Introduction
Blastozoans, a highly diverse group of Paleozoic echinoderms, are an integral component of marine communities during critical times of Earth's history (Foote, Reference Foote1992). Blastozoans lived through times of dramatic climate change, such as the Ordovician, and their diverse morphologies likely reflect responses to changing environments (Lefebvre et al., Reference Lefebvre, Sumrall, Shroat-Lewis, Reich, Webster, Hunter, Nardin, Rozhnov, Guensberg and Touzeau2013). However, the phylogenetic relationships of blastozoans are poorly understood (Paul, Reference Paul, Paul and Smith1988; Sumrall, Reference Sumrall, Waters and Maples1997; Kammer et al., Reference Kammer, Sumrall, Zamora, Ausich and Deline2013) because of their complex morphologies, lack of well-understood skeletal homologies, and a paucity of well-preserved specimens for many taxa. Blastozoans previously have been subdivided into class-level groupings based on the types of respiratory structures present in previously ascribed taxa (Sprinkle, Reference Sprinkle1973). A growing body of evidence indicates that at least some of these respiratory structures are likely homoplastic and, consequently, circumscribe groups of species that are not united by the evolutionary process (Paul, Reference Paul, Paul and Smith1988; Sumrall and Gahn, Reference Sumrall and Gahn2006). To date, a number of blastozoan groups are considered to be polyphyletic (e.g., edrioasteroids, rhombiferans, diploporitans; Sprinkle and Bell, Reference Sprinkle and Bell.1978; Lefebvre and Fatka, Reference Lefebvre and Fatka2003; Zamora and Rahman, Reference Zamora and Rahman2014). Aphyletic groupings represent an obstacle to addressing basic paleobiological questions because no questions rooted in evolutionary theory can be answered in the absence of a phylogeny. Consequently, placing Diploporita into a phylogenetic framework is critical for understanding their paleobiology.
Diploporitans have long been considered one of the most problematic groups of blastozoans, and multiple authors have considered them to be polyphyletic (Paul, Reference Paul, Paul and Smith1988; Sumrall, Reference Sumrall, Harris, Böttger, Walker and Lesser2010; Lefebvre et al., Reference Lefebvre, Sumrall, Shroat-Lewis, Reich, Webster, Hunter, Nardin, Rozhnov, Guensberg and Touzeau2013; Sheffield and Sumrall, Reference Sheffield, Sumrall, Zamora and Rábano2015). These taxa, which ranged from the Ordovician through the Devonian, have been traditionally diagnosed as those blastozoans with diplopore (double pore) respiratory structures that pierce the skeletal plates of the body wall (Sprinkle, Reference Sprinkle1973). However, diplopores are constructed differently across Diploporita, suggesting multiple origins (Paul, Reference Paul, Paul and Smith1988; Sheffield and Sumrall, Reference Sheffield, Sumrall, Zamora and Rábano2015), and non-diploporitan echinoderms have been discovered to have diplopores (e.g., Thresherodiscus Sumrall and Gahn, Reference Sumrall and Gahn2006 is a Late Ordovician isorophid edrioasteroid, highly morphologically distinct from a typical diploporitan, that has diplopores piercing interambulacral plates), and some traditionally ascribed diploporitans do not have diplopores (Haeckel, Reference Haeckel1896). Consequently, diplopores are likely not a reliable diagnostic feature for all taxa presently assigned to diploporitans. Further, diploporitans encompass wide morphological variation across the three previously ascribed groups, which suggests that they are only distantly related and do not constitute a valid phylogeny-based grouping (Fig. 1.1–1.3).

Figure 1. Representative specimens within Diploporita. Diploporita encompasses wide morphological variability, likely indicating that the group is polyphyletic. (1) Eumorphocystis multiporata (Glyptosphaeritida), characterized by ambulacra that are erect distally and lie on biserial floor plates (SUI 97598) (2) Paulicystis densus Frest, Strimple, and Paul, Reference Frest, Strimple and Paul2011 (Sphaeronitida), characterized by a large mouth, proximal thecal plates modified into comparatively large brachiole facets, and humatipore respiratory structures (SUI 48164). (3) Gomphocystites indianensis (Glyptosphaeritida), characterized by a small mouth, diplopores and long, spiraling ambulacra without floor plates (FMNH 19708) (4) Amphoracystis irregularis (Barrande, Reference Barrande, Barrande, Počta, Perner, Waagen and Jahn1887); taxon within proposed group Sphaeronitida, characterized by irregular thecal plating, globular theca, presence of a holdfast, and lack of respiratory structures (NM-L 13063). Known specimens of Amphoracystis do not have preserved oral areas. (5) Oral view of Archegocystis desiderata (Barrande, Reference Barrande, Barrande, Počta, Perner, Waagen and Jahn1887) (NM-L 7687a ; latex cast). This taxon is only known from incomplete oral area; information about the theca, attachment structure, and respiratory structures were largely unavailable and therefore was excluded from this analysis. (6) Side view of Protocrinites oviformis Eichwald, Reference Eichwald1840 (GIT 540-57). While some oral areas of this taxon have been found, it was not able to be examined during this study. Due to conflicting ideas about the morphology of the stem (whether it detaches as an adult or not), as well as different interpretations of the oral area, this taxon was excluded from the analysis. (7) Oral view of Tholocystis sp. Tholocystis is only known from incomplete oral areas. Information concerning the presence and number of oral plates, ambulacral floor plates, hydropore, and gonopore are unavailable, and therefore this taxon was excluded from this analysis (MGM-7192X; latex cast). (8) Celtacystis gotlandicus (Angelin, Reference Angelin1878) was not included in this study; after careful consideration, the authors regard this specimen as a junior synonym of Gomphocystites gotlandicus (RM Ec 27355). Photo credit: Lukáš Laibl. Scale bar = 1 cm.
