1. Introduction
Early Ordovician magmatism is widespread in many parts of the southern Variscides (e.g. von Raumer et al. Reference von Raumer, Stampfli, Borel and Bussy2002; Mattauer, Reference Mattauer2004 and reference therein). It is particularly well known in the northern Ollo de Sapo Domain of the Central Iberian Zone, where it has been extensively dated (e.g. Valverde-Vaquero & Dunning, Reference Valverde-Vaquero and Dunning2000; Montero et al. Reference Montero, Talavera, Bea, Lodeiro and Whitehouse2009; Díez Montes, Martínez Catalán & Mulas, Reference Díez Montes, Martínez Catalán and Mulas2010). Until recently, there was little evidence for magmatism of this age in the southern Schistose-Greywacke Domain of the Central Iberian Zone (Díez Balda, Vegas & González Lodeiro, Reference Díez Balda, Vegas, González Lodeiro, Dallmeyer and Martínez García1990). New U–Pb ages across the border in Portugal (Antunes et al. Reference Antunes, Neiva, Silva and Corfu2009; Neiva et al. Reference Neiva, Williams, Ramos, Gomes, Silva and Antunes2009) indicated that some tonalite–granodiorite-dominated igneous bodies are not Variscan syn-kinematic intrusions, but Early Ordovician in age. These bodies belong to a tonalite–granodiorite suite, along the Beira Baixa–Zarza la Mayor sector of the Schistose-Greywacke Domain, which had already been recognized by Portugal Ferreira (Reference Portugal Ferreira1982). The correlation of the Oledo pluton (Antunes et al. Reference Antunes, Neiva, Silva and Corfu2009) with the Zarza la Mayor tonalite in Spain prompted our interest in testing the Variscan age of the undated Zarza la Mayor tonalite.
Our study was initially focused on the Zarza la Mayor tonalite (Corretgé-Castañón, Reference Corretgé-Castañón1969; García de Figuerola, Corretgé & Suárez, Reference García de Figuerola, Corretgé and Suárez1971). This is a composite body located in the northwestern end of the Central Extremadura Batholith of Castro (Reference Castro1986). It belongs to the group of early syn-kinematic calc-alkaline granitoids with tonalitic affinities in the batholith (Castro, Reference Castro1986; Fernández & Castro, Reference Fernández and Castro1999). Combined U–Pb laser ablation inductively coupled plasma mass spectrometry (LA-ICP-MS) and chemical abrasion isotope dilution thermal ionization mass spectrometry (CA-ID-TIMS) dating revealed an Early Ordovician age and the absence of inherited zircons. In light of these results, we carried out U–Th–Pb electron microprobe analysis (EMPA) chemical dating of monazite from two separate plutons, Arroyo de la Luz granodiorite and Zarza de Montánchez tonalite, to test the extension of the Ordovician tonalitic–granodioritic suite. Our data show that these granodiorites/tonalites of the Central Extremadura Batholith (Castro, Reference Castro1986) are Early Ordovician bodies contemporaneous with the Ollo de Sapo magmatic event further north, instead of early syn-kinematic Variscan intrusions (Fernández & Castro, Reference Fernández and Castro1999). The broad distribution of this tonalite–granodiorite belt reveals that magmatism of Early Ordovician age was widespread throughout the Central Iberian Zone.
These epizonal plutons contain abundant mafic enclaves and gabbroic end-members, and they preserve their contact aureoles and many primary igneous features. The fact that these plutons have escaped major Variscan reworking makes them quite unique among the Early Ordovician intrusions in the southern Variscides, most of which are transformed into orthogneisses (see Mattauer, Reference Mattauer2004). These features make them key in testing the different tectonic models invoked to explain this magmatic event.
2. Geological setting
The Zarza la Mayor pluton is situated in the Schistose-Greywacke Domain of the Central Iberian Zone (Díez Balda, Vegas & González Lodeiro, Reference Díez Balda, Vegas, González Lodeiro, Dallmeyer and Martínez García1990) in the western end of the province of Cáceres, near the Portuguese border. It belongs to a group of partially deformed tonalite-dominated intrusions, which also include the Arroyo de la Luz, Zarza de Montánchez and Santa Cruz intrusions. These tonalites occur close to the large late-kinematic bodies of the Central Extremadura Batholith, such as the Cabeza de Araya granite (Fig. 1; Castro, Reference Castro1986). The tonalites have a classic major-element calc-alkaline geochemical signature (Corretgé, Bea & Suárez, Reference Corretgé, Bea and Suárez1985) and juvenile Sr and Nd isotopic signatures (Castro et al. Reference Castro, Patiño Douce, Corretgé, De La Rosa, El-Biad and El-Hmidi1999). They intruded the Upper Precambrian rocks of the Schistose-Greywacke Group producing hornfels contact metamorphism. In the case of the Zarza la Mayor tonalite the contact aureole is 0.5 to 1.5 km wide and reaches hornblende-hornfels conditions (> 2 kb; García de Figuerola, Corretgé & Suárez, Reference García de Figuerola, Corretgé and Suárez1971; Bascones, Martín Herrero & Corretgé, Reference Bascones, Martín Herrero and Corretgé1987). The Arroyo de la Luz and Zarza de Montánchez tonalites belong to the group of early-deformed intrusions of the Central Extremadura Batholith of Castro (Reference Castro1986); whereas the Zarza la Mayor tonalite, owing to its partial overprint, has been considered as an intermediate type between the early, deformed and the late, undeformed plutons.
