
Introduction
The origins of pottery production in the Americas are complex. Ceramic vessels were invented separately by hunter-fisher-gatherer communities of the lower Amazon Basin around 7500 years ago (Roosevelt 1995), in south-eastern North America around 4500 years ago (Sassaman Reference Sassaman1993), and in north-eastern North America slightly before 3000 years ago (Taché & Hart Reference Taché and Hart2013). Independently, the tradition of producing pots was introduced to Alaska around 2500 years ago from north-east Asia (Ackerman Reference Ackerman1982; Harry & Frink Reference Harry and Frink2009). From each of these centres pottery developed and spread, at different rates and with different levels of acceptance, to eventually become an important cultural artefact in many American prehistoric societies. Although these quite separate innovation and adoption processes occurred in a variety of different environmental settings (from rainforest to coastal arctic ecosystems), each marked a technological breakthrough that allowed hunter-gatherer groups to prepare food and other products in new ways. Pottery was a major innovation in container technology and remained, until the advent of metals and plastics, the most common storage and cooking device. However, little is known of what exact benefits were derived from the invention of pottery and why ceramic vessels remained marginal in archaeological assemblages for a long period following their initial adoption (Sassaman Reference Sassaman1993; Hayden Reference Hayden, Barnett and Hoopes1995; Heidke Reference Heidke1998; Rice Reference Rice1999; Taché Reference Taché2005).
Based on evidence from prehistoric Europe, the introduction of early pottery has long been linked with the Neolithic adoption and spread of agriculture (Childe Reference Childe1936). Cereal agriculture, in particular, is traditionally thought to have reduced mobility, thus permitting the manufacture of fragile and heavy vessels for increased food production. More recently, this view has been challenged by accumulating evidence in the Americas as in many other parts of the world, such as Africa, East and Inner Asia, and Northern Europe, where pottery is found much earlier than any evidence for farming (Jordan & Zvelebil Reference Jordan and Zvelebil2009; Wu et al. Reference Wu, Zhang, Goldberg, Cohen, Pan, Arpin and Bar-Yosef2012). The exact benefits that pottery use bestowed on largely mobile hunter-gatherer communities, and ultimately what drove its innovation, is a major and unresolved question. The transition towards broad-spectrum collecting strategies and an increased reliance on specific kinds of wild foodstuffs more amenable to intensive mass harvesting, processing and storage strategies have replaced the stimulus of agriculture in many culinary-based hypotheses for the innovation of pottery (Rice Reference Rice1999). Such scenarios are typically framed in terms of economic advantages, where pottery is seen as an adaptive means to enhance productivity by increasing efficiency in food preparation techniques (Saunders & Hays Reference Saunders, Hays, Saunders and Hays2004). In North America, early ceramic containers have tentatively been linked to the processing of inedible seeds and nuts, the rendering of high-energy oils from aquatic organisms, and the extraction of fat and grease from inedible parts of hunted game (Ozker Reference Ozker1982; Goodyear Reference Goodyear1988). Yet the production of these resources does not absolutely require pottery as they were all exploited in pre-ceramic contexts, or, more recently, by many aceramic hunter-gatherer groups. Alternative sociopolitical or ritual explanations have also been proposed for the innovation of ceramic vessels (Crown & Wills Reference Crown and Wills1995; Hayden Reference Hayden, Barnett and Hoopes1995; Heidke Reference Heidke1998). For example, pottery may initially have been used selectively to process high-prestige products or itself be regarded as a prestige technology. Similarly, new social contexts of consumption, such as seasonal gatherings of hunter-gatherer groups, might have triggered the innovation of pottery. However, with very little knowledge of vessel use and function, the various motivations for the origins of pottery production by North American hunter-gatherers cannot currently be assessed.
