INTRODUCTION
The radiocarbon (14C) reservoir age offset (R) of an organism is the difference between its 14C age and that of the atmospheric CO2 at the time this organism was alive (Stuiver and Polach Reference Stuiver and Polach1977; Ascough et al. Reference Ascough, Cook and Dugmore2005; Jull et al. Reference Jull, Burr and Hodgins2013; Soulet et al. Reference Soulet, Skinner, Beaupré and Galy2016). 14C reservoir age offsets are in 14C years. Very importantly, any 14C age obtained from an organism that did not incorporate its carbon directly from the atmosphere must be corrected for a reservoir age offset to provide an accurate estimate of its calendar age using the atmospheric 14C calibration curve (Reimer et al. Reference Reimer, Bard, Bayliss, Beck, Blackwell, Bronk Ramsey, Buck, Cheng, Edwards, Friedrich, Grootes, Guilderson, Haflidason, Hajdas, Hatté, Heaton, Hoffmann, Hogg, Hughen, Kaiser, Kromer, Manning, Niu, Reimer, Richards, Scott, Southon, Staff, Turney and van der Plicht2013). These corrections are crucial as they can range from a few hundred to thousands of 14C years (Siani et al. Reference Siani, Paterne, Arnold, Bard, Métivier, Tisnerat and Bassinot2000, Reference Siani, Paterne, Michel, Sulpizio, Sbrana, Arnold and Haddad2001; Bondevik et al. Reference Bondevik, Mangerud, Birks, Gulliksen and Reimer2006; Kuzmin et al. Reference Kuzmin, Nevesskaya, Krivonogov and Burr2007; Soulet et al. Reference Soulet, Ménot, Garreta, Rostek, Zaragosi, Lericolais and Bard2011a).
Recent studies suggested that some geochemical characteristics of an organism could be related to its reservoir age offset. For instance, it has been shown that the 14C reservoir age offset of modern Baltic Sea Macoma bivalve shell was inversely related to its shell 87Sr/86Sr ratio (Lougheed et al. Reference Lougheed, van der Lubbe and Davies2016). Accurately determining the calendar age of 14C-dated archeological remains of human populations that draw their carbon from various sources as a result of mixed diet is challenging. For example, individuals from a population that mostly eats fish can have their stable carbon and nitrogen compositions impacted (Schoeninger et al. Reference Schoeninger, DeNiro and Tauber1983; Schoeninger and Deniro Reference Schoeninger and DeNiro1984) and even exhibit a reservoir age offset (Dewar and Pfeiffer Reference Dewar and Pfeiffer2010; Olsen et al. Reference Olsen, Heinemeier, Lübke, Lüth and Terberger2010; Ascough et al. Reference Ascough, Church, Cook, Dunbar, Gestsdóttir, McGovern, Dugmore, Fridriksson and Edwards2012; Wood et al. Reference Wood, Higham, Buzilhova, Suvorov, Heinemeier and Olsen2013). It has been shown from two archeological sites from the region of Lake Baikal that the 14C reservoir age offset of human bones was linearly correlated to their nitrogen isotopic composition (δ15N) (Schulting et al. Reference Schulting, Ramsey, Bazaliiskii, Goriunova and Weber2014), and for one site to both their δ15N and stable carbon isotopic compositions (δ13C) (Bronk Ramsey et al. Reference Bronk Ramsey, Schulting, Goriunova, Bazaliiskii and Weber2014; Schulting et al. Reference Schulting, Ramsey, Bazaliiskii, Goriunova and Weber2014). Another study showed that 14C reservoir age offset of human bones from a medieval Icelandic cemetery can be inferred using the nitrogen, carbon and sulfur isotopic compositions of the bones (Sayle et al. Reference Sayle, Hamilton, Gestsdóttir and Cook2016). These studies are fundamental in the field of geochronology because they showed that it is possible to untangle the very complicated reservoir age offset correction issue.
