Significant outcomes
Driving while intoxicated tends to re-occur.
NPSR1 T-allele carriers were more prone to re-occurrence of drunk driving.
DAT1 9R carriers had more traffic accidents than 10R homozygotes.
Limitations
The longitudinal design has set limits to the size of the sample and reduces reliability of the findings in stratified analyses.
Sample size also prevents consideration of dependency of significant relationships on age and driving experience.
Introduction
More than 1.2 million lives are lost each year due to road traffic injuries worldwide (1). Behaviour of drivers contributes to 90–95% of motor vehicle crashes (Reference Evans2). Driving while impaired by alcohol (DWI) is among the key behavioural risk factors in traffic (Reference Hels, Lyckegaard, Simonsen, Steentoft and Bernhoft3). DWI is a high-risk behaviour that is not caused only by drinking problems but influenced by concurrent basic behavioural tendencies, temperament and personality (Reference Stacy, Newcomb and Bentler4). DWI offenders have been shown to have higher rates of alcohol use disorders, and indeed most of them meet the criteria of alcohol dependence some time during their life (Reference Lapham, Smith, C’de Baca, Chang, Skipper, Baum and Hunt5).
Due to riskier environment, mistakes that accompany impulsive behaviour tend to be more dangerous in traffic, compared to other aspects of our daily lives. While risk-taking may be necessary in some situations, it can be dangerous and inappropriate in others. Dickman (Reference Dickman6) distinguished between functional (adaptive) and dysfunctional (maladaptive) impulsivity, first being the willingness and ability to take risks in situations where it is mostly appropriate, and the second expressing the tendency for thoughtlessness and inability to plan, leading to negative consequences. The concept of functional and dysfunctional impulsivity applied to traffic has suggested that different types of violations go with distinct aspects of impulsivity: While speeding has been found to be associated more with adaptive impulsivity, DWI was mostly associated with maladaptive impulsivity (Reference Eensoo, Paaver, Pulver, Harro and Harro7,Reference Paaver, Eensoo, Pulver and Harro8).
Obviously, individual biological predispositions should play a role in risky driving behaviour, but while there is a vast literature on the neurobiology of impulsivity little is known on how such findings apply to everyday behaviours involving higher risk. We have previously found that drunk drivers had lower platelet monoamine oxidase (MAO) activity (Reference Eensoo, Paaver, Pulver, Harro and Harro7,Reference Eensoo, Paaver, Harro and Harro9). While dopamine is the preferred substrate for the MAO-B isoenzyme that is the form present in platelets, platelet MAO activity is a reliable peripheral marker of serotonergic activity in the CNS, and low levels of platelet MAO activity have been associated with social maladaptation, impulsivity, sensation seeking and monotony avoidance (Reference von Knorring, Oreland and Winblad10–Reference Oreland12). Platelet MAO activity is also lower in alcohol-dependent subjects (Reference von Knorring and Oreland13) and low-platelet MAO is common in victims of severe trauma (Reference Sabre, Harro, Eensoo, Vaht, Kabel, Pakkanen, Asser and Kõrv14). Furthermore, low-platelet MAO activity has also been associated with criminal behaviour and suicidality, especially in adolescents who come from an unfavourable psychosocial environment (Reference Stalenheim15–Reference Jokinen, Königsson, Moberg, Jönsson, Tiihonen, Nordström, Oreland and Åsberg17). Despite the large body of evidence on the association of platelet MAO activity and low serotonergic activity with impulsivity (Reference Harro and Oreland18), evidence for everyday life significance of such measures from longitudinal observations is scarce.
