1. Introduction
The La Meseta Formation (LMF) crops out in Seymour (Marambio) and Cockburn islands, both located at the northeastern tip of the Antarctic Peninsula. The LMF forms the upper part of Late Jurassic – Cenozoic sedimentation of the James Ross Basin and provides the best palaeontological record of marine and terrestrial Cenozoic biota in Antarctica. The fossils that have been found in the LMF include marine invertebrates such as echinoderms, bryozoans, corals, decapods, brachiopods and molluscs (e.g. Stillwell & Zinsmeister, Reference Stilwell and Zinsmeister1992; Álvarez et al. Reference Álvarez, del Río and Marenssi2014), vertebrates including fishes, reptiles, aquatic birds, whales and marsupials (e.g. Reguero et al. Reference Reguero, Marenssi and Santillana2012 and references therein), plants (e.g. Gandolfo et al. Reference Gandolfo, Hoc, Santillana, Marenssi and Casadío1998; Pujana et al. Reference Pujana, Santillana and Marenssi2014), microfossils such as foraminifera and ostracods (Gaździcki & Majewski, Reference Gaździcki and Majewski2012), and palynomorphs mainly comprising dinoflagellate cysts, pollen and spores (e.g. Wrenn & Hart, Reference Wrenn, Hart, Feldman and Woodburne1988; Cocozza & Clarke, Reference Cocozza and Clarke1992; Askin, Reference Askin and Ricci1997; Douglas et al. Reference Douglas, Affek, Ivany, Houben, Sijp, Sluijs, Schouten and Pagani2014).
The fossil record of the LMF is extremely diverse and abundant, and fossils exhibit an excellent to exceptional preservation. Due to these peculiar characteristics, the LMF is a focus of attention for several palaeontologists and geologists worldwide. This palaeontological wealth exposed in the LMF is crucial not only for studying the taxonomic and phylogenetic relationship among fossil groups, their radiation and the palaeobiogeographic links between Antarctic and Patagonian faunas, but also in reconstructing the Antarctic environments prior to the significant climate deterioration and the onset of glaciation of Antarctica at the beginning of the Oligocene (e.g. Tatur et al. Reference Tatur, Krajewski and del Valle2011), which has been triggered with the Drake Passage opening and deepening (Livermore et al. Reference Livermore, Nankivell, Eagles and Morris2005).
The LMF was assigned to a late early Eocene to late Eocene / ?Oligocene age once the first fossils, including palynology (Wrenn & Hart, Reference Wrenn, Hart, Feldman and Woodburne1988; Cocozza & Clarke, Reference Cocozza and Clarke1992; Askin, Reference Askin and Ricci1997), were studied together with isotopic data from bivalve shells (e.g. Dutton et al. Reference Dutton, Lohmann and Zinsmeister2002; Ivany et al. Reference Ivany, Lohmann, Hasiuk, Blake, Glass, Aronson and Moody2008). However, a subsequent age model based on dinoflagellate cyst biostratigraphy and strontium isotopes proposed for the LMF (Douglas et al. Reference Douglas, Affek, Ivany, Houben, Sijp, Sluijs, Schouten and Pagani2014) suggested a mid- to late Eocene age (45 to 34 Ma, middle-Lutetian – Priabonian). In spite of this, many researchers still support the older age, and indeed a magnetostratigraphic scheme recently performed in the LMF proposes ∼58.4 Ma (upper Thanetian) to ∼45.8 Ma (lower Lutetian) (Montes et al. Reference Montes, Beamud, Nozal, Santillana, Crame, Acosta Hospitaleche and Gelfo2019a).
It is evident that the mid- to late Eocene age for the LMF is not entirely accepted and there are still debates about whether is early, middle or late Eocene. Elucidating and confirming the correct chronology of the LMF has crucial implications, as the LMF has a rich palaeontological record of vertebrates, including the first Antarctic mammals (Woodburne & Zinsmeister, Reference Woodburne and Zinsmeister1982; Gelfo et al. Reference Gelfo, Mors, Lorente, Lòpez and Reguero2015), invertebrates and flowering plants (Gandolfo et al. Reference Gandolfo, Hoc, Santillana, Marenssi and Casadío1998) and also constitutes a significant archive of palaeoclimate data. Given the importance of the Eocene strata on Seymour Island for palaeoclimatic reconstructions, a re-examination of its chronostratigraphy is warranted. It is especially important to confirm the age of the LMF in order to make comparisons with other Eocene assemblages of the Southern Ocean and to understand the events that occurred around the Drake Passage and the consequences that brought about its opening.
Thus, the aim of this contribution is to document the dinoflagellate cyst assemblages recovered throughout the LMF and discuss the stratigraphical distribution of the species in order to complement and firm up the age proposed by Douglas et al. (Reference Douglas, Affek, Ivany, Houben, Sijp, Sluijs, Schouten and Pagani2014) and thus contribute to an accurate chronology. This analysis also provides quantitative data on the dinoflagellate cyst assemblages of the LMF that will be available to seek a robust comparison with other localities of the Drake Passage area. In addition, palaeoceanographical implications and a palaeoenvironmental reconstruction for the LMF based on the dinoflagellate cyst assemblages are presented herein.
2. Geological setting, stratigraphy and age of the La Meseta Formation
2.a. Geology
Seymour (Marambio) Island is located in the Weddell Sea near the NE margin of the Antarctic Peninsula (64° 15′ S, 56° 40′ W) (Fig. 1a). It is located in the most external (SE) area of the Maastrichtian and Palaeogene outcrops in the James Ross Basin (Elliot, Reference Elliot, Feldmann and Woodburne1988).
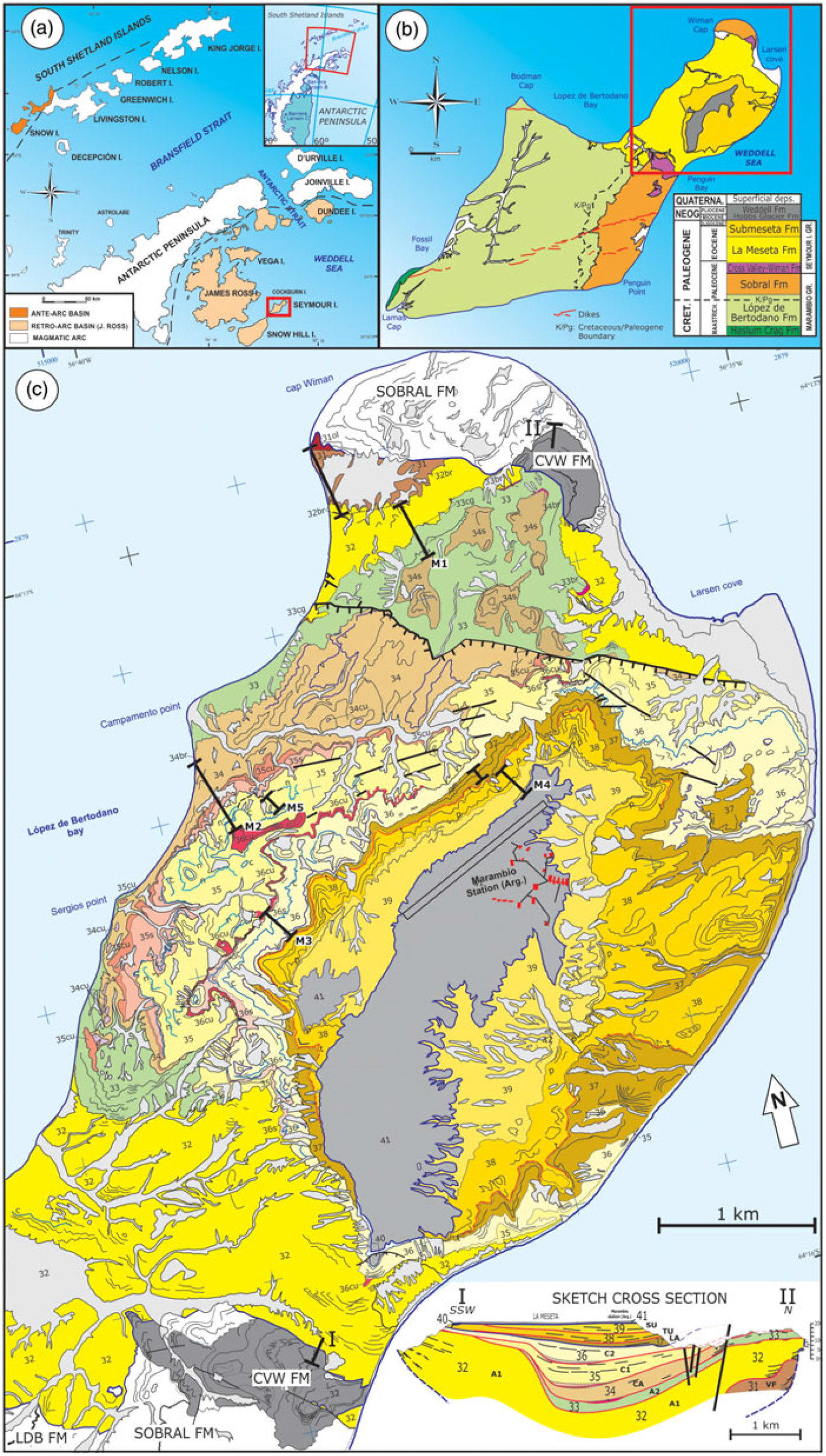
Fig. 1. (a) Location map of Seymour Island related to the Antarctic Peninsula. (b) Location of the La Meseta Formation, showing the study area at Marambio (Seymour) Island. The box shows the area mapping with more resolution in (c) The scheme of stratigraphic units follows that of Montes et al. (Reference Montes, Nozal, Santillana, Marenssi and Olivero2013). (c) Location of stratigraphic columns in the Geological Map of the La Meseta and Submeseta formations of Seymour Island (modified from Montes et al. Reference Montes, Nozal, Santillana, Marenssi and Olivero2013). Numbers 32–41 correspond to cartographic units defined by Montes et al. (Reference Montes, Nozal, Santillana, Marenssi and Olivero2013). The geometry of the units is shown in sketch cross-section (number and name units in Fig. 2). Abbreviations: LDB FM: Lopez de Bertodano Formation; CVW FM: Cross Valley – Wiman Formation. ol breccia and ostolites; cr sandstones with concretions; cg conglomerates and sandstones; br breccia and conglomerates; v veneroidea (bivalve) levels; s sandstones; cu canalized level with gravel and Cucullaea (bivalve) coquinas; cCucullaea (bivalve) levels; tTurritella (gastropod) level.