The Black Sea is currently connected to the global oceans solely via the narrow and shallow Bosporus Strait (Figure 1), leading to restricted water exchanges with the Mediterranean Sea and permanent stratification (Özsoy and Ünlüata Reference Özsoy and Ünlüata1997). During the last glacial lowstand and for much of the deglacial sea-level rise, the Mediterranean connection was closed and the Black Sea was a large lake (e.g., Ross et al. Reference Ross, Degens and MacIlvaine1970; Badertscher et al. Reference Badertscher, Fleitmann, Cheng, Edwards, Göktürk, Zumbühl, Leuenberger and Tüysüz2011). The understanding of the glacial-deglacial hydrologic changes of the Black Sea has been recently refined (e.g., Major et al. Reference Major, Goldstein, Ryan, Lericolais, Piotrowski and Hajdas2006; Bahr et al. Reference Bahr, Arz, Lamy and Wefer2006; Kwiecien et al. Reference Kwiecien, Arz, Lamy, Plessen, Bahr and Haug2009; Soulet et al. Reference Soulet, Ménot, Garreta, Rostek, Zaragosi, Lericolais and Bard2011a, Reference Soulet, Menot, Bayon, Rostek, Ponzevera, Toucanne, Lericolais and Bard2013), as well as of its Holocene evolution (e.g., van der Meer et al. Reference van der Meer, Sangiorgi, Baas, Brinkhuis, Sinninghe Damsté and Schouten2008; Giosan et al. Reference Giosan, Coolen, Kaplan, Constantinescu, Filip, Filipova-Marinova, Kettner and Thom2012; Coolen et al. Reference Coolen, Orsi, Balkema, Quince, Harris, Sylva, Filipova-Marinova and Giosan2013). However, a robust chronological framework for the Holocene Black Sea is still lacking, thereby limiting interpretation and still precluding an understanding of the sequence of events that led to the reconnection of the Black Sea to the Mediterranean Sea that occurred sometime during the early Holocene (Ryan et al. Reference Ryan, Pitman, Major, Shimkus, Moscalenko, Jones, Dimitrov, Gorür, Sakinç and Yüce1997; Aksu et al. Reference Aksu, Hiscott, Mudie, Rochon, Kaminski, Abrajano and Yaşar2002; Major et al. Reference Major, Goldstein, Ryan, Lericolais, Piotrowski and Hajdas2006; Giosan et al. Reference Giosan, Filip and Constatinescu2009; Soulet et al. Reference Soulet, Ménot, Lericolais and Bard2011b; Yanko-Hombach et al. Reference Yanko-Hombach, Mudie, Kadurin and Larchenkov2014). The uncertainty surrounding the timing of the reconnection—and by extension, the Black Sea chronological framework—is related to unconstrained 14C reservoir age offset correction. This weakness has long been recognized (Jones and Gagnon Reference Jones and Gagnon1994; Kwiecien et al. Reference Kwiecien, Arz, Lamy, Wulf, Bahr, Röhl and Haug2008; Giosan et al. Reference Giosan, Filip and Constatinescu2009; Soulet et al. Reference Soulet, Ménot, Garreta, Rostek, Zaragosi, Lericolais and Bard2011a) but it has never been fully solved.
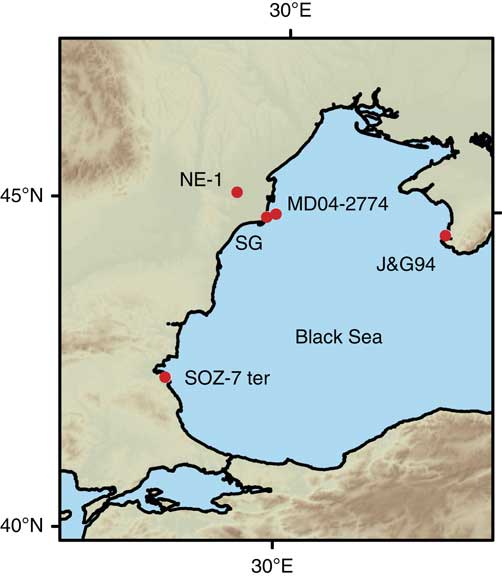
Figure 1 Western Black Sea area with sample locations and labels. (Coordinates are available in Supplementary material Table S1.)
