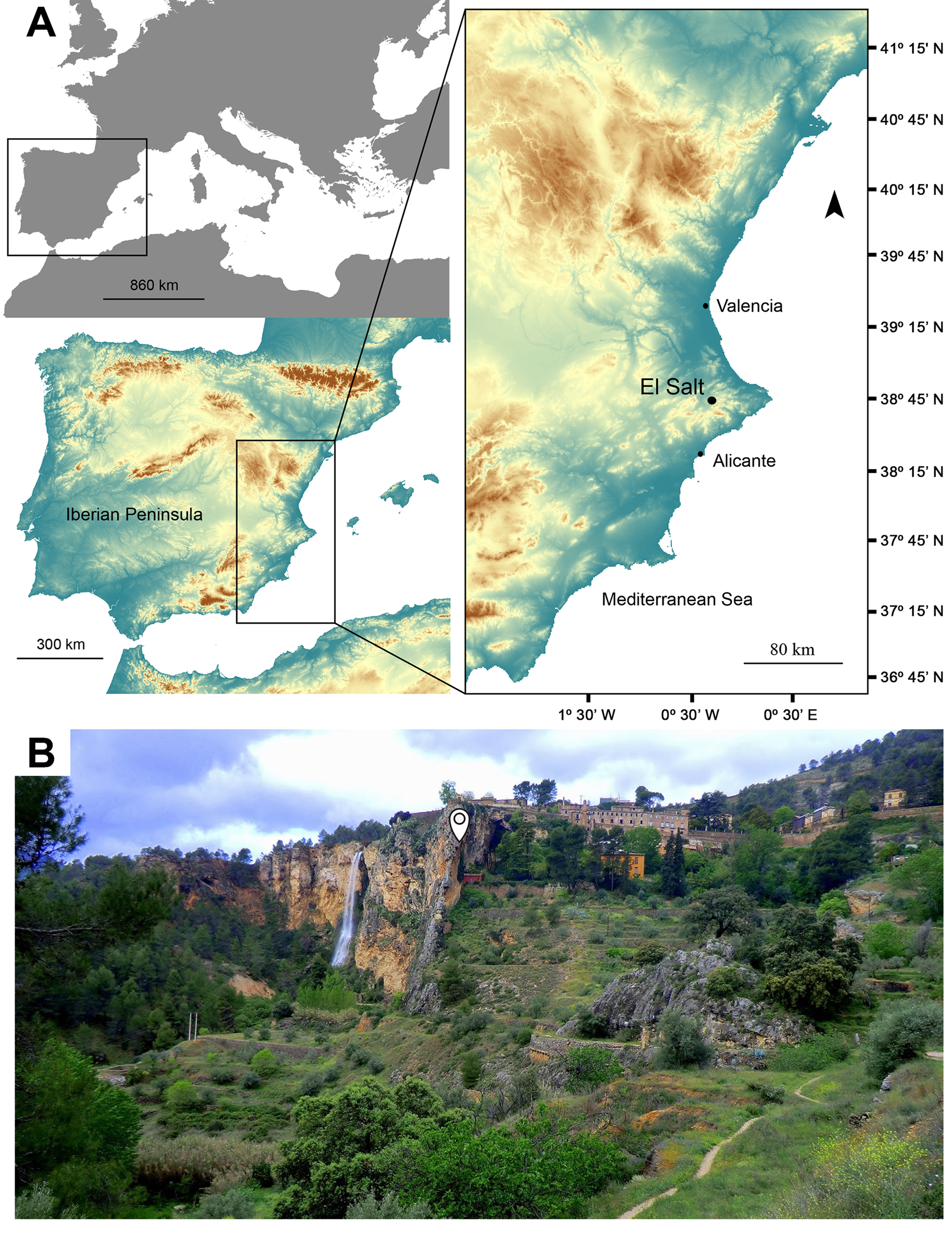
Figure 1. (color online) Geographic location (A) and landscape (B) of the El Salt site. Photograph courtesy of Juan José Dopazo Javier.