To date, Diploporita has not been analyzed in a phylogenetic context. Here we test diploporitan monophyly by analyzing taxa that encompass the wide morphological variation currently assigned to the group and closely related non-diploporitan taxa. Furthermore, this experimental design tests the monophyly of the various named subgroups of Diploporita (Glyptosphaeritida, Sphaeronitida, Asteroblastida). Placing diplopore-bearing taxa within a phylogenetic framework is the first step towards being able to assess evolutionary trends (e.g., trait evolution, biogeography) within these taxa.
Previous phylogenetic investigations of Blastozoa and Diploporita
Although blastozoan echinoderms are a large and globally distributed component of the Paleozoic marine fauna, relatively few phylogenetic studies have been performed on this group and its monophyly has not been assessed. Previous studies have focused on analyzing small subsets of blastozoan groups or have focused only on a few representative taxa to encompass a vast amount of morphology (Breimer and Macurda, Reference Breimer and Macurda1972; Paul, Reference Paul, Paul and Smith1988; Sumrall, Reference Sumrall, Waters and Maples1997; Frest et al., Reference Frest, Strimple and Paul2011; Sumrall and Waters, Reference Sumrall and Waters2012; Ausich et al., Reference Ausich, Kammer, Rhenberg and Wright2015). Regardless of advances in understanding these evolutionary relationships, a number of blastozoan groups are almost certainly polyphyletic (e.g., Rhombifera, Diploporita, Eocrinoidea), masking the true diversity of Paleozoic echinoderms (Sumrall, Reference Sumrall, Waters and Maples1997; Nardin et al., Reference Nardin, Lefebvre, David and Mooi2009).
Very few studies have investigated the phylogenetic relationships of the diploporitans within a rigorous quantitative framework. Paul (Reference Paul, Paul and Smith1988) included sphaeronitid diploporitans within a high-level taxonomy phylogenetic analysis that placed sphaeronitids (those diploporitans described as being spherical to ovoid in shape, with short ambulacra and a small or absent column; Kesling, Reference Kesling and Moore1967) as a sister taxon to the eocrinoid Lichenoides Barrande, Reference Barrande1846. Other diploporitan taxa were excluded from this analysis because of their confusing morphology. The only other phylogenetic analysis involving diploporitans was performed by Frest et al. (Reference Frest, Strimple and Paul2011), and was limited to members of the Silurian Holocystites fauna (see Paul, Reference Paul1971; Frest et al., Reference Frest, Strimple and Paul2011; Sheffield and Sumrall, Reference Sheffield and Sumrall2017 for a comprehensive review). The relationships presented by Frest et al. (Reference Frest, Strimple and Paul2011), however, were derived from an analysis that included some inaccurate character codings, several non-independent characters, and some characters that did not represent logical morphological transitions of homologous elements (e.g., numbers of oral plates as characters; Sheffield and Sumrall, Reference Sheffield and Sumrall2017). Neither of these analyses was performed using rigorous phylogenetic methods or with a full understanding of shared homologous features and, therefore, did not inform the relationships of diplopore-bearing taxa within the larger blastozoan echinoderm group.
Currently defined diploporitan relationships
Diploporita Müller, Reference üller1854, as most recently established in Kesling (Reference Kesling and Moore1967), is divided into three major groups: Glyptosphaeritida Bernard, Reference Bernard1895; Sphaeronitida Neumayr, Reference Neumayr1889; and Asteroblastida Bather, Reference Bather and Lankester1900. Glyptosphaeritids include diplopore-bearing blastozoans with globular, ovate, pear-shaped, or saclike thecae, with ambulacra extending across the theca, ending in one or multiple brachiole facets, and with diplopores present on both thecal plates and plates bearing ambulacral grooves. Within this group, taxa are heterogeneous, including taxa that: (1) either bear or have lost floor plates, (2) have different configurations of the positioning of the food grooves on the oral plates, and (3) have either aboral holomeric stems or lack stems and bear only holdfasts.
Sphaeronitids include diplopore-bearing blastozoans with ovate to elongate thecae that are constricted distally as well as short and unbranched ambulacra (Paul, Reference Paul, Paul and Smith1988). This group includes taxa with and without floor plates, different configurations of proximal food grooves with respect to the oral plates, different configurations of brachial facets on the ambulacral systems, presence or absence of facetal plates, and different types of diplopores borne on the thecal plates.
Asteroblastids include diplopore-bearing blastozoans with bud- or bullet-shaped thecae, with ambulacral floor plates that lie against the theca, and diplopores that are restricted to interambulacral areas. Taxa within this group show a variety of thecal plate configurations and constructional differences of the ambulacra with respect to the underlying thecal plates. All bear holomeric stems.
The high degree of morphological differences among these taxa strongly suggests that they are more distantly related than suggested by placing them into a class, uniquely diagnosed by a single character. Two scenarios could answer this: either a number of features used to diagnose the different higher-level groups (e.g., theca shape, presence of stems or holdfasts) likely re-evolved during the course of the groups’ evolution or these features are clade-diagnostic, but the classification of the clade is incorrect. Further, diplopore construction, the morphological feature that diagnoses Diploporita, varies across these three groups, suggesting multiple originations of these respiratory structures (Kesling, Reference Kesling and Moore1967). Combined, the evidence suggesting diploporitan polyphyly requires phylogenetic revision, which is the central goal of this study.
Respiratory structures as clade-diagnostic features
Classical Linnaean systematics diagnoses groups of organisms based on the presence or absence of key diagnostic features. Blastozoan echinoderms traditionally have been subdivided into groups arbitrarily placed at the class rank, primarily based on the presence of different types of respiratory structures borne by their constituent species (Sprinkle, Reference Sprinkle1973); for example, we identify eocrinoids by their epispire respiratory structures and diploporitans by their diplopore respiratory structures. This classification scheme assumes that respiratory features are complex and, therefore, unlikely to evolve independently in numerous lineages. However, recent evidence has shown that complex respiratory structures do evolve independently in many lineages, suggesting that basing classification primarily on respiratory structures is an oversimplification of a much more complex evolutionary pattern (Sumrall and Gahn, Reference Sumrall and Gahn2006).