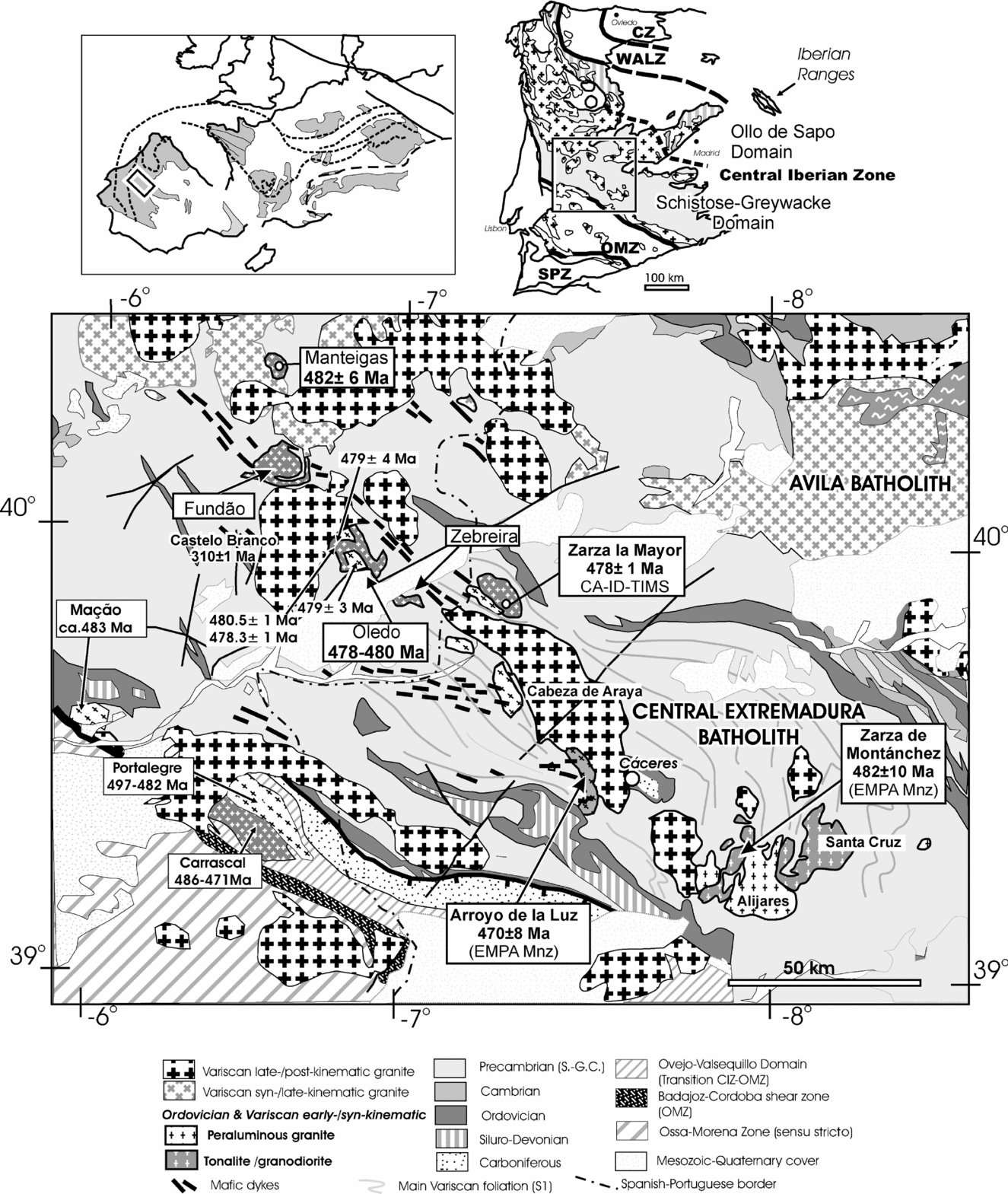
Figure 1. Simplified geological map of the Central Extremadura portion of the Schistose-Greywacke Domain of the Central Iberian Zone showing the location of the different plutons of the Beira Baixa–Central Extremadura tonalite belt (after IGME-LNEG 1:1000000 scale map, unpub. data). U–Pb ages, see references in text. Mafic dyke swarms in the Central Iberian Zone after Portugal Ferreira (Reference Portugal Ferreira1982) and García de Figuerola, Corretgé & Bea (Reference García de Figuerola, Corretgé and Bea1974). Variscan schistosity and elements of the Central Extremadura Batholith after Castro (Reference Castro1986). CZ – Cantabrian Zone, WALZ – West Asturian Leonese Zone, CIZ – Central Iberian Zone, OMZ – Ossa Morena Zone, SPZ – South Portuguese Zone, S-G.C. – Schistose-Greywacke Complex.
Across the border in Portugal, the Zarza la Mayor tonalite was correlated by Portugal Ferreira (Reference Portugal Ferreira1982) with the tonalites/granodiorites of Oledo, Zebreiro and Fundão, which are spatially associated with a lineament of mafic dyke swarms. These mafic dykes have a calc-alkaline petrographic character. Similar dykes are also found associated with the Zarza la Mayor intrusion (García de Figuerola, Corretgé & Suárez, Reference García de Figuerola, Corretgé and Suárez1971; García de Figuerola, Corretgé & Bea, Reference García de Figuerola, Corretgé and Bea1974) and the Arroyo de la Luz granodiorite (Bascones, Martín Herrero & Corretgé, Reference Bascones, Martín Herrero and Corretgé1987).
