Introduction
If men define situations as real, they are real in their consequences.
Thomas and Thomas
The Thomas theorem (Thomas and Thomas, Reference Thomas and Thomas1928) states that our perception influences the way we act. Individuals with schizophrenia usually suffer from a distorted perception of reality and tend to perceive threat in a situation that healthy individuals perceive as non-threatening, possibly leading to an immediate sequel of aggression and violence. Escalated aggression in schizophrenia engenders numerous critical concerns, including (a) it appears as a critical public health concern and a potential threat to caregivers and mental health professionals especially during an acute phase of psychosis with the presence of positive symptoms (Hodgins et al., Reference Hodgins, Alderton, Cree, Aboud and Mak2007; Hoptman, Reference Hoptman2015). (b) Aggressive and violent behaviors evinced by individuals with schizophrenia often aggravate stigmatization of the disorder (Stuart, Reference Stuart2003; Torrey, Reference Torrey2011), which in turn reduce the tendency of affected patients to seek adequate help (Clement et al., Reference Clement, Schauman, Graham, Maggioni, Evans-Lacko, Bezborodovs, Morgan, Rüsch, Brown and Thornicroft2015). (c) Aggressive individuals with schizophrenia increase their chances of institutional admissions and prolonged hospitalization (Wehring and Carpenter, Reference Wehring and Carpenter2011). All these concerns eventually contribute to poor treatment outcomes and prognosis.
Evidence from birth cohorts (Arseneault et al., Reference Arseneault, Moffitt, Caspi, Taylor and Silva2000; Brennan et al., Reference Brennan, Mednick and Hodgins2000; Wallace et al., Reference Wallace, Mullen and Burgess2004), meta-analyses (Douglas et al., Reference Douglas, Guy and Hart2009; Fazel et al., Reference Fazel, Gulati, Linsell, Geddes and Grann2009; Large and Nielssen, Reference Large and Nielssen2011; Dack et al., Reference Dack, Ross, Papadopoulos, Stewart and Bowers2013) and epidemiological studies (Swanson et al., Reference Swanson, Swartz, Van Dorn, Elbogen, Wagner, Rosenheck, Stroup, McEvoy and Lieberman2006) have demonstrated that psychotic symptoms increase the risk of aggression and violence. Nolan et al. (Reference Nolan, Czobor, Roy, Platt, Shope, Citrome and Volavka2003) documented with a semi-structured interview that 11 out of 55 assaults in schizophrenia inpatients were directly related to positive psychotic symptoms. Supporting these findings, a subset of positive psychotic symptoms, categorized as the threat/control-override (TCO) symptoms including persecutory delusion and hallucinations of imposing thoughts or voices to harm others, was further proposed as a useful predictor of aggression and violence in schizophrenia (Link et al., Reference Link, Stueve and Phelan1998; Bjørkly, Reference Bjørkly2002). Indeed, Song and Min (Reference Song and Min2009) revealed that anger was mediating the association between aggressive expressions and positive symptoms. Such a strong negative emotion is usually provoked by a potential proximal threat to perceivers. In a healthy sample, aggressive behaviors were positively associated with psychotic-like experiences mediated by perceived threats (Fanning et al., Reference Fanning, Berman, Mohn and McCloskey2011). Altogether, these studies suggest that aggression in schizophrenia arises from a strong negative affect associated with threats, possibly due to a misperception triggered by positive symptoms (Coid et al., Reference Coid, Ullrich, Kallis, Keers, Barker, Cowden and Stamps2013). Although aggression has been linked to positive symptoms in behavioral studies (Cheung et al., Reference Cheung, Schweitzer, Crowley and Tuckwell1997), one important missing link is whether there is a common neural basis that can explain the close tie between positive symptoms and aggression in schizophrenia. Therefore, an enhanced understanding of neural abnormalities associated with aggression, particularly an impulsive and aggressive response toward threat in schizophrenia, is indispensable for the development of more preventive and effective intervention strategies.
Although violence is sometimes considered as a separate concept from aggression, most social psychologists considered violence as a subset of aggression (Allen and Anderson, Reference Allen, Anderson and Sturmey2017). For our study, therefore, we considered aggression as a board concept of overt social interaction eliciting damage while violence is an extremely physical form of aggression. In other words, being aggressive does not necessarily mean to be violent but being violent would be necessarily considered as being aggressive. Dysfunctional aggression has long been recognized as a maladaptive behavioral expression emanating from an anomalous frontolimbic socio-emotional information processing network (Davidson et al., Reference Davidson, Putnam and Larson2000; Siever, Reference Siever2008; Coccaro et al., Reference Coccaro, Fitzgerald, Lee, McCloskey and Phan2016). Failures in regulating negative emotion may channel provocation into aggression. Recent studies and reviews further acknowledge the role of consequence evaluation (i.e. evaluating rewards or benefits of the action) and decision making in elicited aggression (Blake and Grafman, Reference Blake and Grafman2004; Blair, Reference Blair2016). Individuals with intermittent explosive disorder (IED), a pathologically aggressive population, showed reduced gray matter volume in the orbitofrontal cortex, ventral medial prefrontal cortex, anterior cingulate cortex, amygdala, insula and uncus compared to their healthy or non-aggressive psychiatric counterparts (Coccaro et al., Reference Coccaro, Fitzgerald, Lee, McCloskey and Phan2016). In a healthy adolescent population, cortical thickness alterations within the middle frontal cortex, anterior cingulate cortex, lateral temporal lobe including superior, middle and inferior temporal gyri were associated with measures of aggression (Yang et al., Reference Yang, Joshi, Jahanshad, Thompson and Baker2017). These structural brain studies provided further evidence that aberrance in middle frontal and lateral temporal regions possibly contributed to impulsive aggression due to impeded socioemotional information integration and poor decision making (Shackman et al., Reference Shackman, Salomons, Slagter, Fox, Winter and Davidson2011; Vogt, Reference Vogt2016). In contrast to a large body of literature on brain abnormalities associated with aggression in general, studies on structural changes associated with aggressive behavior in schizophrenia remain scarce and their results vary (Soyka, Reference Soyka2011; Weiss, Reference Weiss2012; Hoptman, Reference Hoptman2015; Fjellvang et al., Reference Fjellvang, Grøning and Haukvik2018). The few studies available generally agree that structural abnormalities in a frontotemporal network seem to differentiate between violent and non-violent schizophrenia patients (Sandyk, Reference Sandyk1993; Hoptman et al., Reference Hoptman, Volavka, Weiss, Czobor, Szeszko, Gerig, Chakos, Blocher, Citrome, Lindenmayer, Sheitman, Lieberman and Bilder2005; Narayan et al., Reference Narayan, Narr, Kumari, Woods, Thompson, Toga and Sharma2007; Hoptman, Reference Hoptman2015; Fjellvang et al., Reference Fjellvang, Grøning and Haukvik2018).