The only morphological feature that currently groups species into Diploporita is the presence of diplopore respiratory structures. Diplopore-bearing blastozoans show wide variation in nearly every other major morphological feature, including the makeup of the body wall, feeding apparati, and attachment structure (Fig. 1.1–1.3). However, even within the class-defining diplopores, there is wide variety across taxa. Some have simple diplopores defined as a double-pore system, connected by a single, uncalcified thecal canal. The pores are contained within the peripore, a depression contained on the thecal plate. Presumably, this formed an attachment point where a fluid-filled, fleshy bulb attached for respiration. A number of diplopore-bearing blastozoans have differently constructed diplopores, humatipores (restricted to taxa within the Holocystitidae), in which a pore pair is connected with multiple canals, that are buried just under the surface of the thecal plate (Paul, Reference Paul1971; Frest et al., Reference Frest, Strimple and Paul2011; Sheffield and Sumrall, Reference Sheffield and Sumrall2017). There have been reports of ‘unbranched’ diplopores in Pachycalix Chauvel, Reference Chauvel1936, haplopores, that consist of a single pore contained within a depression (see Paul, Reference Paul1972 for a thorough discussion of the variety of diplopore morphotypes). Specimens of Pachycalix were not available for study, so these observations cannot be confirmed. Further, there are taxa within Diploporita that have no known respiratory structures, such as Amphoracystis Haeckel, Reference Haeckel1896 (Fig. 1.4), a taxon from Lower Ordovician strata of the Prague Basin.
Increasing evidence indicates that blastozoan respiratory structure types are somewhat plastic, evolving along similar functional lines in distantly related taxa and are, therefore, not appropriate as key characters on which to base evolutionary groupings (Paul, Reference Paul, Paul and Smith1988; Sumrall, Reference Sumrall, Waters and Maples1997; Sumrall and Gahn, Reference Sumrall and Gahn2006). Phylogenetic analyses of other blastozoan echinoderms, such as rhombiferans, indicate that the presence or absence of rhomb-shaped respiratory structures has little bearing on whether taxa are or are not included within the traditionally diagnosed group rhombifera (Brochu and Sumrall, Reference Brochu and Sumrall2001; Zamora et al., Reference Zamora, Sumrall, Zhu and Lefebvre2016). Some early taxa, such as Macrocystella Callaway, Reference Callaway1877 and Cuniculocystis Sprinkle and Wahlman, Reference Sprinkle and Wahlman1994, predate the evolution of pectinirhombs and bear other types of respiratory systems; other taxa, such as Amecystis Ulrich and Kirk, Reference Ulrich and Kirk1921, lost rhombs secondarily (Sumrall and Sprinkle, Reference Sumrall and Sprinkle1995).
Further, Thresherodiscus, a Late Ordovician isorophid edrioasteroid, has numerous diplopores connected by a raised, thin-walled bulb of stereom within the interambulacral plating series (Sumrall and Gahn, Reference Sumrall and Gahn2006). The presence of diplopores in taxa not closely related to Diploporita further illustrates the likely convergent nature of many respiratory systems.
Materials and methods
Methods
Characters were selected and developed to reflect homology across echinoderm taxa utilizing tests for determining potential homology in morphological characters proposed in Patterson (Reference Patterson, Joysey and Friday1982); an explanation of characters utilized in this analysis and the data matrix are available in the supplementary materials (data available from the Dryad Digital Repository: https://doi.org/10.5061/dryad.f420m4q). The Universal Elemental Homology (UEH) scheme (Sumrall, Reference Sumrall, Harris, Böttger, Walker and Lesser2010; Kammer et al., Reference Kammer, Sumrall, Zamora, Ausich and Deline2013), developed to understand the homologous skeletal elements of echinoderms, was used to guide character development in this study. The Extraxial-Axial Theory (EAT; Mooi and David, 1998) is an alternative scheme to understand echinoderm homology, but is not for understanding individual plate homology; therefore, EAT was not used to construct characters in this study. Great care was taken to assure that alternate states of a character were logical transformations of homologous structures. Characters in the analysis are presumed to be hereditary; characters concerning the shape of the theca and holdfast were not emphasized in this analysis because these are likely driven by environmental factors, shown by the highly variable morphologies present across specimens within specimens of a single species (Gil Cid and García-Rincón, Reference Gil Cid and García-Rincón2012; Sheffield and Sumrall, Reference Sheffield and Sumrall2017).
Selection of taxa
Taxa were selected to cover a breadth of morphological diversity spanning Diploporita and other blastozoan groups. Excluded from the analysis are taxa without preserved oral and stem areas to prevent an excessive amount of missing data and taxa that were unable to be examined by the authors. Also excluded from the analysis were taxa that were inferred to be junior synonyms of taxa included in the analysis. Non-diploporitan blastozoans were selected as representative taxa for their groups (e.g., Rhombifera, Crinoidea). Taxa chosen were morphologically well understood and studied personally by the authors to ensure accurate coding. These taxa were added to the analysis to test the hypothesis that Diploporita is monophyletic.
Incomplete or unobtainable taxa
A number of taxa are only known from a small number of very incomplete specimens. The large majority of these taxa were not utilized in this study, to prevent the analysis from being overwhelmed by missing data. Specimens of note that were excluded: Archegocystis Jaekel, Reference Jaekel1899 (known only from incomplete oral areas; Fig. 1.5); Protocrinites (most specimens incomplete; interpretations of morphology of this taxon, especially those concerning the stem, are highly varied and inconclusive at this time; Fig. 1.6); Tholocystis Chauvel, Reference Chauvel1941 (known from incomplete oral and thecal areas; information concerning the majority of the plating of the ambulacral system undeterminable from preserved specimens; Fig. 1.7); and Amphoracystis Haeckel, Reference Haeckel1896 (only known from incomplete specimens of the theca, without oral or stem area; Fig. 1.4).