To address this issue directly in north-eastern North America, one of the major centres for ceramic innovation on the continent, we conducted organic residue analysis on 169 potsherds (representing approximately 133 distinct vessels) from 33 early pottery sites encompassing a range of environmental and cultural contexts (Figure 1A) using well- established methods (see Technical Note). Despite the possible chronological precedence of soapstone bowls, it is generally agreed that these vessels cannot be viewed as a technological precursor to ceramic technology in the temperate woodlands of north-eastern North America (Sassaman 1999), and that pottery was independently invented in this region slightly before 3000 years ago. This is about 1200 years before the first evidence for the arrival of maize cultigens and over 2000 years before maize became an important dietary staple (Hart et al. Reference Hart, Brumbach and Lusteck2007; Scarry Reference Scarry, Reitz, Scarry and Scudder2008). These coarse, cord-marked pots are referred to as Vinette 1 (Ritchie & MacNeish Reference Ritchie and MacNeish1949; Figures 1B & 2) and appear on a wide range of sites but only at low frequency. Indeed, aside from a handful of sites where Vinette 1 sherds are thought to represent 10 to 40 vessels, most components contain sherds from no more than five containers, contrasting with the abundance of pottery vessels in subsequent time periods (Taché Reference Taché2005). Vinette 1 pottery is archaeologically visible between c. 3100 and 2300 cal BP and, based on current dating evidence (Taché & Hart Reference Taché and Hart2013), chronological variation between sub-regions is imperceptible, suggesting a relatively rapid and extensive uptake of this new technology. The presence of sooting and carbonised deposits on the interior surface of many potsherds suggest that at least some Vinette 1 vessels were used to cook food over open fires, but as with other early pottery in America, the uses of these pots and the reasons for their appearance at this juncture in prehistory remain very poorly understood.

Figure 1. Location of sites sampled and illustration of Vinette 1 potsherds. A: 1) Bruce Boyd; 2) Peace Bridge; 3) Scaccia, 4) Vine Valley; 5) Dawson Creek; 6) Pointe-du-Buisson; 7) Batiscan; 8) Parc des Pins; 9) Lambert; 10) Gasser; 11) Carson Farm; 12) Fort Hunter; 13) McCormick Island; 14) Sheldon; 15) Wilson; 16) Barton Complex; 17) Zimmerman; 18) Minisink Island; 19) Drake; 20) Hormell; 21) Roberts; 22) Kirby Brooks; 23) Lovers Leap; 24) Hopkins; 25) Eddy; 26) Hoffman; 27) Morrill Point; 28) Hornblower Shellheap; 29) Small Swamp; 30) Rose; 31) RI1428; 32) Knox; 33) Great Diamond Island. B: Vinette 1 vessel from McCormick Island, Pennsylvania (image courtesy of the State Museum of Pennsylvania, Pennsylvania Historical and Museum Commission).
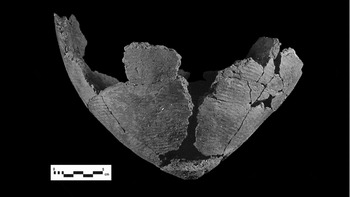
Figure 2. Vinette 1 vessel from the Peace Bridge site, Ontario (image courtesy of Archaeological Services Inc).
Results and discussion
Bulk carbon and nitrogen isotope analysis was conducted on 68 carbonised surface deposits (65 interior and 3 exterior deposits) that were adhering to 44 individual pots, in order to provide some crude indication of their use. Despite some variability, potsherds from coastal sites (n = 19) are significantly enriched in 13C and 15N compared with those from inland sites (n = 25; Figure 3A; Mann–Whitney U test;13C value: U = 1020, P = < 0.001; 15N value: U = 967, P = < 0.001), a distinction attributable to a greater incorporation of isotopically heavier marine foods (Craig et al. Reference Craig, Steeleb, Fischer, Hartz, Andersen, Donohoe, Glykou, Saul, Jones, Koch and Heron2011). The source contributions to the carbonised deposits from pots from inland sites is more difficult to determine based on bulk isotope characteristics alone, as freshwater fish and terrestrial foods are both depleted in 13C. The carbon isotope value is also almost certainly affected by differential preservation of different classes of biomolecules (Craig et al. Reference Craig, Forster, Andersen, Koch, Crombé, Milner, Stern, Bailey and Heron2007). Charred deposits from inland sites have δ15N values that fall within the range of experimentally charred foods from both terrestrial and aquatic animals (Figure 3A), possibly indicating a more complex mixture of sources. Notably, all Vinette 1 vessels have δ15N values well above the median for terrestrial animals and none are consistent with plant foods.