Here, we reconstruct 14C reservoir age offsets of bivalve shells from the coastal Black Sea and relate them to their δ13C values, in an attempt to define 14C reservoir ages on the basis of the relationship, with the potential to considerably improve the chronological framework of the Black Sea sediment archives.
METHODS AND SAMPLES
Reconstructions of 14C reservoir age offsets typically rely upon 14C determinations on terrestrial/marine (or lacustrine) pairs or multiple pairs (Ascough et al. Reference Ascough, Cook and Dugmore2005; Jull et al. Reference Jull, Burr and Hodgins2013; Soulet Reference Soulet2015; Soulet et al. Reference Soulet, Skinner, Beaupré and Galy2016). From cores collected in the western coastal Black Sea (Figure 1), five single pairs of articulated bivalve shells and pieces of terrestrially derived plant material were sampled from the same sediment layers in order to ensure contemporaneous deposition, and their 14C ages and stable carbon isotope values were determined.
Radiocarbon and Stable Carbon Isotope Measurements
As a pre-cleaning, the bivalve shells were sonicated and rinsed in deionized water at least 10 times. Then the shell carbonate was converted into CO2 following National Ocean Sciences Accelerator Mass Spectrometry (NOSAMS) facility’s standard phosphoric acid hydrolysis procedure (McNichol et al. Reference McNichol, Osborne, Gagnon, Fry and Jones1994). The analyzed terrestrial plant materials were subjected to NOSAMS standard acid-base-acid pretreatment and converted to CO2 through the sealed tube combustion method (McNichol et al. Reference McNichol, Osborne, Gagnon, Fry and Jones1994). The CO2 was then converted to graphite and analyzed for its 14C composition by accelerator mass spectrometry (AMS) at NOSAMS. Two shell samples and two terrestrial plant material samples were prepared and graphitized at Centre de Datation par le RadioCarbone (CDRC, Lyon, France) following procedures similar to those performed at NOSAMS and measured by AMS at the Laboratoire de Mesure du Radiocarbone (LMC14-ARTEMIS, Saclay, France). Results are corrected for the 13C/12C ratio as measured on the AMS (Santos et al. Reference Santos, Southon, Griffin, Beaupre and Druffel2007) and are reported in Fm notation. Fm is identical to the ASN/AON metric (Stuiver and Polach Reference Stuiver and Polach1977), the 14aN notation (Mook and van der Plicht Reference Mook and van der Plicht1999), and to the F14C notation (Reimer et al. Reference Reimer, Brown and Reimer2004). Corresponding conventional 14C ages (ρ) reported in 14C years Before Present (AD 1950) were calculated according to:

The stable carbon isotope compositions of the dated samples were obtained from a 10%-aliquot taken out of the produced CO2, and measured using an Optima or VG Prism series II stable Isotope Ratio Mass Spectrometer (IRMS) at NOSAMS. 13C/12C ratios are reported in the δ13C notation (‰ relative to Vienna Pee Dee Belemnite, or VPDB).
Reservoir Age Offset Calculation
14C reservoir age offsets of the bivalve shells, relative to their contemporaneous atmosphere (Rshell-atm), were calculated based on the 14C ages of the paired bivalve shell (ρshell) and terrestrially-derived plant material (ρplant) (e.g., Ascough et al. Reference Ascough, Cook and Dugmore2005; Jull et al. Reference Jull, Burr and Hodgins2013; Soulet et al. Reference Soulet, Skinner, Beaupré and Galy2016):

The 14C ages of the terrestrially derived plant material are assumed to correspond to the 14C age of the atmosphere at the time the bivalves were living. Five Rshell-atm values were calculated according to this method, based on the following paired samples of Black Sea shells and terrestrially-derived plant materials found co-located in the same sediment layer:
i) A pair of an articulated juvenile Dreissena sp. bivalve and a sample of fragile foliar material, picked from a peat where the bivalve was found embedded and dated to 10,600–11,080 (95%) cal yr BP (core SG in the modern Danube delta). The bivalve, being a juvenile, was small and could have easily been transported onto the marsh surface where the peat was developing.
ii) A pair of a Monodacna caspia bivalve (single valve) and a fragile piece of Phragmites reed dated to 9040–9420 (95%) cal yr BP (core MD04-2774; offshore the modern Danube delta).