The sedimentary sequences exposed in Seymour (Marambio) Island are more than 2000 m thick and represent the youngest part of the infill of the James Ross Basin. These sediments are part of the Marambio and Seymour Island Groups (Rinaldi, Reference Rinaldi and Craddock1982 and Elliot & Trautman, Reference Elliot, Trautman and Craddock1982, respectively; Fig. 1b). In Seymour, the Marambio Group comprises the Haslum Crag Formation (Olivero et al. Reference Olivero, Ponce and Martinioni2008), López de Bertodano Formation and Sobral Formation (Rinaldi et al. Reference Rinaldi, Massabie, Morelli, Rosenman and del Valle1978), with ages spanning from the Maastrichtian to the Danian. The group consists of fine-grained sandstone and shales, highly fossiliferous, with subordinate conglomerates and fossiliferous bioclastic concentrations deposited in a middle-external to internal shelf that represent an extended prograding platform in the Weddell Sea for more than 100 km to the east (Olivero et al. Reference Olivero, Ponce and Martinioni2008). The rest of the Palaeogene sediments of Seymour Island are represented by the Seymour Island Group (Elliot & Trautman, Reference Elliot, Trautman and Craddock1982), which comprises the Cross Valley – Wiman Formation (Montes et al. Reference Montes, Santillana, Nozal and Marenssi2008), the LMF (Rinaldi et al. Reference Rinaldi, Massabie, Morelli, Rosenman and del Valle1978; Marenssi et al. Reference Marenssi, Santillana, Rinaldi and Casadío1998) and the Submeseta Formation (Montes et al. Reference Montes, Nozal, Santillana, Marenssi and Olivero2013, Reference Montes, Beamud, Nozal, Santillana, Crame, Acosta Hospitaleche and Gelfo2019a, b). The sediments of this group (Palaeocene–Oligocene) represent the final stage of filling of the James Ross Basin and were deposited in incised valleys on an emergent marine platform (Marenssi et al. Reference Marenssi, Santillana, Rinaldi and Casadío1998).
During deposition of the Marambio and Seymour Groups, the eastern edge of the James Ross Basin acted as a passive continental margin receiving sediments mainly from the magmatic arc located in the Antarctic Peninsula (Marenssi et al. Reference Marenssi, Net and Santillana2002). After a period of emersion and erosion between the Oligocene and the early Miocene, glaciomarine sediments of the Hobbs Glacier Formation were deposited overlying the LMF (Marenssi et al. Reference Marenssi, Casadío and Santillana2010).
Later, in the terminal Miocene (Zinsmeister & Webb, Reference Zinsmeister and Webb1982), a complex of several eruptive phases of alkaline volcanic rocks of the James Ross Volcanic Group (JRVG) defines a post-subduction ensialic volcanism, with eruptions on the NE flanks of the magmatic arc and related to an extensional tectonic period in the area of the Antarctic Peninsula (Smellie et al. Reference Smellie, Pankhurst, Hole and Thomson1988). The youngest sediments of the James Ross Basin correspond to the Weddell Formation, interpreted as glacial deposits of latest Pliocene–Holocene age (Gaździcki et al. Reference Gaździcki, Tatur, Hara and del Valle2004).
2.b. Stratigraphy of the La Meseta Formation
The LMF (Elliot & Trautman, Reference Elliot, Trautman and Craddock1982) consists of poorly consolidated and bioturbated sandstones and siltstones, with tractive structures, alternating with rhythmical alternations of very fine sandstone-siltstone and mudstone with inclined heterolithic stratification and coquina. Its sediments contain very abundant and relatively well-preserved marine and continental vertebrate fauna, marine invertebrates and plant remains. The base of this formation is a diachronic surface that cut older stratigraphic units on the island. The lenticular geometry and the architecture of the internal units were interpreted as the filling of an incised valley, eroded on an emerging platform after the tilting of the Marambio Group and the Cross Valley – Wiman Formation strata (Marenssi et al. Reference Marenssi, Santillana, Rinaldi and Casadío1998).
Based on the architecture of the lithosomes, the relationship between facies and sedimentary palaeoenvironments, the Eocene filling of Seymour Island has been divided into six allomembers (Marenssi et al. Reference Marenssi, Santillana, Rinaldi and Casadío1998; Montes et al. Reference Montes, Nozal, Santillana, Marenssi and Olivero2013), namely: Valle de las Focas (Telm 1), Acantilados I and II (Telm 2 and Telm 3 in partim), Campamento (Telm 3 in partim and Telm 4), and Cucullaea I and II (Telm 5 and Telm 6 in partim). The uppermost Eocene / ?Oligocene sediments previously considered as the upper unit of the LMF, named the Submeseta Allomember (Marenssi et al. Reference Marenssi, Santillana, Rinaldi and Casadío1998), are now considered a new formation, the Submeseta Formation (Montes et al. Reference Montes, Nozal, Santillana, Marenssi and Olivero2013). This was divided into three allomembers denominated Submeseta I or Laminado (Telm 6 in partim and Telm 7 in partim), Submeseta II or Turritella (Telm 7 in partim) and Submeseta III or Superior (upper Telm 7). The correlation between these allomembers and the informal lithofacies, named as Telm 1 and 7 and defined by Sadler (Reference Sadler, Feldman and Woodbu1988), are shown in Marenssi et al. (Reference Marenssi, Santillana, Rinaldi and Casadío1998, fig. 4, p. 140). The Submeseta Formation consists of c. 162 m of muddy shales; fine sandstones with mollusc coquinas and sandstone packages with gravels. The top of the Submeseta Formation unconformably underlay the Plio-Quaternary glaciomarine deposits of the Weddell Formation, in most of its extension. However, in small outcrops located in the SW of Seymour Island, the unit unconformably underlies the Hobbs Glacier Formation (Marenssi et al. Reference Marenssi, Casadío and Santillana2010).
2.c. The age of the La Meseta Formation
2.c.1. Previous palynological studies
The first palynological studies of the LMF were presented by Hall (Reference Hall1977) who reported the presence of pollen and spores and provided the first analysis of fossil dinoflagellate cysts, assigning a late Eocene – early Oligocene age to the unit. Later, Wrenn & Hart (Reference Wrenn, Hart, Feldman and Woodburne1988) used the dinoflagellate cyst assemblages to subdivide the LMF into two units: interval A (upper lower Eocene) and interval B (middle–upper Eocene).
Cocozza & Clarke (Reference Cocozza and Clarke1992) analysed the marine palynoflora (dinoflagellate cysts, acritarchs and Chlorophyta) obtained from the lower part of the LMF (Telm 1 – Telm 2 according to Uchman & Gaździcki, Reference Uchman and Gaździcki2006, p. 155) and divided the section into three assemblages: Association 1 (late early Eocene), Association 2 and Association 3 (possible middle–late Eocene).
Askin (Reference Askin and Ricci1997) subdivided the LMF into four provisional zones based on terrestrial and marine palynomorphs. The lower zone (including Valle de las Focas, Acantilados and basal Campamento allomembers) was dated as late early Eocene; the overlying zone (including the upper Campamento and the basal Submeseta allomembers) was assigned likely to the middle Eocene. The third zone (Submeseta Allomember) was proposed to be late Eocene in age while the upper zone, considered to be the top of the LMF (the uppermost 25 m of the formation), was dated as earliest Oligocene.
Recently, Douglas et al. (Reference Douglas, Affek, Ivany, Houben, Sijp, Sluijs, Schouten and Pagani2014), in order to reconstruct the Eocene climatic variability in southern high latitudes, proposed a biostratigraphic age model for the LMF based on five dinoflagellate cyst samples (from Telm 2 – Telm 7) and concluded that the LMF was deposited during the middle to late Eocene (between 45 and 34 Ma), in contrast to previous early to late Eocene age assignments.
Exclusively terrestrial palynomorph (pollen and spores) studies of the LMF were also carried out (Askin & Elliot, Reference Askin and Elliot1982; Askin & Fleming, Reference Askin and Fleming1982; Askin Reference Askin and Ricci1997). However, they focused on recycled pollen and spores in the Cenozoic Seymour Island sediments; the taxonomy of some pollen genera and species; and the diversity and compositional change of terrestrially derived assemblages in relation to climatic conditions, without engaging in specifying age.
2.c.2. Other palaeontological and isotopic data
Initially, Zinsmeister & Camacho (Reference Zinsmeister and Camacho1980) studied mollusc faunas and proposed a late Eocene – early Oligocene age for the LMF which was then sustained by Stilwell & Zinsmeister (Reference Stilwell and Zinsmeister1992) based on a biostratigraphic provisional zonation using marine gastropods. Considering that proposed age, Marenssi (Reference Marenssi, Francis, Pirrie and Crame2006) related the basal erosion surface of the LMF to the sea-level lowstand established by Sadler (Reference Sadler, Feldman and Woodbu1988) in the late Ypresian (49 Ma).