Figure 3. Bulk and single compound stable isotope data from Vinette 1 pottery from coastal (open circles) and inland/riverine sites (filled circles). A) Bulk stable carbon and nitrogen isotope of interior surface deposits. Exterior deposits (star symbols) and the median and ranges (2σ) of experimentally charred aquatic and terrestrial animals (Craig et al. Reference Craig, Saul, Lucquin, Nishida, Taché, Clarkes, Thompson, Altoft, Uchiyama, Ajimoto, Gibbs, Isaksson, Heron and Jordan2013) are also shown. B) Plot of the δ13C values of C 16: 0 and C 18: 0 fatty acids extracted from Vinette 1 potsherds. Samples that have aquatic biomarkers are shown in blue. Extracts from surface deposits from inland/riverine sites are represented by filled squares. These are compared with reference fats from wild ruminant (n = 19), freshwater (n = 24) and marine (n = 22) organisms (see online supplementary Table S2) plotted as confidence ellipses (1σ; Stastica v.7). Additional North American authentic fat samples from wild herbivorous, omnivorous and piscivorous animals (n = 8) are indicated separately (open diamonds).
To provide more specific information on pottery use, lipids were successfully extracted from 143 ceramic samples (130 interior and 13 exterior samples) and 10 carbonised surface deposits (8 interior and 2 exterior deposits) from 112 vessels (Table 1). Analysis by gas chromatography mass spectrometry (GC-MS) revealed the presence of lipid biomarkers derived from aquatic organisms in a large number of pots from both coastal and inland sites. Of the inland vessels, 40 per cent (n = 29; Table 1) are characterised by the presence of at least one of three isoprenoid alkanoic acids (phytanic, pristanic or 4,8,12-TMTD), which are at high concentration in freshwater and marine organisms, and by a range of positional isomers of ω-(o-alkylphenyl)alkanoic acids containing a minimum of 20 carbon atoms, with trace amount of the C22 homologue also detected in the majority of cases (Figure 4A). These suites of compounds can only be produced by the protracted heating of polyunsaturated fatty acids present in aquatic organisms (Hansel et al. Reference Hansel, Copley, Madurai and Evershed2004; Craig et al. Reference Craig, Forster, Andersen, Koch, Crombé, Milner, Stern, Bailey and Heron2007; Evershed et al. Reference Evershed, Copley, Dickson and Hansel2008).

Figure 4. Examples of partial gas chromatograms of lipid extracts from different Vinette 1 potsherds. Also shown is the number of potsherds exhibiting similar chromatograms. A) Degraded aquatic oil from a potsherd excavated at the Eddy site, New Hampshire (NH38.6-5). The partial m/z 105 ion chromatogram (inset) shows ω-(o-alkylphenyl)alkanoic acids with 16 (+), 18 (*), 20 (#) and 22 (o) carbon atoms; B) degraded terrestrial animal fat from a potsherd (PB03) excavated at the Peace Bridge site; C) degraded possible plant oil from a potsherd (BB09) excavated at the Bruce Boyd site. Cn:x are fatty acids with carbon length n and number of unsaturations x; DCx are α,ω-dicarboxylic acids with carbon length x; ALx are alkanes with carbon length x; br are branched-chain acids; Kn are long chain ketones with carbon length n; p are plasticiser contaminants; IS is internal standard (n-hexatriacontane).