Synonymies
Regnellicystis typicalis Bassler, Reference Bassler1950, a diplopore-bearing taxon found in deposits of the Ordovician Benboldt Formation of Virginia, is considered by both Parsley (Reference Parsley and Sprinkle1982) and here to be a junior synonym of Eumorphocystis multiporata, based on identical plating of the oral area, makeup of the theca, and plating of the stem.
Celtacystis gotlandicus (Angelin, Reference Angelin1878), an Ordovician Baltican diplopore-bearing taxon (Fig. 1.8), was proposed by Bockelie (Reference Bockelie1979). It was described as having a reduced oral area of four oral plates, instead of the seven present in Gomphocystites. This interpretation is rejected here based on drawings and photographs of the specimens that show Celtacystis bearing seven oral plates that were misinterpreted by Bockelie (Reference Bockelie1979). Celtacystis gotlandicus (Angelin, Reference Angelin1878) is, therefore, rejected as a junior synonym of Gomphocystites gotlandicus Angelin, Reference Angelin1878.
Osgoodicystis Frest and Strimple in Frest et al., Reference Frest, Strimple and Paul2011, a Silurian diplopore-bearing taxon from Laurentia, was proposed as a separate genus from Pentacystis Paul, Reference Paul1971 based on the presence of oral plates within the ambulacral system, which were inferred to be absent in Pentacystis (Paul, Reference Paul1971; Frest et al., Reference Frest, Strimple and Paul2011). However, a reanalysis of these specimens showed that the differences were taphonomic (Sheffield and Sumrall, Reference Sheffield, Sumrall, Zamora and Rábano2015; Sheffield and Sumrall, Reference Sheffield and Sumrall2017); therefore, Osgoodicystis was rejected as a valid genus and is not utilized as a separate taxon in this analysis.
Specimens that were unobtainable for this analysis were largely excluded, except when unavoidable to fully assess morphological variability (e.g., Asteroblastus) to prevent incorrect conclusions concerning morphological characters being drawn from previous interpretations. Brightonicystis Paul, Reference Paul1971 was not available for study; previous interpretations of this taxon having ten oral plates are highly unlikely because it would prove to be the only echinoderm taxon known with more than seven oral plates (Sheffield and Sumrall, Reference Sheffield and Sumrall2017). Specimens of Asteroblastida were not available for analysis either; however, in an effort to encompass as much morphological diversity as possible, Asteroblastus stellatus was coded utilizing primary literature (Kesling, Reference Kesling and Moore1967), with the understanding that some of the interpretations may change once specimens are available for study. Calix segwicki Rouault, Reference Rouault1851 also was not included in this analysis due to poor preservation of specimens and diverse interpretations of its morphology.
The remaining taxa used in the analysis were coded at the species level, primarily utilizing physical specimens examined by the authors and supplemented by the primary literature. The character matrix for this analysis was analyzed in phylogenetic program PAUP* v. 4.0a147 (Swofford, Reference Swofford2003) utilizing maximum parsimony. The analysis included 61 characters, of which 41 were parsimony-informative, and 28 taxa spanning traditionally ascribed Diploporita and representatives of other Paleozoic stemmed echinoderm groups; parsimony uninformative characters were removed from the phylogenetic analysis. A heuristic search of most-optimal trees was run utilizing a tree-bisection-reconnection (TBR) branch-swapping algorithm (reconnection limit of eight). Tree support was measured via bootstrap analysis. The matrix was polarized using the outgroup criterion with Gogia spiralis Robison, Reference Robison1965 assigned to the outgroup as sister taxon to the ingroup.
Two constrained topology analyses were performed to test: (1) the proposed monophyly of Diploporita, and (2) the monophyly of the three traditionally proposed groups of diploporitans (Glyptosphaeritida, Sphaeronitida, and Asteroblastida). The first constrained analysis forced all diploporitan taxa to form a single clade without forcing internal relationships within Diploporita. The second constrained tree topology forced taxa from the three groups (Glyptosphaeritida, Sphaeronitida, Asteroblastida) to form three individual clades, but were not forced into a single larger clade of diploporitans. In both analyses, all other taxa were reduced to a polytomy and eocrinoid Gogia was used as the outgroup to polarize the matrix; code to perform this function in PAUP* v. 4.0a147 was added to the original nexus file. This tree topology was explored in PAUP* v. 4.0a147 using the same analytical techniques as above. A Templeton test (Templeton, Reference Templeton1983) was performed to compare trees with and without topological constraints against one another to determine if the trees were statistically different from one another. Two randomly selected trees were placed into a new nexus file (i.e., a most parsimonious tree with no topological constraints placed into a new file with the most parsimonious constraint tree assuming Diploporita is a monophyletic group and a most parsimonious tree with no topological constraints placed into a new file with the most parsimonious constraint tree assuming the three ascribed families within Diploporita are monophyletic) and compared against one another. The code from the Templeton tests is available in the supplementary materials (https://doi.org/10.5061/dryad.f420m4q).
Repositories and institutional abbreviations
All taxa studied for this analysis are listed in Table 1. All specimens are housed in research collections from the following museums or institutions: Cincinnati Museum Center (CMCIP), Field Museum of Natural History (FMNH; UC), The University of Iowa (SUI), Miami University (MUGM), Yale Peabody Museum (YPM), Prague National Museum (NM), Swedish Royal Museum of Natural History (RM), Museo Geominero (Geological Survey of Spain), Madrid, Spain (MGM), and the Geological Institute of Tallinn (GIT).
Table 1. Diplopore-bearing taxa utilized within this phylogenetic analysis. The original classifications of taxa (i.e., Glyptosphaeritida, Sphaeronitida, and Asteroblastida) are indicated, as is paleocontinent data for each species.