Another candidate for impacting traffic behaviour is the dopaminergic system that is critically involved in behavioural activation, motivated behaviour and reward processing (Reference Ikemoto and Panksepp19). People with elevated dopaminergic functioning behave more impulsively (Reference Bergh, Eklund, Södersten and Nordin20). Dopaminergic dysfunction and elevated impulsivity occur in attention-deficit/hyperactivity disorder (ADHD) (Reference Thapar, O’donovan and Owen21), and it has been shown that ADHD is associated with traffic accidents and violations (Reference Barkley, Murphy, Dupaul and Bush22,Reference Fried, Petty, Surman, Reimer, Aleardi, Martin, Coughlin and Biederman23). Different pharmacological, biochemical, lesion and knockout studies in animals provide evidence that impulsivity is causally related to striatal dopamine (Reference Puumala and Sirviö24,Reference Winstanley, Theobald, Dalley, Cardinal and Robbins25). Pharmacological studies in healthy humans have provided similar results (Reference De Wit, Enggasser and Richards26,Reference Friedel27). The dopamine transporter (DAT) plays a critical role in terminating dopamine neurotransmission and in maintaining dopamine homeostasis in the CNS by taking up synaptic dopamine into neurons (Reference Chen and Reith28). The DAT1 gene (SLC6A3) bears a rather widely studied variable number of tandem repeats (VNTR) polymorphism of a 40-base pair sequence in the 3′-untranslated region of the gene (Reference Costa, Riedel, Müller, Möller and Ettinger29). It has been shown that the DAT1 VNTR 9-repeat (9R) allele carriers have higher striatal DAT availability than do the 10-repeat (10R) allele homozygotes (Reference van de Giessen, de Win, Tanck, van den Brink, Baas and Booij30,Reference Faraone, Spencer, Madras, Zhang-James and Biederman31). A significant association of 9R carriers has been found with alcohol dependence, withdrawal seizures and delirium tremens (Reference Ma, Fan and Li32). Although being a 10R homozygote is thought to be a risk factor for ADHD in children, a differential association of DAT1 with ADHD has been suggested in children and adults and being a 9R homozygote has been associated more with persistent ADHD in adults (Reference Franke, Vasquez, Johansson, Hoogman, Romanos, Boreatti-Hümmer, Heine, Jacob, Lesch, Casas and Ribasés33), which might be explained by differential functional consequences of the polymorphism in the matured dopamine system. In addition, compared to 10R homozygotes, 9R carriers have reported higher impulsivity in studies on adult healthy subjects (e.g., Reference Forbes, Brown, Kimak, Ferrell, Manuck and Hariri34). Because impulsive behaviour has been associated with traffic accidents and violations (Reference Pearson, Murphy and Doane35), the 9R carriers may also be more inclined towards risk-taking behaviour in traffic.
Another, recent candidate for regulation of impulse control and alcohol use is the neuropeptide S (NPS) system (Reference Ghazal36) that in turn can stimulate dopaminergic neurotransmission (Reference Si, Aluisio, Okamura, Clark, Fraser, Sutton, Bonaventure and Reinscheid37). Much of this research has been carried out in animals, but in humans, a functional polymorphism of the gene that encodes for the neuropeptide S receptor 1 (NPSR1) (Reference Dannlowski, Kugel, Franke, Stuhrmann, Hohoff, Zwanzger, Lenzen, Grotegerd, Suslow, Arolt and Heindel38) has been associated with the development of personality, hyperactivity, alcohol use and alcohol use disorders (Reference Laas, Reif, Kiive, Domschke, Lesch, Veidebaum and Harro39–Reference Laas, Eensoo, Paaver, Lesch, Reif and Harro42). NPSR1 gene carries a functional A/T single-nucleotide polymorphism (SNP, rs324981) coding for an Asn-Ile exchange at position 107. NPS has up to 10 times higher potency at the receptor encoded by the T-allele (107Ile) compared to the A-allele-encoded receptor, leading to more effective signal transduction with mobilization of intracellular Ca2+, stimulation of cyclic adenosine monophosphate synthesis and induction of mitogen-activated protein kinases phosphorylation (Reference Reinscheid, Xu, Okamura, Zeng, Chung, Pai, Wang and Civelli43). Recently Taranov and colleagues (Reference Taranov, Puchkova, Slominsky, Tupitsyna, Dementiyenko and Dorokhov44) found in a study with bus drivers that NPSR1 was associated with increased risk of a road accident. The T-allele of the NPSR1 rs324981 polymorphism has been associated with increased impulsivity and ADHD-related traits (Reference Laas, Reif, Kiive, Domschke, Lesch, Veidebaum and Harro39,Reference Laas, Eensoo, Paaver, Lesch, Reif and Harro42). Further, an impulsivity-related early-onset pathway to alcohol use disorder was revealed in male T-allele carriers, particularly in T/T homozygotes: already in adolescence, they exhibit more ADHD symptoms and impulsivity that could make them more vulnerable to alcohol use (Reference Laas, Reif, Akkermann, Kiive, Domschke, Lesch, Veidebaum and Harro41).
