Introduction
Pestiviruses of the family Flaviviridae are small enveloped RNA viruses that have substantial impact on the livestock industries. Pestivirus isolates are genetically and antigenically related, which in the past has provided difficulties with classification. Traditional classification of viral isolates according to mammalian host of origin may not always be accurate, as pestiviruses do not demonstrate strict host specificity (Nettleton, Reference Nettleton1990). More recent molecular techniques have allowed classification of Pestivirus species according to genotypic diversity rather than animal host (Giangaspero and Harasawa, Reference Giangaspero and Harasawa2007). Currently, the genus Pestivirus is comprised of Bovine viral diarrhea virus (BVDV) 1, BVDV 2, Border disease virus (BDV) and Classical swine fever virus (CSFV). Recent description of genetically distinct pestiviruses such as the genotypes Pronghorn pestivirus, Giraffe pestivirus, strain V60 (Reindeer-1), Ho_Bi strain and others may lead to further rearrangements of classification (Dekker et al., Reference Dekker, Wensvoort and Terpstra1995; Fischer et al., Reference Fischer, Weiland and Frolich1998; Avalos-Ramirez et al., Reference Avalos-Ramirez, Orlich, Thiel and Becher2001; Becher et al., Reference Becher, Avalos Ramirez, Orlich, Cedillo Rosales, Konig, Schweizer, Stalder, Schirrmeier and Thiel2003; Schirrmeier et al., Reference Schirrmeier, Strebelow, Depner, Hoffmann and Beer2004; Vilcek et al., Reference Vilcek, Ridpath, Van Campen, Cavender and Warg2005).
Pestiviruses employ various strategies that ensure their survival and successful propagation in mammalian hosts, including suppression of the host's immune system, transmission by various direct and indirect routes, and, perhaps most importantly, induction of persistently infected (P1) hosts that shed and transmit BVDV more efficiently than other sources. Accumulating evidence suggests that pestiviruses are able to infect various mammalian host species in the order Artiodactyla, which may play an important role in the viral survival strategy (Vilcek and Nettleton, Reference Vilcek and Nettleton2006). The implications of heterologous pestivirus infections are two-fold. First, infection of hosts other than cattle may drastically handicap the successful implementation of BVDV control strategies if these hosts become reservoirs; and second, clinical disease in other mammalian hosts such as zoo animal and wildlife species may be observed.
Among pestiviruses, BVDV has been the species most intensely studied as an infectious agent of heterologous hosts. BVDV infection in species other than cattle has been detected mainly by seropositivity, especially in wildlife species. Although the presence of antibodies may provide only limited information as to the role of a species in the ecology of BVDV, high seroprevalence rates within populations suggest the existence of endemic cycles (Van Campen et al., Reference Van Campen, Ridpath, Williams, Cavender, Edwards, Smith and Sawyer2001). BVDV infections have been documented in domestic small ruminants and swine, free-ranging and captive wildlife, and in camelids, and the epidemiology of BVDV infection may revolve around these proposed host clusters (Evermann, Reference Evermann2006). Within these proposed host clusters, intra-host maintenance of BVDV occurs but the inter-cluster transmission requires optimal conditions or may be negligible (Evermann, Reference Evermann2006). This review will summarize BVDV infections in each host cluster as well as swine and discuss their implications.
BVDV in domestic small ruminants and swine
Goats and sheep
Among Artiodactyla, sheep and goats may have a unique role in the ecology of BVDV. Sheep and goats are phylogenetically more closely related to cattle than most other species considered in this review; therefore, the chance to establish permissive infections is high (DeFillipis and Villareal, Reference DeFillipis, Villareal and Hurst2000). Furthermore, sheep and goats may have close contact with cattle under certain management conditions, such as subsistence agriculture, alpine farming, or hobby farming, thus contributing to BVDV infections in small ruminants. Although one report did not identify a positive correlation between seropositivity of sheep flocks and presence of cattle (Graham et al., Reference Graham, Calvert, German and McCullough2001), other studies reported significantly higher seroprevalence rates in sheep on farms with cattle (Tegtmeier et al., Reference Tegtmeier, Stryhn, Uttentha, Kjeldsen and Nielsen2000; Krametter-Frotscher et al., Reference Krametter-Frotscher, Loitsch, Kohler, Schleiner, Schiefer, Mostl, Golja and Baumgartner2007), especially where communal alpine pasturing of sheep, goats and cattle was practiced (Krametter-Frotscher et al., Reference Krametter-Frotscher, Loitsch, Kohler, Schleiner, Schiefer, Mostl, Golja and Baumgartner2007).
Several serological surveys have demonstrated the occurrence of BVDV in small ruminants (Table 1); however, cross-reactivity of pestivirus antibodies warrants caution when evaluating the results of such surveys. Surprisingly, in recent reports on pestiviral seroprevalence in sheep, cross-neutralization assays identified highest reactivity against BVDV 1 rather than BDV (Graham et al., Reference Graham, Calvert, German and McCullough2001; Schleiner et al., Reference Schleiner, Krametter-Frobtscher, Schiefer, Loitsch, Golja, Mostl and Baumgartner2006; Krametter-Frotscher et al., Reference Krametter-Frotscher, Loitsch, Kohler, Schleiner, Schiefer, Mostl, Golja and Baumgartner2007; Mishra et al., Reference Mishra, Rajukumar, Tiwari, Nema, Behera, Satav and Dubey2009). In Argentina, which is considered free from BDV, 79.7% (43/54 sheep) of samples were seropositive to BVDV 1 or BVDV 2, indicating BVDV circulation in Patagonian populations (Julia et al., Reference Julia, Craig, Jimenez, Pinto and Weber2009). This is contrasted with another recent report where the majority of seropositive sheep resulted from BDV infection, while the infecting pestivirus in goats remained largely unresolved, as indicated by cross-neutralization assays (Danuser et al., Reference Danuser, Vogt, Kaufmann, Peterhans and Zanoni2009). When antibodies against pestivirus are detected in small ruminants, the distinction of pestivirus species may hold critical information on epidemiology, prevention and control (Konig et al., Reference Konig, Cedillo Rosales, Becher and Thiel2003).