iii) Two paired samples of an articulated Mytilus galloprovincialis bivalve and charcoal from a ~15-cm-thick archeological layer recovered in several cores (SOZ-7 cores; Alepu lagoon, Bulgaria) (Flaux et al. Reference Flaux, Rouchet, Popova, Sternberg, Guibal, Talon, Baralis, Panayotova, Morhange and Riapov2016). The archeological site, dated to 5050–5300 (95%) cal yr BP (beginning of Early Bronze Age), was a pile-dwelling settlement recently discovered in the present Alepu lagoon (Flaux et al. Reference Flaux, Rouchet, Popova, Sternberg, Guibal, Talon, Baralis, Panayotova, Morhange and Riapov2016). The piles were 1 m long and 5–6 cm diameter and are made of oak. The bark was still in place showing that the wood was used directly after felling. The small diameter of the piles suggest that they were less than 40 years old at felling (Flaux et al. Reference Flaux, Rouchet, Popova, Sternberg, Guibal, Talon, Baralis, Panayotova, Morhange and Riapov2016). The piles indicate the beginning of the pile-dwelling occupation and were dated to 4550±30 14C yr BP. The charcoal pieces that we used to calculate the reservoir age offsets were found in the archeological layer of the site occupation that lasted less than 80 years (Flaux et al. Reference Flaux, Rouchet, Popova, Sternberg, Guibal, Talon, Baralis, Panayotova, Morhange and Riapov2016). The two charcoal samples used were dated to 4525±30 and 4475±30 14C yr BP. These 14C ages are not significantly different from those of the piles (Flaux et al. Reference Flaux, Rouchet, Popova, Sternberg, Guibal, Talon, Baralis, Panayotova, Morhange and Riapov2016). This suggests that the charcoals must originate from wood that lived during the occupation, and thus characterized by very little old wood effect, if any.
iv) A pair of an articulated Dreissena polymorpha bivalve and a sample of small fragments of twigs dated to 4540–4820 (95%) cal yr BP (core NE-1 in the inner Danube delta).
One additional Rshell-atm value was obtained from the calendar age of a mussel (Mytilus galloprovincialis) collected alive in 1931 (offshore of west Crimea) (Jones and Gagnon Reference Jones and Gagnon1994). In this case, the 14C composition of the atmosphere in 1931 was obtained from linear interpolation of the 14C calibration curve IntCal13 (Reimer et al. Reference Reimer, Bard, Bayliss, Beck, Blackwell, Bronk Ramsey, Buck, Cheng, Edwards, Friedrich, Grootes, Guilderson, Haflidason, Hajdas, Hatté, Heaton, Hoffmann, Hogg, Hughen, Kaiser, Kromer, Manning, Niu, Reimer, Richards, Scott, Southon, Staff, Turney and van der Plicht2013), then the Rshell-atm value was calculated according to the above equations (substituting the subscript “plant” by “IntCal13”). The 14C reservoir age offset of two bivalve shells collected alive during the 19th century in the Black Sea are reported in Siani et al. (Reference Siani, Paterne, Arnold, Bard, Métivier, Tisnerat and Bassinot2000) but stable carbon isotopes were not published, and thus cannot be included in this study.
All 14C and reservoir age offset data are compiled in the supplementary material (Table S1).
RESULTS AND DISCUSSION
Calculated Rshell-atm values ranged widely between 340 and 1100 14C years. This finding demonstrates that reservoir age offsets varied substantially in Black Sea coastal environments during the Holocene. The δ13Cshell values also ranged widely between –8 to +1‰ VPDB. We also found that the Rshell-atm values are linearly correlated to the δ13Cshell values (r2=0.87; p-value < 0.01; n=6) (Figure 2):


Figure 2 Linear regression of reservoir age offset (Rshell-atm) and δ13C of Black Sea bivalve shells and its 95%-confidence interval.