Aims of the study
In 2001, we conducted a study of police-referred drunk drivers (Reference Eensoo, Paaver, Pulver, Harro and Harro7,Reference Paaver, Eensoo, Pulver and Harro8), and we could follow-up the driver records about 10 years later. Thus, in the present analysis we enquired whether baseline markers of impulsivity, alcohol use and risk-taking behaviour have any predictive value in traffic in a 10-year time period.
Materials and methods
Sample
The longitudinal Estonian Psychobiological Study of Traffic Behaviour (EPSTB) started with the sample of drunk drivers in 2001; the sample has been described in detail previously (Reference Eensoo, Paaver, Harro and Harro9). The group of drunk drivers comprised male subjects who were identified by the police driving drunk at least once during the previous year (n = 203; mean age ± SD, 33±11 years). The control group was formed by computerised random choice of the male subjects in the driving licence database of the Estonian Motor Vehicle Registration Centre. Subjects were contacted by telephone, the description and aims of the study were provided, and they filled in self-reported questionnaires during a visit to laboratory where they donated venous blood samples. The control group consisted of 211 individuals, with a mean age of 36±12 years. The study was approved by the Research Ethics Committee of the University of Tartu (No 229/T-15).
Socio-economic background, tobacco smoking and alcohol use
Subjects reported their socio-economic status, tobacco smoking and alcohol use habits in a self-report questionnaire. Questions about socio-economic background included relationship status, education and monthly income. The latter was dichotomized according to the mean income in the country in 2001. Tobacco smoking status was categorized to non-smokers, ex-smokers, ≤10 cigarettes/day, 11–19 cigarettes/day, ≥20 cigarettes/day (Reference Eensoo, Paaver, Pulver, Harro and Harro7) and was used in data analysis both as a categorical variable (current smoker vs. non-smoker) and as a continuous variable (5-point scale). The alcohol consumption questionnaire contained items about the frequency of using strong and light alcoholic drinks during the previous year on a 6-point scale (none, some-times during the year, one to three times per month, one to two times per week, three to four times per week, almost every day) (Reference Eensoo, Paaver, Harro and Harro9). The frequency of using alcoholic drinks was used in data analysis both as a categorical (at least once a week vs. less than once a week) and as a continuous variable. The score of alcohol-related problems was obtained by summing up five questions based on the Diagnostic and Statistical Manual of Mental Disorders IV criteria for alcohol abuse, relating to specific life events (‘turned aggressive while drunk’, ‘had longer periods of alcohol use’, ‘had conflicts with friends and family’, ‘been absent from work’ and ‘lost one’s job’; reported as present or not, total score 0–5).
Personality measures
Adaptive and Maladaptive Impulsivity Scale (AMIS) was used to measure different facets of impulsivity (fast decision-making, thoughtlessness, disinhibition and excitement seeking) as previously described (Reference Laas, Reif, Herterich, Eensoo, Lesch and Harro45). AMIS is based on the concept of functional and dysfunctional impulsivity as described by Dickman (Reference Dickman6). Subjects were asked to assess how much the 24 different impulsivity-related statements applied to them on a scale of 1 to 5. All of the 414 subjects filled in AMIS.
Database search
Data on violations of traffic law, traffic accidents and the status of subjects driver’s licences (valid or withdrawn) were obtained from databases maintained by the traffic police, the traffic insurance fund and the Estonian Road Administration for the period of January 1, 2002, to December 31, 2011. The traffic behaviour measures were as follows: speeding (penalties for exceeding the speed limit), DWI (penalties for drunk driving with an estimated blood alcohol level of 0.2‰ or more) and other traffic violations (all the traffic violations besides speeding and DWI). The accidents where the subject was at fault were classified as active accidents, and other accidents were classified as passive accidents.