On the other hand, Dutton et al. (Reference Dutton, Lohmann and Zinsmeister2002) examined selected Cucullaea bivalve specimens for strontium isotope (87Sr/86Sr) analyses of Telms 2, 5 and 7 of the LMF and suggested that the deposition of the unit took place during the early–late Eocene. Ivany et al. (Reference Ivany, Lohmann, Hasiuk, Blake, Glass, Aronson and Moody2008) analysed the 87Sr/86Sr bivalve shell ratios of Telms 2–7 and indicated that the LMF spanned almost the entire Eocene, and suggested the presence of an early middle Eocene unconformity. However, Douglas et al. (Reference Douglas, Affek, Ivany, Houben, Sijp, Sluijs, Schouten and Pagani2014) disagree with the age proposed by the above-mentioned authors, pointing out that the strontium-isotope data may be biased because of the presence of freshwater mixing or the incorporation of trace minerals into the shells. They also found that the uncertainty of the data is intensified by the small degree of variation of the global seawater strontium-isotope curve for the early to middle Eocene of McArthur et al. (Reference McArthur, Howarth and Bailey2001). Therefore, Douglas et al. (Reference Douglas, Affek, Ivany, Houben, Sijp, Sluijs, Schouten and Pagani2014) applied the dinoflagellate cyst biostratigraphic framework proposed by Bijl et al. (Reference Bijl, Sluijs and Brinkhuis2013a) for the early Palaeogene of the Southern Ocean, which is magnetostratigraphically calibrated to the 2012 geologic timescale, suggesting that the lower part of the LMF is no older than the base of the middle Eocene, contrary to previous assignments that consider that the formation also spanned the early Eocene.
A recent age model based on a combination of magnetostratigraphy, isotopic data (87Sr/86Sr) and lithostratigraphy was performed in the LMF by Montes et al. (Reference Montes, Beamud, Nozal, Santillana, Crame, Acosta Hospitaleche and Gelfo2019a). According to this model, the age of the LMF ranges from the upper Thanetian (∼58.4 Ma) to the lower Lutetian (∼45.8 Ma).
The vertebrate fossil records also allowed inferences to be made about the age of the LMF. The age of terrestrial fossil mammals, especially ungulates (i.e. astrapotheres and litopterns), recovered from the Cucullaea I and Cucullaea II allomembers, is based on the assumption that Patagonian and Antarctic faunas were similar because they shared some taxa (Gelfo et al. Reference Gelfo, Reguero, López, Carlini, Ciancio, Chornogubsky, Bond, Goin, Tejedor and Albright2009; Tejedor et al. Reference Tejedor, Goin, Gelfo, López, Bond, Carlini, Scillato-Yan, Woodburne, Chornogubsky, Aragón, Reguero, Czaplewski, Vincon, Martin and Ciancio2009; Reguero et al. Reference Reguero, Goin, Acosta, Dutra and Marenssi2013). Gelfo et al. (Reference Gelfo, Reguero, López, Carlini, Ciancio, Chornogubsky, Bond, Goin, Tejedor and Albright2009) and Tejedor et al. (Reference Tejedor, Goin, Gelfo, López, Bond, Carlini, Scillato-Yan, Woodburne, Chornogubsky, Aragón, Reguero, Czaplewski, Vincon, Martin and Ciancio2009) proposed that the Paso del Sapo fauna of west-central Patagonia, whose age, based on the 40K/40Ar method (∼49.5 Ma), is late early Eocene (Ypresian/Lutetian boundary), was putatively coeval and biogeographically related to the LMF fauna. However, Bond et al. (Reference Bond, Kramarz, MacPhee and Reguero2011) pointed out that terrestrial mammals from the LMF closely resemble, but are not exactly similar to those of late early Eocene faunas from southern Patagonia. Besides, the finding of Antarctic whales (basilosaurids) in the Cucullaea I Allomember (Buono et al. Reference Buono, Fernández, Reguero, Marenssi, Santillana and Mörs2016) indicates an early middle Eocene age for that part of the section, consistent with the stratigraphic record of basilosaurids worldwide.
3. Material and methods
The studied material consists of 13 productive samples collected from all the allomembers of the LMF (sensu Marenssi et al. Reference Marenssi, Santillana, Rinaldi and Casadío1998): Valle de las focas, Acantilados I, Acantilados II, Campamento, Cucullaea I and Cucullaea II (Fig. 2). The upper allomembers (Laminado, Turritella and Superior) considered as the Submeseta Formation by Montes et al. Reference Montes, Nozal, Santillana, Marenssi and Olivero2013, Reference Montes, Beamud, Nozal, Santillana, Crame, Acosta Hospitaleche and Gelfo2019a, b) are excluded from this study. Stratigraphic sections were measured by some of the authors (S.S., M.M. and F.N.) as part of the new geological cartography of Seymour Island (Montes et al. Reference Montes, Nozal, Santillana, Marenssi and Olivero2013, Reference Montes, Nozal, Olivero, Gallastegui, Maestro, Santillana, Martín-Serrano, Montes, Nozal and Santillana2019b).

Fig. 2. Occurrence of dinoflagellate cyst species in the La Meseta Formation on Seymour Island, Antarctic Peninsula. On the left, stratigraphic columns of the La Meseta Formation with stratigraphic units (allomember and number in Fig. 1) and sample positions (modified from Montes et al. Reference Montes, Nozal, Santillana, Marenssi and Olivero2013, Reference Montes, Beamud, Nozal, Santillana, Crame, Acosta Hospitaleche and Gelfo2019a, b). On the right, two different age models are shown: one is the dinoflagellate cyst zonation of the early Palaeogene South Pacific Ocean by Bijl et al. (Reference Bijl, Sluijs and Brinkhuis2013a), which is adopted here. The other is the alternative magnetostratigraphic model performed in the La Meseta Formation by Montes et al. Reference Montes, Nozal, Santillana, Marenssi and Olivero2013, Reference Montes, Beamud, Nozal, Santillana, Crame, Acosta Hospitaleche and Gelfo2019a). Note the age contradiction between the two age models, whose difference is ∼11 Ma. Biogeographic distribution: endemic (E) in bold and cosmopolitan (C) according to Wrenn & Hart (Reference Wrenn, Hart, Feldman and Woodburne1988), Bijl et al. (Reference Bijl, Sluijs and Brinkhuis2013a) and Houben et al. (Reference Houben, Bijl, Pross, Bohaty, Passchier, Stickley, Röhl, Sugisaki, Tauxe, Flierdt, Olney, Sangiorgi, Sluijs, Escutia and Brinkhuis2013). The dinoflagellate cyst assemblages were semi-quantitatively characterized using the following scale: very rare: VR (1), rare: R (up to 5), frequent: F (up to 20), common: C (up to 40) and abundant: A (up to 100), very abundant: VA (more than 100) specimens per slide.
Palynomorphs were extracted from rocks using palynological procedures that involved the removal of the organic matter from 10 g of dry sediment by digesting the carbonates with HCl (10 %) and silicates with HF (40 %) followed by several washings with water. The residue was sieved with 40 µm and 10 µm meshes and finally mounted on slides with glycerin jelly (Wood et al. Reference Wood, Gabriel, Lawson, Jansonius and McGregor1996). Light microscopy observation was at 400× and 1000× magnifications using a Nikon Eclipse 80i, and images were taken with a Nikon Coolpix 950 digital camera, both pieces of equipment being available at the Laboratorio de Bioestratigrafía de Alta Resolución of the Universidad de Buenos Aires (FCEN-UBA), Argentina.
For each sample, it was possible to count a minimum of 300 specimens including dinoflagellate cysts, pollen, spores and other palynomorphs (see Appendix, and the online Supplementary Material available at https://doi.10.1017/S0016756819000591). Selected specimens are illustrated in Figures 3 and 4. In this work, only marine palynomorphs (mainly dinoflagellate cysts) were identified in terms of species level and analysed with the aim of obtaining biostratigraphic information. Pollen and spore analysis will be presented in a future contribution. The taxonomic nomenclature of dinoflagellate cysts used in this study was based on the database DINOFLAJ3 of Williams et al. (Reference Williams, Fensome and MacRae2017).

Fig. 3. Dinoflagellate cysts recovered from the La Meseta Formation, Seymour Island, Antarctic Peninsula. (a) Achomosphaera crassipellis, sample AM1-15 (BAFC-Pl 2511: X24). (b) Arachnodinium antarcticum Wilson & Clowes, 1982, sample AM2-5 (BAFC-Pl 2514: L40). (c) Cerebrocysta waipawaensis (Wilson, 1988) Fensome et al. 2009, sample AM1-9 (BAFC-Pl 2513: L59/3). (d) Deflandrea antarctica Wilson, 1967, sample AM2-4 (BAFC-Pl 2513: K21). (e) Deflandrea cygniformis Pöthe de Baldis, 1966, sample AM2-8 (BAFC-Pl 2515: W43/4). (f) Diphyes colligerum (Deflandre & Cookson, 1955) Cookson, 1965, sample AM2-2 (BAFC-Pl 2512: W34). (g) Enigmadinium cylindrifloriferum, sample AM1-9 (BAFC-Pl 2510: L17/1). (h) Enneadocysta dictyostila (Menéndez, Reference Menéndez1965) Stover & Williams, Reference Stover and Williams1995 emend. Sarjeant, Reference Sarjeant1981, sample AM1-2 (BAFC-Pl 2508: K22). (i) Enneadocysta brevistila Fensome et al. Reference Fensome, Guerstein and Williams2006, sample AM1-1 (BAFC-Pl 2507: S46). (j) Glaphyrocysta semitecta (Bujak in Bujak et al. 1980) Lentin & Williams, 1981, sample AM2-5 (BAFC-Pl 2514: K28/1). (k) Hystrichokolpoma sp., sample AM1-15 (BAFC-Pl 2511: L46). (l) Hystrichosphaeridium sp. cf. H. astartes (Sannemann, 1955) in Wrenn & Hart, Reference Wrenn, Hart, Feldman and Woodburne1988, sample AM1-2 (BAFC-Pl 2508: S49/4). Scale bar: 20 μm.