Results
Phylogenetic analysis
The parsimony analysis identified 18 optimal trees of 99 steps, excluding uninformative characters, CI= 0.535, RI= 0.723, HI= 0.465. The strict consensus tree (Fig. 2) inferred a clade that aligns with the relationships currently described as Sphaeronitida, indicating that the original classification of these diplopore-bearing blastozoans represents a monophyletic group, supported by the presence of food grooves that are restricted to the oral summit and a lack of floor plates. Contained within the sphaeronitids is a large clade comprising the Holocystitidae (bootstrap support of 74) supported by the presence of short food grooves that end in single brachiole facets and proximal thecal plates modified into brachiole-bearing plates. Sister group to the Holocystitidae is a clade comprising Haplosphaeronis oblonga and Eucystis angelini supported by the multiterminal ambulacral grooves and a rotation of the grooves to lie on oral plates, instead of the sutures (although the oral plates are radially positioned with this rotation, as opposed to the plesiomorphic interradial position, they are still recognizable as oral plates due to their seven-plate oral area positioning; Sumrall, Reference Sumrall2017).
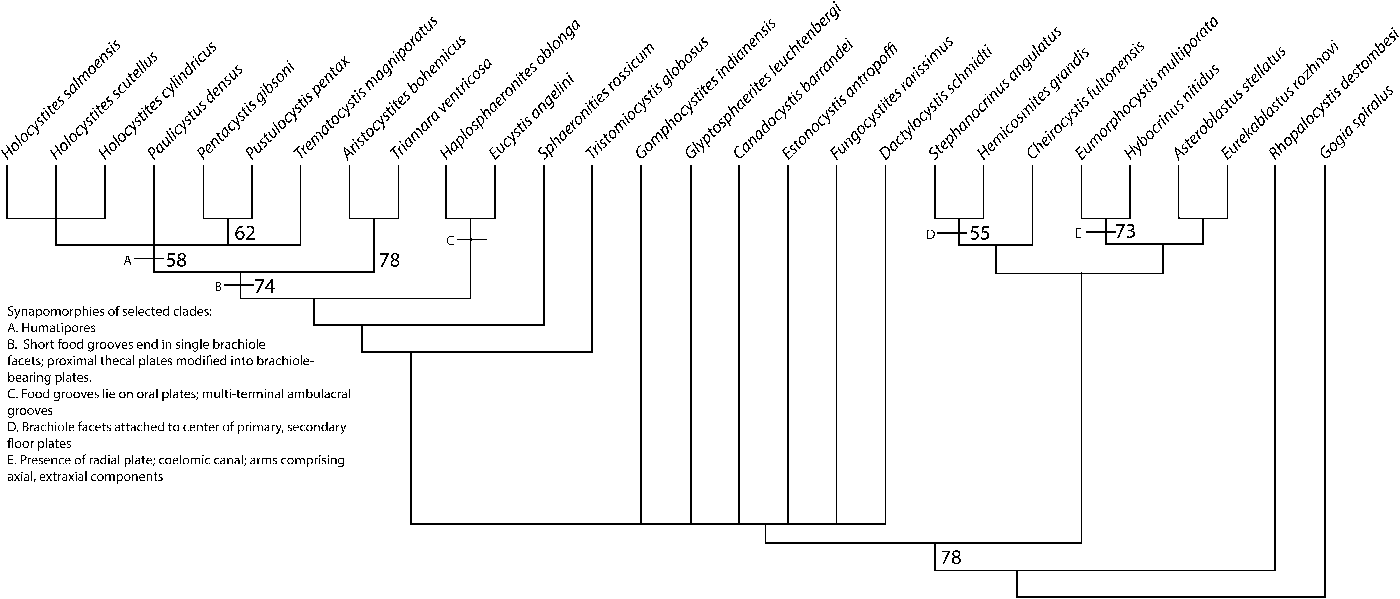
Figure 2. Strict consensus of the 18 most parsimonious trees with a length of 99 steps. Bootstrap values of supported relationships above 50% are indicated by the appropriate node; synapomorphies of selected clades are highlighted by letters at the appropriate node. This tree indicates that the original classification of Sphaeronitida is a natural evolutionary group, whereas Glyptosphaeritida is polyphyletic because paracrinoid Canadocystis is rooted within taxa traditionally grouped with the glyptosphaeritids and Eumorphocystis shares a sister taxon relationship with crinoid Hybocrinus. It is clear that diplopore-bearing taxa are polyphyletic because asteroblastids are contained within another clade of echinoderm taxa and sister group to parablastoid Eurekablastus. Further, this analysis indicates that rhombiferans may be paraphyletic, as evidenced by the relationships of coronoid Stephanocrinus to rhombiferans Cheirocystis and Hemicosmites, although the sampling of rhombiferans in this analysis is not extensive because it is not the focus of this paper. This analysis also further supports the hypothesis that crinoids share a sister group relationship with Eumorphocystis, as evidenced by the most parsimonious placement of Hybocrinus. CI= 0.535, RI= 0.723, HI= 0.465.
Diplopore-bearing taxa traditionally comprising the group Glyptosphaeritida are spread across the tree, representing a polyphyletic group; the paracrinoid Canadocystis barrandei (Billings, Reference Billings1858) is rooted within a cluster of glyptosphaeritids and other traditionally defined glyptosphaeritids are nested within other clades.
Eumorphocystis and Hybocrinus are united as a clade (bootstrap support of 73), supported by the presence of a radial plate, coelomic canal, and arms comprising extraxial and axial components. Parablastoid Eurekablastus and asteroblastid diploporitan Asteroblastus stellatus are sister taxa to Eumorphocystis and Hybocrinus. These two clades are sister taxa to Stephanocrinus, Hemicosmites, and Cheirocystis (the clade comprising Stephanocrinus and Hemicosmities has a nodal support of 55), supported by the presence of brachiole facets being attached to the center of primary and secondary floor plates. This analysis indicates that rhombiferans are polyphyletic because Stephanocrinus is more closely related to Hemicosmites than Cheirocystis. Eocrinoid Rhopalocystis is sister taxon to all of these taxa discussed above.