Biogeochemical Significance of the Rshell-atm-δ13Cshell Line
In order to understand the biogeochemical implications of the line, we explore the potential 14C-13C end-members of the coastal Black Sea. Here, we use the isotopic form of the reservoir age offset. This metric called the δ14R value (Soulet et al. Reference Soulet, Skinner, Beaupré and Galy2016) is calculated based on the exact same 14C measurements used to calculate Rshell-atm:

The link between δ14R and R is straightforward:

The δ14R-δ13C relationship (Figure 3) is also a line (r2=0.87; p-value < 0.01; n=6):


Figure 3 Linear regression of δ14Rshell-atm and δ13C of Black Sea bivalve shells (green squares; r2=0.87; p-value<0.01; n=6)—same samples as in Figure 2. Carbonate-equivalent endmembers of: i) CO2 atmosphere-water equilibration (blue rectangle), ii) CO2 from heterotrophic respiration of terrestrial organic matter supplied by rivers (yellow rectangle), iii) hard water effect from dissolution of outcropping carbonates by carbonic acid or sulfuric acid (red rectangles), and iv) CO2 from oxidation of methane (grey rectangle). Vertical dotted line is δ13C=0‰. (See online version for colors.)
Over the observed range of δ13C values (–8 to +1 ‰ VPDB), the difference in the Rshell-atm inferred from Eq. 3 or Eq. 6 is minimal (<10 14C years). Instead, for very negative δ13C values the difference in calculated Rshell-atm can be up to thousands of 14C years. This is because Rshell-atm is a logarithmic function of the Fm values. This is why we must use the δ14R-δ13C relationship to understand the biogeochemical processes explaining the line.
Bivalves form their carbonate shell primarily from the dissolved inorganic carbon (DIC) component of the water column, over several months to a few years as longevity of the bivalves studied here (Mytilus galloprovinciallis and Dreissena rostriformis) is ~10 years and probably less (Karatayev et al. Reference Karatayev, Burlakova and Padilla2006; Okaniwa et al. Reference Okaniwa, Miyaji, Sasaki and Tanabe2010). Thus, the shell isotopic composition reflects an integrated carbon isotopic composition of the DIC of the water in which they formed (Leng and Marshall Reference Leng and Marshall2004). The δ14Rshell-atm-δ13Cshell line (Eq. 6) indicates a 13C enrichment of the water DIC when the water DIC 14C composition becomes closer to that of the atmosphere (i.e., δ14Rshell-atm becomes closer to 0‰). In other words, the 14C composition of the water DIC becomes equilibrated with that of the atmosphere, when its stable isotope composition becomes enriched in 13C. The enrichment in 13C may also result in DIC stable carbon isotope composition equilibration with that of the atmosphere but with a fractionation in δ13C from atmospheric CO2 to bicarbonate of 7 to 8‰ (Mook et al. Reference Mook, Bommerson and Staverman1974; Romanek et al. Reference Romanek, Grossman and Morse1992).
The slope of the line suggests that the composition of the DIC of Black Sea coastal waters may have been driven mainly by the balance between autochthonous primary productivity and heterotrophic respiration of 14C-depleted (pre-aged) allochthonous terrestrial organic matter supplied by rivers. Indeed, the increased surface primary productivity during phytoplankton photosynthesis leads to 13C enrichment in the DIC (Hollander and McKenzie Reference Hollander and McKenzie1991; Leng and Marshall, Reference Leng and Marshall2004). This also leads to increased transfer of atmospheric CO2 to surface water via green algae and phytoplankton demand for CO2 (Deuser Reference Deuser1970; Hollander and McKenzie Reference Hollander and McKenzie1991; Li et al. Reference Li, Qiang, Jin, Liu, Zhou and Zhang2017), and may result in an equilibration of the surface water 14C composition with that of the atmosphere, meaning that δ14Rshell-atm tends towards 0‰. The latter is supported by 14C activity measurements of the DIC of Black Sea coastal waters in May 2004 showing that it had a similar 14C activity to that of the atmosphere on the day of sampling (Fontugne et al. Reference Fontugne, Guichard, Bentaleb, Strechie and Lericolais2009), with δ14R values of up to –4‰ (equivalent to a reservoir age offset of only ~30 14C years), i.e, very close to 0‰. It is also supported by the presence of bomb 14C in short chain saturated fatty acids and alkenones—both produced by phytoplankton—extracted from core top sediments collected in the early 2000s from the coastal Black Sea (Kusch et al. 2010, Reference Kusch, Rethemeyer, Hopmans, Wacker and Mollenhauer2016).