Widespread cortical and subcortical structural abnormalities associated with positive symptoms were documented in youths and adults with schizophrenia (Gur et al., Reference Gur, Cowell, Turetsky, Gallacher, Cannon, Bilker and Gur1998; Narr et al., Reference Narr, Bilder, Toga, Woods, Rex, Szeszko, Robinson, Sevy, Gunduz-Bruce, Wang, DeLuca and Thompson2005; Ross et al., Reference Ross, Margolis, Reading, Pletnikov and Coyle2006; Satterthwaite et al., Reference Satterthwaite, Wolf, Calkins, Vandekar, Erus, Ruparel, Roalf, Linn, Elliott, Moore, Hakonarson, Shinohara, Davatzikos, Gur and Gur2016) while some cortical abnormalities were associated with positive symptoms. In a medication-free community sample, youths with high psychosis spectrum symptoms showed that global gray matter volume reduction began at an early stage of symptom manifestation (Satterthwaite et al., Reference Satterthwaite, Wolf, Calkins, Vandekar, Erus, Ruparel, Roalf, Linn, Elliott, Moore, Hakonarson, Shinohara, Davatzikos, Gur and Gur2016). Particularly, this study identified regional volume loss in the medial temporal lobe, ventromedial and orbital frontal cortex, posterior cingulate and dorsolateral prefrontal cortex. Individuals with either clinical or non-clinical auditory verbal hallucinations had thinner cortices within the pars orbitalis, paracentral lobule, fusiform gyrus and inferior temporal gyrus compared to the healthy controls (Van Lutterveld et al., Reference Van Lutterveld, Van Den Heuvel, Diederen, De Weijer, Begemann, Brouwer, Daalman, Blom, Kahn and Sommer2014). Other studies demonstrated that the severity of auditory hallucination was associated with gray matter volume of the bilateral superior temporal gyri, supramarginal gyrus, as well as middle/inferior right prefrontal gyri (Gaser et al., Reference Gaser, Nenadic, Volz, Büchel and Sauer2004; Modinos et al., Reference Modinos, Costafreda, Van Tol, McGuire, Aleman and Allen2013). Furthermore, the structural abnormalities in the lateral temporal lobe, anterior cingulate cortex and precuneus were associated with severity of formal thought disorder in schizophrenia (Horn et al., Reference Horn, Federspiel, Wirth, Müller, Wiest, Wang and Strik2009). In individuals with delusional disorder, less gray matter compared to healthy subjects was observed in the medial frontal/anterior cingulate cortex and bilateral insula (Vicens et al., Reference Vicens, Radua, Salvador, Anguera-Camós, Canales-Rodríguez, Sarró, Maristany, McKenna and Pomarol-Clotet2016).
Taken together, positive symptoms in schizophrenia are associated with structural brain abnormalities in the prefrontal cortex, cingulate cortex and lateral temporal lobe as well as the insula. Importantly, these findings indicate that there might be a common pattern of structural brain abnormalities linked to both positive symptoms and aggression. Here, we employed a prospective meta-analytic approach to investigate whether there is an overlapping pattern of brain structural changes in schizophrenia that is associated with both positive symptoms and aggression in two separate meta-regression through pooling over summary statistics computed at each research site within the framework of the ENIGMA Consortium Schizophrenia Working Group (ENIGMA-SZ; working group website: http://enigma.ini.usc.edu/ongoing/enigma-schizophrenia-working-group/). Based on the aforementioned literature, we predicted that an overlapping pattern of structural changes within the ventromedial prefrontal cortex, anterior cingulate cortex, midcingulate cortex, lateral temporal lobe and insula is associated with both positive symptoms and aggression.
Methods
Samples
A total of 902 individuals with a primary diagnosis of schizophrenia or psychosis (hereinafter ‘cases’) and 952 healthy controls (hereinafter ‘controls’) was included in the current analysis, pooling from 10 datasets with MRI scans of the brain and clinical data as part of the ENIGMA-SZ. All participating sites obtained local approval from their institutional review boards and ethics committees, and all study participants provided written informed consent. Please note that initial call of data for the present study successfully gathered 16 datasets with different scales for measuring symptoms severity for schizophrenia, including the Positive and Negative Symptom Scales (N = 11), Scale for the Assessment of Positive Symptoms (N = 4) and Brief Psychotic Symptom Scale (N = 1). Due to the inability of extracting and validating a harmonized aggression symptom score from different scales, only datasets using the Positive and Negative Symptom Scale (PANSS) were included for the current analysis. Furthermore, we excluded one dataset containing only patient data. Accordingly, the final dataset included 10 study sites worldwide (please refer to Table 1 and online Supplementary Table S1 for details).
Table 1. Demographics and clinical profiles of the case and control group (N = 1854)

a FIDMAG, FIDMAG Sisters Hospitallers Research Foundation, Spain; GAP, Genetics and Psychosis First-Episode Study, UK; COBRE, Center for Biomedical Research Excellence, USA; CAMH, The Centre for Addiction and Mental Health, Canada; FSL, Fondazione Santa Lucia, Italy; RSCZ, Mental Health Research Center, Russia; ESNA, Hospital das Clinicas HCFMUSP, Faculdade de Medicina, Universidade de Sao Paulo, Brazil; UNIBA, University of Bari ‘Aldo Moro’, Italy; ESO, National Institute of Mental Health, Czech Republic; UMCU, University Medical Center Utrecht, the Netherlands.
b SZ, schizophrenia; SZAD, schizoaffective disorder; Ps, psychosis.
c DOI, duration of illness (years).
d Aggression is calculated by the sum of the PANSS item P7 for hostility and G14 for poor impulse control.
e PS (positive symptom) score is calculated by the sum of the first 6 PANSS items for positive symptoms (P1–P6).
Positive symptoms and aggression measurements
Positive symptoms were accessed through PANSS (Kay et al., Reference Kay, Fiszbein and Opler1987). We used a composite score derived from the sum of the first six items (P1–P6) as an indicator of the severity of positive symptoms while aggression was indexed by the sum of the item P7-Hostility and item G14-Poor Impulse Control. The P7 and G14, together with P4-Excitement and G8-Uncooperativeness, contributed to a common factor, named as the excitement/hostility factor, in a 5-factor model of PANSS (van der Gaag et al., Reference van der Gaag, Hoffman, Remijsen, Hijman, de Haan, van Meijel, van Harten, Valmaggia, de Hert, Cuijpers and Wiersma2006). Especially, P7 measures verbal and nonverbal expressions of anger and resentment while this description fits the definition of impulsive aggression construct.