The dinoflagellate cyst assemblages were semi-quantitatively characterized using the following scale: very rare (1), rare (up to 5), frequent (up to 20), common (20 to 40), abundant (40–100) and very abundant (more than 100) specimens per slide (Fig. 2). The absolute values can be seen in the online Supplementary Material at https://doi.10.1017/S0016756819000591.
For palaeoenvironmental analyses, the following indexes proposed by Versteegh (Reference Versteegh1994) were considered: the P/G ratio, peridinioid versus gonyaulacoid dinoflagellate cysts and the S/D ratio, sporomorphs versus dinoflagellate cyst (Fig. 5). The P/G cyst ratio characterizes the palaeoproductivity of surface water and is obtained by the equation P/G = nP/(nP + nG), where n is the number of specimens counted, P are the peridinioid (or Protoperidinioid) dinoflagellate cysts and G include the Gonyaulacoid dinoflagellate cysts. The S/D ratio is used as a measure for terrestrial influence relative to marine influence and is calculated as follows: S/D = nS/(nD + nS) where n is the number of counted specimens, S is represented by sporomorphs (pollen and spores) and D by dinoflagellate cyst and acritarchs.

Fig. 4. Dinoflagellate cysts recovered from the La Meseta Formation, Seymour Island, Antarctic Peninsula. (a) Hystrichosphaeridium truswelliae Wrenn & Hart, Reference Wrenn, Hart, Feldman and Woodburne1988, sample AM2-5 (BAFC-Pl 2514: V27/2). (b) Impagidinium parvireticulatum, sample AM1-15 (BAFC-Pl 2511: B25/1). (c) Impletosphaeridium clavus (Wrenn & Hart, Reference Wrenn, Hart, Feldman and Woodburne1988) emend. Bowman et al. 2013, sample AM1-15 (BAFC-Pl 2511: P22/3). (d) Lejeunecysta sp., sample AM2-5 (BAFC-Pl 2514: C33/2). (e) Octodinium askiniae Wrenn & Hart, Reference Wrenn, Hart, Feldman and Woodburne1988, sample AM1-15 (BAFC-Pl 2511: T24). (f) Operculodinium centrocarpum (Deflandre and Cookson, 1955) Wall, 1967, sample AM1-15 (BAFC-Pl 2511: 22). (g) Selenopemphix nephroides Benedek, 1972, sample AM2-5 (BAFC-Pl 2514: O39/2). (h) Selenopemphix crenata Matsuoka & Bujak, 1988, sample (BAFC-Pl 2513: X21/3). (i) Spinidinium macmurdoense (Wilson) Lentin & Williams, 1976, sample AM1-15 (BAFC-Pl 2511: E29). (j) Thalassiphora pelagica (Eisenack, 1954) Eisenack & Gocht, 1960, sample AM2-4 (BAFC-Pl 2513: M32). (k) Turbiosphaera filosa (Wilson, 1967) Archangelsky, 1969, sample AM2-5 (BAFC-Pl 2514: Z17/4). (l) Vozzhennikovia apertura (Wilson, 1967b) Lentin & Williams, 1976, sample AM2-4 (BAFC-Pl 2513: V19/1-3). Scale bar: 20 μm.
Palynological slides are stored in the Palynological Collection of the Facultad de Ciencias Exactas y Naturales, Universidad de Buenos Aires (FCEN-UBA), Argentina, under the acronym BAFC-Pl and the slide numbers 2507–2515, 2645–2646 and 2683–2684.
4. Results
4.a. Dinoflagellate cysts
All samples provided abundant and well-preserved marine palynomorphs including dinoflagellate cysts, acritarchs, microforaminiferal linings, as well as continental taxa such as spores and pollen, freshwater algae and fungi, in variable percentages along the whole section. Reworked Permian taeniate pollen, and Late Cretaceous and Palaeocene dinoflagellate cysts are also present in the analysed samples (Fig. 2).
A total of 38 dinoflagellate cyst species were recorded, 12 of which are part of the Antarctic-endemic assemblage, that consist of species whose distribution is restricted to latitudes south of 45° S (see definition in Bijl et al. Reference Bijl, Pross, Warnaar, Stickley, Huber, Guerstein, Houben, Sluijs, Visscher and Brinkhuis2011). The rest of the species have a cosmopolitan distribution (Lentin & Williams, Reference Lentin and Williams1980; Bijl et al. Reference Bijl, Pross, Warnaar, Stickley, Huber, Guerstein, Houben, Sluijs, Visscher and Brinkhuis2011, Reference Bijl, Sluijs and Brinkhuis2013a).
Among the endemic species, Enneadocysta dictyostila is well represented in all samples, reaching high values in most of them (Fig. 2). The closely related species E. brevistila is recorded only in some samples and in small amounts. Together with E. dictyostila, Deflandrea antarctica was also present throughout the whole unit in variable abundances (Fig. 2). Vozzhennikovia apertura is also an endemic species. It occurs in most of the samples, being abundant in the upper part of the section, in Acantilados I (sample AM1-2) and Cucullaea I horizons (samples AM2-4 and AM2-5). Arachnodinium antarcticum, Spinidinium macmurdoense and Octodinium askiniae are documented throughout the section, with different abundances.
The small dinoflagellate cysts that are also members of the endemic assemblages are the species Enigmadinium cylindrifloriferum and Impletosphaeridium clavus. The first one is abundant in the lowest samples, in Valle de las Focas (sample AM1-1) and Acantilados I (sample AM1-2) allomembers, and occasionally appears in some of the uppermost samples but is poorly represented. Impletosphaeridium clavus was recorded in some of the samples but always in very low proportions (Fig. 2).
Species with cosmopolitan distribution were recorded along the whole section but, except for Lejeunecysta spp. which is well-represented in the Campamento Allomember (sample AM2-2) and Thalasiphora pelagica which was particularly abundant in one sample of the Cucullaea I Allomember (sample AM2-5), they never dominate the assemblages (Fig. 2). Other cosmopolitan taxa that are present as frequent elements in the LMF are Achomosphaera crassipellis, which was recorded in the Campamento Allomember (sample AM2-2 with 15 specimens), and Cerebrocysta waipawaensis in Acantilados I (sample AM1-9) and Cucullaea I (samples AM2-4 and AM2-5) allomembers.
Other marine palynomorphs recorded in the section include the marine algae Paralecaniella indentata in Acantilados II (sample AM1-15), Palambages sp. in Campamento (sample AM2-2) and Cucullaea I (samples AM2-4 and AM2-5) and other prasinophytes in Cucullaea I (sample AM2-8). Just one specimen of the acritarch Veryhachium sp. is found in the Valle de las Focas (sample AM1-1) Allomember while microforaminiferal linings are spread over some samples but in all cases with few specimens.
The reworked specimens documented in this study include the Late Cretaceous species Isabelidinium cretaceum, Isabelidinium sp., Odontochitina operculata, Oligosphaeridium pulcherrimum and Palaeocystodinium australinum, and Palaeoperidinium pyrophorum was probably reworked from lower Palaeocene Seymour sections (online Supplementary Material, at https://doi.10.1017/S0016756819000591).
5. Discussion
5.a. Comments about the middle Eocene index species Enneadocysta dictyostila
In palynological studies carried out prior to 1995, the species Enneadocysta dictyostila (Menéndez, Reference Menéndez1965) Stover & Williams, Reference Stover and Williams1995 emend. Sarjeant, Reference Sarjeant1981 was published as Areosphaeridium diktyoplokum (Klumpp, Reference Klumpp1953) emend. Eaton, Reference Eaton and Farinacci1971, Stover & Williams, Reference Stover and Williams1995; the latter being wide-distribution taxa from the Northern Hemisphere. Nevertheless, Stover & Williams (Reference Stover and Williams1995) taxonomically differentiated Enneadocysta dictyostila (as E. partridgei) from Areosphaeridium diktyoplokum, and this allowed assigning the specimens of the Southern Hemisphere as Enneadocysta dictyostila, separating them from Areosphaeridium diktyoplokum, from the Northern Hemisphere. It was only after this separation that Enneadocysta dictyostila was recognized as a member of the Antarctic-endemic assemblage, and it was subsequently reinforced by other authors (e.g. Hannah, Reference Hannah1997; Truswell, Reference Truswell1997; Sluijs et al. Reference Sluijs, Brinkhuis, Stickley, Warnaar, Williams, Fuller, Exon, Kennett and Alone2003). Moreover, Stover & Williams (Reference Stover and Williams1995) established a spatial differentiation between the two species based on the recognition that all records of Areosphaeridium diktyoplokum correspond to the Northern Hemisphere while Enneadocysta dictyostila (as E. partridgei) is restricted to the Southern Hemisphere with a wide distribution (different localities of the SW Atlantic Ocean, Antarctica, Tasmania, Australia and New Zealand; Guerstein & Daners, Reference Guerstein and Daners2010; Bijl et al. Reference Bijl, Pross, Warnaar, Stickley, Huber, Guerstein, Houben, Sluijs, Visscher and Brinkhuis2011; Douglas et al. Reference Douglas, Affek, Ivany, Houben, Sijp, Sluijs, Schouten and Pagani2014; Daners et al. Reference Daners, Guerstein, Amenábar and Morales2016).
Fensome et al. (Reference Fensome, Guerstein and Williams2006) re-examined specimens from eastern Canada and southern Argentina in order to differentiate the two genera. They concluded that, although both species are superficially similar, they differ in the number of the antapical processes, the shape of the central body and the morphology of the processes. While Enneadocysta has two antapical processes (more or less equal size at the antapex, neither of which is centrally located or distinctively large), Areosphaeridium has a single one, centrally located, that is commonly larger than other processes on the same specimen. Besides, Enneadocysta tends to have a lenticular central body and an asymmetrical overall shape while Areosphaeridium has a subspherical central body and symmetrical shape (Fensome et al. Reference Fensome, Guerstein and Williams2006). Regarding the process morphology, both Enneadocysta and Areosphaeridium can have licrate (solid or closed processes in which the distal endings are drawn out, commonly asymmetrically, into two orthogonal or curved branches that are serrate or denticulate on the outer margin sensu Sarjeant, Reference Sarjeant1982 in Fensome et al. Reference Fensome, Guerstein and Williams2006) or clypeate (a process bearing a distal perforate, quadrate to polygonal platform processes sensu Fensome & Williams (Reference Fensome and Williams2005) in Fensome et al. Reference Fensome, Guerstein and Williams2006) processes. Nevertheless, in Enneadocysta dictyostila the processes vary distally from bifid to most commonly ragged clypeate and, in contrast, Areosphaeridum diktyoplokum has predominantly entire clypeate distal process terminations (Fensome et al. Reference Fensome, Guerstein and Williams2006).