Table 1. Reported prevalence rates for antibodies directed against BVDV in domestic small ruminants
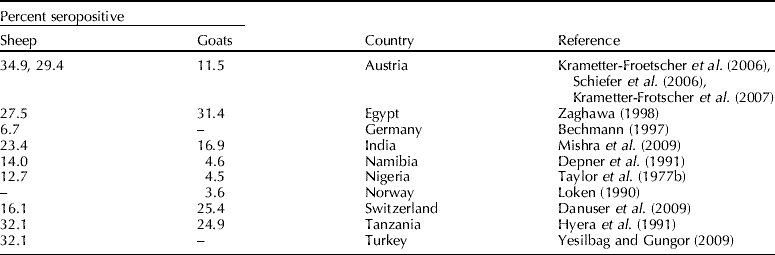
–, no data available.
From reports on pestiviral infections in small ruminants prior to the 1990s, determination of whether BDV or BVDV was the infecting species may be difficult. Of 53 viral strains designated as BDV in the nucleotide sequence databases, 22 were revealed to be BVDV by genotyping based on palindromic nucleotide substitutions (Giangaspero and Harasawa, Reference Giangaspero and Harasawa1999). Infections with both species of BVDV have been reported in domestic small ruminants worldwide (Paton et al., Reference Paton, Carlsson, Lowings, Sands, Vilcek and Alenius1995; Pratelli et al., Reference Pratelli, Martella, Cirone, Buonavoglia, Elia, Tempesta and Buonavoglia2001; Yadav et al., Reference Yadav, Barde, Jadi, Gokhale, Basu, Joshi, Mehla, Kumar, Athavale and Mourya2004; Kim et al., Reference Kim, Hyun, Shin, Lee, Lee, Cho and Kang2006; Willoughby et al., Reference Willoughby, Valdazo-Gonzalez, Maley, Gilray and Nettleton2006; Krametter-Frotscher et al., Reference Krametter-Frotscher, Loitsch, Kohler, Schleiner, Schiefer, Mostl, Golja and Baumgartner2007; Mishra et al., Reference Mishra, Dubey, Rajukumar, Tosh, Tiwari, Pitale and Pradhan2007, Reference Mishra, Rajukumar, Vilcek, Tiwari, Satav and Dubey2008). The inverse, infection and clinical signs of pestivirus infection in cattle associated with BDV appear to be rare (Schirrmeier et al., Reference Schirrmeier, Strebelow, Tavella and Stifter2008). Therefore, determination of which pestivirus is the cause of infection in small ruminants is especially important where BVDV control programs in cattle are in progress.
Infections with BVDV in domestic small ruminants result in clinical signs of Border disease. Postnatal infections commonly cause mild clinical signs, including pyrexia and leucopenia (Taylor et al., Reference Taylor, Okeke and Shidali1977a). Experimental inoculation of juvenile sheep by the intranasal, intratracheal and intrabronchial routes resulted in anorexia, tachycardia, pyrexia and lung lesions especially associated with the pulmonary vasculature (Meehan et al., Reference Meehan, Lehmkuhl, Cutlip and Bolin1998). Strain-dependent severity of BVDV-associated pathology was observed in 62 gnotobiotic lambs infected with 1 of 10 BVDV strains, and pyrexia, pneumonia, myocarditis and encephalitis were observed (Jewett et al., Reference Jewett, Kelling, Frey and Doster1990). Infections with BVDV in pregnant small ruminants may result in uteroplacental pathology and pregnancy loss by fetal resorption or abortions (Loken and Bjerkas, Reference Loken and Bjerkas1991). The outcome of transplacental infection depends on the length of gestation and biotype of infecting BVDV strain. Fetal mummification, stillbirth, premature birth and birth of offspring with congenital disorders may result from in utero BVDV infection. Congenital disorders of affected fetuses commonly involve the central nervous system, and neuropathogenicity may be more severe with cytopathic biotypes (Gruber et al., Reference Gruber, Hewicker-Trautwein, Liess and Trautwein1995; Hewicker-Trautwein et al., Reference Hewicker-Trautwein, Trautwein, Frey and Liess1995). Although fetal death and non-viability of lambs are common sequelae of transplacental BVDV in sheep, reports of viable PI offspring exist (Carlsson and Belak, Reference Carlsson and Belak1994; Scherer et al., Reference Scherer, Flores, Weiblen, Caron, Irigoyen, Neves and Maciel2001). In contrast to cattle and sheep, viable PI kids appear to be a rare result of in utero BVDV infection in goats and reproductive failure or severe gross and histological pathology of affected fetuses are the likely result of infection (Loken and Bjerkas, Reference Loken and Bjerkas1991; Kim et al., Reference Kim, Hyun, Shin, Lee, Lee, Cho and Kang2006; Broaddus et al., Reference Broaddus, Lamm, Kapil, Dawson and Holyoak2009).
Swine
The close antigenic relationship between ruminant pestiviruses and CSFV has important implications for diagnostic testing and control of CSFV. Currently, examination of blood samples for CSFV antibodies relies on a combination of enzyme linked immunosorbent assay (ELISA) and virus neutralization techniques, and considerable efforts are required to establish a CSFV-specific diagnosis (de Smit et al., Reference de Smit, Eble, de Kluijver, Bloemraad and Bouma1999). During the CSFV outbreak in the Netherlands in 1997/1998, 26.5% of CSFV ELISA positive samples were demonstrated to be caused by the presence of antibodies to ruminant pestiviruses (de Smit et al., Reference de Smit, Eble, de Kluijver, Bloemraad and Bouma1999). The presence of antibodies against ruminant pestivirus in swine resulting in suspicion of CSFV infection has prompted the unnecessary depopulation of herds, further emphasizing the impact of porcine infections with ruminant pestiviruses (Snowdon and French, Reference Snowdon and French1968; Oguzoglu et al., Reference Oguzoglu, Floegel-Niesmann, Frey and Moennig2001).
Comparative cross-neutralization experiments or monoclonal antibodies have been commonly used to differentiate the causative viral species in seropositive samples (Liess and Moennig, Reference Liess and Moennig1990). However, CSFV challenge in swine with prior BVDV infection resulted in higher titers against BVDV than CSFV according to the neutralization peroxidase linked assay, indicating that prior BVDV infection could result in false-negative CSFV diagnosis (Wieringa-Jelsma et al., Reference Wieringa-Jelsma, Quak and Loeffen2006). Furthermore, a recently developed E2 subunit CSFV marker has been used in combination with an antibody-ELISA against the ERNS to differentiate vaccinated pigs from pigs exposed naturally to CSFV; however, BVDV infection and subsequent seroconversion may result in false-positive CSFV serologic tests, complicating the use of this vaccination and testing strategy in a control scenario (Blome et al., Reference Blome, Meindl-Bohmer, Loeffen, Thuer and Moennig2006; Loeffen et al., Reference Loeffen, van Beuningen, Quak and Elbers2009).