Image acquisition and processing
Each site acquired high-resolution (at least 1 × 1 × 1 mm voxel size) T 1-weighted structural brain scans locally. All imaging data were processed using the standardized ENIGMA analysis pipeline (see http://enigma.ini.usc.edu/protocols/imaging-protocols/ for details) to harmonize analysis and quality control processes across multiple sites (Thompson et al., Reference Thompson, Stein, Medland, Hibar, Vasquez, Renteria, Toro, Jahanshad, Schumann, Franke, Wright, Martin, Agartz, Alda, Alhusaini, Almasy, Almeida, Alpert, Andreasen, Andreassen, Apostolova, Appel, Armstrong, Aribisala, Bastin, Bauer, Bearden, Bergmann, Binder, Blangero, Bockholt, Bøen, Bois, Boomsma, Booth, Bowman, Bralten, Brouwer, Brunner, Brohawn, Buckner, Buitelaar, Bulayeva, Bustillo, Calhoun, Cannon, Cantor, Carless, Caseras, Cavalleri, Chakravarty, Chang, Ching, Christoforou, Cichon, Clark, Conrod, Coppola, Crespo-Facorro, Curran, Czisch, Deary, de Geus, den Braber, Delvecchio, Depondt, de Haan, de Zubicaray, Dima, Dimitrova, Djurovic, Dong, Donohoe, Duggirala, Dyer, Ehrlich, Ekman, Elvsåshagen, Emsell, Erk, Espeseth, Fagerness, Fears, Fedko, Fernández, Fisher, Foroud, Fox, Francks, Frangou, Frey, Frodl, Frouin, Garavan, Giddaluru, Glahn, Godlewska, Goldstein, Gollub, Grabe, Grimm, Gruber, Guadalupe, Gur, Gur, Göring, Hagenaars, Hajek, Hall, Hall, Hardy, Hartman, Hass, Hatton, Haukvik, Hegenscheid, Heinz, Hickie, Ho, Hoehn, Hoekstra, Hollinshead, Holmes, Homuth, Hoogman, Hong, Hosten, Hottenga, Hulshoff Pol, Hwang, Jack, Jenkinson, Johnston, Jönsson, Kahn, Kasperaviciute, Kelly, Kim, Kochunov, Koenders, Krämer, Kwok, Lagopoulos, Laje, Landen, Landman, Lauriello, Lawrie, Lee, Le Hellard, Lemaître, Leonardo, Li, Liberg, Liewald, Liu, Lopez, Loth, Lourdusamy, Luciano, Macciardi, Machielsen, MacQueen, Malt, Mandl, Manoach, Martinot, Matarin, Mather, Mattheisen, Mattingsdal, Meyer-Lindenberg, McDonald, McIntosh, McMahon, McMahon, Meisenzahl, Melle, Milaneschi, Mohnke, Montgomery, Morris, Moses, Mueller, Muñoz Maniega, Mühleisen, Müller-Myhsok, Mwangi, Nauck, Nho, Nichols, Nilsson, Nugent, Nyberg, Olvera, Oosterlaan, Ophoff, Pandolfo, Papalampropoulou-Tsiridou, Papmeyer, Paus, Pausova, Pearlson, Penninx, Peterson, Pfennig, Phillips, Pike, Poline, Potkin, Pütz, Ramasamy, Rasmussen, Rietschel, Rijpkema, Risacher, Roffman, Roiz-Santiañez, Romanczuk-Seiferth, Rose, Royle, Rujescu, Ryten, Sachdev, Salami, Satterthwaite, Savitz, Saykin, Scanlon, Schmaal, Schnack, Schork, Schulz, Schür, Seidman, Shen, Shoemaker, Simmons, Sisodiya, Smith, Smoller, Soares, Sponheim, Sprooten, Starr, Steen, Strakowski, Strike, Sussmann, Sämann, Teumer, Toga, Tordesillas-Gutierrez, Trabzuni, Trost, Turner, Van den Heuvel, van der Wee, van Eijk, van Erp, van Haren, van ’t Ent, van Tol, Valdés Hernández, Veltman, Versace, Völzke, Walker, Walter, Wang, Wardlaw, Weale, Weiner, Wen, Westlye, Whalley, Whelan, White, Winkler, Wittfeld, Woldehawariat, Wolf, Zilles, Zwiers, Thalamuthu, Schofield, Freimer, Lawrence and Drevets2014). The images were analyzed using the fully automated and validated segmentation on FreeSurfer (Fischl et al., Reference Fischl, Salat, Busa, Albert, Dieterich, Haselgrove, Van Der Kouwe, Killiany, Kennedy, Klaveness, Montillo, Makris, Rosen and Dale2002). Segmentations of 68 (34 per each hemisphere) cortical gray matter regions of interest (ROIs) were created based on the Desikan–Killiany atlas (Desikan et al., Reference Desikan, Ségonne, Fischl, Quinn, Dickerson, Blacker, Buckner, Dale, Maguire, Hyman, Albert and Killiany2006). Segmented regions were visually inspected and statistically evaluated through histogram plots for outliers.
Prospective meta-analyses and meta-regression
Analyses were conducted in R, version 3.4.1 (R Core Team, 2003). The outcome measures were standardized mean difference (SMD) between cases and controls from each of the 68 cortical regions of interest (ROIs). SMDs were computed by ‘compute.es’ package (Del Re, Reference Del Re2013) at each site using the Hedges' g metric that corrected for biased upward for sites with a smaller sample size. The intercept in the models represented the estimation of the average effect size of group differences (i.e. cases v. control). Effects sizes of SMDs per each ROI were analyzed using this brain structural modality with meta-regression models using the ‘rma’ function from the ‘Metafor’ package (Viechtbauer, Reference Viechtbauer2010) and a restricted-maximal likelihood method. This function fits the meta-analytic mixed-effects regression model with regressors and covariates:

We then conducted two primary meta-regression models to study the association of positive symptoms and aggression with the SMDs of cortical thickness and surface areas in each ROI:


In these models, all βs are standardized. β 0 represents the intercept: the effect sizes of the difference between cases and controls while β 1 is the standard coefficient of our main outcomes. We set sex and age of the participants as the covariates (β c1 and β c2) of the models. All p values were corrected for multiple testing using false discovery rate (FDR) with a Benjamini–Hochberg procedure limited at 5% (i.e. p FDR < 0.05) for 68 ROIs (Benjamini and Hochberg, Reference Benjamini and Hochberg1995). In addition, we calculated heterogeneity scores (I 2) for each ROI. I 2 indicates the percent of the total variance in effect size explained by heterogeneity alone. Thus, lower values of I 2 represent lower variance in the effect size estimation across study sites.