2.a. Field relationships and petrography
The Zarza la Mayor pluton consists of three main facies: biotite tonalite, coarse-grained muscovite ± biotite granite and aplitic leucogranite (Corretgé, Reference Corretgé1971). The muscovite ± biotite granite is an elongated body, approximately 3 to 4 km wide and 10 km long, located along the southern border of the tonalite. It belongs to group B of the alkali-feldspar granites of Castro (Reference Castro1986). It is locally overprinted by a mylonitic foliation producing gneissic and cataclastic textures. The aplitic leucogranite intrudes the tonalite forming a ring-dyke complex with dykes feeding sills on the cupola of the stock (Rubio-Ordóñez, Corretgé & Cuesta, Reference Rubio-Ordóñez, Corretgé and Cuesta2007). Biotite-rich tonalite, quartz diorite to granodiorite constitutes the largest unit of the pluton. It belongs to group A of the quartz dioritic granitoids of Castro (Reference Castro1986). The Zarza la Mayor tonalite has a medium-grained hypidiomorphic texture with quartz, plagioclase and biotite as the main minerals and K-feldspar, apatite, oxides, zircon, titanite, anatase and rutile as minor and accessory minerals. The plagioclase crystals are anhedral to subhedral and locally partially oriented defining magmatic flow structures. They show a strong zonation with cores with An50–60 and rims with An6–10, which is evidence of strong changes in magma composition. Despite the lack of penetrative foliation in most of the intrusion, the quartz shows evidence of tectonic overprint such as undulose extinction, formation of subgrains and recrystallization. Other local facies of the intrusion are granodiorite with K-feldspar forming an adcumulate texture, K-feldspar-rich syenite formed by the hydrothermal alteration of monzonite–monzodiorite, minor amphibole-bearing dioritic porphyry and hornblendite (García de Figuerola, Corretgé & Suárez, Reference García de Figuerola, Corretgé and Suárez1971; Rubio, Reference Rubio1982; Bascones, Martín Herrero & Corretgé, Reference Bascones, Martín Herrero and Corretgé1987). In addition, the tonalite contains mafic dykes, as well as microgranular enclaves and country rock xenoliths (Fig. 2).

Figure 2. Zarza la Mayor tonalite: (a) enclaves of metasediments from the country rock Schistose-Greywacke complex and blobs of tonalite; (b) undeformed mafic microgranular enclave.
The Zarza de Montánchez intrusion is very similar to the Zarza la Mayor tonalite. It is dominated by quartz diorite and biotite granodiorite with subordinate muscovite leucogranite and aplitic granite (Gil Serrano, Pérez Rojas & Pineda Velasco, Reference Gil Serrano, Pérez Rojas and Pineda Velasco1982). The Arroyo de la Luz intrusion is slightly more felsic with prevailing biotite granite to granodiorite (Bascones, Martín Herrero & Corretgé, Reference Bascones, Martín Herrero and Corretgé1987). These two bodies also contain abundant enclaves of country rock xenoliths and mafic enclaves.
2.b. Previous geochronology
Prior to the current work, geochronological data for the tonalites of the Central Extremadura Batholith consisted of Rb–Sr whole-rock isochron ages for rocks from several plutons that gave an age of 326 Ma (Castro et al. Reference Castro, Patiño Douce, Corretgé, De La Rosa, El-Biad and El-Hmidi1999). In the rest of the Central Extremadura Batholith, geochronological data are scarce. The neighbouring Cabeza de Araya granite has a reported Rb–Sr age of 303 ± 7 Ma (Bea, Montero & Zinger, Reference Bea, Montero and Zinger2003) coincident with a monazite U–Th–Pb EMP chemical age of 302 ± 7 Ma (Carracedo et al. Reference Carracedo, Gil Ibarguchi, García de Madinabeitia and Berrocal2005). Across the border in Portugal, the Oledo intrusion is very similar to Zarza la Mayor. It also includes tonalite/granodiorite and a muscovite ± biotite granite. It has been recently dated by U–Pb ID-TIMS with ages of 480.5 ± 1 Ma (zircon) and 478.3 ± 1.1 Ma (monazite) for the biotite granodiorite; 479 ± 4 Ma for the biotite + muscovite granodiorite; and the 479 ± 4 Ma for the muscovite + biotite granite (Antunes et al. Reference Antunes, Neiva, Silva and Corfu2009). The Oledo pluton is intruded by the 310 ± 1 Ma Castelo Branco granite (U–Pb ID-TIMS; Antunes et al. Reference Antunes, Neiva, Silva and Corfu2008), which is a late-kinematic Variscan intrusion similar to Cabeza de Araya.
3. Geochronology
Our initial purpose was to obtain the intrusion age and the age of the inherited zircon of the Zarza la Mayor tonalite. For this reason, we combined the advantage of the spatial resolution of the LA-ICP-MS technique with the high precision of the U–Pb ID-TIMS. In light of the U–Pb results, monazite EMPA dating using polished petrographic thin-sections was used, as a reconnaissance tool, to check if the Zarza de Montanchez and the Arroyo de la Luz intrusions belonged to the same age group. The U–Pb LA-ICP-MS dating was carried out at the University of Frankfurt am Main (Germany), the U–Pb ID-TIMS at IGME laboratories (Tres Cantos, Spain) and the monazite EMPA dating at the University of Oviedo (Spain). Details of the analytical techniques are given in the Appendix.