Platelet MAO activity
Platelet MAO activity was analysed in platelet-rich plasma by a radioenzymatic method with β-phenylethylamine as the substrate according to the procedure described by Hallman et al. (Reference Hallman, Oreland, Edman and Schalling46) after modification (Reference Harro, Eensoo, Kiive, Merenäkk, Alep, Oreland and Harro47). Platelet MAO activity was analysed in 405 subjects and used both as a categorical variable (low 25th percentile vs. high) and as a continuous variable in the statistical analyses.
Genotyping
DAT1
DNA was extracted from venous blood and DAT1 3′UTR VNTR was genotyped as previously described (Reference Maksimov, Vaht, Murd, Harro and Bachmann48). Altogether 399 subjects were successfully genotyped. As the most common DAT1 alleles are the 9- (9R) and 10-repeat (10R) forms (Reference Bannon, Michelhaugh, Wang and Sacchetti49), 8 subjects who had a rare VNTR genotype (10R/11R, 6R/10R) were excluded, leaving 391 subjects for the DAT1 analyses. Genotype distribution was as follows: 10R/10R 63.7%, 9R/10R 31.5% and 9R/9R 4.8%. Genotype frequencies were in Hardy–Weinberg equilibrium.
NPSR1
NPSR1 rs324981 was genotyped by routine polymer chain reaction followed by restriction enzyme digest and gel electrophoresis as previously described (Reference Domschke, Reif, Weber, Richter, Hohoff, Ohrmann, Pedersen, Bauer, Suslow, Kugel and Heindel50). NPSR1 was successfully genotyped in 402 subjects. Genotype distribution was as follows: A/A 28.1%, A/T 46.3% and T/T 25.6%. Genotype frequencies were in Hardy–Weinberg equilibrium.
Statistical analysis
Data were analysed using SPSS (version 23.0 SPSS, Chicago, IL) and SAS (version 9.4 SAS Inc., Cary, NC) software. Pearson’s chi-square test and survival analysis were conducted to compare the differences between control group and drunk drivers group in DWI from 2002 to 2011. Control group and drunk drivers group were additionally separated by whether they had committed a DWI after the initial recruitment (2002–2011) or not. As a result, four subgroups were obtained: controls without DWI (n = 189), controls with DWI (n = 22), drunk drivers without further DWI (n = 136) and drunk drivers with repeat DWI (n = 67). Differences between these subgroups regarding categorical variables (DAT1 VNTR, NPSR1, occurrence of traffic accidents and violations, status of driver’s licence, education, relationship status, income, frequency of using alcohol and tobacco smoking) were analysed with Pearson’s chi-square test and post hoc Fisher test, and for continuous variables (platelet MAO activity, personality measures, alcohol-related problems and age) with ANOVA and post hoc Fisher least significant difference test. Kruskal–Wallis test was used to analyse the differences between subgroups by nonparametrically distributed variable (time without drivers licence).
Cox regression analyses were used to investigate the effect of different variables upon the time subjects committed DWI after the initial recruitment to the study. First, the effect of independent variables was analysed one by one, then the analysed variables were adjusted by the occurrence of DWI before recruitment by adding it as a covariate. In order, to investigate the role of NPSR1 in predicting DWI in combination with other significant variables, the best Cox regression model with NPSR1 was found.
Logistic regression analyses were used for predicting the occurrence of active traffic accidents in 2002–2011. First, simple logistic regression analyses were used for predicting active traffic accidents by independent variables. Next independent variables predicting the occurrence of active traffic accidents were adjusted by the occurrence of DWI after recruitment. Finally, the variables significantly predicting the occurrence of active traffic accidents independently were entered into a logistic regression model together.
Results
In 2002–2011, the members of the original drunk drivers group committed a new DWI with significantly higher probability than the incidence of DWI in controls (33.0% vs. 10.4%, χ2 = 31.3, p<0.001). Survival analysis (Fig. 1) showed that in years 2002–2007 (500–2000 days after recruitment) there was a rapid reoccurrence of DWI in the drunk drivers group (p<0.001). After that the DWI plateaued, meaning that most of the subjects in the drunk drivers group who committed repetitive DWI did it in up to 6 years after the initial recruitment, and very few of them afterwards.