Figure 2. (color online) Stratigraphic composite log of the El Salt site, showing levels containing small-mammal fossil remains (modified from Galván et al., Reference Galván, Hernández, Mallol, Mercier, Sistiaga and Soler2014a). Detail of Subunit Xb surface and Upper Unit V (V-23, V-24, and V-25). The arrow indicates the position of a single hearth found in Upper Unit V.
The older unit analyzed in this study is Unit X (Subunit Xb), near the bottom part of the sequence, which was dated by thermoluminescence methods as 52.3 ± 4.6 ka (Galván et al., Reference Galván, Hernández, Mallol, Mercier, Sistiaga and Soler2014a) (Fig. 2). It is a silty, microgranular and calcitic unit, with abundant earthworm channels and tufa fragments. This unit has yielded 61 combustion features (Sistiaga et al., Reference Sistiaga, March, Hernández and Galván2011; Mallol et al., Reference Mallol, Hernández, Cabanes, Sistiaga, Machado, Rodríguez, Pérez and Galván2013; Galván et al., Reference Galván, Hernández, Mallol, Mercier, Sistiaga and Soler2014a, Reference Galván, Hernández, Mallol, Machado, Sistiaga, Molina, Pérez-Luis and Salab; Herrejón Lagunilla, Reference Herrejón Lagunilla, Carrancho, Villalaín, Mallol and Hernández2019; Leierer et al., Reference Leierer, Jambrina-Enríquez, Herrera-Herrera, Connolly, Hernández, Galván and Mallol2019). The combustion area, which is near the travertine wall, contains abundant faunal and flint remains and anthropogenically modified cobbles, forming a dense palimpsest of recurrent human occupations. Recent research combining micromorphology and lipid biomarker analyses from different combustion structures recovered in part of Subunit Xb revealed at least four human occupations (which most likely occurred in winter, spring, and/or summer), allowing the formation of organic-rich soils between occupations (Leierer et al., Reference Leierer, Jambrina-Enríquez, Herrera-Herrera, Connolly, Hernández, Galván and Mallol2019).
The most abundant large mammal species recorded at the site are the Spanish ibex (Capra pyrenaica), red deer (Cervus elaphus), and wild horse (Equus ferus). The bones from these species exhibit substantial anthropogenic alterations, including cut-marks, percussion marks, or thermal alterations (Pérez et al., Reference Pérez, Sanchis, Hernández, Galván, Sanchis and Pascual2017a; Pérez, Reference Pérez2019). Less-abundant, human-induced modifications have been observed on bones of auroch (Bos primigenius), wild boar (Sus scrofa), and Mediterranean tortoise (Testudo hermanni) at the site. Other faunal remains show the testimonial presence of Rhinocerotidae, Panthera pardus, Cuon sp. Canis cf. C. lupus, and Lynx sp. (Sanchis et al, Reference Sanchis, Morales, Pérez, Hernández, Galván, Sanchis and Pasual2015; Pérez et al., Reference Pérez, Sanchis, Hernández, Galván, Sanchis and Pascual2017a, Reference Pérez, Sanchis, Hernández, Galván, Sala and Mallolb), but do not bear any evidence of human manipulation.
Regarding the small mammal assemblages, Fagoaga et al. (Reference Fagoaga, Ruiz-Sánchez, Laplana, Blain, Marquina, Marin-Monfort and Galván2018, Reference Fagoaga, Laplana, Marquina, Machado, Marin-Monfort, Crespo, Hernández, Mallol, Galván and Ruiz-Sánchez2019a, Reference Fagoaga, Blain, Marquina-Blasco, Laplana, Sillero, Hernández, Mallol, Galván and Ruiz-Sánchezb) documented seven rodent species (Microtus arvalis, Microtus agrestis, Microtus duodecimcostatus, Microtus cabrerae, Arvicola sapidus, Eliomys quercinus, and Apodemus sylvaticus), four insectivore taxa (Erinaceus cf. E. europaeus, Crocidura sp., Sorex sp., and Talpidae indet.), and one lagomorph species (Oryctolagus cf. O. cuniculus). Paleoclimatic analysis based on the small mammal association from Unit X Subunit Xb shows depositional conditions that were wetter and colder than the current ones (Fagoaga et al., Reference Fagoaga, Ruiz-Sánchez, Laplana, Blain, Marquina, Marin-Monfort and Galván2018, Reference Fagoaga, Laplana, Marquina, Machado, Marin-Monfort, Crespo, Hernández, Mallol, Galván and Ruiz-Sánchez2019a, b). These climatic results agree with anthracological studies, which suggest the prevalence of bioclimatic conditions belonging to the supramediterranean belt (Vidal-Matutano et al., Reference Vidal-Matutano, Pérez-Jordà, Hernández and Galván2018). At the same time, these interpretations are consistent with a Marine Isotope Stage 3 chronology, as indicated by the associated thermoluminescence dates (Galván et al., Reference Galván, Hernández, Mallol, Mercier, Sistiaga and Soler2014a).
The other unit studied here, Unit V, records decreased evidence of human impact and progressively more geogenic sedimentation (Galván et al., Reference Galván, Hernández, Mallol, Mercier, Sistiaga and Soler2014a). Six upper teeth of a single Neanderthal individual were recovered at the base of this unit. This constitutes evidence of one of the last Neanderthal groups that occupied the site, and perhaps the region (Galván et al., Reference Galván, Hernández, Mallol, Mercier, Sistiaga and Soler2014a). The upper part of Unit V comprises a 50-cm-thick bed of massive sandy silt and a 20- cm layer of heterogeneous gravel on top. The base of this unit, corresponding to field facies 23, is archaeologically sterile, except for two small flint blades, a few undifferentiated flakes, and a small combustion feature at the top (Garralda et al., Reference Garralda, Galván, Hernández, Mallol, Gómez and Maureille2014). Overlying this quasi-sterile segment, field facies 24 is composed of silts with coarse sand and gravel (1–3 mm) and minor amounts of heterometric limestone and travertine fragments. Capping the Upper Unit V sequence is field facies 25, which is a fine-grained deposit similar to facies 23 (Fig. 2). The small mammal assemblage studied here was recovered from field facies 24 and 25.
Overall, the small mammals from Lower Unit X and Upper Unit V are very similar in species composition. However, the increase of open, dry environment species, such as Microtus arvalis and Oryctolagus cuniculus, in Unit V represents an important paleontological feature, which has been interpreted by Fagoaga et al. (Reference Fagoaga, Laplana, Marquina, Machado, Marin-Monfort, Crespo, Hernández, Mallol, Galván and Ruiz-Sánchez2019a) as indicating an increasingly dry environment from Unit X to Unit V, thus supporting that the decline of the Neanderthal population took place in a progressively more open and arid environmental context (Fagoaga et al., Reference Fagoaga, Laplana, Marquina, Machado, Marin-Monfort, Crespo, Hernández, Mallol, Galván and Ruiz-Sánchez2019a).
MATERIALS AND METHODS
The small mammal (rodents and insectivores) material analyzed in this study were recovered during the 2013 and 2014 excavation seasons from Units X and V at the El Salt site. In order to determine their anthropogenic context, the excavation methodology implemented at El Salt focused on recovering paleosurfaces. These are identified based on sharp stratigraphic contacts, textural or lithological changes, and lateral associations between large objects. Thus, this study includes materials excavated systematically in extension from Subunit Xb (specifically paleosurface 3: S3) in the lower part of Unit X, and Subunits V-24 (with three paleosurfaces analyzed: S9, S8, and S7) and V-25, in the uppermost Unit V. The taphonomic analysis was carried out for each of the subunits and paleosurfaces taxonomically analyzed by Fagoaga et al. (Reference Fagoaga, Laplana, Marquina, Machado, Marin-Monfort, Crespo, Hernández, Mallol, Galván and Ruiz-Sánchez2019a) to evaluate whether the paleoecological interpretation indicated by these authors was correct. The observed taphonomic differences between the paleosurfaces are non-significant; therefore, the data referred in the results section are shown by subunits. A full dataset, including the detailed taphonomic results referred to each paleosurface, can be found in the supplementary material (Supplementary Tables 1–5).
Table 1. Indices of skeletal proportions for the different units analyzed.