3.a. U–Pb LA-ICP-MS and ID-TIMS: Zarza la Mayor tonalite
The zircons from the tonalite and the leucogranitic facies of the Zarza la Mayor intrusion were studied following the method of Pupin & Turco (Reference Pupin and Turco1972). Zircon is more abundant in the tonalitic facies, but both rocks have zircon with similar typologies, dominated by {110} prismatic faces and {211} pyramidal forms (Rubio-Ordóñez, Corretgé & Cuesta, Reference Rubio-Ordóñez, Corretgé and Cuesta2007). The zircons from the leucogranite fall into the S6 and Q2 morphologies, while those from the tonalite are mainly S1–L1 and Q1 types (Fig. 3). In general, the length/width ratio ranges from 3:1 to 5:1. The back-scattered electron (BSE) images show crystals either with complex cores and resorption features, indicating separate stages of zircon growth and corrosion, or well-defined concentric growth zoning (Fig. 3).

Figure 3. Back-scattered electron images of the zircons dated by LA-ICP-MS. Top, zircons with complex cores and resorption features; bottom, zircons with a simple concentric zoning.
3.a.1. U–Pb LA-ICP-MS: Zarza la Mayor tonalite
Nineteen crystals were dated by U–Pb LA-ICP-MS, including zircons with complex cores as well as zircons with concentric zoning. Four points were rejected, the remaining 15 analyses providing 206Pb–238U ages between 475 and 512 (± 10–20) Ma (Table 1) and a ‘concordia age’ of 495.8 ± 5.6 Ma (MSWD of 2.4), which is in agreement with the weighted average age of 497 ± 6 Ma (MSWD of 1.8) derived from the 206Pb–238U ages (Fig. 4).
Table 1. U–Pb LA-ICP-MS data, Zarza la Mayor tonalite

Isotopic ratios corrected for background signal, common Pb, laser induced elemental fractionation, instrumental mass discrimination and time-dependant elemental fractionation of Pb/Th and Pb/U. Sample, zircon morphology: cz. – concentric zone; rz. – resorbed zone; c. – core; r. – rim.

Figure 4. U–Pb LA-ICP-MS data, U–Pb concordia diagram of the LA-ICP-MS (large open ellipses) and the ID-TIMS data (small white filled ellipses – zircon; grey ellipses – monazite).
3.a.2. U–Pb ID-TIMS: Zarza la Mayor tonalite
Six fractions were analysed by U–Pb ID-TIMS, two of monazite and four of zircon (Table 2). Each zircon fraction comprised 8 to 15 euhedral zircon prisms with a length/width ratio of 3:1 to 5:1, such as those in Figure 3. Two fractions, a monazite and a zircon, are discordant. The six fractions define a discordia line (MSWD 3.6) with an upper intercept of 476 ± 10 Ma and a lower intercept at 7 ± 380 Ma (Fig. 4). The upper intercept is best constrained by three concordant zircon fractions and a monazite fraction with a slight reverse discordia, but with an identical 207Pb–235U age (Fig. 5). The three concordant zircon fractions cluster at 478 Ma and provide a ‘concordia age’ of 478.13 ± 0.82 Ma (MSWD 0.25). We interpret this zircon crystallization age as the age of intrusion.
Table 2. U–Pb ID-TIMS geochronological data, Zarza la Mayor tonalite

Z – small (< 100 μm) euhedral zircon prisms 1:3 to 1:5 width/length ratio. All chemically abraded (CA; Mattison, Reference Mattinson2005). Weight estimated before CA. M – clear monazite, 60 to 80 μm. Number of grains in each fraction is given within brackets. Pb (pg) – total common Pb blank. * Measured ratio corrected for blank and fractionation. Atomic ratios corrected for fractionation (0.11 ± 0.02% AMU Pb; 0.10 ± 0.02% AMU, U), spike (208Pb–235U), laboratory blanks (6 pg Pb; 0.1 pg U) and initial common Pb after Stacey & Kramers (Reference Stacey and Kramers1975). Errors are at the 2-sigma level. Data reduced with PbMacDat (Isachsen, Coleman & Schmitz, Reference Isachsen, Coleman and Schmitz2007; www.earth-time.org).

Figure 5. U–Pb concordia diagram with the concordant ID-TIMS data; crosses – centroids of the error ellipses. For comparison, grey ellipses are data from the Oledo granodiorite (Portugal; sample H1 of Antunes et al. Reference Antunes, Neiva, Silva and Corfu2009); M – monazite, Z – zircon.
3.b. Monazite EMPA dating: Arroyo de la Luz and Zarza de Montánchez tonalites
Monazites from the Arroyo de la Luz granodiorite (sample 14445) and the Zarza de Montánchez granodiorite (sample 14449) were dated using the U–Th–Pb EMPA chemical dating method (Table 3). In the case of the Arroyo de la Luz sample, a total of 29 points on 12 monazite crystals were made. These monazites are Ce-rich, with a small xenotime component (XYPO4 0.1–0.7), plotting close to the brabantite compositional vector (Fig. 6). They show a high concentration of light rare earth elements (LREEs), common in this kind of monazite (Förster, Reference Förster1998). The individual ages range from 441 to 504 (± 78–146) Ma, show a normal distribution, with a weighted average of 470 ± 15 Ma (MSWD 0.34) and a Th/Pb–U/Pb age of 470 ± 8 Ma (Fig. 7).