Fig. 1 Occurrence of driving while impaired by alcohol (DWI) in 2002–2011 after recruitment in 2001.
The initial, new and relapsing drunk drivers
As for platelet MAO activity, DAT1 VNTR and NPSR1, significant differences between the DWI subgroups were only found in platelet MAO activity (Table 1): drunk drivers without further DWI and drunk drivers with repeat DWI had lower platelet MAO activity compared to controls without DWI [F(3,401) = 4.7, p = 0.003]. Since cigarette smokers have been shown to have reduced MAO activity (Reference Fowler, Logan, Wang and Volkow51), we controlled for smoking status in the statistical analyses of MAO and with smoking as a covariate significant difference between the subgroups in platelet MAO activity disappeared (p = 0.19). When smoking dose-dependent effect on platelet MAO activity was taken into account (Reference Eensoo, Paaver, Pulver, Harro and Harro7), then among subjects smoking less than 10 cigarettes per day, there was however a significantly higher proportion of subjects with low-platelet MAO activity among drunk drivers without further DWI compared to controls without DWI (respectively, 26.6% (n = 21) vs. 15.1% (n = 23), χ2 = 4.4, p = 0.036), and a tendency for higher proportion of subjects with low-platelet MAO activity in drunk drivers with repeat DWI compared to controls without DWI (respectively, 27.3% (n = 9) vs. 15.1% (n = 23), p = 0.095).
Table 1 Comparison of different variables between DWI subgroups

DWI, driving while impaired by alcohol; MAO, monoamine oxidase; NPSR1, neuropeptide S receptor 1.
† With smoking as a covariate significant difference between the subgroups disappeared
‡ Proportion of subjects whose driver’s licence was withdrawn at some time during 2002–2011
* p<0.05 statistically significant difference from controls without DWI
** p<0.001 statistically significant difference from controls without DWI
# p<0.05 statistically significant difference from controls with DWI
¤ p<0.05 statistically significant difference from drunk drivers without further DWI
¤¤ p<0.001 statistically significant difference from drunk drivers without further DWI.
There were no significant differences in speeding or traffic accidents in 2002–2011 between the four subgroups separated on the basis of single and repeat drunk driving, but there were significant differences in other traffic violations (χ2 = 21.6, p<0.001). Controls with DWI and drunk drivers without further DWI had more other traffic violations compared to controls without DWI, and drunk drivers with repeat DWI had more other traffic violations than all the other groups.
While this is irrelevant for the conducted survival analysis, it can be of interest whether any group differences were observed in suspension of driver’s license that could also limit the period of legal driving. There were a few subjects from the original drunk drivers group who had had their drivers licence withdrawn already at the beginning of the observation period (n = 5). During the observation period, drunk drivers with repeat DWI and controls with DWI had more subjects whose drivers licence was withdrawn (χ2 = 118.2, p<0.0001) and also longer periods of time without a valid drivers licence per person, compared to controls without DWI (Kruskal–Wallis χ2 = 123.6, p<0.0001). The period of time without a valid licence per person was also higher in drunk drivers with repeat DWI compared to drunk drivers without further DWI.
While there were no significant differences in excitement seeking and fast decision-making (the facets of adaptive impulsivity), both original drunk driver groups and controls with DWI had significantly higher scores in disinhibition compared to controls without DWI [F(3,410) = 9.1, p<0.001] and both original drunk driver groups had also significantly higher scores in thoughtlessness, compared to controls without DWI [F(3,410) = 6.6, p<0.001].
Statistically significant differences between DWI subgroups were found in education (χ2 = 22.4, p<0.001), tobacco smoking status (χ2 = 49.9, p<0.001) and alcohol consumption (χ2 = 15.2, p = 0.002, χ2 = 11.8, p = 0.008, for light and strong alcohol, respectively); compared to all the other three groups, controls without DWI had more subjects with higher education, less subjects who were smokers and less subjects who consumed light and strong alcohol more than once a week. Both original drunk driver groups and controls with DWI also reported more alcohol-related problems compared to controls without DWI [F(3,410) = 21.9, p<0.001]. There were however also differences in mean age between the groups: drunk drivers with repeat DWI were significantly younger than controls without DWI and drunk drivers without further DWI [F(3,410) = 4.8, p = 0.003]. There were no significant differences in relationship status or income between the DWI subgroups.