Constraint analysis
Secondary analyses were performed constraining the original systematic placement of diplopore-bearing taxa to form a monophyletic group and to constrain the original groupings within Diploporita as clades (i.e., Glyptosphaeritida, Sphaeronitida, Asteroblastida, and non-diploporitan taxa). This was done in order to test if the original assumption that Diploporita is monophyletic is as parsimonious, or that the groupings within Diploporita are monophyletic, as the phylogenetic hypothesis presented in this study. For the constraint analysis testing diploporitan monophyly (Fig. 3), the resulting set of most parsimonious trees (1,218 trees retained) had a tree length of 108, nine steps longer than the most parsimonious tree without topological constraints of diplopore-bearing taxa, CI= 0.491, RI= 0.669, HI= 0.509. For the constraint tree assuming that Glyptosphaeritida, Sphaeronitida, and Asteroblastida each represent a separate monophyletic group, but not necessarily contained within a monophyletic Diploporita (Fig. 4), the resulting set of most parsimonious trees (109 trees retained) had tree lengths of 109 steps, 10 steps longer than the most parsimonious tree without topological constraints of diplopore-bearing taxa, CI = 0.486; RI= 0.663, HI = 0.514. None of the relationships among the non-diplopore bearing taxa was inferred in all of the most parsimonious trees.

Figure 3. Strict consensus tree of the 1,218 most parsimonious trees, if the diploporitan taxa are forced into a single clade. Group relationships (i.e., Sphaeronitida, Glyptosphaeritida, Asteroblastida) were not preserved in the constraint topology. The resulting tree had a tree length of 108, nine steps longer than the most parsimonious tree without topological constraints of diplopore-bearing taxa. CI= 0.491, RI= 0.669, HI= 0.509.

Figure 4. Strict consensus of the 246 most parsimonious trees, if the three groups (i.e., Sphaeronitida, Glyptosphaeritida, Asteroblastida) were forced to each be a clade, without forcing the three to also be contained within a larger Diploporita clade. The resulting tree had a length of 109, 10 steps longer than the most parsimonious tree without topological constraints of diplopore-bearing taxa. CI = 0.486; RI= 0.663, HI = 0.514.
Templeton test
The Templeton test that was run in PAUP* v. 4.0a147 compared a randomly selected most parsimonious tree (without topological constraints) with a length of 99 steps against the most parsimonious trees with topological constraints (i.e., Diploporita as a monophyletic group and the three families within Diploporita being monophyletic, with lengths of 108 and 109, respectively). This test was run to quantify whether or not these randomly selected trees were significantly different from one another. The results of the Templeton test indicate that the phylogenetic hypothesis presented here (that diplopore-bearing blastozoans are not a clade) is significantly different and more parsimonious than the alternate hypothesis that each of the three ascribed groups within Diploporita is monophyletic (p = 0.0254). The result of the Templeton test comparing the phylogenetic hypothesis presented here against the hypothesis that Diploporita represents a monophyletic group is p = 0.0527, which is considered significant here.
Discussion
Diploporitans
Both of the constraint analyses indicate that it is less parsimonious to treat Diploporita as a clade and it is less parsimonious to treat Glyptosphaeritida, Sphaeronitida, and Asteroblastida as separate clades, even if not constrained within the larger Diploporita group. The most parsimonious solution is to treat diplopore-bearing taxa as multiple groups spread throughout the blastozoan tree of life. The Templeton tests that were run to determine if the constraint trees are significantly different from one another support this interpretation; the constraint tree that forced the three proposed groups within Diploporita to be three separate monophyletic groups is significantly less parsimonious than the hypothesis presented here, that Diploporita is not a clade. The constraint tree that forced Diploporita into a single monophyletic group is also significantly less parsimonious. While the p-value for this result was 0.0527, an insignificantly different number than the traditionally accepted 0.05, p-values are generally subjective and we consider this result to be significant (see Gelman, Reference Gelman2013).
Sphaeronitids
Only the sphaeronitids have been argued to represent a monophyletic group (Paul, Reference Paul, Paul and Smith1988); this analysis supports the Sphaeronitida as a natural evolutionary group. The sphaeronitids share short ambulacral grooves restricted to the oral area and a lack of floor plating associated with the ambulacral grooves. Two major groups within the sphaeronitids are inferred: those that end in multiple terminal brachiole facets (Haplosphaeronis, Eucystis, and Sphaeronites; Fig. 5.1–5.4) and those with ambulacra ending in a single terminal brachiole facet (those diplopore-bearing blastozoans within Holocystitidae; Fig. 7.5, 7.6).

Figure 5. Representative diploporitan taxa used in this analysis. (1) Oral view of Eucystis angelini (NM-L7695). Ambulacral grooves are short and restricted to the summit; grooves are multiterminal and end in a varying number of brachiole facets without underlying floor plates. Grooves are positioned on the oral plates, as opposed to lying on the oral plate sutures. (2) Side view of Eucystis angelini (NM-L7694). Diplopores randomly arranged on irregularly plated theca, which narrows distally into a holdfast. (3) Oral view of Haplosphaeronis sp. (GIT 540-3). Ambulacral grooves are extremely short, restricted to the summit, and multiterminal; grooves are positioned on the oral plates, instead of lying on the sutures between the oral plates. Note that first primary peristomial cover plate is split into two. (4) Side view of Haplosphaeronis sp. (GIT 540-3). Note that the diplopores are aligned vertically on the thecal plates. Holdfast is approximately the same width as the theca. (5) Oral view of Pustulocystis pentax (MUGM-T 266). Extremely short ambulacral grooves that lie on oral plate sutures end on thecal plates modified with large, single brachiole facets. (6) Side view of Pustulocystis pentax (MUGM-T-266). Humatipores distributed randomly across thecal plates; theca narrows distally into holdfast. (7) Oral view of Estonocystis antropoffi (GIT 540-80). Ambulacral grooves that lie on the oral plate sutures anastomose down the theca; brachiole facets begin after the oral plate series. (8) Side view of Estonocystis antropoffi (GIT 540-80). Short grooves extending from the main food groove connect to brachioles that are situated in the center of single ambulacral floor plates. Diplopores align horizontally along the floor plates. Basals, 4, form around circular stem. Scale bar = 1 cm.