Microvertebrate fossils belonging to birds, amphibians, reptiles, and small mammals were collected by wet sieving using 0.5-mm mesh screens and sorted at the Laboratory of the Department of Botany and Geology (Universitat de València, Spain). The taxonomic identification of the small mammals was presented in Fagoaga et al. (Reference Fagoaga, Ruiz-Sánchez, Laplana, Blain, Marquina, Marin-Monfort and Galván2018, Reference Fagoaga, Laplana, Marquina, Machado, Marin-Monfort, Crespo, Hernández, Mallol, Galván and Ruiz-Sánchez2019a). The present taphonomic analysis has considered fossils from Orders Rodentia and Eulipotyphla.
Taphonomic modifications were identified and photographed under a stereomicroscope (Leica M205A). A selection of these samples was analyzed at the Museo Nacional de Ciencias Naturales of Madrid (Spain) in an environmental scanning electron microscope (Quanta 200) equipped with an X-ray energy dispersive spectrometer (EDS). The fossil collection available for this analysis is temporarily housed at the Universitat de València (Spain) until its final deposit at the Museu Arqueològic Camil Visedo Moltó in Alcoi (Spain).
Taphonomic analysis
The taphonomic analysis is based on the methodology and the dataset provided by Andrews (Reference Andrews1990), Fernández-Jalvo and Andrews (Reference Fernández-Jalvo and Andrews1992), and Fernández-Jalvo et al. (Reference Fernández-Jalvo, Andrews, Denys, Sesé, Stoetzel, Marin-Monfort and Pesquero2016). Taphonomic aspects, such as the representation of skeletal elements, breakage, digestion, and post-depositional damage of the elements, were quantified in order to (1) identify the agent(s) responsible for accumulation of the micromammal assemblage and (2) determine any additional taphonomic process, either biostratinomic or fossil diagenetic. The following sections describe in detail the different parameters considered in our analysis.
Skeletal proportions
The identified specimens were counted (NISP) and the minimum number of elements (MNE) was estimated (Lyman, Reference Lyman1994). The minimum number of individuals (MNI) also was evaluated by counting the most abundant represented element and taking into account laterality (left/right specimens). In order to evaluate the loss of skeletal elements, their relative abundance was calculated using the formula (Eq. 1) proposed by Dodson and Wexlar (Reference Dodson and Wexlar1979):

Ri is the relative abundance of element i; MNEi is the minimum number of element i observed in the sample, MNI is the minimum number of individuals, and Ei is the number of element i in a complete skeleton. This equation denotes the proportion of observed vs. expected elements given a specific MNI.
In order to evaluate the proportions of the different skeletal elements the following indices have been calculated, following Andrews (Reference Andrews1990):
Post-cranial in relation to cranial elements (Pc/C) was expressed in two ways (Eq. 2):

There are 16 cranial elements (two maxillae, two mandibles, and 12 isolated molars) for only 10 post-cranial elements (two humeri, two ulnae, two radii, two femora, and two tibiae). As shown in the formula for index Pc/C1, to compensate and obtain a percentage proportion, cranial elements were multiplied by 10 and post-cranial elements by 16 (Eq. 3).

The relationship between distal and proximal limbs (D/P) was calculated (Eq. 4) as follows:

Breakage
The breakage patterns were evaluated on cranial and post-cranial elements, sensu Andrews (Reference Andrews1990). For cranial elements, Andrews (Reference Andrews1990) established different categories of progressive skull fragmentation, including unbroken skull and maxillae with and without the zygomatic process. We also include hemi-palates, representing the maximal breakage. Hemi-mandible breakage was classified as complete, broken ascending ramus, missing ascending ramus, and missing ascending ramus with broken inferior border (i.e., the lower edge of the hemimandible).
In addition, to assess the degree of induced cranial breakage by the predator, the teeth loss and isolated teeth indices were calculated separately for incisors (I) (Eq. 5, 7) and molars (M) (Eq. 6, 8):




For incisors, alveoli refers to the number of expected incisors (based on 1 per hemimandible), given the number of mandibles in the sample. For molars, alveoli refers to the number of expected molars (based on 3 per hemimandible), given the number of mandibles in the sample.
The degree of breakage for post-cranial elements was identified considering separately the representation of complete remains from the number of proximal epiphyses, diaphysis, and distal epiphyses for limb bones (humerus, radius, ulna, femur, and tibia). For immature individuals, diaphyses of long bones with unfused epiphyses were considered as complete elements.
Additionally, recent breakage produced during the excavation and subsequent sample treatment (e.g., transport, sieving, or other laboratory procedures, etc.) also was taken into account (sullegic and trephic factors; see Clark and Kietzke, Reference Clark, Kietzke, Clark, Beerbower and Kietzke1967; Marin-Monfort et al., Reference Marin-Monfort, Suñer and Fernández-Jalvo2018). All this breakage was discarded to assess breakage related to predation and post-depositional processes. Recent post-depositional breakage was distinguished by its sharp and shiny edges that are different from the rest of the fossil (in color or texture), and not covered with sediment or other taphonomic modifications (e.g., manganese oxides, calcite, discoloration, root-marks, etc.). In contrast, old fractures are similar in texture, brightness, and color to the rest of the bone and may show some corrosion and/or precipitation evidences.
Digestion
Digestion traces were investigated for cranial and post-cranial elements to determine the kind of predator, following the criteria proposed by Andrews (Reference Andrews1990), Fernández-Jalvo and Andrews (Reference Fernández-Jalvo and Andrews1992), and Fernández-Jalvo et al. (Reference Fernández-Jalvo, Andrews, Denys, Sesé, Stoetzel, Marin-Monfort and Pesquero2016). According to these authors, five degrees of digestion were distinguished for molars and incisors: 0 = no digestion; 1 = light, 2 = moderate, 3 = heavy, and 4 = extreme. These were evaluated separately for in situ and isolated teeth.
Digestion in post-cranial elements was recognized on proximal epiphyses of the femora and distal ends of humeri following Marin-Monfort et al. (Reference Marin-Monfort, García-Morato, Olucha, Yravedra, Piñeiro, Barja, Andrews and Fernández-Jalvo2019). The number of digested remains was compared to the total number of femora and humeri in order to obtain proportions of affected fossil bones.
Post-depositional modification
The surfaces of long bones (humeri, radii, ulnae, femora, and tibiae) were examined to detect evidence of post-depositional processes. Modifications (e.g., cut marks, tooth marks, rounding, polishing, cracking, trampling, corrosion, burning, manganese deposits, root marks, etc.) can be used to identify agents that could have altered the original assemblages, before, during, or after burial. Comparative data for all features can be found in Shipman et al. (Reference Shipman, Foster and Schoeninger1984), Andrews and Cook (Reference Andrews and Cook1985), Andrews (Reference Andrews1990), Lyman (Reference Lyman1994), Cáceres (Reference Cáceres2002), and Fernández-Jalvo and Andrews (Reference Fernández-Jalvo and Andrews2016), among others.
The analysis of root etching is based on the classification proposed by Stoessel (Reference Stoessel2007) and Alcaraz (Reference Alcaraz2015), and modified by Martínez et al. (Reference Martínez, Martínez, Alcaráz and Stoessel2018). These authors established five categories based on the percentage of surface covered by roots: 0 (absent), 1 (25%), 2 (50%), 3 (75%), and 4 (100%). In this work, due to the high number of samples that showed <10% of the surface affected, a new category was included (5–10%). The same classification was applied for manganese staining and cementation.
Specimens also were grouped on the basis of their potential for hydraulic transport, following the generalized sequence established by Korth (Reference Korth1979). Proportions of the elements within the groups for the general sequence were calculated and compared with the proportions of the elements found in the site.
Statistical analysis
Values obtained for the analyzed taphonomic variables (Ri, breakage, and digestion) were compared to corroborate the presence of similarities or differences between levels. Because the obtained data fit a non-normal distribution, the square root of the relative abundances was obtained (√Ri), and the result was analyzed using a simple ANOVA applying a post-hoc Tukey test.
Breakage, digestion, and post-depositional modifications were analyzed applying a Fisher test. This non-parametric test is most applicable because it works well with small sample sizes and allows introducing 0 values for post-hoc multiple comparisons. All statistical analyses were performed with the free software program R, version 3.6.2 (R Core Team, 2020).
RESULTS
Skeletal element representation
The anatomical composition of the fossil assemblage recovered from Unit X (Xb) and Unit V (V-24 and V-25) is shown in Figure 3. We recovered 3383 remains (MNE)—2573 cranial and 810 post-cranial—from both units. The relative skeletal element frequency (Ri) reveals that all the anatomical elements are present, even for the small-sized elements, such as phalanges. In this respect, calcanei and astragali are abundant in Unit V and slightly scarcer in Unit X (Fig. 3). Statistical analyses revealed no differences between the anatomical representation of the different stratigraphic levels (ANOVA: F = 2.53, Df = 2, p = 0.0903, n = 51).