The primary productivity versus heterotrophic respiration balance hypothesis is further supported by the composition of the end-members defining the δ14Rshell-atm-δ13Cshell linear relationship. Indeed, assuming a complete productivity-driven equilibration of the surface water with the atmosphere, i.e., δ14Rshell-atm=0‰, the line predicts a δ13Cshell value of 6.0±1.9‰ VPDB for the carbonate phase (i.e., the mineral phase of the shell). At a range of temperatures typical for Black Sea surface waters, the δ13C of the carbonate phase is enriched by ~11–13‰ compared to that of the CO2 phase (Romanek et al. Reference Romanek, Grossman and Morse1992). Thus, the δ13C value of a carbonate derived from atmospheric CO2 with a pre-industrial δ13CO2 value of –6.4‰ with respect to VPDB (Schmitt et al. Reference Schmitt, Schneider, Elsig, Leuenberger, Lourantou, Chappellaz, Kohler, Joos, Stocker, Leuenberger and Fischer2012) would be of 4.5 to 6.5‰ VPDB. This range is in excellent agreement with the shell δ13C value of 6.0±1.9‰ VPDB, predicted by the line in the case of CO2 equilibration of the surface water with the atmosphere (Figure 3). In addition, in the case of no autochthonous productivity at all, the organic matter utilized during heterotrophic respiration would have δ13C values of –25 to –27‰ VPDB, typical for Black Sea terrestrial organic matter (Kusch et al. Reference Kusch, Rethemeyer, Schefuß and Mollenhauer2010). Carbonate δ13C originating from pure respiration of terrestrial organic matter would thus range around –12 to –16‰ VPDB (Romanek et al. Reference Romanek, Grossman and Morse1992), corresponding to predicted δ14Rshell-atm values of –150 to –180‰. These latter values are compatible with surface sediment δ14Rsed-atm of –200‰, calculated from total organic carbon 14C ages offshore of the Danube River (Kusch et al. Reference Kusch, Rethemeyer, Schefuß and Mollenhauer2010) (Figure 3). Mineralization of pre-aged terrestrial organic matter by microbial respiration occurs in temperate lakes and streams of Quebec (McCallister and del Giorgio Reference McCallister and del Giorgio2012). Our results suggest that pre-aged terrestrial organic matter as old as ~1500 14C years supplied by rivers is also converted to CO2 by heterotrophic bacteria in the Black Sea coastal waters.
A hard water effect (HWE) origin, i.e., reservoir age offset mainly explained by the contribution of 14C-depleted riverine DIC from the dissolution of outcropping carbonates, seems unlikely. Indeed, carbonate minerals can be weathered through two main pathways. The carbonic acid pathway:

This pathway involves CaCO3 and atmospheric CO2 with δ14R values of –1000 and 0‰ respectively, and δ13C values of ~0 and –6.5‰ VPDB (pre-industrial). Thus, the carbonate derived from the resulting DIC
$\left( {{\rm HCO}_{3}^{{\minus}} } \right)$
would have a δ13C value of –2 to 0‰ VPDB (Romanek et al. Reference Romanek, Grossman and Morse1992) and δ14RHWE-atm of –500‰ (Figure 3).
The second carbonate weathering pathway involves sulfuric acid, itself produced by the oxidation of sulfide minerals like pyrite (Calmels et al. Reference Calmels, Gaillardet, Brenot and France-Lanord2007):

In this case the carbonate derived from the resulting DIC would have a δ13C value of ~0‰ VPDB and δ14RHWE-atm would be of –1000‰. None of these endmembers fits the coastal Black Sea δ14R-δ13C line (Figure 3).