Since the first description of an antigenic relationship between CSFV and BVDV in 1960 (Darbyshire, Reference Darbyshire1960), scientific interest has revolved around the use of BVDV to immunize swine against CSFV (Beckenhauer et al., Reference Beckenhauer, Brown, Lidolph and Norden1961; Simonyi and Biro, Reference Simonyi and Biro1967; Baker et al., Reference Baker, Coggins, Robson, Sheffy and Volenec1969; Liou et al., Reference Liou, Chen, Lin, Sheffy and Lee1975). Difficulties arise when comparing these studies, as various BVDV isolates or vaccine titers were used for immunization, which may explain some of the discordant results. In extensive studies using strain Oregon C24V BVDV vaccine prior to challenge with various CSFV laboratory and field isolates, Baker et al. (Reference Baker, Coggins, Robson, Sheffy and Volenec1969) demonstrated protection rates of up to 95% in challenged vaccinates. Furthermore, BVDV vaccination was safe, provided protection from clinical CSFV infection for over 2 years, and BVDV was not spread to in-contact pigs or cattle (Baker et al., Reference Baker, Coggins, Robson, Sheffy and Volenec1969). In contrast, all but one pig immunized with BVDV C24V died after challenge with CSFV (Simonyi and Biro, Reference Simonyi and Biro1967). When immunizing swine with one of four strains of BVDV and subsequent challenge with CSFV, no clinical signs were observed after immunization with BVDV strain Tobias, while mild clinical signs of disease were observed in swine immunized with BVDV strains NY-1 or VJGM, and typical signs of classical swine fever were observed in swine immunized with BVDV strain Egan (Liou et al., Reference Liou, Chen, Lin, Sheffy and Lee1975). In a comparison of vaccination efficacy of a BVDV strain NY-1 vaccine with a modified live CSFV vaccine, BVDV vaccinated swine were not as well protected as CSFV vaccinates when challenged with 1 of 10 CSFV isolates (Tamoglia et al., Reference Tamoglia, Tellejohn, Phillips and Wilkinson1965). In that study, transmission of vaccine virus was observed in CSFV vaccinates, but not in BVDV vaccinates (Tamoglia et al., Reference Tamoglia, Tellejohn, Phillips and Wilkinson1965). Recovery of BVDV was possible from the respiratory tract of swine vaccinated with strain CL10, indicating that BVDV may be transmitted by vaccinates (Phillip and Darbyshire, Reference Phillip and Darbyshire1972). More recently, the BVDV strain St. Oedenrode, which had been isolated from a naturally infected pig, was used to immunize swine prior to CSFV challenge (Wieringa-Jelsma et al., Reference Wieringa-Jelsma, Quak and Loeffen2006). Immunization with this strain resulted in a self-limiting BVDV infection (R=0.2) and protected vaccinates from clinical disease and CSFV transmission upon challenge (Wieringa-Jelsma et al., Reference Wieringa-Jelsma, Quak and Loeffen2006).
Only a limited number of serosurveys have been performed in domestic swine and reported seroprevalence rates are relatively low when compared to other domestic livestock. Risk factors associated with seropositivity of domestic swine include the presence of cattle on the same farm, high density of small ruminants near swine populations, vaccination with BVDV contaminated vaccines, and age of tested swine (Lenihan and Collery, Reference Lenihan and Collery1977; Vannier et al., Reference Vannier, Leforban, Carnero and Cariolet1988; Liess and Moennig, Reference Liess and Moennig1990; Loeffen et al., Reference Loeffen, van Beuningen, Quak and Elbers2009). In an Irish serosurvey, 27.8% of swine in contact with cattle possessed neutralizing antibodies to BVDV, as compared to 4.0% in swine without contact with cattle (Lenihan and Collery, Reference Lenihan and Collery1977). The feeding of bovine offal was also described as a source of BVDV infection in swine (Stewart et al., Reference Stewart, Carbrey, Jenney, Brown and Kresse1971). Increasing specialization of farms resulting in decreased chances for interspecific contacts and improved quality control in vaccine production were suggested to be reasons for a decrease in seroprevalence rates in Dutch swine, where 20.0% of slaughterhouse samples from sows and boars were positive in 1991 (Terpstra and Wensvoort, Reference Terpstra and Wensvoort1991), as compared to 2.5% in sows and 0.42% in finishing pigs, and 11.0 and 3.2% at the respective herd level in 2009 (Loeffen et al., Reference Loeffen, van Beuningen, Quak and Elbers2009). Only one of 660 serum samples was determined to be positive for BVDV antibodies in a study in Northern Ireland, and the authors suggested that use of a BDV ELISA rather than BVDV ELISA for initial serosurveillance may have confounded this comparatively low result (Graham et al., Reference Graham, Calvert, German and McCullough2001). Other reports found seroprevalence rates of 3.01% in Canada, 6.4% in Denmark, 3.2% in Ireland and 2.2% in Norway (Jensen, Reference Jensen1985; Afshar et al., Reference Afshar, Dulac and Bouffard1989; Loken et al., Reference Loken, Krogsrud and Larsen1991; O'Connor et al., Reference O'Connor, Lenihan and Dillon1991).