Table 3. EMPA analyses of monazite

Calculated ages based on the method of Cocherie & Albarede (Reference Cocherie and Albarede2001). Errors are at the 2-sigma level.

Figure 6. Monazite composition, Arroyo de la Luz and Zarza de Montánchez tonalites. Plot of the formula proportions (Th+U+Si) v. (REE+Y+P) calculated on the basis of 16 oxygen atoms. The arrows represent the brabantite (ThCaUPb(PO4)2) and huttonite (ThSiO4) substitution vectors.

Figure 7. U–Th–Pb monazite EMPA chemical ages, Th/Pb–U/Pb diagrams: (a) Arroyo de la Luz; (b) Zarza de Montánchez.
In the Zarza de Montánchez granodiorite, 21 points were analysed on 11 monazites and give ages from 439 to 549 (± 50–250) Ma. These monazites are Ce-rich, as well, with a low Y content (XYPO4 0.1–0.9). They plot on the brabantite vector, with a larger spread of compositions than those from Arroyo de la Luz (Fig. 6). The age population displays a normal distribution with a calculated weighted average age of 482 ± 10 Ma (MSWD 0.38) and a Th/Pb–U/Pb age of 482 ± 10 Ma (Fig. 7).
4. Discussion
The age of 478 ± 1 Ma of the Zarza la Mayor granodiorite matches within error the ages of the Oledo pluton (Antunes et al. Reference Antunes, Neiva, Silva and Corfu2009) and the Manteigas granodiorite (Neiva et al. Reference Neiva, Williams, Ramos, Gomes, Silva and Antunes2009) located along strike in Portugal, and the Mação-Penhascoso granite (c. 483 Ma, U–Pb zircon ID-TIMS; Romão et al. Reference Romão, Dunning, Marcos, Dias and Ribeiro2010) near the boundary with the Ossa-Morena Zone (Fig. 1). Sample H-1 of Antunes et al. (Reference Antunes, Neiva, Silva and Corfu2009) is plotted in the concordia diagram (Fig. 5) to show the high degree of overlap of the zircon and monazite TIMS data, including the slightly reverse discordant monazite. North of the Oledo pluton, Neiva et al. (Reference Neiva, Williams, Ramos, Gomes, Silva and Antunes2009) dated the Manteigas granodiorite with a U–Pb sensitive high-resolution ion microprobe (SHRIMP) zircon age of 481.8 ± 5.9 Ma. Like the Zarza la Mayor granodiorite, this biotite granodiorite also contains zircons, without inheritance, with complex cores and concentric zoning of Early Ordovician age. The EMPA U–Th–Pb monazite chemical ages of 470–482 Ma for the Arroyo de la Luz and the Zarza de Montánchez granodiorites overlap within error with the U–Pb ages, confirming that these bodies belong to the same tonalite–granodiorite suite.
4.a. The Beira Baixa–Central Extremadura tonalite–granodiorite belt
This suite has a calc-alkaline affinity similar to the I-type granites of Chappell & White (Reference Chappell and White1992), with juvenile Sr and Nd values (Castro et al. Reference Castro, Patiño Douce, Corretgé, De La Rosa, El-Biad and El-Hmidi1999; Antunes et al. Reference Antunes, Neiva, Silva and Corfu2009; Neiva et al. Reference Neiva, Williams, Ramos, Gomes, Silva and Antunes2009). Portugal Ferreira (Reference Portugal Ferreira1982) recognized the unique character of this calc-alkaline suite, which he considered Variscan early/syn-kinematic (inter D1–D2). He grouped the tonalite–granodiorite bodies and the associated mafic dykes in what he defined as the Beira Baixa–Zarza la Mayor lineament. Since the tonalite–granodiorite belt extends for 250 km from the Beira Baixa region of Portugal to Central Extremadura (Spain), we propose to name it the Beira Baixa–Central Extremadura tonalite–granodiorite belt. This belt might also include other undated tonalites/granodiorites such as those of Fundão and Zebreira (Fig. 1; see Portugal Ferreira, Reference Portugal Ferreira1982) or the Alijares and Santa Cruz plutons, which belong to the early-deformed granitoids of the Central Extremadura Batholith (Fig. 1; Castro, Reference Castro1986).
4.b. Distribution of the Early Ordovician magmatism in the Central Iberian Zone
The age of intrusion of the Beira Baixa–Central Extremadura tonalite belt broadly coincides with the ages of the nearby calc-alkaline Carrascal granodiorite and the Portalegre granite, and the felsic volcanic rocks of the Urra Formation in the transition zone between the Central Iberian and Ossa-Morena zones, the Obejo-Valsequillo Domain (Fig. 1; Lancelot & Allegret, Reference Lancelot and Allegret1982; Cordani et al. Reference Cordani, Nutman, Andrade, Santos, Azevedo, Mendes and Pinto2006; Solá et al. Reference Solá, Pereira, Williams, Ribeiro, Neiva, Montero, Bea and Zinger2008). The presence of dioritic–gabbroic members in the Carrascal granodiorite with juvenile Sr and Nd numbers similar to those of the Oledo granodiorite is particularly remarkable (Solá et al. Reference Solá, Pereira, Williams, Ribeiro, Neiva, Montero, Bea and Zinger2008; Antunes et al. Reference Antunes, Neiva, Silva and Corfu2009). This would suggest that this part of the Obejo-Valsequillo Domain was already in close proximity, if not already attached, to the Schistose-Greywacke Domain of the Central Iberian Zone.