Figure 3. Relative abundance of the skeletal elements (Ri%) from Subunits Xb, V-24, and V-25.
When comparing the total post-cranial with cranial skeletons, the results point to an index below 100, showing an abundance of cranial elements when teeth are included (Pc/C1) (Table 1). However, when teeth are not considered in the formula (Pc/C2), all values increase and Subunit V-24 shows values slightly above 100, which indicates a predominance of post-cranial elements. In the case of Subunit V-25, both indices yield similar results, with cranial and post-cranial skeletons equally represented. The relationship between distal and proximal elements (D/P) showed a better representation of distal remains in Unit X, while proximal elements of the limbs are dominant for Unit V (Table 1).
Breakage
This taphonomic modification was analyzed considering the degree of breakage observed both in the cranial and the post-cranial elements (Fig. 4). As a whole, the El Salt assemblage showed a high degree of breakage. No complete maxilla has been recovered, with the exception of Subunit V-24, which had one maxilla preserving the zygomatic process. Fractured mandibles are dominant in the sample—only two (8.33%) complete mandibles were found in Unit X and one (2.55%) in Unit V (specifically in Subunit V-24). Mandibles frequently show broken inferior borders, indicating intensive breakage. No statistical differences were found for maxilla and mandibles between both units (Fisher Test maxilla: p = 0.1834, n = 66; Fisher Test mandibles: p = 0.8136, n = 90).

Figure 4. Representation percentage of the different portions of small mammal cranial and long bones.
The high percentage of isolated teeth suggests a high amount of cranial breakage, which is also supported by abundant tooth loss in the analyzed mandibles (Fig. 4). In Unit X, more than 75% of teeth were missing from the alveoli (75.76% for incisors; 86.87% for molars), while in Unit V even more molars were detached from maxilla and mandibles (80–98%).
A high degree of breakage also has been observed in long bones, except for the sample recovered from Unit X, where several unbroken elements have been found. Among the fragmented skeletal limbs, overall, the best-preserved portions are the proximal epiphyses of femora, ulnae, and radii and the distal portions of humeri, with the exception of tibiae shafts, which are generally scarce. Recent breakage resulting from sullegic and trephic factors is observed in a range of ~10–20% of the long bones in the sample (femora, tibiae, humeri, ulnae, and radii). Results of the statistical analysis comparing the breakage of each element between the different units are shown in Table 2. Only femora and humeri show significant differences.
Table 2. Results for the Fisher test applied to post-cranial elements. Significant p-values are shown in bold.

Digestion
Teeth with evidence of digestion have been recorded in both Unit X and Unit V (Figs. 5, 6): ~33% of molars were digested in Unit X and 23% of them in Unit V. As for incisors, this rate reaches 53% in Unit V, but is clearly lower in Unit X (19%) where a low number of them have been recovered (57 both isolated and in situ). Moderate and heavy damage was observed in both units. Extreme digestive corrosion also was observed, but at low percentages.

Figure 5. Representation of the percentage of digestion in cranial (molars and incisors) and post-cranial (proximal femur and distal humerus) elements accumulated from Subunits Xb, V-24, and V-25 in the El Salt site.