Predicting DWI
Next, using Cox regression, models were found that predicted the occurrence of DWI in the 10-year time period. Table 2 presents the variables predicting DWI in 2002–2011 independently, and models predicting DWI in 2002–2011 with analysed variables adjusted by DWI before recruitment. The following variables predicted DWI in the years 2002–2011 independently: age, education, AMIS disinhibition, AMIS thoughtlessness, frequency of using light alcoholic beverages, alcohol-related problems, tobacco smoking, NPSR1 genotype, active traffic accidents and other traffic violations (in 2002–2011) and drunk driving before recruitment. Adjusting these associations by drunk driving before recruitment, models with age, education, alcohol-related problems, tobacco smoking, NPSR1 and other traffic violations predicted DWI in 2002–2011 significantly. Because our focus was on biological mechanisms behind behaviour, and NPSR1 was the only biological marker significantly predicting DWI, we ran Cox regression models with NPSR1 and other significant variables to see which variables predict DWI best together with NPSR1. The final model included drunk driving before recruitment [hazard ratio (HR) = 3.42; 95% confidence interval (CI) = 2.08–5.63], committing other traffic violations (HR = 2.41; 95%CI = 1.30–4.45) and being an NPSR1 T-allele carrier (HR = 1.85; 95%CI = 1.07–3.18). The Cox regression model was statistically significant, χ2(3) = 42.9, p<0.001 (–2 log likelihood = 941.6).
Table 2 Cox regression models predicting DWI during 2002–2011

MAO, monoamine oxidase; NPSR1, neuropeptide S receptor 1.
† Adjusted by drunk driving in 2001
‡ Adjusted for tobacco smoking; Bold indicates significant predictor; hazard ratio (HR) with 95% confidence intervals (CIs).
DAT1 genotype and active traffic accidents
There were no significant differences between the DWI subgroups when we compared them regarding DAT1 VNTR genotype. However, there were significant differences with respect to DAT1 VNTR when we compared the occurrence of active traffic accidents in the whole sample (Fig. 2): a significantly higher proportion of 9R carriers had been involved in active accidents in 2002–2011 (χ2 = 4.5, p = 0.033).

Fig. 2 Dopamine transporter gene (DAT1) variable number of tandem repeats (VNTR) distribution in subjects with (n = 102+74) and without (n = 147+68) active accidents in the years 2002–2011. *p<0.05, statistically significant difference from the 10R/10R homozygotes with active accidents.
Simple logistic regression analyses showed that in addition to DAT1, the following variables predicted occurrence of active traffic accidents from 2002 until 2011 independently: age, income, AMIS excitement seeking, AMIS fast decision-making, committing DWI and other traffic violations in 2002–2011. The associations in the models were adjusted by committing DWI in 2002–2011 to control for the effect of DWI after recruitment: all the variables except age remained significant (Table 3). From significant predictors by simple logistic regression analyses, multiple logistic regression model was found that predicted the occurrence of active traffic accidents: higher income (OR = 2.47; 95%CI = 1.43–4.24), committing DWI (OR = 1.80; 95%CI = 1.08–3.00), committing other traffic violations (OR = 1.83; 95%CI = 1.14–2.93) and being a DAT1 9R-allele carrier (OR = 1.58; 95%CI = 1.02–2.44). The logistic regression model was statistically significant, χ2(4) = 29.050, p<0.001, explained 9.7% (Nagelkerke R 2) of the variance and correctly classified 62.9% of cases.
Table 3 Logistic regression models predicting occurrence of active traffic accidents in 2002–2011
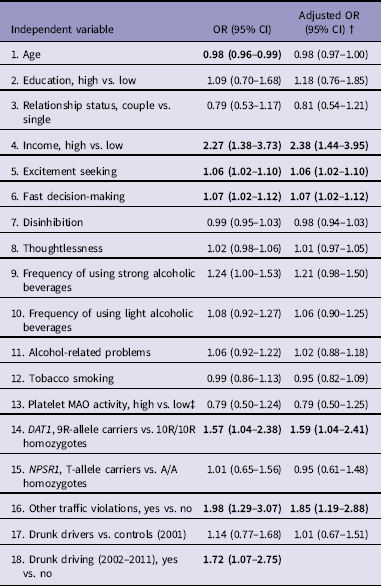
MAO, monoamine oxidase; NPSR1, neuropeptide S receptor 1.