Most of the palynological contributions on Antarctic sections (Wrenn & Hart, Reference Wrenn, Hart, Feldman and Woodburne1988; Cocozza & Clarke, Reference Cocozza and Clarke1992; Askin, Reference Askin and Ricci1997) agree to assign Antarctic specimens to Areosphaeridium cf. diktyoplokus, which first appears in the late early Eocene in the Northern Hemisphere. Therefore all the mentioned authors dated LMF as late early to late Eocene. For instance, Wrenn & Hart (Reference Wrenn, Hart, Feldman and Woodburne1988) reported the presence of Areosphaeridum diktyoplokum in the LMF. They based the age of the unit on the stratigraphical range of Areosphaeridum diktyoplokum and they also considered it as a cosmopolitan species that spans from the late early Eocene to the Oligocene. They dismissed the comments of Goodman & Ford (Reference Goodman and Ford1983) who stated that the specimens referable to Areosphaeridum diktyoplokus from localities of the Southern Hemisphere have incomplete margins similar to those specimens they recovered from the uppermost Eocene / lower Oligocene from the Falkland Plateau (SW Atlantic) and they referred to Areosphaeridum sp. cf. A. diktyoplokum. Goodman & Ford (Reference Goodman and Ford1983) pointed out that none of the Southern Hemisphere specimens should be attributed to A. diktyoplokus s.s. Finally, Wrenn & Hart (Reference Wrenn, Hart, Feldman and Woodburne1988) end up stating that Northern and Southern dinoflagellate cysts assigned to Areosphaeridum diktyoplokum are a single species whose biostratigraphical distribution ranges from the late early Eocene to early Oligocene. But lastly, Douglas et al. (Reference Douglas, Affek, Ivany, Houben, Sijp, Sluijs, Schouten and Pagani2014) assigned the Antarctic specimens to Enneadocysta dictyostila following the dinoflagellate cyst zonation applied to the Southern Ocean (Bijl et al. Reference Bijl, Sluijs and Brinkhuis2013a).
Based on all the above remarks and in agreement with Douglas et al. (Reference Douglas, Affek, Ivany, Houben, Sijp, Sluijs, Schouten and Pagani2014) we consider that the specimens here studied from the LMF are assignable to Enneadocysta dictyostila instead of Areosphaeridum cf. diktyoplokum.
5.b. Biostratigraphy and correlation based on dinoflagellate cysts
The biostratigraphic analysis here presented is based on dinoflagellate cyst zonation applied to the entire Southern Ocean proposed by Bijl et al. (Reference Bijl, Sluijs and Brinkhuis2013a). These authors calibrated the Palaeogene dinoflagellate cyst assemblages obtained from the Ocean Drilling Program (ODP) holes from the Tasmanian Platform and the Wilkes Land margin with the Geomagnetic Polarity Time Scale of Vandenberghe et al. (Reference Vandenberghe, Speijer, Hilgen, Gradstein, Ogg, Schmitz and Ogg2012) using magnetostratigraphy and stable isotope stratigraphy. They distinguished 13 dinoflagellate cyst zones (SPDZ 1–13) for the late Palaeocene to late Eocene (58–36 Ma) using the first occurrences (FO), last occurrences (LO), first common (∼25 %) occurrences (FCO) and last common (∼25 %) occurrences (LCO) of dinoflagellate cyst species to define boundaries between the zones. In the present work, it is assumed the synchronicity of bioevents occurred at the East Tasmania platform (65° S palaeolatitude), Wilkes Land (67° S) and Marambio (Seymour) Island (64° S). So, considering the Bijl et al. (Reference Bijl, Sluijs and Brinkhuis2013a) zonation scheme, we observed the following occurrence of dinoflagellate cyst species in the LMF (Fig. 2).
Deflandrea antarctica (FO: 58.20, LO: ∼36.00 Ma) and Enneadocysta dictyostila (FO: 46.2 Ma; FCO: 45.20, LCO: 35.5 Ma; LO: 33.40 Ma) are recorded in all samples in the whole section. This indicates that the age of the LMF is between 46.20 and 36.00 Ma, where the base is defined by the FO of Enneadocysta dictyostila and the top is marked by the co-occurrence of Deflandrea antarctica and Enneadocysta dictyostila.
Arachnodinium antarcticum (FO: 48.70, LO: 36.00 Ma) and Spinidinium macmurdoense (FO: 55.50, LO: 35.95 Ma) first occur in the upper part of the Acantilados I Allomember (sample AM1-9). The first species reaches the upper sample of Cucullaea I Allomember (sample AM2-8) while the second extends to the top of the LMF.
Impagidinium parvireticulatum (FO: 43.7 Ma, LO: 35.5 Ma) is present in the Acantilados I (AM1-9) and the Acantilados II (AM1-15) allomembers. This means that sample AM1-15 could not be younger than 43.7 Ma.
Glaphyrocysta semitecta (FO: 39.70, LO: 39.10 Ma) and Deflandrea cygniformis (FO: 40.00, LO: 35.90 Ma) occur in sample AM2-5 in the Cucullaea I Allomember. G. semitecta is present only in this allomember, but D. cygniformis continues in sample AM2-8. Thus, from sample AM2-5 to AM2-8 (Cucullaea I Allomember) the unit has a maximum age of 39.10 Ma.
The FO of Deflandrea convexa is 58.20 Ma (Bijl et al. Reference Bijl, Sluijs and Brinkhuis2013a), and Brinkhuis et al. (Reference Brinkhuis, Munsterman, Sengers, Sluijs, Warnaar, Williams, Exon, Kennett and Malone2003) gave a LO at 35.8 Ma. The presence of a single specimen of Deflandrea sp. cf. D. convexa in sample AM2-8 (BAFC-Pl 2515) indicates that the Cucullaea I Allomember could not be younger than 35.8 Ma.
Diphyes colligerum (FO: 40.60, LO: 39.10 Ma) occurs in sample AM2-2 (Campamento Allomember), indicating that this allomember could not be younger than 39.10 Ma.
Some long-ranging species are also part of the assemblage and their ranges fall within the lapse between 45.20 and 36.00 Ma. They are Thalassiphora pelagica (FO: 55.30, LO: ∼ 36.00 Ma), Vozzhennikovia apertura (FO: 65.10, LO: 35.5 Ma) and Spinidinium macmurdoense (FO: 55.50, LO: 35.95 Ma) among others.
The base of the LMF (the lowest sample AM1-1 in Valle de las Focas Allomember) is correlatable with Zone SPDZ10 (middle Lutetian, 46.2–45.2 Ma) in which Enneadocysta dictyostila has its FO. This zone is characterized by the alternation of Deflandrea antarctica, Enneadocysta multicornuta and Enneadocysta dictyostila. Although E. multicornuta is not recorded in the LMF, the fluctuation of D. antarctica and E. dictyostila is observable in the unit and the species are present as abundant and common elements, respectively (Fig. 2). Samples AM1-2 to AM1-8 (Valle de las Focas to Acantilados I Allomembers) of LMF are comparable to Zone SPDZ11 (middle Lutetian, 45.2–44.0 Ma) defined by the FCO of Enneadocysta dictyostila, and samples AM1-9 to AM2-8 (Acantilados I to Cucullaea II Allomembers) are comparable to Zone SPDZ12 (44.0–40.0 Ma, middle Lutetian to early Bartonian) defined by the FO Impagidinium parvireticulatum of Bijl et al. (Reference Bijl, Sluijs and Brinkhuis2013a). The SPDZ13 (Bartonian–Priabonian, 40.0–35.95 Ma) is not easily correlated with LMF because neither the species of the base nor the top are found in the Antarctic samples; only the characteristic species of the zone such as Spinidinium macmurdoense, Vozzhennikovia apertura and Glaphyrocysta semitecta are recorded. Nevertheless, a significant event in Zone SPDZ13 is the FO Deflandrea cygniformis; this species occurs in sample AM2-8 (Cucullaea I Allomember), but only in this sample, and has no continuous record in the section. It is important to point out that Spinidinium macmurdoense is an abundant element in the uppermost sample (AM3-2) of the LMF and in Zone SPDZ13 this species has its FCO, suggesting that Zone SPDZ13 would be recognized in the LMF, but the equivalence of both dinoflagellate cyst assemblages is not assured.