Results
Demographics and clinical characteristics
The demographic and clinical characteristics of 1854 subjects (902 cases and 952 healthy controls) are summarized in Table 1 for each site. Weighted mean age across individuals with schizophrenia was 34.94 (range: 22.16–44.46). Within the schizophrenia group, 27.71% of the subjects (range: 0–52.08%) were female and their duration of illness was 12.87 years (range: 0.51–19.41). The mean score of positive symptoms (i.e. sum of P1–P6) was 14.54 (range: 10.09–18.83) and the mean score of aggression symptom (i.e. sum of P7 and G14) was 3.19 (range: 2.68–4.77). Weighted mean age across healthy controls was 32.70 (range: 22.41–43.60) and 44.67% of participants (range: 0–62.50%) were female.
Meta-analysis: cortical differences between cases and controls
No regional difference in surface areas between cases and controls was found. However, we observed cortical thinning in the case group compared to their healthy controls. ROI analysis revealed significant cortical thinning (psFDR < 0.05) in the lateral orbitofrontal cortex bilaterally, left medial orbitofrontal, bilateral pars opercularis, bilateral pars orbitalis, bilateral pars triangularis, bilateral precuneus, bilateral rostral middle frontal gyrus, left superior frontal gyrus, right caudal middle frontal gyrus and right posterior cingulate gyrus. Most brain regions have a low-to-median level of unexplained variances (I 2: 10–40%). This means that after controlling for sex and age, group differences (diagnosis of schizophrenia) can explain around 60–90% of variances of the differences. Details are displayed in Table 2.
Table 2. Full meta-analytic results for thickness of brain regions of interests for cases v. controls comparison controlling for age and sex. Only significant regions are displayed

Meta-regression: association between symptoms and structural changes
Since no significant differences of cortical surface areas were found between the schizophrenia patients and the healthy control group, its association with symptoms was not examined. We examined whether positive symptoms and aggression were significantly associated with the difference of cortical thickness in each ROI in two separate meta-regression models. For positive symptoms (see Fig. 1a and Table 3a), significant (psFDR < 0.05) associations between symptom severity and SMDs were documented within the bilateral medial orbitofrontal gyrus (MOFG; left β 1 = 0.064, right β 1 = 0.89), right caudal anterior cingulate cortex (cACC; β 1 = 0.09), left inferior (ITG; β 1 = 0.075) and middle temporal gyrus (MTG; β 1 = 0.091). For aggression (see Fig. 1b and Table 3b), significant (psFDR < 0.05) associations between symptom severity and SMDs were observed within the bilateral rACC (left β 1 = 0.287, β 1 = 0.229), right insula (β 1 = 0.237) right cACC (β 1 = 0.266), left ITG (β 1 = 0.22) and left MTG (β 1 = 0.23). By comparing two association patterns, we found that both positive and aggression symptoms were associated with differences of cortical thickness between schizophrenia and their healthy controls within the right midcingulate cortex (MCC), left MTG and ITG. The heterogeneity indices showed that with group differences (diagnosis of schizophrenia), age, sex and symptom severity, the meta-regression models explained most variances (>90%) except medial orbitofrontal gyrus (unexplained variances: 51.76%) in the positive symptom meta-regression model and insula (unexplained variances: 24.36%) in the aggression meta-regression model.
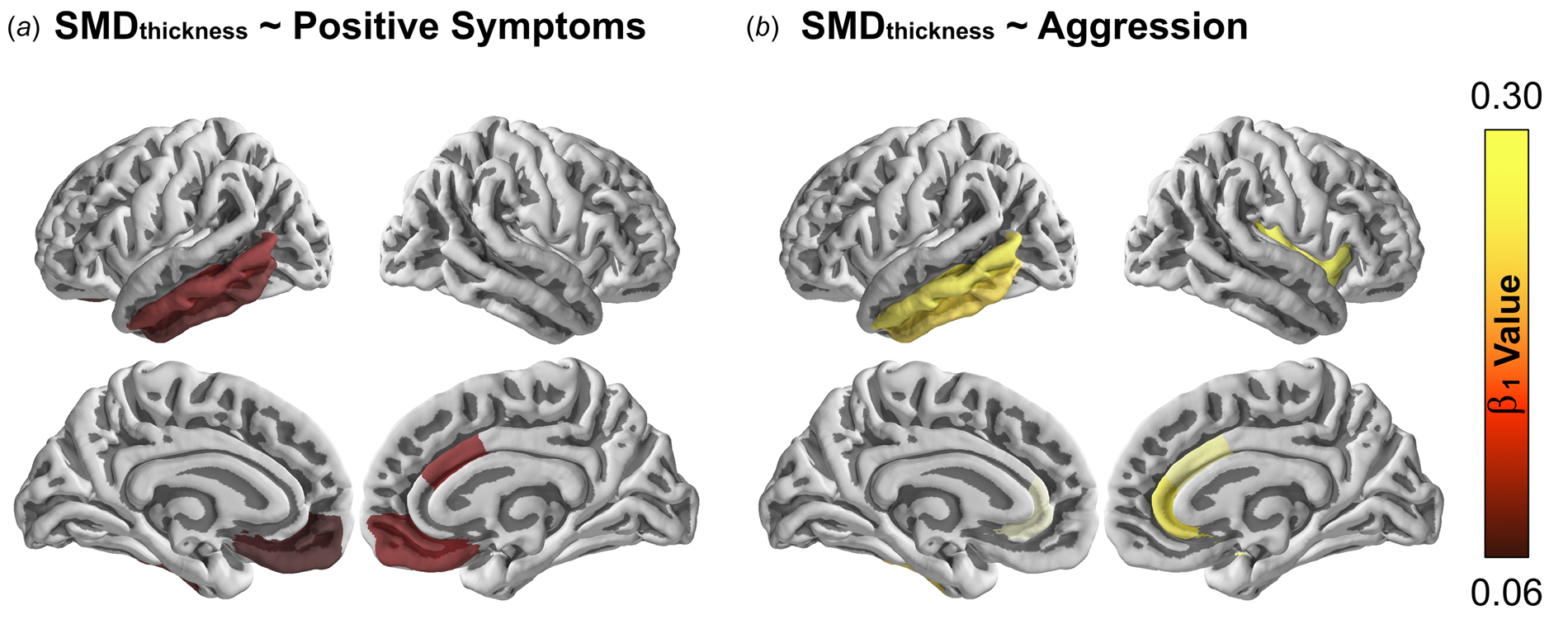
Fig. 1. Moderation effects on regional cortical thinning controlled for sex and age. The right color bar represents the standardized beta coefficients on the cortical thinning between the control group compared to the case group. Those beta coefficients with an FDR adjusted p value higher than 0.05 was set to 0 for a clearer visual inspection. The surface brain is visualized by PySurfer (version 0.9.0; https://pysurfer.github.io/index.html). The color figure can refer to the online version of this paper.