Studies on postnatal BVDV infections in non-pregnant swine have rarely described the occurrence of clinical signs based on observation of infected animals (Beckenhauer et al., Reference Beckenhauer, Brown, Lidolph and Norden1961; Carbrey et al., Reference Carbrey, Stewart, Kresse and Snyder1976; Liess and Moennig, Reference Liess and Moennig1990; Dahle et al., Reference Dahle, Schagemann, Moennig and Liess1993; Walz et al., Reference Walz, Baker, Mullaney, Kaneene and Maes1999; Woods et al., Reference Woods, Kunkle, Ridpath and Bolin1999; Makoschey et al., Reference Makoschey, Liebler-Tenorio, Biermann, Goovaerts and Pohlenz2002). When evaluated, an elevation of the rectal temperature after BVDV inoculation was not detected in most studies despite evidence of viremia and seroconversion (Beckenhauer et al., Reference Beckenhauer, Brown, Lidolph and Norden1961; Carbrey et al., Reference Carbrey, Stewart, Kresse and Snyder1976; Walz et al., Reference Walz, Baker, Mullaney, Kaneene and Maes1999). Experimental infection of six- to ten-week-old pigs with a BVDV 2 inoculum containing non-cytopathic and cytopathic biotypes, resulted in slight pyrexia, leucopenia and thrombocytopenia (Makoschey et al., Reference Makoschey, Liebler-Tenorio, Biermann, Goovaerts and Pohlenz2002), which were less pronounced than had been reported in cattle experimentally inoculated using a very similar protocol (Makoschey et al., Reference Makoschey, Janssen, Vrijenhoek, Korsten and Marel2001). Significant, but less severe than observed with homologous pestivirus infections, decreases in lymphocyte populations were detected in intranasally inoculated pigs and a more marked decrease in CD8+ lymphocytes was noticed (Rypula, Reference Rypula2003). Experimental infection of eight-week-old pigs with either BVDV 1 or BVDV 2 did not result in clinical signs or increases in body temperature in any group, despite the use of a BVDV 2 isolate that was demonstrated to be virulent for cattle (Walz et al., Reference Walz, Baker, Mullaney, Kaneene and Maes1999). In contrast, inoculation of three-day-old piglets with either BVDV strain NY-1 or a cell-culture contaminant, BVDV-C, resulted in loss of appetite, diarrhea and intestinal pathology for 1–4 days (Woods et al., Reference Woods, Kunkle, Ridpath and Bolin1999). Importantly, the co-infection of either BVDV NY-1 or BVDV-C with attenuated transmissible gastroenteritis virus resulted in severe clinical signs that were worse with the cell-culture contaminant BVDV-C (Woods et al., Reference Woods, Kunkle, Ridpath and Bolin1999). That report underscored the potential impact of cell culture contamination with BVDV, which may result in enhanced pathogenicity of attenuated vaccines (Woods et al., Reference Woods, Kunkle, Ridpath and Bolin1999).
Carbrey et al. (Reference Carbrey, Stewart, Kresse and Snyder1976) suspected BVDV infection as the cause of reproductive failure, including poor conception, small litter size and abortions after antibody titers against BVDV were determined to be higher than against CSFV in breeding sows. Experimental infection of pregnant gilts with one of four BVDV isolates resulted in reproductive disease including intrauterine infection, pregnancy loss and reduction in litter size (Stewart et al., Reference Stewart, Miller, Kresse and Snyder1980). In that study, fetal infection was established in 1 of 20 infected gilts (Stewart et al., Reference Stewart, Miller, Kresse and Snyder1980). Similarly, experimental transplacental infection was documented in only one of 43 fetuses after intranasal inoculation of four pregnant gilts, indicating an inconsistent ability of BVDV to cross the blood-placental barrier in swine (Walz et al., Reference Walz, Baker, Mullaney and Maes2004). Seroconversion, failure to thrive and premature death have been reported in transplacentally infected swine (Carbrey et al., Reference Carbrey, Stewart, Kresse and Snyder1976; Paton et al., Reference Paton, Simpson and Done1992). Petechiation and hemorrhages, enlarged lymph nodes, meningitis, myocarditis, nephritis and hepatic necrosis were observed in piglets born to a pregnant sow that was exposed to a persistently infected calf (Paton et al., Reference Paton, Simpson and Done1992). Contamination of a CSFV vaccine with BVDV resulted in dead or mummified fetuses, and many living fetuses from vaccinated sows were BVDV positive and showed alopecia, congenital tremors, lymphadenopathy, multifocal petechiation and ascites. Experimental infection with one of four strains of BVDV between 28 and 55 days of gestation resulted in fetal infection in only one of 20 gilts (Stewart et al., Reference Stewart, Miller, Kresse and Snyder1980). The authors suggest that passages in porcine PK-15 cells may have resulted in a loss of virulence and the inability to cause transplacental infection in the other BVDV strains evaluated in the experiment (Stewart et al., Reference Stewart, Miller, Kresse and Snyder1980). In the transplacentally infected 13 fetuses, low antibody titers (<1:4–1:16) and histopathological lesions within leptomeninges and choroid plexus were detected (Stewart et al., Reference Stewart, Miller, Kresse and Snyder1980). In contrast, antibodies against BVDV did not develop in a persistently viremic pig until slaughter at 26 months and in a littermate, seroconversion was not detected until six to eight months of age (Terpstra and Wensvoort, Reference Terpstra and Wensvoort1997). In the affected litter of 13, 10 piglets died or were euthanized within weeks after birth and multiple clinical signs, including thymic atrophy, were detected. Consistent shedding of BVDV until slaughter was detected only in the seronegative PI pig and ceased in its littermates upon seroconversion (Terpstra and Wensvoort, Reference Terpstra and Wensvoort1997).
BVDV in New and Old World Camelids (NWC and OWC)
BVDV infections were identified through detection of antibodies as early as 1975 in OWC and 1983 in NWC (Burgemeister et al., Reference Burgemeister, Leyk and Goessler1975; Doyle and Heuschele, Reference Doyle and Heuschele1983). In different countries, seroepidemiologic studies identified BVDV antibodies in 0–19.7% of tested OWC (Burgemeister et al., Reference Burgemeister, Leyk and Goessler1975; Hedger et al., Reference Hedger, Barnett and Gray1980; Bornstein and Musa, Reference Bornstein and Musa1987; Bohrmann et al., Reference Bohrmann, Frey and Liess1988; Wernery and Wernery, Reference Wernery and Wernery1990; Raoofi et al., Reference Raoofi, Hemmatzadeh and Ghanaei2009), but a much higher seroprevalence (52.5%) was reported from two regions in Egypt (Zaghawa, Reference Zaghawa1998). Wernery and Wernery (Reference Wernery and Wernery1990) explained a higher seroprevalence rate in breeding camels of 9.2% as compared to 3.6% in racing camels by close contact with cattle herds, more intensive management and large herd sizes in breeding camels. While the seroprevalence was not different between male and female dromedary camels, higher age was associated with an increased rate of seropositivity (Raoofi et al., Reference Raoofi, Hemmatzadeh and Ghanaei2009).
In an outbreak of reproductive disease among nine pregnant dromedary camels, abortion, still births, weak calves, early neonatal death and neonatal hemorrhagic disease were observed (Hegazy et al., Reference Hegazy, Lotifa, Saber, Aboellail, Yousif and Chase1996). Gross and histopathological lesions included congenital cataracts, mild cerebellar hypoplasia, lymphoid depletion, vasculitis, optic neuritis and underpopulated cerebellar molecular layer. Cytopathic BVDV was isolated from two stillborn and one live-born camel calves (Hegazy et al., Reference Hegazy, Lotifa, Saber, Aboellail, Yousif and Chase1996). Viral isolates were further characterized later by the same laboratory and were typed as BVDV 1 and BVDV 2 (Yousif et al., Reference Yousif, Braun, Saber, Aboellail and Chase2004). Non-cytopathic BVDV has also been isolated from tissues of camels in which histopathological changes were consistent with a BVDV infection (Wahab et al., Reference Wahab, Hussein, Asfowr and Shalaby2005).