The tonalitic–granodioritic event in the Schistose-Greywacke Domain is also contemporaneous with the Early Ordovician magmatic event along the northern Ollo de Sapo Domain of the Central Iberian Zone (U–Pb ages; Lancelot, Allegret & de Leon, Reference Lancelot, Allegret and de Leon1985; Gebauer, Martínez García & Hepburn, Reference Gebauer, Martínez García and Hepburn1993; Fernández-Suárez et al. Reference Fernández-Suárez, Gutiérrez-Alonso, Jenner and Tubrett1999; Valverde-Vaquero & Dunning, Reference Valverde-Vaquero and Dunning2000; Bea et al. Reference Bea, Montero, Talavera and Zinger2006, Reference Bea, Montero, Gonzalez-Lodeiro and Talavera2007; Montero et al. Reference Montero, Bea, González-Lodeiro, Talavera and Whitehouse2007, Reference Montero, Talavera, Bea, Lodeiro and Whitehouse2009; Díez Montes, Martínez Catalán & Mulas, Reference Díez Montes, Martínez Catalán and Mulas2010). The broad extension of the tonalite–granodiorite belt (Fig. 1) reveals that magmatism of Early Ordovician age was widespread throughout the Central Iberian Zone. The Central Iberian Zone has strong Palaeozoic lithostratigraphic and palaeontological affinities with the West Asturian Leonese and the Cantabrian Zone in the north of the Iberian Massif (e.g. Julivert & Martinez, Reference Julivert, Mártinez, Schaer and Rodgers1987), indicating that these three zones formed a single terrane since Early Cambrian time: the Iberian Autochthonous terrane (Quesada, Reference Quesada1991). The Early Ordovician magmatism in this terrane appears to form two major parallel belts running along the Central Iberian Zone: the Beira Baixa–Central Extremadura and the Ollo de Sapo magmatic belts (Fig. 8). The deep Cambro-Ordovician sedimentary trough of the West Asturian Leonese Zone (e.g. Aramburu et al. Reference Aramburu, Truyols, Arbizu, Méndez-Bedia, Zamarreño, García-Ramos, Suárez de Centi, Valenzuela, Gutiérrez-Marco, Saavedra and Rábano1992) separates the Central Iberian Zone from the Cantabrian Zone, where coeval Early Ordovician magmatism is also present as local discrete felsic ash beds (477.47 ± 0.93 Ma U–Pb zircon; Gutiérrez-Alonso et al. Reference Gutiérrez-Alonso, Fernández-Suárez, Gutiérrez-Marco, Corfu, Murphy and Suárez2007) and within-plate alkaline basalts (Gallastegui et al. Reference Gallastegui, Aramburu, Barba, Fernández, Cuesta, Rábano, Gutiérrez-Marco and Saavedra1992). Such magmatism is also found east of the Central Iberian Zone in the Iberian Chains, where two separate Tremadocian–Arenig rhyolitic and dacitic volcanic events are followed by an Arenig within-plate alkaline basaltic flow (Álvaro et al. Reference Álvaro, Ezzouhairi, Ribeiro, Ramos and Solá2008).

Figure 8. Tectonic model and distribution of the Early Ordovician magmatism in the Central Iberian Zone and neighbouring areas of the Iberian Autochthonous Terrane.
4.c. Tectonic interpretation
Most characteristic of the Early Ordovician magmatism in the Central Iberian Zone is the Ollo de Sapo volcanism and the associated granite intrusions. These felsic volcanic rocks are ignimbrites with coarse feldspar crystals and volcanic quartz (see Schäfer, Reference Schäfer1969; Díez-Montes, Martinez Catalán & Mulas, Reference Díez Montes, Martínez Catalán and Mulas2010). Fernandez et al. (Reference Fernández, Becchio, Castro, Viramonte, Moreno-Ventas and Corretgé2008) have noted the striking geochemical and petrographic analogies between the Ollo de Sapo Formation and the ferrosilicic Cambro-Ordovician felsic volcanics of the eastern Puna eruptive belt in the Famatinian orogen of northern Argentina, and proposed the formation of the Ollo de Sapo volcanism in a continental back-arc environment. The outward position of the Beira Baixa–Central Extremadura calc-alkaline belt of I-type granitoids with respect to the Ollo de Sapo magmatic belt further strengthens the analogies with the Famatinian orogen, as it resembles the position of the calc-alkaline western Puna magmatic belt (Pankhurst et al. Reference Pankhurst, Rapela, Saavedra, Baldo, Dahlquist, Pascua, Fanning, Pankhurst and Rapela1998; Pankhurst, Rapela & Fanning, Reference Pankhurst, Rapela and Fanning2000). This belt, formed by I-type granite, a calc-alkaline trondhjemite–tonalite–granodiorite (TTG) suite and S-type peraluminous granite, represents the continental magmatic arc bounding the eastern Puna back-arc. Owing to their geochemistry and the local association with Cu-porphyry mineralization, Portugal Ferreira (Reference Portugal Ferreira1982) interpreted the calc-alkaline tonalites and granodiorites of the Beira Baixa–Central Extremadura belt as a magmatic arc. This might suggest that the Beira Baixa–Central Extremadura belt represents an outward continental magmatic arc bounding the Ollo de Sapo continental back-arc. The presence of within-plate alkaline basalts in the Cantabrian Zone and the Iberian Chains indicate the injection of plume-like mantle-derived melts in a more internal position of the Iberian Autochthonous terrane, during or shortly after this felsic magmatic event (Fig. 8). Therefore, the proposed tectonic scenario needs to be taken with caution, since continental rifting has also been considered as a possible explanation of the Ollo de Sapo magmatic event (e.g. Fernández-Suárez et al. Reference Fernández-Suárez, Gutiérrez-Alonso, Jenner and Turbett2000; Bea et al. Reference Bea, Montero, Gonzalez-Lodeiro and Talavera2007).