LMF is also equivalent to zones RTF1 (middle Lutetian, ∼46 Ma) and RTF2 (middle Lutetian to late Bartonian, 46–39 Ma) and probably to RTF3 (late Bartonian to middle Priabonian, 39–36 Ma) as proposed by González Estebenet et al. (Reference González Estebenet, Guerstein, Rodríguez Raising, Ponce and Alperín2016) for the Río Turbio Formation (upper member), Santa Cruz province, Austral Basin, Argentina. The zones of the Austral Basin were calibrated with the South West Pacific Ocean zonation (Bijl et al. Reference Bijl, Sluijs and Brinkhuis2013a) and complemented with isotopic ages of 87Sr/86Sr (Casadío et al. Reference Casadío, Griffin, Marenssi, Net, Parras, Rodriguez Raising and Santillana2009), foraminifera (Malumián, Reference Malumián1990) and calcareous nannofossils (Concheyro, Reference Concheyro1991) data obtained from the Río Turbio Formation (upper member). Zone RTF 1 is correlated with Zone SPDZ10 of Bijl et al. (Reference Bijl, Sluijs and Brinkhuis2013a), because of the co-dominance of Deflandrea antarctica and Enneadocysta dictyostila, being the FO of the E. dictyostila, a significant event in this zone. The same is observed in the lowermost LMF (sample AM1-1). The base of RTF 2 is equivalent to the base of Zone SPDZ11, both defined by the FCO of Enneadocysta dictyostila, while the upper part of RTF 2 is correlated with the SPDZ12 based on the range of the characteristic species of both zones. The FCO of Enneadocysta dictyostila is recorded in samples AM1-2 to AM3-1 of the LMF (Valle de las Focas to Cucullaea II allomembers). Zone RTF 3 is equivalent to Zone SPDZ 13 of Bijl et al. (Reference Bijl, Sluijs and Brinkhuis2013a) due to the FCO of Spinidinium macmurdoense that is recognizable in both the Austral and South Pacific Ocean dinoflagellate cyst biozonation schemes. Although in the uppermost LMF (sample AM3-2) S. macmurdoense increases in number of specimens in comparison with the samples below it, the species is considered as an abundant (A: 40 to 100 specimens, Fig. 2) and not very abundant (VA: more than 100 specimens, Fig. 2) element as in the biozonation schemes. Besides, the base of Zone RTF 3 is defined by the LO of Arachnodinium antarcticum and Hystrichosphaeridium truswelliae, but in the LMF the H. truswelliae disappears earlier than A. antarcticum (Fig. 2). However, the LO of Impagidinium parvireticulatum and the LCO of Enneadocysta dictyostila are two significant events dated at the middle Priabonian (Bijl et al. Reference Bijl, Sluijs and Brinkhuis2013a). The same is seen in sample AM2-8 (Cucullaea I) of the LMF, which could indicate its equivalence to Zone RTF 3. Because the distribution of the dinoflagellate cyst species of the LMF has some important differences from Zone RTF 3, the correlation between the two assemblages is not well defined. The same occurs with the correlation between the LMF and Zone SPDZ13.
González Estebenet et al. (Reference González Estebenet, Guerstein, Rodríguez Raising, Ponce and Alperín2016) recognized Zone RTF 1 in the base of La Despedida Formation, Tierra del Fuego province (Argentina), while Zone RTF 2 was documented in the Man Aike Formation, Santa Cruz province, and in the Leticia Formation (Río de la Turba section), Tierra del Fuego province, both in Argentina. Thus, the lowest LMF (sample AM1-1) is correlatable with Río Turbio (upper member) and the base of La Despedida formations, whereas the rest of the LMF is correlatable with the Río Turbio (upper member), Man Aike and Leticia (Río de la Turba section) formations. The correlation and comparison between high south latitude localities of the Austral Basin have been carried out by González Estebenet et al. (Reference González Estebenet, Guerstein, Rodríguez Raising, Ponce and Alperín2016) taking into account the presence/absence of species rather than abundance data. Comparison of the dinoflagellate cyst assemblages of the James Ross Basin (Antarctic Peninsula) and the Austral Basin (south of Argentina), based on the quantitative data, is a work in progress and will give more strength to the correlation from localities of the Drake Passage area.
The new analysis of dinoflagellate cysts presented here complements the study of Douglas et al. (Reference Douglas, Affek, Ivany, Houben, Sijp, Sluijs, Schouten and Pagani2014), confirming the age of the LMF, and especially adding to the study the lowest part of the unit (corresponding to Telm 1 that has not been analysed by the mentioned authors) suggesting a middle Lutetian to Priabonian age (∼46.2 to ∼36 Ma) for the LMF, younger than the one documented so far.
As was mentioned in Section 2.c.2 above, there is a recent age model proposed for the LMF. This model merits inclusion in this discussion since it has been carried out in the LMF itself and the palynological samples analysed herein were collected during the geological mapping of the succession for the new geological cartography of Seymour Island proposed by Montes et al. (Reference Montes, Nozal, Santillana, Marenssi and Olivero2013, Reference Montes, Nozal, Olivero, Gallastegui, Maestro, Santillana, Martín-Serrano, Montes, Nozal and Santillana2019b). Based on the combination of isotopic data (87Sr/86Sr), magnetostratigraphy and lithostratigraphy in relation to the global curves of sea level, the age model offers a late Thanetian to early Lutetian age (∼56.4 to ∼45.8 Ma) for the LMF. So, the age here obtained based on dinoflagellate cysts involves a hiatus of 11 Ma at the base of the LMF with respect to the top of the underlying Cross Valley – Wiman Formation (56.9 Ma; Beamud et al. Reference Beamud, Montes, Santillana, Nozal and Marenssi2015; Montes et al. Reference Montes, Beamud, Nozal, Santillana, Crame, Acosta Hospitaleche and Gelfo2019a). This hiatus would imply the absence of the Ypresian (lower Eocene) and a large part of the lower Lutetian. Such a hiatus of 11 Ma, would not be caused by eustatic sea-level drop alone; rather, it could be due to tectonic–erosive mixed origin and thus a tectonic control for the incised valley of LMF considering the proposal of Marenssi et al. (Reference Marenssi, Santillana, Rinaldi and Casadío1998) about the cause of the unconformities in the unit. In contrast, the age for the LMF proposed in the magnetostratigraphic scheme by Montes et al. (Reference Montes, Beamud, Nozal, Santillana, Crame, Acosta Hospitaleche and Gelfo2019a) would not involve such a hiatus, explaining how continued sedimentation would have prevailed during the regressive and gradual evolution in the filling of the James Ross Basin.
6. Palaeoceanographic and palaeoenvironmental implications
6.a. Palaeoceanography during the Eocene in the Southern Ocean and surrounding areas
Climatic and tectonic changes occurring during the Palaeogene strongly influenced the distribution of dinoflagellate cyst assemblages in the Southern Ocean (Stickley et al. Reference Stickley, Brinkhuis, Schellenberg, Sluijs, Röhl, Fuller, Grauert, Huber, Warnaar and Williams2004; Bijl et al. Reference Bijl, Sluijs and Brinkhuis2013a; Guerstein et al. Reference Guerstein, Daners, Palma, Ferreira, Premoar, Amenábar and Belgaburo2016). During the Palaeocene and the early Eocene circum-Antarctic dinoflagellate cysts, assemblages were dominated by cosmopolitan early Palaeogene taxa while Antarctic-endemic species were rare (Bijl et al. Reference Bijl, Sluijs and Brinkhuis2013a, b). In the early middle Eocene (50–48 Ma), endemic taxa began to dominate the dinoflagellate cyst assemblages in the Southern Ocean south of 45° S, i.e. areas east of the Tasmanian Gateway and Drake Passage (Bijl et al. Reference Bijl, Pross, Warnaar, Stickley, Huber, Guerstein, Houben, Sluijs, Visscher and Brinkhuis2011). The dominance of endemic dinoflagellates became stronger at least until the mid- to late Eocene (38–36 Ma). Huber et al. (Reference Huber, Brinkhuis, Stickley, Döös, Sluijs, Warnaar, Schellenberg and Williams2004) and González Estebenet et al. (Reference González Estebenet, Guerstein and Alperin2014) explained that the distribution of the middle–late Eocene endemic taxa in the Southern Ocean would be in response to surface ocean-circulation pattern characterized by wide clockwise subpolar gyres surrounding Antarctica that favoured the endemism of the species tolerant to relatively low surface-water temperatures. Towards the Eocene/Oligocene transition, endemic species became extinct as a consequence of the deepening of the Tasmanian Gateway and Drake Passage (Sluijs et al. Reference Sluijs, Brinkhuis, Stickley, Warnaar, Williams, Fuller, Exon, Kennett and Alone2003; Stickley et al. Reference Stickley, Brinkhuis, Schellenberg, Sluijs, Röhl, Fuller, Grauert, Huber, Warnaar and Williams2004; Guerstein et al. Reference Guerstein, Brinkhuis and Daners2008; Houben et al. Reference Houben, Bijl, Pross, Bohaty, Passchier, Stickley, Röhl, Sugisaki, Tauxe, Flierdt, Olney, Sangiorgi, Sluijs, Escutia and Brinkhuis2013), and were replaced by Oligocene cosmopolitan taxa, typically heterotrophic species (protoperidinacean) with modern analogues that live today in sea-ice environments (Houben et al. Reference Houben, Bijl, Pross, Bohaty, Passchier, Stickley, Röhl, Sugisaki, Tauxe, Flierdt, Olney, Sangiorgi, Sluijs, Escutia and Brinkhuis2013).
In reference to the establishment of dominantly Antarctic-endemic assemblage in the middle Eocene, Bijl et al. (Reference Bijl, Pross, Warnaar, Stickley, Huber, Guerstein, Houben, Sluijs, Visscher and Brinkhuis2011) considered that in the early middle Eocene (∼50 Ma) the dominance of the Antarctic-endemic taxa has little relation to the sea surface temperature (SST) because the SSTs did not decrease abruptly at that time. In contrast, during the middle Eocene, when the endemic assemblage was well established, the SST controlled the abundances of endemic species. The causes of the proliferation of the endemic assemblage just after 50 Ma would be linked to other physicochemical parameters of the surface waters, such as large-scale changes in surface-ocean nutrient availability. That ocean richness would be generated by a significant diatom production recorded at 47 Ma onward, as a consequence of the global cooling occurring in the middle Eocene that increased latitudinal gradients and stimulated upwelling, bringing waters enriched in silica to the surface for utilization by the diatom walls. Besides, increased weathering may have led an additional nutrient supply to the oceans, especially the co-limitation diatom nutrients, Si and Fe. The diatom production caused a rapid reorganization of Southern Ocean dinoflagellate cyst assemblages. Once the endemic dinoflagellate cyst species came to dominate the assemblages in the early middle Eocene, they were more controlled by SST variations than during the Palaeocene and early Eocene (Bijl et al. Reference Bijl, Houben, Schouten, Bohaty, Sluijs, Reichart and Sinnighe Damste2010, Reference Bijl, Pross, Warnaar, Stickley, Huber, Guerstein, Houben, Sluijs, Visscher and Brinkhuis2011).