Table 1. Summary of organic residue analysis results obtained from Vinette 1 pottery. Further details are available in online supplementary Table S1.

* > 5μg/g for ceramic samples, > 0.5μg/mg for carbonised deposits.
†Complete set of aquatic biomarkers defined by the presence of at least one of the three isoprenoid alkanoic acids (phytanic, pristanic or 4,8,12-TMTD) and ω-(o-alkylphenyl)alkanoic acids containing a minimum of 20 carbon atoms. Partial set of aquatic biomarkers defined by the presence of either one of the three isoprenoid alkanoic acids (phytanic, pristanic or 4,8,12-TMTD) or ω-(o-alkylphenyl)alkanoic acids containing a minimum of 20 carbon atoms.
A significant proportion of pots from coastal sites (53 per cent, n = 21) also contained similar arrays of biomarkers for aquatic foods (Table 1). These proportions represent only the minimum values as an absence of aquatic biomarkers does not mean that aquatic resources were not processed in pots. Firstly, the highly diagnostic ω-(o-alkylphenyl)alkanoic acids are only formed from parent polyunsaturated fatty acids under specific conditions, i.e. high temperature ( > 270 °C; Hansel et al. Reference Hansel, Copley, Madurai and Evershed2004), which are often difficult to replicate experimentally. Second, both these compounds and other diagnostic lipid components may have been lost through exposure to the burial environment. When considering Vinette 1 samples that yielded either isoprenoid or ω-(o-alkylphenyl)alkanoic acids containing a minimum of 20 carbon atoms, in addition to medium to long-chain saturated (C14–C24) and mono-unsaturated (C16: 1–C22: 1) fatty acids, which are also common to many aquatic oils, the proportion of vessels implicated in the processing of aquatic resources rises to 63 per cent (n = 45) from inland sites and 75 per cent (n = 30) from coastal sites. This is still a minimum estimate as a large number of samples are characterised by lipid profiles that could not be assigned to any food class due to extensive degradation. Overall, these data provide compelling evidence that Vinette 1 pottery vessels were principally used to process marine and freshwater faunal resources, such as fish, invertebrates or mammals.
Finally, GC-combustion-isotope ratio MS (GC-C-IRMS) analysis was carried out on 60 samples representing 52 individual vessels to investigate further the source of lipids recovered from the assemblage (Figure 2 B). By comparing the δ13C values of the most abundant n-alkanoic acids preserved in potsherds, i.e. octadecanoic (C18: 0) and hexadecanoic (C16: 0) acid, with corresponding values from authentic reference fats and oils, more specific identifications can be achieved (Dudd et al. Reference Dudd, Evershed and Gibson1999; Craig et al. Reference Craig, Allen, Thompson, Stevens, Steele and Heron2012, Reference Craig, Saul, Lucquin, Nishida, Taché, Clarkes, Thompson, Altoft, Uchiyama, Ajimoto, Gibbs, Isaksson, Heron and Jordan2013). Notably, reference fats from marine fish and marine mammals are consistently enriched in 13C compared with those from freshwater and terrestrial organisms (Figure 3B; see also online supplementary Table S2). Additionally, C18: 0 acids in ruminant animals are generally depleted in 13C by c. 1–6‰ compared with C16: 0 and, with the exception of Rangifer tarandus (caribou), which feeds partially on 13C-enriched lichens, the values are tightly constrained (Figure 3B). The carbon isotopic composition of fats from non-ruminant animals and freshwater fish are harder to discriminate. This is mainly because freshwater fish, which include diadromous and estuarine species, and animals that feed on freshwater organisms exhibit a broad range of values (Figure 3B), reflecting environmental variation in primary sources of carbon.