Figure 6. Scanning electron microscope micrographs showing examples of digestion traces observed at the El Salt site. A. Incisor with light digestion. B. Incisor heavily digested. C. Second upper murid molar showing moderated digestion. D. Murid molar heavily affected by digestion process. E. Arvicoline molar showing extreme digestion. F. Humeri lightly digested and detail of the modification. G. Femora lightly digested and detail of the modification.
Regarding long bones, digestion is shown in Figure 5C. Approximately 30% of the proximal parts of the femora and the distal portions of the humeri were modified by digestive corrosion in the two analyzed sedimentary units at El Salt. These elements displayed characteristic traces of dissolution on their articular ends, and some showed their whole surface altered, as well as broken edges with rounded surfaces (Fig. 6F, G). No statistical differences were found in teeth and post-cranial elements between the two analyzed units (Fisher Test cranial remains: p = 0.0579, n = 660; Fisher Test post-cranial remains: p = 0.9345, n = 91).
Post-depositional modifications
The majority of post-depositional modifications (Fig. 7) on the surfaces of the studied material appear to have been caused by manganese coatings, as revealed by Energy Dispersive Spectrometry (EDS) (Fig. 8A). In Unit X, the degree of manganese deposit mostly corresponds to Category 2 (i.e., 25% of the surface was covered with manganese). In Unit V, manganese is more scattered and, when present, fits in Category 1 (~5–10% of the surface is covered with manganese) in both Subunits V-24 and V-25. Statistical analyses show significant differences between Unit X and Unit V (Fisher Test, p = 4.998e-04, n = 265). Calcareous concretions adhered to the surface of the remains also have been detected in El Salt, as revealed by EDS analysis (Fig. 8B). Nevertheless, the degree of cementation never exceeded 35% in any unit, and the category most commonly assigned was Category 1 (5–10%) (Fig. 7).

Figure 7. Post-depositional modifications identified on the micromammal remains from Subunits Xb, V-24, and V-25 in the El Salt site.

Figure 8. (color online) A. Electron micrograph showing massive manganese oxide deposits on the fossil surface. The chemical composition analysis was performed on a dark coating developed on a long bone fragment. B. Electron micrograph showing the presence of carbonate concretions. The chemical composition analysis was carried out on the crusts covering the surface of a bone fragment.
Perforations and sinuous superficial grooves related to plant root action leading to chemical etching are the main effects observed in Unit X. Almost all of the faunal material recovered in Unit X showed these perforations (Fig. 7), whereas they were less abundant in Unit V. Manganese and carbonate deposits frequently have been found inside the root marks, indicating that the plant activity was previous to the mineral deposits. In Unit X, the degree of surface covered with root marks has been commonly ascribed to Categories 2 and 3 (25 and 50% of the surface, respectively). In Unit V, root etching (Fig. 9A) has been assigned mainly to Categories 1 and 2 (5–25% of the surface covered). As it is the case with manganese coatings, the presence of root marks is significantly different between Unit X and Unit V (Fisher Test, p = 4.998e-04, n = 265). It should be taken into account that both manganese and root etching could cover and obliterate other taphonomic alterations, such as tooth or beak marks, cut and percussion marks or trampling, which were not identified in this sample.

Figure 9. Examples of post-depositional damages found at the El Salt site. A. Rodent femur with intense root corrosion. B. Tibia with stage 1 weathering. Detail of a cracking is shown in the lateral inset magnification C. Left: brown colored, weakly burned femur; right: black colored carbonized bone. (For interpretation of the references to color in this figure legend, the reader is referred to the web version of this article.)
Weathering, cracking by humidity, abrasion, and thermal alteration have been detected only in Unit X. Weathering is characterized by small cracks parallel to the fibrous structure of the bone, along with some chipped teeth, and corresponds to weathering stage 1, according to the classification of Andrews (Reference Andrews1990) (Fig. 9B). Some of these cracks showed elevated and contorted sides, which constitutes a specific feature produced by high levels of humidity or damp conditions (Fernández-Jalvo and Andrews, Reference Fernández-Jalvo and Andrews2016). Thermo-alteration of the analyzed fossils never exceeded carbonization (charred black color) (Fig. 9C). Abrasion and rounding of the salient angles and surface polishing have been described only in a single specimen, corresponding to Category 1 of Cáceres (Reference Cáceres2002). Korth groups represented in Figure 10 show the presence of elements from all groups and low-density remains, such as ribs and vertebrae.

Figure 10. Representation of the Korth groups (Korth, Reference Korth1979) in Subunits Xb, V-24, and V-25. Group I: ribs; Group I/II: vertebrae, radius, ulna, pelvis; Group II: scapula, maxilla, calcaneum, astragalus, humerus, femur; Group II/III: molars; Group III: mandible, tibia.
DISCUSSION
Origin of the small mammal accumulation
Despite the consistent presence of Neanderthals at El Salt Subunit Xb (Galván et al., Reference Galván, Hernández, Mallol, Mercier, Sistiaga and Soler2014a), our results do not show small mammal remains bearing traits attributable to anthropogenic activity (Dewar and Jerardino, Reference Dewar and Jerardino2007), except for some burnt specimens that were most likely modified by exposure to heat when already buried in sediment underneath a hearth (De Graaff, Reference De Graaff1961; Stiner et al., Reference Stiner, Kuhn, Weiner and Bar-Yosef1995; Bennett, Reference Bennett1999; Pérez et al., Reference Pérez, Sanchis, Hernández, Galván, Sala and Mallol2017b; Fernández-Jalvo et al., Reference Fernández-Jalvo, Tormo, Andrews and Marin-Monfort2018).
Apart from predators, other possible causes for the accumulation of micromammal fossils are natural death, depositional agents such as water, wind, or falling blocks, as well as catastrophic events (Denys, Reference Denys1985; Andrews, Reference Andrews1990; Laudet et al., Reference Laudet, Denys and Fernández-Jalvo1997). The absence of relative skeletal completeness and/or articulated skeletons (Andrews, Reference Andrews1990; Stahl, Reference Stahl1996) does not support natural death or accidents due to roof spall. The anatomical representation of all Korth groups (Fig. 10) and the low incidence of rounding and polishing (Fig. 7) do not support water or wind transport.
Evidence of digestion observed in both cranial and postcranial remains of the small mammals at El Salt indicates (Fig. 5) the involvement of predators. Predation is the most likely agent involved in large accumulations of small mammal fossil assemblages from archaeological and paleontological sites (e.g., Andrews, Reference Andrews1990; Fernández-Jalvo and Andrews, Reference Fernández-Jalvo and Andrews1992; Fernández-Jalvo et al., Reference Fernández-Jalvo, Andrews, Denys, Sesé, Stoetzel, Marin-Monfort and Pesquero2016). The presence of digestive corrosion is the most distinctive trait observed on both modern and fossil assemblages (Mellet, Reference Mellett1974; Raczynski and Ruprecht, Reference Raczynski and Ruprecht1974; Mayhew, Reference Mayhew1977; Andrews, Reference Andrews1990; Fernández-Jalvo and Andrews, Reference Fernández-Jalvo and Andrews1992; Fernández-Jalvo et al., Reference Fernández-Jalvo, Andrews, Denys, Sesé, Stoetzel, Marin-Monfort and Pesquero2016).
The percentage of digested molars varies between 24.41% in Unit V and 32.72% in Unit X. Most incisors recovered in Unit V were digested (54 out of a total of 101), contrasting with only 11 digested incisors out of a total of 57 (19.3%) in Unit X, although results based on fewer than 100 specimens are less reliable (Fernández-Jalvo et al., Reference Fernández-Jalvo, Andrews, Denys, Sesé, Stoetzel, Marin-Monfort and Pesquero2016). Most of the altered specimens examined are only slightly to moderately digested, with heavily digested specimens being the exception. These values agree with Category 3 predators (11–22% for molars and 50–70% for incisors) in the classification of Andrews (Reference Andrews1990). The intensity of digestion (from light to extreme degrees) is consistent in both units, so the low percentage of digested incisors in Unit X is attributable to the small sample size.
The predators listed in Category 3 are considered to produce ‘intermediate modifications’ and include the spotted eagle owl (Bubo africanus), the European eagle owl (Bubo bubo), the tawny owl (Strix aluco), and the little owl (Athene noctua) (Andrews, Reference Andrews1990). Skeletal representation indices (Ri, Pc/C1, Pc/C2, and D/P indices) are representative of Categories 1 and 2 (Table 3), thus also suggesting the involvement of a nocturnal raptor.
Table 3. Categories assigned to each taphonomic variable analyzed for the different units of El Salt. Light gray refers to the anatomical representation; dark gray refers to breakage and black to digestion.