On the other hand, González Estebenet et al. (Reference González Estebenet, Guerstein and Alperin2014) carried out a qualitative comparison (presence/absence of species) between the middle Eocene dinoflagellate cyst assemblages from localities to the north (upper member of Río Turbio, Man Aike and Leticia formations of the Austral Basin) and south (La Meseta Formation of the Antarctic Peninsula and core IO1578-59 in Bruce Bank of the Scotia Sea) of the Drake Passage area. They observed that, although both areas surrounding the Drake Passage were characterized by a high presence of the Antarctic-endemic species, a notable difference in non-endemic taxa existed between the north and the south localities and these differences might be due to the influence of surface waters from different sources. The sites located to the south of Drake Passage (Antarctic Peninsula and the Scotia Sea) would reflect the influx of surface waters originating from Antarctic coasts and directed northwards through the clockwise subpolar gyre known as the proto-Weddell Gyre (p-WG). On the other hand, the shallow flows through the incipient opening of Drake Passage in the early Eocene (Scher & Martin, Reference Scher and Martin2006; Livermore et al. Reference Livermore, Hillenbrand, Meredith and Eagles2007; Barbeau et al. Reference Barbeau, Olivero, Swanson-Hysell, Zahid, Murray and Gehrels2009) would have allowed the input of shallow waters with cosmopolitan taxa from the Pacific to the South Atlantic Ocean. Thus, the cosmopolitan taxa would have been subsequently transported together with the Antarctic-endemic components into the Austral Basin by the northwestern sector of the p-WG.
6.b. Palaeoenvironment of the La Meseta Formation
The usefulness of dinoflagellate cysts in providing age determinations within the Palaeogene of the South Atlantic Ocean and, consequently, their potential for stratigraphically correlating different basins of that region has been well established (e.g. Pross & Brinkhuis, Reference Pross and Brinkhuis2005). Besides this, dinoflagellate cysts are also useful as proxy indicators for environmental conditions (Sluijs et al. Reference Sluijs, Pross and Brinkhuis2005) because of their high sensitivity to physicochemical changes of surface water, such as salinity, temperature and depth, and also in productivity (i.e. the trophic levels). Thus, the palaeoproductivity of surface water can be estimated using the relationship between peridinioid (P) cysts and gonyaulacoid (G) cysts by means of the P/G cyst ratio. We assumed that the dinoflagellate generators of P cysts of extinct species would have had the same heterotrophic habit as the living dinoflagellate genus Protoperidinium, while the G cysts would have been originated by dinoflagellates with an autotrophic or mixotrophic behaviour (Sluijs et al. Reference Sluijs, Pross and Brinkhuis2005; Esper & Zonneveld, Reference Esper and Zonneveld2007). Therefore, the high relative abundance of P cysts could indicate a high concentration of dissolved nutrients of surface water, e.g. upwelling or estuaries (Goodman & Ford, Reference Goodman and Ford1983; Mao & Mohr, Reference Mao and Mohr1995; Brinkhuis et al. Reference Brinkhuis, Munsterman, Sengers, Sluijs, Warnaar, Williams, Exon, Kennett and Malone2003; Sluijs et al. Reference Sluijs, Brinkhuis, Stickley, Warnaar, Williams, Fuller, Exon, Kennett and Alone2003, among others). In contrast, the high relative abundance of G cysts would suggest nutrient-poor waters, e.g. outer shelf or oceanic waters. In terms of the index values, a ratio of 0.5–1 is indicative of higher-productivity waters, while the ratio of 0–0.5 indicates less productive waters.
On the other hand, the S/D ratio, sporomorphs (pollen and spores) versus dinoflagellate cysts, is used as a measure for terrestrial influence relative to marine influence whereby an increase in pollen and spores relative to dinoflagellate cysts is interpreted as a sea level lowering as the section becomes more proximal to the coast. Therefore, an S/D ratio of 0.5–1 indicates more proximal environments while a ratio of 0–0.5 means a more distal position. Thus, the S/D ratio was employed together with the P/G ratio in deducing the depositional environment and the direction of the trend line which reflects the regression or progression of the shoreline.
Previous sedimentological evidence suggests that LMF is indeed a composite valley fill containing not only estuarine but also deltaic and shallow-marine sediments (Stilwell & Zinsmeister, Reference Stilwell and Zinsmeister1992; Marenssi et al. Reference Marenssi, Santillana, Rinaldi and Casadío1998, Reference Marenssi, Net and Santillana2002). So, the joint analysis of the frequency distribution of dinoflagellate cysts, P/G and S/D ratios and the results of the sedimentological study of the LMF allows us to reconstruct the palaeoenvironment and surface water conditions during the middle to late Eocene in the study area.
The P/G ratio varied little throughout the section. In Valle de las Focas, Acantilados I and Acantilados II allomembers, the P/G values are below 0.5, indicating poor productive waters; these samples are characterized by a high number of autotrophic dinoflagellates (G – note the large number of Enneadocysta specimens counted; Fig. 2; online Supplementary Material at https://doi.10.1017/S0016756819000591). The samples with the lowest P/G values are AM1-7 (P/G: 0.07), AM1-9 (P/G: 0.08) and AM1-8 (P/G: 0.11); they would indicate the least productive waters (Fig. 5). That lowest productivity is coincident with the presence of calcareous nannofossils which were recorded only in these three samples from the thirteen processed samples of the entire LMF (Concheyro et al. Reference Concheyro, Amenábar, Santillana, Montes Santiago and Borja Nozal Martín2016) suggests somewhat more open marine conditions. Moreover, dinoflagellate cyst assemblages from the Southern Ocean (ODP Leg 189, Site 1170) recorded the highest Enneadocysta spp. that is correlatable with high CaCO3 content in sediments possibly reflecting more offshore, less eutrophic marine conditions (Röhl et al. Reference Röhl, Brinkhuis, Stickley, Fuller, Schellenberg, Wefer, Williams, Exon, Malone and Kennett2004). Turning to the LMF, the P/G ratio values greater than 0.5 are observed in samples AM3-2 (P/G: 0.92), AM2-4 (P/G: 0.67) and AM3-1 (P/G: 0.57), i.e. Campamento, Cucullaea I and Cucullaea II allomembers, evidencing more productive waters. This means that from Campamento to Cucullaea II allomembers, a tendency to more productive waters or closer to the coast is suggested. In these samples an increase in the number of heterotrophic peridinioid dinoflagellates, such as Deflandrea and Vozzhennikovia (Fig. 5), is noticeable.

Fig. 5. Abundances (number of species) of gonyaulacoides and peridinoides cyst species per sample. Peridinioid/Gonyaulacoid curve (G/P index). The ratio of the abundance of gonyaulacoid versus peridinioid dinoflagellate cysts can reflect the productivity (Versteegh, Reference Versteegh1994). Sporomorph/Dinoflagellate cyst curve (S/D index). The S/D curve plots the continental versus marine influences. The presence of dinoflagellate cysts is indicative of marine influence, whereas the presence of spores and pollen reflects terrestrial influences.
Referring to the S/D ratio, LMF displays low values in most of the samples (S/D < 0.5, Fig. 5). These low values fluctuate throughout the section, but in sample AM2-2 (Campamento Allomember) the S/D ratio is c. 0.5, reaching the highest value in sample AM3-1 of the Cucullaea II Allomember (S/D: 0.515, Fig. 5). Sample AM3-1 reflects the closest condition to the coast and is the only sample in which the number of pollen and spore specimens exceeds the number of dinoflagellate cysts. The scarce dinoflagellate cysts are represented by Enneadocysta dictyostila and Vozzhennikovia apertura. The general increase in the S/D ratio observed from Campamento to Cucullaea II allomembers means an increase in terrestrial influence. This compositional change reflects a shift in the depositional environment from a more distal setting to one closer to the shoreline, i.e. a shallowing-upwards trend, or deposition further up inside the La Meseta estuary. The overall trend through the section is for a move from marine-dominated assemblages (Valle de las Focas to Acantilados II allomembers) to more terrestrially dominated assemblages (from Campamento to Cucullaea II allomembers). This would probably be a consequence of infilling of the incised valley estuary system within which these sediments were being deposited; within this are smaller-scale changes (cf. JC Greenhalgh, unpub. PhD thesis, UCL, Univ. London, 2003). Fluctuations within the individual members can be attributed to the large variation in environments and energy settings within an estuary. Differences between the individual members, e.g. across unconformable boundaries, reflect relative sea level change, caused by eustatic sea level changes, localized tectonism, sedimentary infill or a combination of these events (Porebski, Reference Porebski1995).