The δ13C values of individual fatty acids from Vinette 1 vessels from coastal sites (n = 15) are significantly enriched in 13C compared with those from inland sites (n = 45; e.g. for C16: 0, Mann-Whitney U test; U = 98; P = < 0.001). This result is consistent with the preferential cooking of marine foods in pottery from coastal settings. Interestingly, three samples from inland riverine locations, all with aquatic biomarkers, have isotope values within the range (1σ) for marine fish. A likely explanation is that diadromous fish, such as shad, alewives, salmonids or eels, were processed in these vessels. A further three samples from coastal sites without any aquatic biomarker could be assigned to a marine origin based on their isotope characteristics. Overall, the fatty acid isotope data confirms that the vast majority of samples submitted for analysis are consistent with a marine or freshwater origin. In contrast, the evidence for ruminant lipids in Vinette 1 pottery is surprisingly limited considering the high abundance of deer and elk in temperate woodland environments. Two vessels, one each from Peace Bridge and Pointe-du-Buisson, have fatty acid δ13C values comparable with wild ruminant fats, although the identification of aquatic biomarkers in both of them suggests that fish and terrestrial meat were mixed or processed sequentially in these vessels. Of the entire sample of Vinette 1 pottery analysed, only seven vessels have lipid profiles characterised by an absence of aquatic biomarkers, low C16: 0/C18: 0 ratios ( < 1), short-chain fatty acids, and odd or branched-chain fatty acids, which are typical of animal fats (Figure 4B). Similarly, lipid profiles typical of plant oils characterised by high C16: 0/C18: 0 ratios (i.e. > 2), abundance of C12: 0 and C14: 0 fatty acids, and n-alkanes in the carbon-chain range C20: 0 to C35: 0 (Figure 4C), were only observed in a small number (n = 16) of the vessels analysed. Interestingly, a significant proportion of these come from just two sites, Dawson Creek and Scaccia. Other than these observations, there were no apparent regional differences in the use of pots. By comparing the residue analysis data with a recently compiled inventory of AMS dates (Taché & Hart Reference Taché and Hart2013), there was also no evidence of any chronological patterning in the use of Vinette 1 pottery. Vessels from across the study area were mainly associated with freshwater or marine resources.
The preference for the processing of aquatic resources in the earliest pottery from north-eastern North America is not a simple reflection of the wider subsistence strategy at the time. From the analysis of macroscopic food remains from these sites, it is clear that subsistence practices varied widely, both seasonally and geographically. Where comparable faunal data exists, marine fish appear to have been heavily exploited at coastal sites, particularly during the spring and summer (Belcher Reference Belcher1989). However, significant proportions of large and small terrestrial mammals are also present and these coastal groups are most accurately described as broad-spectrum opportunistic hunter-gatherers (Bunker 2006–Reference Craig, Forster, Andersen, Koch, Crombé, Milner, Stern, Bailey and Heron2007). At inland and riverine sites, terrestrial mammals, notably the whitetail deer (Odocoileus virginianus), consistently dominate faunal assemblages. For example, at the Riverhaven 2 site in the Niagara Peninsula, terrestrial mammals make up 82 per cent of the faunal assemblage, 38 per cent of the number of individuals identified, and 96 per cent of usable meat by weight (Granger Reference Granger1978). Of this, over half the meat was derived from whitetail deer despite the proximity of the site to productive fishing grounds and the good potential for the preservation of faunal remains. The fact that pottery was mainly used to process aquatic resources, most likely freshwater fish, is therefore perplexing given that deer fat must have been very abundant and is easily distinguishable through lipid residue analysis (Craig et al. Reference Craig, Allen, Thompson, Stevens, Steele and Heron2012).