Haplosphaeronis and Eucystis are united by a feature that appears to re-evolve more than once within diplopore-bearing blastozoans. In both taxa, there is a rotation of ~36° (Sumrall, Reference Sumrall, Zamora and Rábano2015), so that the ambulacral grooves are not lying on the sutures of the oral plates (the plesiomorphic condition), but they are centered on the oral plates (Fig. 5.1, 5.3). This feature also appears in Glyptosphaerites, as well as in Holocystites. The poorly documented Ordovician taxon Tholocystis (Fig. 1.7) from peri-Gondwana, likely belongs within the Eucystis and Haplosphaeronis clade, potentially as sister taxon to Haplosphaeronis. Both taxa are united by the first primary peristomial cover plate being split into two, a feature not seen in any other diplopore-bearing echinoderm taxa. However, due to incomplete preservation of the theca, respiratory structures, and a large portion of the oral area, the relationships of Tholocystis were not assessed in this analysis.
The Holocystitidae, a group of diplopore-bearing taxa largely restricted to the North American continent (a single example of a holocystitid outside of Laurentia has been found in upper Silurian rocks of South Wales; Jell, Reference Jell2010), represent a clade within the sphaeronitids. This group is united by a number of synapomorphies: (1) floor plate-less food grooves that end in a single brachiole facet, (2) extremely large brachiole facets, and (3) proximal thecal plates that are differentiated into facetal plates upon which brachiole facets are born (Figs. 1.2, 5.5). While it has been suggested by multiple authors that this fauna does represent a monophyletic group (Paul, Reference Paul1971; Frest et al., Reference Frest, Strimple and Paul2011; Sheffield and Sumrall, Reference Sheffield and Sumrall2017), it was not understood if Triamara, found in many of the same middle Silurian deposits as the Holocystites fauna, was a member. The simple diplopores found in Triamara differ fundamentally in construction when compared to the humatipores found in the rest of the Holocystites fauna (Sheffield and Sumrall, Reference Sheffield and Sumrall2017). However, Triamara and Aristocystites (known from Early Ordovician deposits of the Prague Basin) are found to be sister taxa (nodal support of 68) to the humatipore-bearing members of the Holocystites fauna in this analysis.
Asteroblastids
Asteroblastus stellatus is sister taxon to parablastoid Eurekablastus (parablastoids are diagnosed as blastozoans with endothecal respiratory structures in the form of cataspires) in this analysis, indicating that the placement of this group within a monophyletic Diploporita is not supported by current data. It also further suggests that diplopore respiratory structures have evolved independently throughout the course of echinoderm evolution in the most parsimonious optimization of this character. The sister group to the Eurekablastus-Asteroblastus clade contains crinoid Hybocrinus and Eumorphocystis. The most parsimonious explanation for the evolution of diplopore respiratory structures within this clade is that diplopores were evolved independently in both Eumorphocystis and Asteroblastus. However, it is also possible that the presence of diplopores evolved earlier in the tree and was lost on four separate occasions. While this is a less parsimonious explanation, it is difficult to fully ascertain trait history with the limited sampling present in Eumorphocystis and asteroblastid-type taxa. Further sampling of these groups will likely refine the results of this analysis and better constrain the history of diplopore respiratory structure evolution.
It is possible that the asteroblastid group (comprising Asteroblastus, Asterocystis, and Metasterocystis) could represent a monophyletic group. Although lack of available samples prevented full phylogenetic analysis, this phylogenetic analysis indicates that diplopores evolved independently within Asteroblastus.
Glyptosphaeritids
The glyptosphaeritids, as previously described, represent a polyphyletic group because Eumorphocystis (Fig. 1.1) is contained within a clade of non-diploporitan taxa, including crinoids. The group that does appear rooted within diplopore-bearing taxa (excluding Eumorphocystis) is paraphyletic because non-diplopore bearing paracrinoid Canadocystis is contained within it (Fig. 2). These blastozoans are united by ambulacra that extend down the theca and end in alternating brachiole facets (e.g., Estonocystis; Fig. 5.7, 5.8). Ambulacral floor plates are present in the majority of the taxa, including Canadocystis, but they are lost in Gomphocystites indianensis (Fig. 1.3).
Crinoids
The origin of crinoids has been debated in many studies—the two major hypotheses posit crinoids being derived either from Cambrian edrioasteroids (Guensberg and Sprinkle, Reference Guensburg and Sprinkle2007, Reference Guensburg and Sprinkle2009; Guensberg et al., Reference Guensburg, Blake, Sprinkle and Mooi2016) or from within blastozoans (Sumrall, Reference Sumrall, Waters and Maples1997; Ausich et al., Reference Ausich, Kammer, Rhenberg and Wright2015; O'Malley et al., Reference O'Malley, Ausich and Chin2016). This analysis supports Hybocrinus as sister taxon to Eumorphocystis multiporata. This relationship is supported in the analysis by multiple shared features: (1) presence of a coelomic canal, (2) arms extraxial and axial skeletal components, (3) an extraxial thecal plate (radial plate) supporting the exothecal arm, and (4) a single oral plate within the CD interray. This relationship is corroborated by another recent phylogenetic analysis that also places Eumorphocystis as sister taxon to crinoids (Sheffield and Sumrall, Reference Sheffield and Sumrall2019), which has important implications for understanding the origins of crinoids.