Worth taking into consideration is the small spiny palynomorph assigned to the species Enigmadinium cylindrifloriferum which is abundant in the Valle de las Focas and lower samples of Acantilados I allomembers (Fig. 5). This species was defined by Wrenn & Hart (Reference Wrenn, Hart, Feldman and Woodburne1988) as a chorate dinoflagellate cyst of the Order Gonyaulacales, while recently Williams et al. (Reference Williams, Fensome and MacRae2017) considered it as an acritarch. This duality in the assignment is founded on the position of the processes (non-tabular) and the doubtful existence of an archaeopyle in these spiny forms. Depending on whether it is considered to be an acritarch or a dinoflagellate cyst, the palaeoenvironmental interpretation differs. In this sense, some authors (Smith, Reference Smith1992; Schrank, Reference Schrank2003) proposed that the abundance of small acritarchs may be related to depositional environment; e.g. the small spiny acritarch Micrhystridium reaches greater abundances and diversity in shallow marine sandstones (in relatively coarse sediments), while it is much less frequent in open marine facies (finer-grained sediments) characterized by diverse dinoflagellate cyst assemblages. However, assuming that these small spiny palynomorphs are dinoflagellate cysts, it is expected to be considered that the lifestyle of the dinoflagellates produced these cysts; this implies their affinity to gonyaulacacean or peridinacean cysts, with autotrophic or heterotrophic habits, respectively. Taking into account the highest relative abundances of E. cylindfloriferum registered in the lowest samples of the LMF (Fig. 5), this can be linked to a more distal environment with low productivity reflected by the low S/D and P/G ratio values (whether it is considered as a dinoflagellate cyst or an acritarch). Cocozza & Clarke (Reference Cocozza and Clarke1992) suggested stressed marine depositional conditions in a shallow water environment for the lowermost part of the LMF. They support such palaeoenvironment on the assumption that low-diversity, high-dominance dinoflagellate cyst assemblages are compatible with shallow, inshore conditions (e.g. Goodman, Reference Goodman1979), i.e. low-salinity or estuarine/brackish. E. cylindfloriferum seems to be restricted to the lower part of the LMF, suggesting that the stressed marine-influenced conditions existed only in that part of the section, e.g. deposition was occurring more proximally. In spite of the fact that the species decreases in abundance towards to the middle and upper part of the section until it almost disappears, a single specimen is registered in sample AM2-5 (Cucullaea I allomember). This would indicate that its presence in the assemblage is related to a facial factor or the stress palaeoenvironmental conditions.
7. Conclusions
Although the LMF has previously been analysed in terms of its dinoflagellate cyst assemblages, the re-study of the unit presented here has allowed us to reach new conclusions as follows:
The age of the LMF – from Valle de las Focas to Cucullaea II allomembers (sensu Montes et al. Reference Montes, Nozal, Santillana, Marenssi and Olivero2013) – is middle Lutetian to Priabonian (46.2–36 Ma), supported by the co-occurrence of Enneadocysta dictyostila and D. antarctica, allowing the establishment of a maximum and minimum age for these deposits. The proposed age, which is in agreement with Douglas et al. (Reference Douglas, Affek, Ivany, Houben, Sijp, Sluijs, Schouten and Pagani2014), is based on the dinoflagellate cyst biostratigraphic scheme of the early Palaeogene of the Southern Ocean performed by Bijl et al. (Reference Bijl, Sluijs and Brinkhuis2013a), which at the moment is the most accurate scheme for the Eocene assemblages of southern high-latitudes sections. The LMF is correlatable with zones SPDZ 10, 11 and 12 of the Palaeogene biozonation of the Southern Ocean, but the correlation with Zone SPDZ 13 is not very clear. The LMF is also equivalent to zones RTF1 and RTF2 of the Austral Basin defined for the Río Turbio Formation (upper member), Austral Basin, Argentina, but the correlation between the LMF and Zone RTF 3 is not well established.
There is an apparent contradiction between dinoflagellate cysts data presented herein and the magnetostratigraphic age model performed for the LMF (Beamud et al. Reference Beamud, Montes, Santillana, Nozal and Marenssi2015; Montes et al. Reference Montes, Beamud, Nozal, Santillana, Crame, Acosta Hospitaleche and Gelfo2019a) that suggests a late Thanetian to early Lutetian (∼58.4–45.8 Ma) age. The consistency of the absolute age model offered by the magneto-, litho- and Sr-isotope data would imply the earliest appearance of the Enneadocysta dictyostila in the LMF (Antarctic Peninsula), compared with the later occurrence of the species in the rest of the Southern Ocean localities. If we consider that these differences exist, a convincing explanation should be found, or alternatively, a hiatus of 11 Ma between the LMF and the underlying Cross Valley – Wiman Formation should be assumed and a new geotectonic model developed for the James Ross Basin to elucidate its cause. The reasons for the discrepancies and the feasibility of the magnetostratigraphic age model performed for the LMF deserve deeper discussion in a future contribution.
The data on the LMF offered here are useful to elucidate and support the South Atlantic Ocean circulation proposals. The LMF has a dominance of Antarctic-endemic taxa during the middle to late Eocene, as occurred with the dinoflagellate cyst assemblages from other localities in the Southern Ocean south of 45° S. The distribution of the endemic taxa reflects an ocean-circulation scheme with wide clockwise gyres surrounding Antarctica that were disrupted as a consequence of the deepening of the Tasmanian Gateway and Drake Passage. The deepening of those gateways and their definitive and final apertures occurred towards the Eocene/Oligocene transition, changing the environmental conditions producing the extinction of the endemic taxa.
The palaeoenvironmental analyses of the LMF based on P/G and S/D ratios allowed the proposal of an overall trend through the section from marine-dominated assemblages with poor productive waters (Valle de las Focas to Acantilados II allomembers) to more terrestrially dominated assemblages, with an increase in productivity in the upper part of the unit (from Campamento to Cucullaea II allomembers) reflecting a shallowing trend to the top.
This study complements the contribution of Douglas et al. (Reference Douglas, Affek, Ivany, Houben, Sijp, Sluijs, Schouten and Pagani2014) since it confirms the age suggested by the authors and provides quantitative palynological data of the whole LMF that will enable more reliable comparisons between similar assemblages of localities around Drake Passage. The correct chronology will allow many important Palaeogene issues to be elucidated, such as biostratigraphy, phylogenies, palaeoclimatic features and Southern Ocean palaeocirculation, among others.
Author ORCIDs
Cecilia R. Amenábar 0000-0003-1280-3903
Acknowledgments
The authors are grateful to the Dirección Nacional del Antártico – Instituto Antártico Argentino (DNA-IAA) and Argentinian Air Force for scientific and logistic support during the Argentine Summer Antarctic Expedition 2015. We also thank M. Barbé for sample preparations. C.R.A. would like to express her deep gratitude to Dr Raquel Guerstein (INGEOSUR-CONICET, Argentina) for her advice and valuable assistance in the analysis of the palynological samples. Dr Eduardo Olivero (CADIC-CONICET, Argentina) is also acknowledged by C.R.A. for his constructive suggestions exchanged during different scientific meetings. We should like to thank the anonymous reviewers, as well as Dr Prof. Paul Upchurch, Editor of the journal, who put valuable time and effort into reviewing this contribution. The reviewers’ constructive comments greatly helped to improve the manuscript. This research was supported by the Agencia Nacional de Promoción Científica y Tecnológica – Dirección Nacional del Antártico from Argentina (Institutional Project no. 4: Geology and Topology) and the Instituto Geológico y Minero de España (IGME) and Dirección General de Investigación (DGI) of Spain (CTM 011-30241-C02-02, CTM2014-60451-C2-2-P). This is contribution number R-241 of the Instituto de Estudios Andinos ‘Don Pablo Groeber’ (IDEAN-CONICET).
Conflict of interest
None.
Supplementary material
To view supplementary material for this article, please visit https://doi.org/10.1017/S0016756819000591.
Appendix Dinoflagellate cysts cited in the text
Achomosphaera crassipellis (Deflandre & Cookson, 1955) Stover & Evitt, 1978
Arachnodinium antarcticum Wilson & Clowes, 1982
Brigantedinium ? spp.
Cerebrocysta waipawaensis (Wilson, 1988) Fensome et al., 2009
Deflandrea antarctica Wilson, 1967
Deflandrea cygniformis Pöthe de Baldis, 1966
Deflandrea oebisfeldensis Alberti, 1959
Deflandrea sp. cf. D. convexa Wilson, 1988
Diphyes colligerum (Deflandre & Cookson, 1955) Cookson, 1965
Enigmadinium cylindrifloriferum Wrenn & Hart, 1988
Enneadocysta brevistila Fensome et al., Reference Fensome, Guerstein and Williams2006
Enneadocysta dictyostila (Menéndez, Reference Menéndez1965) Fensome et al., Reference Fensome, Guerstein and Williams2006
Glaphyrocysta semitecta (Bujak in Bujak et al., 1980) Lentin & Williams, 1981
Hystrichokolpoma sp.
Hystrichosphaeridium salpingophorum (Deflandre, 1935) ex Deflandre, 1937 emend. Davey & Williams, 1966
Hystrichosphaeridium sp. cf. H. astartes Sannemann, 1955
Hystrichosphaeridium truswelliae Wrenn & Hart, Reference Wrenn, Hart, Feldman and Woodburne1988
Impagidinium parvireticulatum Wilson, 1988
Impagidinium sp.
Impletosphaeridium clavus (Wrenn & Hart, Reference Wrenn, Hart, Feldman and Woodburne1988) emend. Bowman et al., 2013
Impletosphaeridium ligospinosum Wrenn & Hart, Reference Wrenn, Hart, Feldman and Woodburne1988
Lejeunecysta spp.
Octodinium askiniae Wrenn & Hart, Reference Wrenn, Hart, Feldman and Woodburne1988
Oligosphaeridium pulcherrimum (Deflandre & Cookson, 1955) Davey & Williams, 1966
Operculodinium centrocarpum (Deflandre & Cookson) Wall, 1967
Palaeocystodinium australinum (Cookson, 1965) Lentin & Williams, 1976
Phelodinium magnificum (Stanley, 1965) Stover & Evitt, 1978
Selenopemphix crenata Matsuoka & Bujak, 1988
Selenopemphix nephroides Benedek, 1972
Senegalinium asymmetricum (Wilson, 1967) Stover & Evitt, 1978
Spinidinium macmurdoense (Wilson, 1967) Lentin & Williams, 1976
Spiniferites ramosus (Ehrenberg, 1838) Mantell, 1854
Thalassiphora pelagica (Eisenack, 1954) Eisenack & Gocht, 1960
Turbiosphaera filosa (Wilson, 1967) Archangelsky, 1969
Vozzhennikovia apertura (Wilson, 1967) Lentin & Williams, 1976