In the past, seroepidemiologic and experimental infection studies suggested that BVDV may cause infections without presenting a serious risk to NWC. Low seroprevalence rates, infections with mild or no clinical signs, and limited viral replication within buffy coat cells supported the conclusion that BVDV may not be a significant pathogen in NWC (Mattson, Reference Mattson1994; Evermann, Reference Evermann2006). Experimental inoculation of four pregnant llamas with a non-cytopathic isolate from an aborted llama fetus did not result in clinical signs or fetal infection (Wentz et al., Reference Wentz, Belknap, Brock, Collins and Pugh2003). Reported seroprevalence rates in South American domestic NWC are 11.1% in Peruvian alpacas, 2.05% in Argentinean llamas and 10.8 and 14% in Chilean alpacas and llamas, respectively (Rivera et al., Reference Rivera, Madewell and Ameghino1987; Puntel et al., Reference Puntel, Fondevila, Blanco Viera, O'Donnell, Marcovecchio, Carrillo and Schudel1999; Celedon et al., Reference Celedon, Sandoval, Drougett, Calfio, Ascencio, Pizarro and Navarro2001). Seropositivity was associated with the presence of other domestic livestock and domestic NWC without exposure to other livestock or free-ranging NWC were seronegative (Karesh et al., Reference Karesh, Uhart, Dierenfeld, Braselton, Torres, House, Puche and Cook1998; Celedon et al., Reference Celedon, Sandoval, Drougett, Calfio, Ascencio, Pizarro and Navarro2001). In North America, a seroprevalence rate of 4.4% was reported for llamas in Oregon (Mattson, Reference Mattson1994). In southern California, 18.6% of tested alpacas were seropositive and antibody titers were higher against BVDV 1 than BVDV 2 in most cases (Shimeld, Reference Shimeld2009). The highest titers were detected on farms on which PI crias were present (Shimeld, Reference Shimeld2009). Antibodies against BVDV were detected in crias from 16 of 63 (25.4%) herds in the United States (Topliff et al., Reference Topliff, Smith, Clowser, Steffen, Henningson, Brodersen, Bedenice, Callan, Reggiardo, Kurth and Kelling2009).
Reports of BVDV isolation and identification of persistent infections in alpacas have prompted increasing attention by industry and the scientific community and the virus is now considered an emerging pathogen of NWC (Byers et al., Reference Byers, Snekvik, Righter, Evermann, Bradway, Parish and Barrington2009). Preceding the descriptions of persistent infections in alpacas, a cytopathic BVDV isolate was identified in kidney, liver, lung and spleen samples from a stillborn llama (Belknap et al., Reference Belknap, Collins, Larsen and Conrad2000). In the same report, BVDV was detected in tissues of a late pregnant adult llama and an emaciated juvenile llama. Non-cytopathic BVDV 1b isolates were detected in tissues of a stillborn alpaca (lung, liver, kidney and spleen) and tissues from a seven-month-old alpaca (lung, liver and kidney) with ill-thrift, respectively (Goyal et al., Reference Goyal, Bouljihad, Haugerud and Ridpath2002; Foster et al., Reference Foster, Houlihan, Higgins, Errington, Ibata and Wakeley2005). The first description of a persistent infection in alpacas was made in Canada following natural exposure of a pregnant alpaca to a chronically ill cria (Carman et al., Reference Carman, Carr, DeLay, Baxi, Deregt and Hazlett2005). From the persistently infected cria, a BVDV 1b strain was isolated from buffy coat cells on different occasions before euthanasia of this animal. Several cases of PI alpacas have since been reported in North America and Great Britain (Mattson et al., Reference Mattson, Baker, Catania, Imbur, Wellejus and Bell2006; Foster et al., Reference Foster, Houlihan, Holmes, Watt, Higgins, Errington, Ibata and Wakeley2007; Barnett et al., Reference Barnett, Twomey, Millar, Bell, Bradshaw, Higgins, Scholes, Errington, Bromage and Oxenham2008; Byers et al., Reference Byers, Snekvik, Righter, Evermann, Bradway, Parish and Barrington2009; Kim et al., Reference Kim, Anderson, Yu, Zylich, Kinde, Carman, Bedenice and Dubovi2009). Persistently infected alpacas may survive for several months but are affected by low birth weight, failure to thrive, inappetence, lethargy, chronic diarrhea and chronic recurrent infections especially of the respiratory tract. In PI alpacas, BVDV antigen may be detected in epithelial and immune cells of many tissues, including skin, brain, thyroid gland, parotid salivary gland, testis, prostate, esophagus, gastric compartments, kidneys, bone marrow, liver, lung, thymus and lymph nodes, and skin staining patterns are consistent with those in cattle (Carman et al., Reference Carman, Carr, DeLay, Baxi, Deregt and Hazlett2005; Foster et al., Reference Foster, Houlihan, Holmes, Watt, Higgins, Errington, Ibata and Wakeley2007; Byers et al., Reference Byers, Snekvik, Righter, Evermann, Bradway, Parish and Barrington2009).