Oxidation of old methane could be another explanation, as it seems to impact the 14C composition of DIC of the present day Black Sea waters in the deeper part of the basin (slope and deep basin) (Fontugne et al. Reference Fontugne, Guichard, Bentaleb, Strechie and Lericolais2009). However, for very negative δ13C values typical of methane oxidation (e.g. −60‰; Kessler et al. Reference Kessler, Reeburgh, Southon, Seifert, Michaelis and Tyler2006), the extension of the line predicts a δ14Rshell-atm of –400 to –450‰, in disagreement with δ14R values for Black Sea methane being as low as –850‰ (Kessler et al. Reference Kessler, Reeburgh, Southon, Seifert, Michaelis and Tyler2006). Thus, oxidation of methane is unlikely to explain the reservoir age offset in the coastal settings of the western Black Sea.
Improving Black Sea Geochronology
The coastal Black Sea Rshell-atm-δ13Cshell line (Eq. 3; Figure 2) has fundamental implications for the Black Sea geochronology. It is now possible to use bivalve shell δ13C values as a proxy for reconstructing the reservoir age offset in the coastal Black Sea. Moreover, this δ13C-based tool can be applied on the same bivalve shell that is being 14C dated, leading to a customized reservoir age offset correction, which is crucial to providing an accurate estimate of the calendar age for the 14C-dated shell. Using this proxy is inexpensive since most 14C laboratories can provide the δ13C values of the dated material. One should note that the δ13C determination must be performed by IRMS on the CO2 produced from the dated material and reported vs. VPDB. The δ13C value measured on the AMS during 14C measurement should not be used because of potential large instrumental fractionation effects (Santos et al. Reference Santos, Southon, Griffin, Beaupre and Druffel2007).
At this stage of the research, i) any vital effect on the δ13C value seems of second order importance, but the use of the Rshell-atm-δ13Cshell line should be restricted to bivalve shells and for δ13C values of 2 to –10‰; ii) the application of the line should be restricted to the Holocene, in coastal to near coastal settings of the western Black Sea; iii) the line applies for both marine and lacustrine periods of the basin evolution—suggesting that the composition of the endmembers did not change much through the Holocene and that the pathway of terrestrial carbon mineralization (from Black Sea lacustrine or marine biotic communities) is also of second order importance.
CONCLUSION
Our results provide new pieces of information about carbon cycling in the coastal Black Sea. They suggest that the balance between primary productivity and heterotrophic respiration of terrestrial carbon supplied by rivers is governing the carbon isotopic composition of the Black Sea coastal waters since early Holocene. As a consequence, they provide an invaluable δ13C-based tool to quantify reservoir age offset for the Holocene Black Sea coastal settings. This will undoubtedly help refine our understanding of the hotly debated last reconnection of the Black Sea and more generally provide constrained calendar age-depth models to the numerous studied sediment archives of the Black Sea. Other basins, e.g., the Caspian Sea, the Aral Sea or large lakes, may potentially yield such correlation between the 14C reservoir age offset and δ13C value of shells and deserve future investigation. This study, in line with other pioneering studies (Bronk Ramsey et al. Reference Bronk Ramsey, Schulting, Goriunova, Bazaliiskii and Weber2014; Schulting et al. Reference Schulting, Ramsey, Bazaliiskii, Goriunova and Weber2014; Lougheed et al. Reference Lougheed, van der Lubbe and Davies2016), shows that correlations between the reservoir age offset and another geochemical parameter from the same material must be sought, with the aim of untangling the problem of reservoir age offset when deriving calendar age chronologies from non-atmospheric 14C measurements.
ACKNOWLEDGMENTS
G.S. acknowledges the Postdoctoral Scholar Program at the Woods Hole Oceanographic Institution (WHOI) with funding provided by the NOSAMS facility (OCE-1239667). He thanks the entire NOSAMS crew with special thanks to Ann McNichol and William Jenkins, as well as Mary Lardie, Liz Klein and Al Gagnon for assistance in the lab. L.G. thanks Florin Filip and Stefan Constantinescu for help in collecting Danube delta cores as well as WHOI for continuous support to his work in the Danube delta and the Black Sea. We thank Gilles Lericolais from Ifremer for providing access to core MD04-2774. This work is a contribution to the ASSEMBLAGE project funded by the European Commission (EVK3-CT-2002-00090).
SUPPLEMENTARY MATERIAL
To view supplementary material for this article, please visit https://doi.org/10.1017/RDC.2018.61