Table 3. Effects of moderators controlling for sex and age on significant cortical thinning in individuals with schizophrenia compared to their healthy controls

Discussion
With a prospective meta-analytic approach, we investigated structural neural integrity in a large sample of individuals with schizophrenia compared to healthy controls. Reduced cortical thickness, but not surface area, was observed in our sample within the lateral and medial orbitofrontal cortex, inferior frontal gyrus, cingulate cortex, lateral temporal lobe and insula. These results replicate previous findings (Fornito et al., Reference Fornito, Yücel, Dean, Wood and Pantelis2009; Schultz et al., Reference Schultz, Koch, Wagner, Roebel, Nenadic, Schachtzabel, Reichenbach, Sauer and Schlösser2010; Nenadic et al., Reference Nenadic, Yotter, Sauer and Gaser2015; Satterthwaite et al., Reference Satterthwaite, Wolf, Calkins, Vandekar, Erus, Ruparel, Roalf, Linn, Elliott, Moore, Hakonarson, Shinohara, Davatzikos, Gur and Gur2016; van Erp et al., Reference van Erp, Walton, Hibar, Schmaal, Jiang, Glahn, Pearlson, Yao, Fukunaga, Hashimoto, Okada, Yamamori, Bustillo, Clark, Agartz, Mueller, Cahn, de Zwarte, Hulshoff Pol, Kahn, Ophoff, van Haren, Andreassen, Dale, Doan, Gurholt, Hartberg, Haukvik, Jørgensen, Lagerberg, Melle, Westlye, Gruber, Kraemer, Richter, Zilles, Calhoun, Crespo-Facorro, Roiz-Santiañez, Tordesillas-Gutiérrez, Loughland, Carr, Catts, Cropley, Fullerton, Green, Henskens, Jablensky, Lenroot, Mowry, Michie, Pantelis, Quidé, Schall, Scott, Cairns, Seal, Tooney, Rasser, Cooper, Shannon Weickert, Weickert, Morris, Hong, Kochunov, Beard, Gur, Gur, Satterthwaite, Wolf, Belger, Brown, Ford, Macciardi, Mathalon, O'Leary, Potkin, Preda, Voyvodic, Lim, McEwen, Yang, Tan, Tan, Wang, Fan, Chen, Xiang, Tang, Guo, Wan, Wei, Bockholt, Ehrlich, Wolthusen, King, Shoemaker and Sponheim2018). More importantly, within these regions with thinning, the right MCC, left MTG and ITG were positively associated with both positive symptoms and aggression, indicating that the severity of these symptoms is associated with these differences in cortical thickness between schizophrenia patients and their healthy controls.
Language, sources of threats and the lateral temporal lobe
The lateral temporal lobe is implicated in language and semantic memory processing (Levy et al., Reference Levy, Bayley and Squire2004) as well as multimodal sensory integration (Mesulam, Reference Mesulam1998). Brain damage in this region leads to impairments of auditory perception and language abilities (Catani et al., Reference Catani, Dell'Acqua, Bizzi, Forkel, Williams, Simmons, Murphy and Thiebaut de Schotten2012). Structural abnormalities within the lateral temporal lobe have been associated with positive symptoms, especially auditory hallucination, in schizophrenia patients (Kuperberg et al., Reference Kuperberg, Broome, McGuire, David, Eddy, Ozawa, Goff, West, Williams, van der Kouwe, Salat, Dale and Fischl2003; Onitsuka et al., Reference Onitsuka, Shenton, Salisbury, Dickey, Kasai, Toner, Frumin, Kikinis, Jolesz and McCarley2004; Kuroki et al., Reference Kuroki, Shenton, Salisbury, Hirayasu, Onitsuka, Ersner-Hershfield, Yurgelun-Todd, Kikinis, Jolesz and McCarley2006; Allen et al., Reference Allen, Modinos, Hubl, Shields, Cachia, Jardri, Thomas, Woodward, Shotbolt, Plaze and Hoffman2012). Furthermore, a recent meta-analysis pooling over fMRI studies provided convergent evidence that superior temporal gyrus (STG) and MTG were linked to disorganized and incoherent speech (i.e. thought disorder; Wensing et al., Reference Wensing, Cieslik, Müller, Hoffstaedter, Eickhoff and Nickl-Jockschat2017). These studies implicate that the lateral temporal lobe is critical for impairments in formal thought processes and auditory misperception in schizophrenia. Similarly, IED individuals have lower gray matter volume not only in frontolimbic regions but also in the ITG and STG, compared to non-IED patients with a similar psychiatric profile and healthy controls (Coccaro et al., Reference Coccaro, Fitzgerald, Lee, McCloskey and Phan2016). Another study focusing on white matter reported that IED was associated with lower white matter integrity in the superior longitudinal fasciculus that has a role in executive functions, visuospatial working memory and language (Lee et al., Reference Lee, Arfanakis, Evia, Fanning, Keedy and Coccaro2016). Behaviorally, lower verbal abilities in adolescent boys with serious conduct problems predicted later life violence (Manninen et al., Reference Manninen, Lindgren, Huttunen, Ebeling, Moilanen, Kalska, Suvisaari and Therman2013). In a population-based longitudinal study, children with poor language abilities showed more physical aggression (Girard et al., Reference Girard, Pingault, Falissard, Boivin, Dionne and Tremblay2014). Consistent with our findings, this data suggests that language abilities associated with the lateral temporal lobe might play a key role in both aggression and positive symptoms. One can speculate that the lateral temporal lobe abnormalities in schizophrenia become a potential trigger for aggression because of impaired cognitive abilities – which, in turn, will negatively influence coping strategies and perceived threats due to positive symptoms like auditory misperceptions. Failures in utilizing language as an effective method to resolve conflicts further increase the likelihood of overreacting or even aggressive responses in a non-threatening situation.