Similarly, it is very unlikely that the fruits of forest trees, such as acorns or nuts, were significantly processed in early pottery from this region, despite the fact that such commodities were readily available and have been closely linked with the appearance of pottery in the Great Lakes region (Ozker Reference Ozker1982). The analysis of modern oil from several species of authentic wild mast (Juglans cinerea, Juglans nigra, Quercus alba, Quercus macrocarpa, Fagus grandifolia, Carya ovate, Corylus cornuta; n = 8) by GC-MS produced very low relative abundances of C18: 0 fatty acid (C16: 0/C18: 0 = 4.5, ± 3.9 (s.d.); C18: 0/total fatty acid = 0.03, ± 0.01 (s.d.)), whereas this compound was relatively abundant in a majority of archaeological pottery extracts (Figure 4A & 4B; online supplementary Table S1). The long-term exposure of nut oils to the burial environment would be expected to reduce the abundance of C16: 0 relative to C18: 0 through preferential dissolution, but this would also result in the almost complete loss of the even shorter chain acids that are observed in many of the samples (supplementary Table S1). The relatively high δ15N values observed from bulk isotope analysis of Vinette 1 food crust deposits are also inconsistent with charred plant material. Therefore, except at one or two sites, our analysis rules out the processing and storage of nut resources as the main use of early pottery in north-eastern North America. This mismatch between the evidence from the analysis of food remains and organic residue data, even accounting for differential preservation, implies that early ceramic containers were used selectively to process aquatic resources rather than opportunistically to process anything and everything that was available in the environment. The small number of Vinette 1 vessels at any one site and the absence of a significant shift in environmental conditions or increased abundance of a particular faunal species in archaeological deposits also suggest that the initial production and use of ceramic containers were not solely linked to some functional demand for new resources or intensified exploitation of existing resources. A more likely explanation is that new social contexts of consumption triggered pottery innovation.
In north-eastern North America the innovation of pottery co-occurred with key social developments such as the increased regionalisation and complexity of hunter-gatherer groups (Versaggi Reference Versaggi1999), the emergence of new elaborate mortuary practices (Farnsworth & Emerson Reference Farnsworth and Emerson1986; Heckenberger et al. Reference Heckenberger, Petersen, Basa, Cowie, Spiess and Stuckenrath1990), and the creation of long-distance interaction networks that ensured the circulation of prestige items (Taché Reference Taché2011). Archaeologically, this is recognised by increased evidence for surplus accumulation (e.g. storage features) and social inequalities (e.g. differential distribution of grave goods), the advent of burial precincts distinct from habitation sites, complex burial practices shared between distinct groups (e.g. cremations, intentional destruction and burning of grave goods, abundance of red ochre in burials), and by the widespread distribution of stylistically and technologically homogeneous artefacts (e.g. Onondaga chert bifaces, ground slate objects) and exotic raw materials (e.g. native copper, marine shells). The social context for such long-distance interaction typically takes place at large, episodic and multi-ethnic gathering sites. So-called ‘trade fairs’ have been ethnographically documented among a diverse range of hunter-fisher-gatherer groups not only in north-eastern North America (Bouchard Reference Bouchard2002) but also in regions as diverse as Alaska, Australia and the American Northwest (Jackson Reference Jackson and Gregg1991). These large social gatherings generally occurred when concentrations of aquatic resources peaked and when surpluses could be produced for exchange.
We suggest that many of the early pottery sites in north-eastern North America were important focal points for such large seasonal gatherings. Now, based on the new molecular evidence, it seems that the invention and widespread uptake of pottery throughout north-eastern North America was associated with the preparation of aquatic resources within this context. Seasonal peaks in either the abundance of migratory fish or marine and freshwater species during spawning are likely to have attracted multiple local bands and promoted cooperative exploitation. Intriguingly however, the relatively small size and low abundance of vessels at these sites precludes a major economic role for pottery. Instead, pottery may have been used to prepare fish as part of small-scale celebratory feasts. The act of cooking and consuming fish with novel ceramic containers would have been largely symbolic, serving to cement social relations during these important periods of aggregation. Therefore, we would expect the bulk of aquatic resources harvested during these gatherings to be processed and consumed using other means.