So far the only detailed petrogenetic study in this tonalite–granodiorite belt is that of Antunes et al. (Reference Antunes, Neiva, Silva and Corfu2009), which has demonstrated the coexistence of coeval calc-alkaline, I-type hybrid melts and S-type peraluminous melts. We would like to point out that the mafic igneous bodies in the Early Ordovician Beira Baixa–Central Extremadura tonalite–granodiorite belt still preserve their primary mineralogy and igneous features, making them a prime target for future geochemical studies. Such studies will be key to understanding the nature of the mantle component, and thus crucial for testing the different tectonic models proposed to explain Early Ordovician magmatism in the Central Iberian Zone (e.g. Valverde-Vaquero & Dunning, Reference Valverde-Vaquero and Dunning2000; Bea et al. Reference Bea, Montero, Gonzalez-Lodeiro and Talavera2007; Fernández et al. Reference Fernández, Becchio, Castro, Viramonte, Moreno-Ventas and Corretgé2008).
5. Conclusions
The U–Pb CA-ID-TIMS dating of the Zarza la Mayor tonalite provides an intrusion age of 478 ± 1 Ma, coeval within error with the 480–478 Ma Oledo granodiorite across the border in Portugal (Antunes et al. Reference Antunes, Neiva, Silva and Corfu2009). The LA-ICP-MS dating of individual crystals indicate that these zircons, including some with complex internal features, do not contain Precambrian inherited cores. This feature is also observed in the zircons from the SHRIMP-dated 482 ± 6 Ma Manteigas granodiorite in Portugal (Neiva et al. Reference Neiva, Williams, Ramos, Gomes, Silva and Antunes2009).
The monazite U–Th–Pb EMPA chemical ages from the Arroyo de la Luz granodiorite (470 ± 15 Ma) and the Zarza de Montánchez tonalite (482 ± 10 Ma) coincide with the U–Pb age of Zarza la Mayor, and demonstrate that a significant number of the deformed tonalites of the Central Extremadura Batholith are Early Ordovician in age.
The Zarza la Mayor and Zarza de Montánchez tonalites, and the Arroyo de la Luz granodiorite with the Oledo and Manteigas granodiorites in Portugal, define the Early Ordovician Beira Baixa–Central Extremadura tonalite–granodiorite belt. This belt extends for 250 km along the Schistose-Greywacke Domain of the Central Iberian Zone, and it is contemporaneous with the Ollo de Sapo magmatic event in the Central Iberian Zone.
The Beira Baixa–Central Extremadura tonalite–granodiorite belt is tentatively interpreted as a continental volcanic arc. This interpretation has to be taken with caution, and needs to be tested with future petrogenetic studies of the mafic end-members of the suite.
Acknowledgements
We thank Fernando Corfu and Jane Scarrow for helpful and constructive reviews. This research was done within the frame of projects CGL2004-05681/BTE and CGL2008-05952-CO2-O2 to P.V.V., and CGL2004-06808-C04-03 to L.G.C. from the Spanish Ministry of Science and Innovation (MICINN).
Appendix. Analytical methods
Rock pulverization and mineral separation using a Wilfley table, heavy liquids and a Frantz isodynamic separator were done at the University of Oviedo (Spain). The selected zircon and monazite fractions for U–Pb LA-ICP-MS and ID-TIMS work were hand-picked under a microscope at IGME.
U–Pb LA-ICP-MS method
The zircon mount for LA-ICP-MS was made using double-sided tape, a plexiglass ring and Buehler Epoxicure resin. The grain mount was polished and back-scattered electron images were taken with the Cameca SX100 electron microprobe of the University of Oviedo of each zircon crystal to assess the internal morphology before carrying out the laser work.