Cingulate cortex, auditory hallucination and cognitive control
Thinner cortices in schizophrenia were associated with both positive symptoms and aggression in the right midcingulate cortex (MCC). The cingulate cortex is part of the limbic system and densely connected to the prefrontal cortex (Devinsky et al., Reference Devinsky, Morrell and Vogt1995). The cingulate cortices are implicated in functions including emotion regulation, motivation, conflict monitoring, error detecting and cognitive control. In a review, Etkin et al. (Reference Etkin, Egner and Kalisch2011) proposed that the rostral anterior cingulate cortex (ACC) together with dorsomedial prefrontal cortex (dmPFC) are involved in emotion regulation while the MCC together with supplementary motor area (SMA) and pre-SMA are involved in reappraisal and expression. A thinner MCC may be associated with impairment in information integration and generating adaptive responses (Shackman et al., Reference Shackman, Salomons, Slagter, Fox, Winter and Davidson2011; Hoffstaedter et al., Reference Hoffstaedter, Grefkes, Zilles and Eickhoff2013, Reference Hoffstaedter, Grefkes, Caspers, Roski, Palomero-Gallagher, Laird, Fox and Eickhoff2014). For example, violent schizophrenia patients demonstrated ACC hyperactivations compared to their non-violent or healthy counterparts when viewing negative pictures (Tikàsz et al., Reference Tikàsz, Potvin, Lungu, Joyal, Hodgins, Mendrek and Dumais2016). Aberrant activities of cingulate cortex and structural changes are often reported to be linked to emotion-related negative symptoms (Bersani et al., Reference Bersani, Minichino, Fojanesi, Gallo, Maglio, Valeriani, Biondi and Fitzgerald2014) while other studies discovered the auditory hallucinations were associated with ACC (Gleghorn et al., Reference Gleghorn, Garnett, Nahmias, Brown, Kaplan, Szechtman, Szechtman, Franco, Dermer and Cook1990; Noga et al., Reference Noga, Aylward, Barta and Pearlson1995). Particularly, a study had shown that the caudal ACC (i.e. midcingulate cortex) was recruited only when the healthy subjects heard either a real or hallucinated stimulus but not imagination (Szechtman et al., Reference Szechtman, Woody, Bowers and Nahmias1998). It is noteworthy that structural abnormalities within the cingulate cortex are not specific to auditory hallucination in schizophrenia. IED individuals also displayed reduced gray matter volume within the cingulate cortex (Coccaro et al., Reference Coccaro, Fitzgerald, Lee, McCloskey and Phan2016). The transdiagnostic structural abnormalities suggest that the cingulate cortex serves more general cognitive control processes including emotion regulation, self-regulation, socioemotional information integration and decision making. Thinner midcingulate cortices in schizophrenia may lead to confusion in evaluating a non-threatening situation (e.g. positive symptoms) and impairment in controlling inappropriate responses (e.g. aggression) (Shackman et al., Reference Shackman, Salomons, Slagter, Fox, Winter and Davidson2011; Vogt, Reference Vogt2016).
From misperception to aggression in schizophrenia
Previous studies showed that TCO symptoms increase the risk of violent behaviors in schizophrenia (Fanning et al., Reference Fanning, Berman, Mohn and McCloskey2011). A certain degree of variation in aggressive traits of schizophrenia patients can be explained by positive symptoms, suggesting an at least partially shared neurobiological basis between aggression and positive symptoms. Our results demonstrated that there is an overlapping pattern of thinner cortical thickness associated with both positive symptoms and aggression. Impaired structural integrity of the lateral temporal lobe may lead to auditory misperception in schizophrenia. The impaired midcingulate cortex may, in turn, not be able to suppress or channel such perceived threats, increasing the likelihood of aggressive expressions. This could offer a neurobiological explanation for why a subset of positive symptoms (i.e. TCO symptoms) may be a useful predictor of aggression. However, an important question whether both positive symptoms and aggression are moderated by a common cognitive factor or aggression is moderated or mediated by positive symptoms remains unanswered. Future studies should address this question and also focus on a specific assessment of TCO symptoms.
Surprisingly, we did not observe any structural abnormalities within prefrontal regions associated with aggression. These regions were deemed to be an important hub for a top-down ‘brake’ of initiated responses and proposed in many neural models of human aggression (Davidson et al., Reference Davidson, Putnam and Larson2000; Blair, Reference Blair2010, Reference Blair2016; Coccaro et al., Reference Coccaro, Sripada, Yanowitch and Phan2011). This could be due to reduced power since our aggression score was only indexed by two items in the PANSS. Especially the use of the item ‘poor impulse control’ may bias our findings toward reactive aggression (i.e. impulsive aggressive response to an immediate threat) and against proactive aggression (i.e. being aggressive instrumentally to obtain a desirable outcome). Please note that although reactive aggression is comparatively well studied, little is known about the neural correlates of proactive aggression (Wrangham, Reference Wrangham2018). At least regarding structural brain anomalies, a clear difference between reactive and proactive aggression has not been found so far (Yang et al., Reference Yang, Joshi, Jahanshad, Thompson and Baker2017). Still, indirect evidence links proactive aggression to prefrontal regions. For example, a study found that applying non-invasive brain stimulation to the right dorsolateral prefrontal cortex reduced proactive aggressive behavior in male participants (Dambacher et al., Reference Dambacher, Schuhmann, Lobbestael, Arntz, Brugman and Sack2015). The General Aggression Model (Anderson and Bushman, Reference Anderson and Bushman2002; Allen et al., Reference Allen, Anderson and Bushman2018) proposed that a reappraisal process is critical in a thoughtful outcome (even an aggressive one). Given that proactive aggression requires a higher level of cognitive abilities such as self-regulation and reappraisal, abnormalities in the ventral medial prefrontal cortex (Etkin et al., Reference Etkin, Egner and Kalisch2011) may play a role too. Shifting the focus toward proactive aggression may indeed lead toward a characterization of anomalies within prefrontal regions. Furthermore, measuring and observing aggression and/or impulsivity is certainly a challenge for research, as respective studies hardly measure acts of aggression per se, but usually rely rather on proxy measures, such as rating scales, etc. In the present study, the differences in cortical thickness within the ventral medial prefrontal cortex were only moderated by positive symptoms.
Heterogeneity of aggression and clinical manifestations in schizophrenia
Aggression in schizophrenia is a multifaceted construct attributed to various factors (Blake and Grafman, Reference Blake and Grafman2004; Volavka and Citrome, Reference Volavka and Citrome2008, Reference Volavka and Citrome2011; Hoptman, Reference Hoptman2015), including personal dispositions (e.g. personality, genetics and epigenetics), life-history (e.g. childhood adversities, exposure to violence and socioeconomic status), clinical manifestations (e.g. schizophrenia symptomatology and comorbidity with substance misuse disorders or others) and treatment (e.g. cumulative antipsychotic dosage). In particular, comorbidity, such as substance abuse (Fazel et al., Reference Fazel, Gulati, Linsell, Geddes and Grann2009) in schizophrenia increases the risk of violence by more than 2-fold (Arseneault et al., Reference Arseneault, Moffitt, Caspi, Taylor and Silva2000). The current study design did not allow us to clarify the developmental trajectory of aggression in schizophrenia. The differences in cortical thickness in schizophrenia associated with both positive symptoms and aggression might indicate that these regions are the critical hubs that are severely influenced by these abovementioned factors. Particularly, regions like the medial orbitofrontal gyrus and insula showed relatively higher unexplained variances after addressing sex, age and symptom severity. Future studies could examine whether other factors, such as dosage of antipsychotic drugs or a history of substance abuse, could explain these variances. Similar to aggression, schizophrenia is often described as a heterogeneous disorder while individual with schizophrenia may present clinical presentations differently (Tsuang et al., Reference Tsuang, Lyons and Faraone1990). Data collected across the globe also enhance heterogeneity. As mentioned above, clinical manifestations could contribute to expression of aggression. Therefore, future studies could investigate the effect of diagnostic heterogeneity on aggression.