† Adjusted by drunk driving (2002–2011)
‡ Adjusted for tobacco smoking; Bold - significant predictor; Odds ratio (OR) with 95% confidence intervals (CIs).
Discussion
The aim of the current study was to investigate the factors associated with recurrence of drunk driving and whether such factors are also associated with other traffic violations and accidents, with particular focus on established biological markers of excessive risk-taking. People who commit more DWI represent the most hazardous drivers, and police records offer clear advantage of obtaining objective record of behaviour. Previously conducted similar studies on impulsivity and risk-taking behaviour have mostly been cross-sectional. In the current analysis, we could use longitudinal data, including information on traffic violations and accidents.
It has been shown previously that approximately one-third of those arrested for DWI are repeat offenders (Reference Warren-Kigenyi and Coleman52) which is in accordance with our study, where approximately one-third of former drunk drivers had committed DWI within the next 10 years after the original recruitment, which is significantly more compared to controls. Only a few people of the original drunk drivers group were reintroduced into police records as drunk drivers during the second half of the observation period, so the probability of committing DWI again was highest in the 6 years following the initial violation. Although drunk drivers with repeat DWI group had the most subjects whose driver’s licence was withdrawn before and during the observation period and also the longest period of time without a valid driver’s licence per person, it seems that it was not enough for preventing their new DWI or other traffic violations. Therefore, the temporary withdrawal of drunk drivers’ driving licences does not seem to be an efficient enough preventive method, and licences should be withdrawn for a longer period of time and/or the drivers should be introduced to a psychological rehabilitation program.
Platelet MAO activity is suggested to reflect the capacity of the central serotonergic system (Reference Oreland12) and possibly the developmental differences during the foetal stage, eventually leading to higher risk-taking and impaired decision-making, in particular if intoxicated by alcohol (Reference Harro and Oreland18). We had previously shown in this sample that platelet MAO activity was lower in drunk drivers as compared to controls and how smoking has a dose-dependent effect on platelet MAO activity (Reference Eensoo, Paaver, Pulver, Harro and Harro7). It is not surprising that with smoking as a covariate significant differences between the subgroups in platelet MAO activity disappeared, while also the number of non-smokers decreased in these subgroups. It has also been shown before, that both low- and high-platelet MAO activity increases the probability of becoming a smoker, suggesting that smoking is associated with low-platelet MAO activity not only because of the direct inhibitory effect of tobacco constituents on the enzyme but also because subjects with low-platelet MAO activity are more likely to become smokers (Reference Harro, Fischer, Vansteelandt and Harro53). Indeed, separate consideration of low-intensity smoking, not likely to have any large impact on MAO activity, suggested that higher smoking prevalence in drunk drivers does not explain this association.
We expected to see more DWI in the T-allele carriers of the NPSR1 rs324981 polymorphism, which has been associated with increased impulsivity, ADHD-related traits (Reference Laas, Reif, Kiive, Domschke, Lesch, Veidebaum and Harro39,Reference Laas, Eensoo, Paaver, Lesch, Reif and Harro42), earlier and higher alcohol use and higher probability of developing alcohol use disorder (Reference Laas, Reif, Akkermann, Kiive, Domschke, Lesch, Veidebaum and Harro41). Being a T-allele carrier did contribute significantly to the risk of repeatedly committing DWI, showing indeed increased impulsivity and more serious alcohol-related problems among T-allele carriers. Recently in a study of bus drivers, NPSR1 A/A homozygotes had higher incidence of self-reported traffic accidents (Reference Taranov, Puchkova, Slominsky, Tupitsyna, Dementiyenko and Dorokhov44,Reference Dorokhov, Puchkova, Taranov, Ermolayev, Tupitsyna, Slominsky and Dementiyenko54). In our study, we did not find any association between NPSR1 and traffic accidents. Our study is also highly different with regard to the sample formation: bus drivers are highly trained and experienced professionals, and it is unlikely that their involvement in accidents could be largely driven by impulsivity. Indeed, we have demonstrated that impulsivity reduction in traffic schools does diminish the involvement in traffic accidents (Reference Paaver, Eensoo, Kaasik, Vaht, Mäestu and Harro55,Reference Eensoo, Paaver, Vaht, Loit and Harro56). The findings in the bus drivers’ study instead suggest that the higher innate anxiety of the A/A homozygotes, that under environmental pressures can develop into maladaptive traits (Reference Laas, Reif, Kiive, Domschke, Lesch, Veidebaum and Harro39), could have contributed to their higher proneness to accidents.