From NWC in Chile, both BVDV 1 and BVDV 2 have been isolated (Celedon et al., Reference Celedon, Osorio and Pizarro2006). In contrast, in reports on BVDV isolations from alpacas in North America and the United Kingdom in which the subgenotype of the infecting isolate was determined, all isolates belonged to BVDV 1b (Carman et al., Reference Carman, Carr, DeLay, Baxi, Deregt and Hazlett2005; Foster et al., Reference Foster, Houlihan, Higgins, Errington, Ibata and Wakeley2005, Reference Foster, Houlihan, Holmes, Watt, Higgins, Errington, Ibata and Wakeley2007; Byers et al., Reference Byers, Snekvik, Righter, Evermann, Bradway, Parish and Barrington2009). A recent study analyzed 46 BVDV isolates from over 12,000 North American alpacas and classified all isolates as non-cytopathic strains of subgenotype 1b. Nucleotide identity of ≥99% was demonstrated in 45 of 46 isolates using the highly conserved 5′-UTR, indicating an unusual association of the 1b genotype with BVDV infections in North American alpacas (Kim et al., Reference Kim, Anderson, Yu, Zylich, Kinde, Carman, Bedenice and Dubovi2009). Kim et al. (Reference Kim, Anderson, Yu, Zylich, Kinde, Carman, Bedenice and Dubovi2009) offer two explanations for this peculiarity, firstly that exposures of alpacas to BVDV are rare and spread of the existing BVDV 1b strain is by extensive movement of a few PI animals or secondly, only unique 1b subgenotypes are able to establish transplacental infections in alpacas. Interestingly, the simultaneous inoculation of pregnant alpacas with two BVDV 1b isolates of cattle or alpaca origin, respectively, and a BVDV 2 strain of cattle origin, resulted in birth of crias PI with 1b of cattle or alpaca origin, but not BVDV 2 (Edmonson et al., Reference Edmondson, Walz, Johnson, Gard, Marley, Eason and Givens2009). This may further support a unique role of BVDV 1b in alpacas; however, viremia, nasal shedding and seroconversion were observed when alpacas were inoculated with BVDV 1b or BVDV 2 strains (Johnson et al., Reference Johnson, Edmonson, Walz and Givens2009). Movement of alpacas (including dams with cria by foot) between farms, mainly for breeding purposes, are common practice and were described in reports of reproductive disease and birth of PI offspring, highlighting the importance of sound biosecurity practices (Carman et al., Reference Carman, Carr, DeLay, Baxi, Deregt and Hazlett2005; Foster et al., Reference Foster, Houlihan, Holmes, Watt, Higgins, Errington, Ibata and Wakeley2007; Barnett et al., Reference Barnett, Twomey, Millar, Bell, Bradshaw, Higgins, Scholes, Errington, Bromage and Oxenham2008; Topliff et al., Reference Topliff, Smith, Clowser, Steffen, Henningson, Brodersen, Bedenice, Callan, Reggiardo, Kurth and Kelling2009).
BVDV in wildlife
In addition to the ability to induce persistent infections, the large number of wildlife species in which infections with BVDV have been demonstrated, emphasizes the potential for another important survival strategy of BVDV, the ability to cross species barriers. With few exceptions, infections with BVDV appear to be limited to species in the order Artiodactyla. Antibodies were detected in 2 of 44 Bennett's wallaby (Macropus rufogriseus); however, the titers against BVDV C24V were at dilutions of less than 1:3 (Munday, Reference Munday1972). Antibodies against BVDV were also detected in free-ranging rabbits (Oryctolagus cuniculus), but virus isolation or detection of viral genome from spleen samples were not successful (Frolich and Streich, Reference Frolich and Streich1998).
The mammalian order Artiodactyla is comprised of 10 families and 240 species (Grubb, Reference Grubb, Wilson and Reeder2005), and evidence of BVDV infection exists in the seven families Antilocapridae, Bovidae, Camelidae, Cervidae, Giraffidae, Suidae and Tragulidae, including over 50 species (Nettleton, Reference Nettleton1990; Van Campen et al., Reference Van Campen, Ridpath, Williams, Cavender, Edwards, Smith and Sawyer2001; Grondahl et al., Reference Grondahl, Uttenthal, Houe, Rasmussen, Hoyer and Larsen2003). In most species, evidence of BVDV infection mainly has been by the identification of antibodies, therefore it may be difficult to estimate the implications on health of affected species or on BVDV control programs; however, identification of high seroprevalence rates may suggest the presence of endemic cycles (Van Campen et al., Reference Van Campen, Ridpath, Williams, Cavender, Edwards, Smith and Sawyer2001). Several seroepidemiological studies in free-ranging wildlife have been performed globally and large variations of seroprevalence status exist among the studied populations (Table 2). The scale, both of the number of samples collected and the size of the sampled habitat, and the employed sampling strategies vary tremendously among serosurveys, perhaps partially explaining the observed variations in seroprevalence.
Table 2. Reported prevalence rates for antibodies directed against BVDV in free-ranging wildlife

a Seropositive: roe deer and red deer; seronegative: fallow deer, wild boar and European mouflon.
b Seropositive: fallow deer, red deer, Chinese water deer and sika deer; seronegative: roe deer.
c Seropositive: axis deer; seronegative: fallow deer.
d Seropositive: African buffalo, nyala, kudu, eland, waterbuck, defassa waterbuck, lechwe, reedbuck, sable antelope, oryx, tsessebe, hartebeest, wildebeest, impala, springbok, duiker, giraffe and wart hog; seronegative: bush buck, puku, kob, roan antelope, topi, blesbok, Grant's gazelle, klipspringer, oribi, steinbok, grysbok, hippopotamus, elephant, bush pig and zebra.
e Seropositive: caribou; seronegative: dall sheep, moose and American bison.
f Seropositive: sika deer and white-tail deer.
g Seropositive: roe deer and red deer; seronegative: fallow deer and European mouflon.
h Seropositive: giraffe, gemsbok, roan antelope, blue wildebeest, eland, kudu and sable antelope; seronegative: red hartebeest, black wildebeest and springbok.
i Seropositive: African buffalo, wildebeest and topi.
j Seropositive: mule deer and wapiti.
k Seropositive: fallow deer, roe deer and red deer.
l Seropositive: nyala, eland, bushbuck, Lichtenstein's hartebeest, kudu, reedbuck, African buffalo, sable antelope, impala, wildebeest, waterbuck, tsessebe and giraffe; seronegative: warthog and white rhinoceros.
m Seropositive: red deer; seronegative: roe deer, fallow deer and sika deer.
n Seropositive: roe deer, reindeer, moose and red deer.
o Seropositive: red deer; seronegative: roe deer, fallow deer and chamois.
p Seropositive: red deer, wild boar and chamois; seronegative: roe deer.
q Seronegative: red deer, roe deer and fallow deer.