Limitations of generalization and methodological considerations
This study encounters several limitations related to generalization. First, our operationalization of aggression through using only two items (i.e. hostility and poor impulse control) from the PANSS might bias our findings. The concept of aggression may not be fully covered by only using these two items. Future study could employ a comprehensive scale like Buss–Perry Aggression Questionnaire (Buss and Perry, Reference Buss and Perry1992) to measure aggression. Second, the current symptom scores relied on observation from medical clinicians. A multi-perspective measurement, adding self-reports and reports from close friends or relatives, could offer a complete profile of the participants. Another issue is that the current study does not have an actual measurement of clinical characteristics of healthy controls. In the analysis, healthy controls were assumed to have no manifestation of any positive symptoms and aggression. However, it is likely that some healthy subjects would have subclinical positive symptoms or elevated aggressive traits. However, with a large sample in this study the majority of healthy participants should score 0 in most of the symptom items, while only a very small portion of them could be reasonably expected score high in some of them. Thus, the results should not be biased significantly, as outliers within the healthy population should average out due to the small sample size. Though, we acknowledge the lack of available scores for healthy controls as a limitation of this study.
This study also encounters some methodological considerations. First, confounding variables like the FreeSurfer version and working environment might affect the results of parcellation although other published studies within the Schizophrenia Working Group using a similar dataset did not document the effect of FreeSurfer version and working environment on the structural difference across study sites (van Erp et al., Reference van Erp, Hibar, Rasmussen, Glahn, Pearlson, Andreassen, Agartz, Westlye, Haukvik, Dale, Melle, Hartberg, Gruber, Kraemer, Zilles, Donohoe, Kelly, McDonald, Morris, Cannon, Corvin, Machielsen, Koenders, de Haan, Veltman, Satterthwaite, Wolf, Gur, Gur, Potkin, Mathalon, Mueller, Preda, Macciardi, Ehrlich, Walton, Hass, Calhoun, Bockholt, Sponheim, Shoemaker, van Haren, Pol, Ophoff, Kahn, Roiz-Santiañez, Crespo-Facorro, Wang, Alpert, Jönsson, Dimitrova, Bois, Whalley, McIntosh, Lawrie, Hashimoto, Thompson and Turner2016). Second, similar to retrospective meta-analyses, our approach is limited by the availability of information. Although the brain imaging protocol was synchronized, the availability of clinical information still varies. Missing data like duration of illness and information on actual and past medication impaired the power of the current analyses. However, the effect sizes in our models after controlling sex and age as well as the symptom severity could be explained by a relative low heterogeneity alone, indicating lower variance in the effect size estimation across sites in different brain ROIs. Third, we should interpret these results with caution since the biological nature of cortical thickness is still unclear, but it is considered to reflect the integrity of cortical neurons. Since the organization of the cortical thickness network showed vigorous small-world properties (He et al., Reference He, Chen and Evans2007), a thinner cortex may indicate poorer neuronal connectivity. Finally, meta-regression analyses must be interpreted with caveats because of possible confounds such as Yule-Simpson's Paradox (Goltz, Reference Goltz2010). However, it is still useful for hypothesis generation for future studies.
Conclusions
An overlapping pattern of thinner cortical thickness in the left lateral temporal lobe and right midcingulate cortex between schizophrenia and their healthy controls was moderated by both positive symptoms and aggression, providing neurobiological evidence to elucidate the link between these symptoms. Our findings suggested that a portion of aggressive behaviors in schizophrenia can be explained by loss of tissue integrity in regions related to positive symptoms such as formal thought disorder and auditory misperception, and cognitive impairments reflecting the difficulties to deploy an adaptive reaction toward perceived threats. Follow-up studies are necessary to address issues such as heterogeneity and medication effect.
Supplementary material
The supplementary material for this article can be found at https://doi.org/10.1017/S0033291719002149.
Acknowledgement
ENIGMA was supported in part by a Consortium grant (U54 EB020403) from the NIH Institutes contributing to the Big Data to Knowledge (BD2K) Initiative, including the NIBIB and NIMH. This study is also supported by Deutsche Forschungsgemeinschaft (DFG, International Research Training Group IRTG2150). Acknowledgments for the various participating data contributors are listed in the Appendix.
Conflict of interest
All authors have no conflict of interest related to this study.
Appendix
ENIGMA Schizophrenia Working Group: authors’ affiliations and their acknowledgment
Joaquim Radua1,2,3,4,5, Edith Pomarol-Clotet1,2, Raymond Salvador1,2, Anton Albajes-Eizagirre1,2, Aleix Solanes1,3, Erick J. Canales-Rodriguez1,2,6,7, Amalia Guerrero-Pedraza8 and Salvador Sarro1,2
1FIDMAG Germanes Hospitalàries, Sant Boi de Llobregat, Barcelona, Spain
2Mental Health Research Networking Center (CIBERSAM), Madrid, Spain
3Institut d'Investigacions Biomediques August Pi i Sunyer (IDIBAPS), Barcelona, Spain
4Department of Psychosis Studies, Institute of Psychiatry, Psychology and Neuroscience, King's College London, London, UK
5Centre for Psychiatric Research and Education, Department of Clinical Neuroscience, Karolinska Institutet, Stockholm, Sweden
6Department of Radiology, Centre Hospitalier Universitaire Vaudois (CHUV), Lausanne, Switzerland
7Signal Processing Lab (LTS5), École Polytechnique Fédérale de Lausanne, Lausanne, Switzerland
8Benito Menni Complex Assistencial en Salut Mental, Sant Boi de Llobregat, Spain
Acknowledgment: This work was supported by the Catalan Government (2017-SGR-1271 to FIDMAG) and several grants from the Plan Nacional de I+D+i 2008–2011 and 2013–2016, and the Instituto de Salud Carlos III and co-funded by European Union (ERDF/ESF, ‘Investing in your future’): Miguel Servet Research Contracts (MS14/00041 to JR, CPII13/00018 to RS and CPii16/00018 to EP-C) and Research Project Grants (PI14/01151 to RS, PI14/01148 to EP-C, PI14/00292 and CP14/00041 to JR, and PI15/00277 to EC-R).