A related interpretation is that fish oil, a valued exchange commodity among native populations in historical times (Martin Reference Martin1999), was produced during these episodic gatherings and that ceramic containers were used for its preparation. An interesting comparison can be done with eulachon oil (Thaleichthys pacificus), a major source of fat for prehistoric and early historic native communities of coastal British Columbia (Kuhnlein et al. Reference Kuhnlein, Chan, Thompson and Nakai1982). After harvest, the process of rendering oil begins with the ageing of this anadromous fish for one to two weeks in large bins or pits. The ripened fish are then placed into boiling water in a large vat set on a platform with a fire built beneath. The fish are boiled, mashed into small pieces, reboiled and left to simmer for 5 to 12 hours. Eventually, the layer of clear oil is skimmed from the surface to smaller pots and further refined by direct heating to facilitate its long-term storage. If valuable fish oil was processed in a similar manner by early pottery-using communities in north-eastern North America, Vinette 1 pots would have been useful containers for this last processing stage. And as large quantities of fish yield limited amounts of oil, only a few ceramic containers would have been required. The production of fish oil either for exchange with more distant communities, or as a highly valued prestige food for conspicuous consumption, would also reinforce social relations during periodic aggregation of scattered hunter-gatherer groups, in keeping with the ‘cooperative harvesting’ hypothesis.
Conclusion
Based on the presence of lipids derived from aquatic organisms in a large number of pots from an extensive area of north-eastern North America, we suggest that pottery initially developed in this region to process freshwater and marine organisms at episodic social gatherings during periods of high resource abundance. Interestingly, comparing other cases of early pre-agricultural pottery use points to several converging factors that may highlight a common purpose for the independent invention and development of pottery in different parts of the world. Lipids from marine and freshwater resources have been identified in other studies of pottery use by temperate Holocene and Palaeolithic hunter-gatherer societies at water-edge environments. These include the Ertebølle of Northern Europe c. 7.5–6 k BP (Craig et al. Reference Craig, Steeleb, Fischer, Hartz, Andersen, Donohoe, Glykou, Saul, Jones, Koch and Heron2011) and the Jōmon of Japan c. 15.5–11.5 k BP (Craig et al. Reference Craig, Saul, Lucquin, Nishida, Taché, Clarkes, Thompson, Altoft, Uchiyama, Ajimoto, Gibbs, Isaksson, Heron and Jordan2013). In each of these cases, seasonally abundant aquatic resources may have drawn communities together, encouraged investment in the production of pottery and promoted the articulation of new kinds of social relations. Surpluses derived from these rich aquatic ecotones may have facilitated a reduction in mobility, contributing to increased social complexity and also resulting in population increases. Similarly, such environments may have facilitated the widespread uptake of pottery technologies outside the early centres of innovation, as has been suggested for the dispersal of pottery westwards across northern Eurasia (Jordan & Zvelebil Reference Jordan and Zvelebil2009). If these predictions are correct, a close association between aquatic resources and the emergence of pottery in early Holocene Eurasian and African hunter-gatherer societies should be observable through the widespread application of organic residue analysis.
Acknowledgements
We thank A. Gledhill and A. Thompson for assistance with bulk IRMS analysis and GC-C-IRMS analysis, respectively; A. Lucquin for assistance in the analysis of modern nut samples; and E. Reber for conducting lipid analysis in a pilot experiment. The following provided pottery samples: Museum of Ontario Archaeology, University of Western Ontario, Archaeological Services Inc., Pointe-du-Buisson Museum, Ministère de la culture du Québec, Mashantucket Museum, Robert S. Peabody Museum, Delaware Water Gap National Recreation Area, State Museum of Pennsylvania, Towson University, Institute for American Indian Studies, New Hampshire Division of Historical Resources, University of Southern Maine Gorham, University of Maine Orono, New York State Museum and Université de Montréal. The Ministère de l’Energie et des Resources naturelles du Québec, the Club des producteurs de noix comestibles du Québec and several anonymous individuals provided modern reference samples. This work was supported by a Marie Curie Incoming International Fellowship (grant # 273392) and the Social Sciences and Humanities Council of Canada (grant # 756-2011-0321).