Although sources of BVDV infections in free-ranging wildlife are unknown, a likely source is contact with cattle, as has been suggested by various authors (Riemann et al., Reference Riemann, Ruppanner, Willeberg, Franti, Elliott, Fisher, Brunetti, Aho, Howarth and Behymer1979; Stauber et al., Reference Stauber, Autenrieth, Markham and Whitbeck1980; Kocan et al., Reference Kocan, Franzmann, Waldrup and Kubat1986; Nielsen et al., Reference Nielsen, Roensholt and Bitsch2000). This is further supported by the absence of antibodies in deer that were without contact with cattle for over 50 years (Sadi et al., Reference Sadi, Joyal, St-Georges and Lamontagne1991). Significantly higher seroprevalence rates were found in white-tailed deer on ranches where cattle were present as compared to ranches without cattle (Cantu et al., Reference Cantu, Ortega, Mosqueda, Garcia-Vazquez, Henke and George2008). In contrast, an association between BVDV seroprevalence and cattle density was not found in another study (Frolich, Reference Frolich1995). Similar findings were described in a recent report from Minnesota where a greater percentage of deer were seropositive in a region with lower cattle density (Wolf et al., Reference Wolf, Deperno, Jenks, Stoskopf, Kennedy-Stoskopf, Swanson, Brinkman, Osborn and Tardiff2008). The authors of that report concluded that cattle use and management (i.e. dairy or beef) may have an important impact on interspecific BVDV transmission, as there is likely less wildlife contact with housed dairy cattle as compared to pastured beef cattle (Wolf et al., Reference Wolf, Deperno, Jenks, Stoskopf, Kennedy-Stoskopf, Swanson, Brinkman, Osborn and Tardiff2008). This factor was not evaluated in the earlier publication from Germany where the majority of cattle operations are dairies (Statistisches Bundesamt, 2007). Various factors likely influence the transmission of BVDV between cattle and wildlife as has been described for other disease such as bovine tuberculosis (Schmitt et al., Reference Schmitt, O'Brien, Bruning-Fann and Fitzgerald2002). With the presence of suitable environmental and management factors, transmission of BVDV from cattle to deer is possible as has been demonstrated recently in a cohabitation experiment (Passler et al., Reference Passler, Walz, Ditchkoff, Brock, Deyoung, Foley and Givens2009a). In that study, BVDV was efficiently transmitted from PI cattle to white-tailed deer and resulted in seroconversion in all adult deer and PI offspring in four of seven pregnancies (Passler et al., Reference Passler, Walz, Ditchkoff, Brock, Deyoung, Foley and Givens2009a).
Maintenance of BVDV within a cervid population without the presence of cattle was suggested by the presence of antibodies in over 60% of caribou that had not been in contact with domestic ruminants for over 25 years (ElAzhary et al., Reference ElAzhary, Frechette, Silim and Roy1981). The presence of endemic infections with BVDV as indicated by high seroprevalence rates was also suggested in reindeer in Norway and US cervid populations (Stuen et al., Reference Stuen, Krogsrud, Hyllseth and Tyler1993; Aguirre et al., Reference Aguirre, Hansen, Starkey and McLean1995; Van Campen et al., Reference Van Campen, Ridpath, Williams, Cavender, Edwards, Smith and Sawyer2001; Lillehaug et al., Reference Lillehaug, Vikoren, Larsen, Akerstedt, Tharaldsen and Handeland2003). This view is further supported by identification of high seroprevalence rates in eland populations of Zimbabwe, in which a PI and a virus-isolation-positive eland were identified (Anderson and Rowe, Reference Anderson and Rowe1998). In a follow-up study, the non-cytopathic strains isolated from elands were further characterized with monoclonal antibodies and partial genetic sequencing and were demonstrated to be very similar to the BVDV 1a strains NADL and SD1, indicating that cattle had originally introduced BVDV into the eland population (Vilcek et al., Reference Vilcek, Paton, Rowe and Anderson2000).
Only a limited number of studies have surveyed free-ranging wildlife populations for the presence of BVDV or BVDV antigen. Anderson and Rowe (Reference Anderson and Rowe1998) utilized an antigen capture ELISA to detect BVDV in a subset of 303 seronegative animals during their serosurvey in Zimbabwe and detected two virus positive eland. In Germany, cytopathic BVDV was detected in spleen samples from two of 203 deer and both animals were seronegative roe deer (Frolich and Hofmann, Reference Frolich and Hofmann1995). These isolates were further characterized by monoclonal antibody typing and sequencing of the 5′-UTR, and results indicated that distinct BVDV strains may circulate within the sampled roe deer population (Fischer et al., Reference Fischer, Weiland and Frolich1998). Recently, three surveys utilizing the immunohistochemistry (IHC) or ELISA technique, investigated the occurrence of BVDV in free-ranging cervids in the US and results suggest that PI cervids exist in wildlife populations (Duncan et al., Reference Duncan, Van Campen, Soto, Levan, Baeten and Miller2008b; Passler et al., Reference Passler, Walz, Ditchkoff, Walz, Givens and Brock2008; Pogranichniy et al., Reference Pogranichniy, Raizman, Thacker and Stevenson2008). In Alabama, 1 of 406 skin samples from white-tailed deer was positive on IHC and the antigen distribution resembled that of PI cattle (Passler et al., Reference Passler, Walz, Ditchkoff, Walz, Givens and Brock2008). Similarly, the skin sample of 1 of 5597 deer in Colorado was positive on IHC and this result was confirmed by the detection of viral RNA in skin and lymph node samples (Duncan et al., Reference Duncan, Van Campen, Soto, Levan, Baeten and Miller2008b). In Indiana, 2 of 745 white-tailed deer were positive for BVDV by antigen capture ELISA with subsequent isolation of cytopathic and non-cytopathic BVDV (Pogranichniy et al., Reference Pogranichniy, Raizman, Thacker and Stevenson2008). To date, the validation of BVDV assays for their use in wildlife has not been performed, and this may be critical as considerable variations were observed among the IHC and antigen capture ELISA on skin samples of white-tailed deer (Passler and Walz, unpublished observations). In addition to surveys, isolation of BVDV was successful in free-ranging roe deer in Hungary, a mule deer in Wyoming and two white-tailed deer in South Dakota (Romvary, Reference Romvary1965; Van Campen et al., Reference Van Campen, Ridpath, Williams, Cavender, Edwards, Smith and Sawyer2001; Chase et al., Reference Chase, Braun, Leslie-Steen, Graham, Miskimins and Ridpath2008). In these reports, clinical illness including emaciation, weakness and death prompted further investigations leading to the isolation of BVDV from tissues.