Rhombiferans
Rhombiferan blastozoans previously have been hypothesized to represent a non-monophyletic grouping (Paul, Reference Paul, Paul and Smith1988; Sumrall, Reference Sumrall, Waters and Maples1997; Nardin et al., Reference Nardin, Lefebvre, David and Mooi2009). This analysis, although incomplete in its sampling of rhombiferans, supports this idea because hemicosmitoid rhombiferan Hemicosmites and Stephanocrinus are sister taxa, and glyptocystitoid rhombiferan Cheirocystis is sister taxon to them. This indicates that rhomb-type endothecal respiratory structures may have been lost in some taxa or evolved more than once in the echinoderm tree, and is further evidence that respiratory structure types are likely not clade-defining features. Hemicosmites and Stephanocrinus share: (1) erect ambulacra without extraxial components, (2) large peristome, and (3) brachiole facets on the oral plates. Cheirocystis, Hemicosmites, and Stephanorinus share the presence of brachiole facets being attached to the center of primary and secondary floor plate pairs.
Increased sampling
While this research is the first wide-scale phylogenetic analysis of diplopore-bearing echinoderms, further sampling of critical taxa is necessary to more fully document the evolutionary transitions of diplopore-bearing blastozoans. To understand how many times diplopore respiratory structures evolved among echinoderms, better sampling of eumorphocystitid-type and asteroblastid-type taxa is necessary. Further, sampling of diplopore-bearing blastozoans from under-sampled times in Earth's history (e.g., late Cambrian) and under-sampled areas of Earth (e.g., Gondwana, South China) has the potential to change much of what is currently understood about the evolution of these taxa.
Future implications
Echinoderms, with their complex and highly disparate morphologies, have been shown to respond to long-term oceanic environmental patterns such as: oxygenation levels and seawater ion ratios (Paul, Reference Paul1968; Dickson, Reference Dickson2002, Reference Dickson2004; Clausen, Reference Clausen2004; Clausen and Smith, Reference Clausen and Smith2005, Reference Clausen and Smith2008; Zamora and Smith, Reference Zamora and Smith2008; Rahman and Zamora, Reference Rahman and Zamora2009). Blastozoan echinoderms during the early Paleozoic would have been responding to significant global climate changes during the Ordovician (Lefebvre et al., Reference Lefebvre, Sumrall, Shroat-Lewis, Reich, Webster, Hunter, Nardin, Rozhnov, Guensberg and Touzeau2013). These responses to climate change are likely driving the convergence of respiratory structures and other morphological features. Previous studies have focused on attempting to understand how global climate change affected the biodiversity and biogeography of Paleozoic echinoderms (e.g., Lefebvre and Fatka, Reference Lefebvre and Fatka2003; Lefebvre, Reference Lefebvre2007; Lefebvre et al., Reference Lefebvre, Sumrall, Shroat-Lewis, Reich, Webster, Hunter, Nardin, Rozhnov, Guensberg and Touzeau2013; Zamora et al., Reference Zamora, Lefebvre, Àlvaro, Clausen, Elicki, Fatka, Jell, Kouchinsky, Lin, Nardin, Parsley, Rozhnov, Sprinkle, Sumrall, Vizcaino and Smith2013; Sumrall et al., Reference Sumrall, Deline, Colmenar, Sheffield, Zamora, Zamora and Rábano2015). However, without a full understanding of the phylogenetic relationships of blastozoans, it is impossible to assess how these taxa responded.
Conclusions
This analysis indicates that diplopore-bearing blastozoan echinoderms do not represent a monophyletic group, but rather a polyphyletic grade. Diplopores have evolved as respiratory structures multiple times within Paleozoic blastozoan echinoderms and, as such, are not a synapomorphy, as presently used to diagnose Diploporita. This finding adds to the growing body of evidence that respiratory structures in blastozoans can be convergent and are not always clade defining. Sphaeronitidae represents a natural group of diplopore-bearing blastozoans, characterized by short, floor plate-less food grooves that are restricted to the oral surface. The Holocystites fauna is a clade within the sphaeronitids, united by proximal thecal plates modified into facetal plates for bearing single brachiole facets. The proposed glyptosphaeritids are a polyphyletic clade of diplopore-bearing blastozoans. The asteroblastids are sister taxon to parablastoids and are not contained within the traditionally ascribed Diploporita. Eumorphocystis is sister taxon to crinoids and adds support to the hypothesis that crinoids are rooted within blastozoans. Constraint analyses to test both the monophyly of Diploporita and the monophyly of the three groups within Diploporita indicate that both are less parsimonious than the phylogenetic hypothesis presented in this analysis. To better constrain the evolutionary significance of why certain respiratory structures are convergent across echinoderms, further sampling of enigmatic blastozoan groups and sampling at critical times during Earth's history and in underrepresented areas of the globe can have major implications for our understanding of echinoderm evolution throughout the Paleozoic.
Acknowledgments
We are grateful to the following for their assistance with museum specimens: T. Adrain, The University of Iowa; S. Butts, Yale Peabody Museum; K. Hollis, U.S. National Museum of Natural History; B. Hunda, Cincinnati Museum Center; B. Hussaini, American Museum of Natural History; P. Mayer, The Field Museum; K. Hauer, Miami University; U. Toom, Geological Institute of Tallinn; G. Baranov, Geological Institute of Tallinn; L. Laibl, Université de Lausanne; Jonas Hagström, Swedish Royal Museum of Natural History. We would like to thank J. Waters, B. O'Meara, S. Drumheller, M. McKinney, K. Massana, and J. Bauer for comments on earlier drafts of this study and to B. Deline and an anonymous reviewer for their thorough comments on this manuscript. This work was supported by grants awarded to SLS from The Paleontological Society, The Association of Applied Paleontological Sciences, The Paleontological Research Institution, The Dry Dredgers, SEPM, the Schuchert and Dunbar Grants-in-Aid Program, and NSF Assembling the Echinoderm Tree of Life project, DEB-1036260 awarded to CDS.
Accessibility of supplemental data
Data available from the Dryad Digital Repository: https://doi.org/10.5061/dryad.f420m4q