Technical Note: Methods
For bulk isotope ratio mass spectrometry (EA-IRMS), crushed surface residues ( ≈ 1mg) were analysed exactly as previously reported (Craig et al. Reference Craig, Forster, Andersen, Koch, Crombé, Milner, Stern, Bailey and Heron2007). Samples yielding less than 1% N were discarded and instrument precision on repeated measurements was ± 0.2‰ (s.e.m.). The standard for δ13C was Vienna PeeDee Belemnite (V-PDB) and the standard for δ15N is air N2. Lipids were extracted and analysed by gas chromatography-mass spectrometry (GC-MS) and GC-C-IRMS using well-established protocols (Craig et al. Reference Craig, Forster, Andersen, Koch, Crombé, Milner, Stern, Bailey and Heron2007, Reference Craig, Allen, Thompson, Stevens, Steele and Heron2012, Reference Craig, Saul, Lucquin, Nishida, Taché, Clarkes, Thompson, Altoft, Uchiyama, Ajimoto, Gibbs, Isaksson, Heron and Jordan2013). For food crusts, each sample ( ≈ 10–20mg scraped from the potsherd surface) was solvent-extracted by ultrasonication with dichloromethane:methanol (2:1 vol/vol; 3 × 2mL, 15min). The solvent was removed from the food crust and evaporated under a gentle stream of N2 to obtain the total lipid extract (TLE). An aliquot of each TLE was silylated with BSTFA, dissolved in hexane and analysed by GC-MS. Fatty acid methyl esters (FAMEs) were prepared from another aliquot of the TLE through treatment with BF3-Methanol complex (14% w/v; 70°C, 1h). FAMEs were extracted with hexane (3 × 1mL) and analysed by GC-MS and by GC-combustion-isotope ratio MS (GC-C-IRMS).
Ceramic samples ( ≈ 1–2g drilled from the potsherd interior surface) were weighed and extracted as described above or by direct methylation with acidified methanol. For the latter, methanol (4mL) was added and homogenised with the ceramic powder, the mixture was ultrasonicated for 15min and then acidified with concentrated sulphuric acid (800μL). The acidified suspension was heated in sealed tubes for four hours at 70°C and then cooled, and lipids were extracted with n-hexane (3 × 2mL) and analysed by GC-MS and GC-C-IRMS. Finally, modern samples were extracted using either a modified Bligh and Dyer extraction (Craig et al. Reference Craig, Allen, Thompson, Stevens, Steele and Heron2012) or directly by methanolic acid extraction, as described above.
Instruments and instrument conditions for GC-MS and GC-C-IRMS were exactly as previously reported (Craig et al. Reference Craig, Allen, Thompson, Stevens, Steele and Heron2012) except that to improve separation, several samples were re-analysed by GC-MS using a VF3-23ms (J&W Scientific), high-cyanopropyl modified methylpolysiloxane capillary column (60m × 0.32mm i.d.). For GC-C-IRMS, instrument precision on repeated measurements was ± 0.3‰ (s.e.m.) and the accuracy determined from FAME and n-alkane isotope standards was ± 0.5‰ (s.e.m.). Methanolic acid extraction and solvent extraction were compared by extracting four Vinette 1 ceramic samples using both protocols. Similar δ13C values for the C16 and C18 n-alkanoic acids were obtained from these extracts (mean difference = 0.3‰ and 0.5‰ respectively). As previously reported (Correa-Ascencio & Evershed Reference Correa-Ascencio and Evershed2014), the small differences in δ13C fatty acid values observed between extraction protocols did not change the interpretation and were not systematic.