Breakage values (lack of complete skulls, abundant detached teeth, and high proportions of distal humeri and proximal femora) (Table 3) exceed those described by Andrews (Reference Andrews1990) in modern pellets of strigiforms, which are characterized by a higher percentage of complete elements. Therefore, breakage increased by post-predation processes, while exposed on surface by weathering or trampling, or when already buried by the action of plant roots or damp environments (indicated by manganese staining).
Category 3 avian predator species are considered opportunistic hunters that produce accumulations with a high specific diversity (Mikkola, Reference Mikkola1983; Andrews, Reference Andrews1990), as observed in previous taxonomic analyses at the El Salt Units X and V (Fagoaga et al., Reference Fagoaga, Ruiz-Sánchez, Laplana, Blain, Marquina, Marin-Monfort and Galván2018, Reference Fagoaga, Laplana, Marquina, Machado, Marin-Monfort, Crespo, Hernández, Mallol, Galván and Ruiz-Sánchez2019a). The presence of relatively large taxa (e.g., lagomorphs) also has been identified in Units X and V (Fagoaga et al., Reference Fagoaga, Ruiz-Sánchez, Laplana, Blain, Marquina, Marin-Monfort and Galván2018, Reference Fagoaga, Laplana, Marquina, Machado, Marin-Monfort, Crespo, Hernández, Mallol, Galván and Ruiz-Sánchez2019a). The abundance and intensity of digestion observed in small mammals, and recently confirmed in lagomorphs by Pérez (Reference Pérez2019), also corroborates the activity of a Category 3 predator. In a preliminary study of Subunit Xb by Fagoaga et al. (Reference Fagoaga, Ruiz-Sánchez, Laplana, Blain, Marquina, Marin-Monfort and Galván2018), digestion characteristics provided values slightly higher than those expected for Bubo bubo, which could suggest the additional intervention of most extreme members of this Category 3 (e.g., Strix aluco and Athene noctua). The taphonomic revision of Units X and V in this paper supports a Category 3 predator, better fitting with Bubo bubo, mainly based on the obtained digestion values here (molar digestion Category 3, Fernández-Jalvo et al., Reference Fernández-Jalvo, Andrews, Denys, Sesé, Stoetzel, Marin-Monfort and Pesquero2016). Other representatives of this category should have yielded more frequent and intense digestion in both rodents and lagomorphs (Andrews, Reference Andrews1990; Fernández-Jalvo et al., Reference Fernández-Jalvo, Andrews, Denys, Sesé, Stoetzel, Marin-Monfort and Pesquero2016). Moreover, preliminary studies of herpetofaunal remains also suggest the activity of owls (Marquina et al., Reference Marquina, Fagoaga, Crespo, Ruiz-Sanchez, Bailon, Hernandez and Galvan2017).
The implications derived from considering the European eagle owl (Bubo bubo) as the main agent responsible for the micromammal accumulation instead of the little owl (Athene noctua) are relevant. First of all, although both species are generalists and hunt what is available in the nearby ecosystem, the prey of the little owl is highly restricted by its own size, being incapable to capture a part of the spectrum of prey from the environment, such as rabbits, which are present at El Salt. The habitat preferences of Bubo bubo depend highly on the abundance and distribution of Oryctolagus cuniculus (Ortego and Díaz, Reference Ortego and Díaz2004), whereas Athene noctua is insectivorous and has little significance in the accumulation of small mammals (Andrews, Reference Andrews1990). Currently, the proportion of insects in detriment of mammals in the diet of Athene noctua increases from central Europe toward southern latitudes, and it is the highest in the Mediterranean region (Mikkola, Reference Mikkola1983). Murids are scarcely hunted by little owls, but they constitute one of the best-represented groups at El Salt (Fagoaga et al., Reference Fagoaga, Ruiz-Sánchez, Laplana, Blain, Marquina, Marin-Monfort and Galván2018, Reference Fagoaga, Laplana, Marquina, Machado, Marin-Monfort, Crespo, Hernández, Mallol, Galván and Ruiz-Sánchez2019a). In contrast, the European eagle owl is larger and hunts a huge variety of prey from a wide range of habitats. Therefore, prey assemblages accumulated by eagle owls appear to be accurate representations of the animals living in the local ecosystem (Andrews, Reference Andrews1990). In addition, the little owl is a crepuscular species that prefers flat open grasslands (Hůrka, Reference Hůrka1996) and avoids closed woodlands, high humidity, and mountainous environments (Blas and Muñoz, Reference Blas, Muñoz, Martí and Del Moral2007). Nevertheless, small mammals characteristic of open grasslands (e.g., soricids or talpids) are almost absent in the studied assemblages at El Salt. In fact, this habitat is certainly not the most represented by the Habitat Weighting Method when applied to Units X and V of El Salt; instead, it rather indicates a surrounding landscape (Fagoaga et al., Reference Fagoaga, Ruiz-Sánchez, Laplana, Blain, Marquina, Marin-Monfort and Galván2018) more in accordance to Bubo bubo's hunting area, which may extend from 4–20 km2 (in contrast to the more restricted hunting area of the little owl, 0.3 km2). Bubo bubo preferably nests in areas close to streams because these areas might supply a higher abundance of prey (Ortego and Díaz, Reference Ortego and Díaz2004), represented in the case of El Salt site by the proximity of the Serpis Valley (Fig. 11).