In addition to free-ranging populations, BVDV has been identified in captive herds and zoo collections, and contact with other ruminant species was described in some reports (Nettleton et al., Reference Nettleton, Herring and Corrigall1980; Neumann et al., Reference Neumann, Buitkamp and Ploger1980; Weber et al., Reference Weber, Hurter and Commichau1982; Doyle and Heuschele, Reference Doyle and Heuschele1983; Grondahl et al., Reference Grondahl, Uttenthal, Houe, Rasmussen, Hoyer and Larsen2003; Nelson et al., Reference Nelson, Dark, Bradway, Ridpath, Call, Haruna, Rurangirwa and Evermann2008). Although the role of BVDV as a pathogen of wildlife is largely unclear, isolation of BVDV from clinically ill wildlife emphasizes the potential for BVDV to induce disease in zoo collections. The isolation of BVDV from deceased captive deer submitted for necropsy was successful in different reports; however, whether clinical BVDV infection was cause for the fatalities was mostly uncertain (Neumann et al., Reference Neumann, Buitkamp and Ploger1980; Weber et al., Reference Weber, Hurter and Commichau1982; Diaz et al., Reference Diaz, Steen, Rehbinder and Alenius1988). Severe mucosal disease-like lesions were observed in a nilgai, an axis deer, and a barasingha deer that were co-infected with BVDV and the virus of malignant catarrhal fever, suggesting a possible immunosuppressive effect of BVDV in wildlife (Doyle and Heuschele, Reference Doyle and Heuschele1983). The close vicinity of ruminant species in zoo collections increases the potential for disease transmission and prompted the euthanasia of six PI mousedeer, emphasizing the threat of BVDV for captive endangered species (Grondahl et al., Reference Grondahl, Uttenthal, Houe, Rasmussen, Hoyer and Larsen2003; Uttenthal et al., Reference Uttenthal, Hoyer, Grondahl, Houe, van Maanen, Rasmussen and Larsen2006). In addition to the aforementioned species, the presence of persistent infection was recently demonstrated in a captive mountain goat (Nelson et al., Reference Nelson, Dark, Bradway, Ridpath, Call, Haruna, Rurangirwa and Evermann2008).
The BVDV antigen distribution in two PI white-tailed deer was similar to that of cattle with broad tissue distribution, most notably in epithelium and vascular endothelium (Duncan et al., Reference Duncan, Ridpath, Palmer, Driskell and Spraker2008a). The role that PI wildlife may have in the epidemiology of BVDV, including the frequency of occurrence, transmission potential and survivability is largely unknown. Seroconversion and viral RNA were detected in a calf exposed to a PI Lesser Malayan mousedeer by indirect and direct routes; however, BVDV infection was not evident in a second calf exposed by the same routes (Uttenthal et al., Reference Uttenthal, Hoyer, Grondahl, Houe, van Maanen, Rasmussen and Larsen2006). The maternal line of PI mousedeer remained without clinical signs and were reproductively sound, giving birth to PI offspring (Uttenthal et al., Reference Uttenthal, Hoyer, Grondahl, Houe, van Maanen, Rasmussen and Larsen2006). Experimental inoculation of white-tailed deer fawns did not result in clinical signs, but BVDV was identified by reverse transcription-polymerase chain reaction (RT-PCR) on nasal and rectal swab samples, indicating a potential of transmission (Raizman et al., Reference Raizman, Pogranichniy, Levy, Negron, Langohr and Van Alstine2009). In a PI white-tailed deer, virus isolation titers from nasal swab samples were equivalent to those in PI cattle (Passler et al., Reference Passler, Walz, Ditchkoff, Givens, Maxwell and Brock2007). The cohabitation of pregnant white-tailed deer with a PI white-tailed deer resulted in seroconversion of all exposed animals and birth of a PI fawn, indicating that maintenance of BVDV in cervid populations is possible when a PI animal is present (Passler et al., Reference Passler, Walz, Ditchkoff, Givens, DeYoung and Brock2009b). Superinfection of a PI mousedeer with a cytopathic BVDV strain of partial antigenic homology did not result in clinical signs of mucosal disease (Semrau et al., Reference Semrau, Wibbelt, Hilbe, Lieckfeldt, Hermes, Muller, Heckert, Hoyer and Frolich2008). In contrast to PI Lesser Malayan mousedeer, persistent infection may result in decreased survivability in white-tailed deer (Passler et al., Reference Passler, Walz, Ditchkoff, Brock, Deyoung, Foley and Givens2009a). This may have implications on the risk of transmission, but would also affect the ability to detect PI white-tailed deer in hunter-harvested samples that are usually collected from adult deer.
Few reports on the clinical picture and outcome of BVDV infection in wildlife exist, but reported clinical signs are similar to BVDV infections in cattle. Experimental inoculation with BVDV NY-1 did not result in clinical signs in four mule deer and one white-tailed deer fawns, despite evidence of viremia and nasal shedding (Van Campen et al., Reference Van Campen, Williams, Edwards, Cook and Stout1997). Similar findings were made in yearling elk that were inoculated either with BVDV 1 Singer or BVDV 2 24515 (Tessaro et al., Reference Tessaro, Carman and Deregt1999). In two young reindeer, loose, bloody and mucoid feces, transient laminitis, or coronitis were observed after inoculation with BVDV Singer and mild lesions were detected at necropsy (Morton et al., Reference Morton, Evermann and Dieterich1990). Naïve white-tailed deer fawns developed moderate pyrexia and marked to moderate decreases in lymphocyte populations in response to either BVDV 1 or BVDV 2; and lethargy or coughing was observed in individual fawns (Mark et al., Reference Mark, Ridpath and Chase2005; Ridpath et al., Reference Ridpath, Mark, Chase, Ridpath and Neill2007). Similar to other species, BVDV infections in wildlife may have the most important implications on reproductive health. Experimental inoculation of pregnant white-tailed deer may result in reproductive failure, including fetal resorption, fetal mummification, stillbirth and abortion (Passler et al., Reference Passler, Walz, Ditchkoff, Givens, Maxwell and Brock2007, Reference Passler, Walz, Ditchkoff, Walz, Givens and Brock2008; Ridpath et al., Reference Ridpath, Driskell, Chase, Neill, Palmer and Brodersen2008).
Summary
BVDV is one of the most important infectious agents affecting the cattle industry worldwide; however, BVDV is not host-specific to cattle as numerous wild and domestic species have been reported susceptible to BVDV infection. Infection with BVDV in heterologous species, with the potential for PI offspring, may have great implications for BVDV control in cattle populations, as these species may become reservoirs and sources of infection for cattle populations that are free from BVDV. In addition, BVDV disease and mortality in heterologous species, including rare and endangered livestock or zoo animal species, threaten natural resources and complicate laboratory testing strategies. Further research, including studies on interspecific interactions and BVDV transmission, are warranted to fully understand the importance of BVDV in heterologous species.