It had been shown that DAT1 VNTR 9R carriers have higher striatal DAT availability and higher impulsivity than 10R homozygotes (Reference van de Giessen, de Win, Tanck, van den Brink, Baas and Booij30,Reference Faraone, Spencer, Madras, Zhang-James and Biederman31,Reference Forbes, Brown, Kimak, Ferrell, Manuck and Hariri34), therefore we expected 9R carriers to have more traffic accidents and violations, especially DWI. Significantly more 9R-allele carriers overall had active traffic accidents which reflects impulsive behaviour in traffic. Additionally, DAT1 VNTR may enhance the effect of socio-economic environment.
In addition to their DWI violation, repeat offenders had more other traffic violations than subjects in all the other groups. There were also significantly more other traffic violations among controls with DWI and drunk drivers without further DWI, compared to controls without DWI. Therefore, by their other traffic violations, subjects who had committed DWI at least once were more hazardous drivers in traffic than controls without DWI, and subjects with repeat DWI were more hazardous than those who did not repeat drunk driving in the 10-year period.
DWI has previously mostly been associated with maladaptive impulsivity (thoughtlessness and disinhibition; 7,8) and all groups with DWI differed in their maladaptive impulsivity from controls without DWI. Interestingly drunk drivers without further DWI and drunk drivers with repeat DWI had no difference in thoughtlessness and disinhibition, so maladaptive impulsivity is consistently associated with DWI but does not differentiate subjects with repetitive DWI from one-time offenders.
Finally, consistent with former studies (Reference Lapham, Smith, C’de Baca, Chang, Skipper, Baum and Hunt5,Reference Eensoo, Paaver, Harro and Harro9,Reference Sloan, Eldred and Davis57), all groups with DWI had significantly more alcohol-related problems, and subjects in those groups were more frequent alcohol users than controls without DWI. Further, repeat offenders reported more alcohol-related problems than drunk drivers without further DWI, which reflects their more serious substance use problems. Nevertheless, previous DWI was a better indicator of future DWI than self-reported alcohol-related problems. All in all, the results show a clear need for preventive programs of alcohol abuse and dependence, and the need for more thorough examination of driver licence applicants’ fitness to drive.
In conclusion, drunk driving is a serious violation that some people repeat. Drunk driving can be predicted by higher alcohol use and more frequent occurrence of alcohol-related problems and aspects of impulsivity. Biological markers of impulsivity can be reliably associated with everyday traffic behaviour and help in contributing to the understanding of the need for more personalized prevention activities.
Acknowledgements
We are grateful to the EPSTB participants and the whole study team. This work was supported by Estonian Ministry of Education and Research Project IUT20-40, the European Commission Seventh Framework Programme (FP7 2007-2013) Project Aggressotype (n°602805), Horizon 2020 Programme Projects CoCA (n°667302) and Eat2beNICE (n°728018) and Estonian Road Administration.
Financial Support
This work was supported by Estonian Ministry of Education and Research Project IUT20-40, the European Commission Seventh Framework Programme (FP7 2007-2013) Project Aggressotype (n°602805), Horizon 2020 Programme Projects CoCA (n°667302) and Eat2beNICE (n°728018) and Estonian Road Administration.
Conflicts of Interest
None.
Ethical Standards
The authors assert that all procedures contributing to this work comply with the ethical standards of the relevant national and institutional committees on human experimentation and with the Helsinki Declaration of 1975, as revised in 2008.