Figure 11. (color online) Minimum (4 km2) and maximum (20 km2) hunting area of Bubo bubo according to Cramp (Reference Cramp1985) and León-Ortega (Reference León-Ortega2016) around the El Salt site between the Serra de Mariola and Font Roja mountain ranges.
In summary, the results obtained in this work suggest a large owl species—more specifically, the European eagle owl—as the most likely predator responsible for the small mammal fossil accumulation in Units X and V. We have found no ground to consider any mixture with any other predator as described in other cases (e.g., Fernández-Jalvo, Reference Fernández-Jalvo1995; Fernández-García et al., Reference Fernández-García, López-García, Royer, Lécuyer, Allué, Burjachs, Chacón, Saladié, Vallverdú and Carbonell2020). Apart from the taphonomic traits, Bubo bubo is considered one of the main rabbit predators in the Iberian Peninsula and in Mediterranean ecosystems (Hiraldo et al., Reference Hiraldo, Andrada and Parreño1975; Donázar, Reference Donázar1988, Reference Donázar1989; Serrano, Reference Serrano1998; Rufà and Laroulandie, Reference Rufà and Laroulandie2019), which agrees with our results. Its current hunting area ranges between 4–20 km2, depending on the quality of territory and availability of prey (Cramp, Reference Cramp1985; León-Ortega, Reference León-Ortega2016). Due to its generalist feeding behavior, a solid paleoenvironmental reconstruction can be applied to its entire hunting area (Fig. 11) (Andrews, Reference Andrews1990; Fagoaga et al., Reference Fagoaga, Ruiz-Sánchez, Laplana, Blain, Marquina, Marin-Monfort and Galván2018, Reference Fagoaga, Laplana, Marquina, Machado, Marin-Monfort, Crespo, Hernández, Mallol, Galván and Ruiz-Sánchez2019a). Results obtained here highlight the need of doing complete taphonomic analyses in order to (1) identify the predator responsible of the accumulation, (2) know how representative of its paleoenvironment is the recorded prey, and (3) understand to what extent paleoecological inferences can be made.
Post-depositional modifications
Several post-depositional modifications are similar in the two studied units of El Salt, with only quantitative differences. Weathering, abrasion, and burning have only been identified in Subunit Xb, in negligible amounts. These data indicate a short pre-burial exposure (Behrensmeyer, Reference Behrensmeyer1978; Andrews, Reference Andrews1990) in both Units X and V. This is supported by the low incidence of abrasion (1.16%), which indicates a low influence of water/wind action/transport, as inferred from the analysis of Korth groups (Korth, Reference Korth1979) and from the absence of cracking associated with temperature/humidity changes.
Breakage, as mentioned above, exceeds the expected rates in both cranial and post-cranial elements (Fig. 4) caused by predation, as described by Andrews (Reference Andrews1990) for Category 3 predators, especially of large raptors such as the European eagle owl. Experimental trampling of pellets performed by Andrews (Reference Andrews1990, p. 10) resulted in complete and rapid disintegration of skulls, under-representation of jaws (leading to large numbers of isolated teeth), loss of teeth in jaws, and breakage and some degree of loss of larger post-cranial elements; but no loss or breakage of smaller and robust elements, namely astragali and calcanei.
Our results from El Salt specimens agree well with a trampling-derived breakage pattern. Trampling marks are absent at El Salt, but striations of this type are rarely recorded on small mammal bones because these are generally included in pellets and therefore protected from direct friction against the sediment (Andrews, Reference Andrews1990; Fernández-Jalvo and Avery, Reference Fernández-Jalvo and Avery2015). Breakage, therefore, might be due to trampling of already formed pellets exposed on the ground. Trampling could be caused by animals entering and temporarily inhabiting the cave, including humans, but also owls, who usually build their nests with pellets (Mikkola, Reference Mikkola1983; Andrews, Reference Andrews1990; Williams, Reference Williams2001).
Roof spall from the back wall of the site may have produced additional breakage. Breakage rates are particularly high in Unit V, where more abundant clasts and higher sedimentation rates have been documented (Galván et al., Reference Galván, Hernández, Mallol, Mercier, Sistiaga and Soler2014a). Exposure to high temperatures can modify the chemical properties and the structural integrity of bone, resulting in fractures (Shipman et al., Reference Shipman, Foster and Schoeninger1984, Stiner et al, Reference Stiner, Kuhn, Weiner and Bar-Yosef1995) and fragmentation (Koch and Lambert, Reference Koch and Lambert2017). Nevertheless, only a few burnt fossils have been recovered in Unit X. Finally, sullegic and trephic factors related to recent recovery processes (e.g., excavation, sieving, and sorting), potentially increase percentages of breakage.
Manganese coatings, present in both Units V and X, are often interbedded with travertine (Roy, Reference Roy1992). Mineral deposits can be explained by the weathering of limestones and travertine above the site, and the subsequent filtration/leaching of carbonate- and manganese-rich waters, eventually impregnating the bones. In Unit X, most fossils were almost entirely covered by mineral coatings (25–50%), but this was relatively rare (0–10%) in Unit V (Table 3). Such a difference may indicate a relatively more humid environment in Unit X than in Unit V.
Overall, a similar pattern can be inferred from our cementation (carbonate deposits) data, which show a somewhat higher incidence in Unit X. In fact, Unit V shows a similar percentage of carbonate deposition, but this is of lesser significance considering the surface affected (<25% Category 2), versus more than the half of the fossil surface cemented (Category 4) in Unit X (Fig.7). This again supports a more humid fossiliferous environment in Unit X.
Perforations and sinuous grooves associated with plant roots revealed a similar pattern, coherent with that inferred from mineral precipitates. In Unit X, most fossils are included in Categories 1–3 (25–75% of the surface affected by roots), but in Unit V, almost no surfaces were modified by root activity. None of the scarce, burnt fossils shows root-marks, which might be attributed to the low number of specimens or to chemical changes caused by the burning process that remove organic component (nutrients), thus rendering bone unprofitable to plants (Cáceres et al., Reference Cáceres, Bennàsar, Huguet, Rosell, Saladié, Allué, Solé and Carbonell i Roura2012).