Tilo Kircher1, Igor Nenadic1, Axel Krug1, Dominik Grotegerd2 and Udo Dannlowski2
1University of Marburg, Department of Psychiatry and Psychotherapy, Marburg, Germany
2University of Münster, Department of Psychiatry, Marburg, Germany
Acknowledgment: This work was funded by the German Research Foundation (DFG, grant FOR2107 KI 588/14-1, KI 588/14-2, KI 588/17-1 to TK; grant KR 3822/7-1, KR 3822/7-2 to AK, grant NE 2254/1-2 to IN; grant DA1151/5-1, DA1151/5-2 to UD; SFB-TRR58, Projects C09 and Z02 to UD) and the Interdisciplinary Center for Clinical Research (IZKF) of the medical faculty of Münster (grant Dan3/012/17 to UD).
Stefan Borgwardt1, Anita Riecher-Rössler1, Andre Schmidt1, Christina Andreou1 and Christian Huber1
1Department of Psychiatry (UPK), University of Basel, Basel, Switzerland
Acknowledgment: We would like to thank the UPK Forschungsförderungsfonds for grant support.
Jessica Turner1, Vince Calhoun2,3, Wenhao Jiang1, Sarah Clark1 and Esther Walton4
1Department of Psychology, Georgia State University, Atlanta, Georgia, USA
2The Mind Research Network, Albuquerque, NM, USA
3Department of Electrical and Computer Engineering, University of New Mexico, Albuquerque, NM, USA
4Department of Psychology, University of Bath, Bath, UK
Acknowledgment: The imaging data and phenotypic information were collected and shared by the Mind Research Network and the University of New Mexico funded by a National Institute of Health Center of Biomedical Research Excellence (COBRE) grant 1P20RR021938-01A2.
Gianfranco Spalletta1,2, Nerisa Banaj1, Fabrizio Piras1, Valentina Ciullo1,3 and Daniela Vecchio1
1Laboratory of Neuropsychiatry, Department of Clinical and Behavioral Neurology, IRCCS Santa Lucia Foundation, Rome, Italy
2Division of Neuropsychiatry, Menninger Department of Psychiatry and Behavioral Sciences, Baylor College of Medicine, Houston, TX, USA
3Department of Neurosciences, Psychology, Drug Research and Child Health (NEUROFARBA), University of Florence, Italy
Acknowledgment: This work was supported by the Italian Ministry of Health grants RC 12-13-14-15-16-17-18-19/A.
Irina Lebedeva1, Alexander S. Tomyshev1, Vasily Kaleda1 and Tatyana Klushnik1
1Mental Health Research Center, Moscow, Russia
Geraldo Busatto Filho1, Marcus Vinicius Zanetti1,2, Mauricio Henriques Serpa1 and Pedro Gomes Penteado Rosa1
1Laboratory of Psychiatric Neuroimaging (LIM-21), Departamento e Instituto de Psiquiatria, Hospital das Clinicas HCFMUSP, Faculdade de Medicina, Universidade de Sao Paulo, Sao Paulo, SP, Brazil
2Instituto de Ensino e Pesquisa, Hospital Sírio-Libanês, Sao Paulo, SP, Brazil
Ryota Hashimoto1,2 and Masaki Fukunaga3
1Department of Pathology of Mental Diseases, National Institute of Mental Health, National Center of Neurology and Psychiatry, Tokyo, Japan
2Osaka University, Osaka, Japan
3Division of Cerebral Integration, National Institute for Physiological Sciences, Aichi, Japan
Anja Richter1,2, Bernd Krämer1 and Oliver Gruber1
1Section for Experimental Psychopathology and Neuroimaging, Department of General Psychiatry, University Hospital Heidelberg, 69115 Heidelberg, Germany
2Department of Psychosis Studies, Institute of Psychiatry, Psychology and Neuroscience, King's College London, London, UK
Aristotle N. Voineskos1,2 and Erin W. Dickie1,2
1Addiction and Mental Health, Toronto, Canada
2Department of Psychiatry, University of Toronto, Toronto, Canada
Acknowledgment: The CAMH datasets were collected and shared with support from the CAMH Foundation, Canadian Institutes of Health Research, the Ontario Mental Health Foundation and the Brain and Behavior Research Foundation.
David Tomecek1,2,3, Antonin Skoch1,4, Filip Spaniel1, Cyril Hoschl1
1National Institute of Mental Health, Klecany, Czech Republic
2Institute of Computer Science, Czech Academy of Sciences, Czech Technical University in Prague, Prague, Czech Republic
3Faculty of Electrical Engineering, Czech Technical University in Prague, Prague, Czech Republic
4MR Unit, Department of Diagnostic and Interventional Radiology, Institute for Clinical and Experimental Medicine, Prague, Czech Republic
Acknowledgment: The National Institute of Mental Health, Klecany, Czech Republic was supported by project no. LO1611 with funding from the MEYS under the NPU I program.
Alessandro Bertolino1, Aurora Bonvino1 and Annabella Di Giorgio2
1Department of Basic Medical Science, Neuroscience and Sense Organs, University of Bari ‘Aldo Moro’, Italy
2Section of Clinical Neuroscience, Department of Experimental and Clinical Medicine, Università Politecnica delle Marche, Ancona, Italy
Laurena Holleran1
1Neuroimaging and Cognitive Genomics Group, NUI Galway, Ireland
Simone Ciufolini1, Tiago Reis Marques1, Paola Dazzan1 and Robin Murray1
1Department of Psychosis Studies, Institute of Psychiatry, Psychology and Neuroscience, King's College London, London, UK
Jelle Lamsma1,2, Wiepke Cahn1,3 and Neeltje van Haren1,4
1Department of Psychiatry, University Medical Centre Utrecht, Utrecht, The Netherlands
2Department of Psychiatry, University of Oxford, Oxford, UK
3Utrecht Mental Health Institute, Utrecht, The Netherlands
4Department of Child and Adolescent Psychiatry/Psychology, Erasmus MC-Sophia Children's Hospital, Rotterdam, The Netherlands
Ana M. Díaz-Zuluaga1, Julián A. Pineda-Zapata2, Cristian Vargas1 and Carlos López-Jaramillo1,3
1Research Group of Psychiatry GIPSI, Department of Psychiatry, Faculty of Medicine, Universidad de Antioquia, Medellín, Colombia
2Research Group, Instituto de Alta Tecnología Médica (IATM), Medellín, Antioquia, Colombia
3Mood Disorders Program, Hospital Universitario San Vicente Fundación, Medellín, Colombia
Theo G. M. van Erp1,2
1Clinical Translational Neuroscience Laboratory, Department of Psychiatry and Human Behavior, University of California, Irvine, California, USA
2Center for the Neurobiology of Learning and Memory, University of California Irvine, Irvine, CA 92697, USA
Acknowledgement: TGMvE is supported by the ENIGMA's NIH Big Data to Knowledge (BD2K) initiative U54 EB020403, and the ENIGMA Sex Differences Initiative R01 MH116147.