Paleoenvironmental interpretation of El Salt and site formation, based on taphonomic information
Manganese and carbonate deposits, together with root marks, are related to water availability (Fernández-Jalvo and Andrews, Reference Fernández-Jalvo and Andrews2016 and references therein). The study of these variables at El Salt indicates an increase in aridification from base to top of the sequence (Table 3). Higher percentages of manganese, carbonate deposits, and evidence of plant root activity in Unit X suggest more-humid conditions (damper substrates/water dropping related to seasonal rains) at the base of the sequence than at its top, thus suggesting some aridification. The taphonomic inferences obtained from fossil bone surface modifications agree with the paleoecological interpretations based on taxonomy (Fagoaga et al., Reference Fagoaga, Ruiz-Sánchez, Laplana, Blain, Marquina, Marin-Monfort and Galván2018, Reference Fagoaga, Laplana, Marquina, Machado, Marin-Monfort, Crespo, Hernández, Mallol, Galván and Ruiz-Sánchez2019a), which supports the absence of mixtures due to resedimentation or reworking.
An abrupt sedimentary change, consisting of massive to diffusely bedded, well-sorted, fresh, calcitic, fine- and very fine-grained, organic-poor sand towards the top of Unit V, also suggests a strong aridification episode where micromorphology, lipid analysis, and magnetic susceptibility show a marked difference between Unit V and the rest of the underlying sequence (Galván et al., Reference Galván, Hernández, Mallol, Mercier, Sistiaga and Soler2014a).
The general scenario that can be interpreted from our taphonomic study indicates persistent occupation of eagle owls, which could have nested on the site floor or in a cliff salient above it. Fossils of lagomorphs, birds, and tortoises at the site show cut marks and breakage features linked to human activity (Pérez, Reference Pérez2019). Considering the human presence in the site, we could speculate that some rodents incidentally could have been part of the human diet at the site, together with lagomorphs, birds and tortoises. However, despite their occurrence in human occupation levels and some human-induced modifications, the general digestion characteristics observed in small mammal fossils do not fit with human digestion (Category 4 or 5, Crandall and Stahl, Reference Crandall and Stahl1995), but rather with a Category 3 predator, most probably Bubo bubo. The presence of burnt small mammal fossils in Unit X suggests that humans possibly shared the site with the raptors (interestingly, eagle owls are not as territorial as other birds, such as tawny owls; Mikkola, Reference Mikkola1983). Burning could have been intentional for food or waste treatment, or through accidental burning of owl pellets lying on the ground when the hearths were made. Both an alternative and a simultaneous occupation by humans and raptors could have been possible.
CONCLUSIONS
This work has greatly improved the reinterpretation of the taphonomic source of small mammals and provides a much more comprehensive and detailed interpretation of the surrounding paleoecosystem in El Salt. This study indicates that the predator responsible for the small mammal accumulations in Units X and V of El Salt was Bubo bubo, and revises a preliminary analysis that wrongly attributed the fossil assemblage in Subunit Xb to Athene noctua (Fagoaga et al., Reference Fagoaga, Ruiz-Sánchez, Laplana, Blain, Marquina, Marin-Monfort and Galván2018).
This reassessment improves the paleoenvironmental interpretation because Bubo bubo covers a widerer variety of habitats and prey than Athene noctua, and gives additional information on the surrounding habitat and the landscape of the El Salt site. Paleoenvironmental and paleoclimatic approaches obtained from their prey should be considered as representative of the past ecosystems around the El Salt site with a relatively better representation of closed woodland and high humidity areas preferred by Bubo bubo (and avoided by Athene noctua). Bubo bubo is an opportunistic predator, forming prey assemblages that are an accurate representation of the animals living in the habitat.
The European eagle owl, one of the largest strigiforms, tends to ingest prey as a whole, producing less breakage than that observed at El Salt. The excess of fragmentation observed in the small mammal fossils in El Salt fits well with a trampling-derived breakage pattern.
The rare proportion of abraded, weathered small mammal fossils and the simultaneous presence of all Korth groups suggest that the remains were not transported from close proximity to the site. The manganese coatings and the evidence of activity of root plants reveal that these post-depositional processes probably took place under humid conditions with abundant vegetation coverage at the bottom of the sequence (Unit X), which became more arid towards the top of the sequence (Unit V).
No evidence of reworking has been detected, and it is therefore assumed that the original bone assemblages do not include significant space or time mixtures in the site. The material from El Salt can be now considered as a reliable base for paleoecological interpretations. Paleoclimatic and paleoenvironmental results derived from this taphonomic analysis support previous paleoecological interpretations that point to an increase in aridity, which may have been a possibly influential factor for the regional disappearance of Neanderthals.
Supplementary Material
To view the supplementary material for this article, please visit https://doi.org/10.1017/qua.2021.4.
Acknowledgments
We would like to thank the whole team of El Salt for their effort and dedication during the field excavations and laboratory tasks. We also thank the Alcoi Archaeological Museum and the Town Hall of Alcoi for their support. Finally, we are grateful to Christiane Denys and an anonymous reviewer for their time and effort, whiche greatly improved the original paper with comments and suggestions.
Financial Support
This investigation has been carried out thanks to the Spanish Government project HAR2015-68321-P: “Neandertales en la montaña alicantina. Un enfoque multianalítico” (2016–2019) MICINN-FEDER, and the project UV-INV_AE17-708551 (University of Valencia), Conselleria d'Educació, Investigació, Cultura i Esport from the Valencian Government and the University of La Laguna (Tenerife, Spain).