Zircon was analysed for U, Th and Pb isotopes by LA-ICP-MS techniques at the Institute of Geosciences, Johann Wolfgang Goethe-University Frankfurt, using a Thermo-Finnigan Element II sector field ICP-MS coupled to a New Wave UP213 ultraviolet laser system. Laser spot-sizes varied from 20 to 40 μm for zircon and were placed based on the scanning electron microscope and cathodoluminescence (CL) images of the individual grains. The typical depth of the ablation crater was ~ 20 μm. Data were acquired in peak-jumping mode over 900 mass scans during 20 second background measurement followed by 32 second sample ablation. A teardrop-shaped, low volume laser cell was used to enable the precise detection of heterogeneous material (e.g. inclusion or different growth zones) during time-resolved data acquisition (see Janousek et al. Reference Janousek, Gerdes, Vrana, Finger, Erban, Friedl and Braithwaite2006). The signal was tuned for maximum sensitivity for Pb and U while keeping oxide production well below 1%. A common-Pb correction based on the interference- and background-corrected 204Pb signal and a model Pb composition (Stacey & Kramers, Reference Stacey and Kramers1975) was carried out if necessary. The necessity of the correction is judged on whether the corrected 207Pb/206Pb lies outside of the internal errors of the measured ratios. Raw data were corrected for background signal, common Pb, laser-induced elemental fractionation, instrumental mass discrimination and time-dependant elemental fractionation of Pb/Th and Pb/U using an Excel® spreadsheet program. Laser-induced elemental fractionation and instrumental mass discrimination were corrected by normalization to the reference zircon GJ-1 (Jackson et al. Reference Jackson, Pearson, Griffin and Belousova2004), which was analysed during the analytical session under exactly the same conditions as the samples. Prior to this normalization, the change of elemental fractionation (e.g. the Pb/Th and Pb/U ratios as a function of ablation time and thus crater depth) was corrected for each set of isotope ratios (c. 40) collected during the time of each single spot analysis. The correction was done by applying a linear regression through all measured ratios, excluding some outliers (> ±2 s.e.), and using the intercept with the y-axis as the initial ratio. Datasets obtained under optimal analytical conditions required that less than 10% of the raw data were treated as outliers. Exceptions arise when the laser penetrates domains with distinct Pb/U ratios (Janousek et al. Reference Janousek, Gerdes, Vrana, Finger, Erban, Friedl and Braithwaite2006), the epoxy resin, mineral inclusions in zircons and/or zircon zones affected by Pb-loss either due to metamictization or along cracks. All these effects, however, can be detected by careful monitoring of the time-resolved signal. Commonly they are obvious from abrupt changes in the signal strength of the measured isotopes and/or the isotope ratios. Nevertheless, small age differences (< 3 s.e. of the individual analysis) between distinct zircon growth domains or different grains cannot be resolved. The total offset of the measured drift-corrected 206Pb/238U ratio from the ‘true’ ID-TIMS value of the analysed GJ-1 grain was about 3–4%. Reported uncertainties (2σ) were propagated by quadratic addition of the external reproducibility (2 s.d.) obtained from the standard zircon GJ-1 (n = 20; 1.3% and 1.2% for the 207Pb/206Pb and 206Pb/238U, respectively) during the analytical session and the within-run precision of each analysis (2 s.e.). Concordia diagrams (2σ error ellipses) and concordia ages with 2 sigma uncertainty were produced using Isoplot/Ex 2.49 (Ludwig, Reference Ludwig2001). For further details on analytical protocol and data processing for the U–Th–Pb method see Gerdes & Zeh (Reference Gerdes and Zeh2006).
U–Pb ID-TIMS method
The zircon fractions processed for ID-TIMS at IGME were pre-treated with the chemical abrasion method (Mattinson, Reference Mattinson2005). Zircon annealing was carried out at 900°C for 48 hours and the chemical attack was done in Parrish-type minibombs inside Parr bombs at 180°C for 12 hours. Final zircon dissolution was achieved after placing the bomb at 240°C for 72 hours (Parrish, Reference Parrish1987). The procedure for extraction and purification of Pb and U is a scaled-down version of that of Krogh (1973). A 208Pb–235U spike was used to obtain the U/Pb ratios by isotope dilution (ID); this is the spike used in Valverde-Vaquero et al. (Reference Valverde-Vaquero, Dörr, Belka, Franke, Wiszniewska and Schastok2000). For a control, the 91500 (Wiedenbeck et al. Reference Wiedenbeck, Allé, Corfu, Griffin, Meier, Oberli, Quadt, Roddick and Spiegel1995) and R33 reference zircons (Black et al. Reference Black, Kamo, Allen, Davis, Aleinikoff, Valley, Mundil, Campbell, Korsch, Williams and Foudoulis2004) were dated and provided ‘concordia’ ages of 1065 ± 2.3 Ma and 419.7 ± 1.9 Ma. The spike was also checked against the 500 Ma artificial solution of the Earthtime Network (www.earth-time.org), and recalibrated using the Earthtime gravimetric solutions. The purified Pb and U of the ID fraction were collected together. The Pb and U from the IC and ID fractions were loaded in outgassed single renium filaments with a mixture of SiGel and H3PO4 (Gerstenberger & Haase, Reference Gerstenberger and Haase1997). Total procedural blanks for zircon are below 5 pg Pb and 0.2 pg U. Isotopic ratios were measured with a Triton TIMS multi-collector mass spectrometer equipped with a MassCon axial secondary electron multiplier (SEM) ion counter. The instrument is set up to do measurements both in static and peak-jumping mode using the SEM. For static measurements, the 204Pb was measured with the calibrated SEM (92–93% yield calibration). The Pb measurements were done in the 1300–1460°C range, and U was measured at 1420–1500°C. For further details on mass spectrometry see Valverde-Vaquero (Reference Valverde-Vaquero2009). The linearity of the SEM and deadtime correction were checked using the U500 and NBS982 standards, with a similar method to that in Arden & Gale (Reference Arden and Gale1974) and Richter et al. (Reference Richter, Goldberg, Mason, Traina and Schwieters2001). These standards were also used to estimate an instrumental fractionation of 0.10 ± 0.02% AMU for U and 0.11 ± 0.02% AMU for Pb. Data reduction was done using the PbMacDat spreadsheet (Isachsen, Coleman & Schmitz, Reference Isachsen, Coleman and Schmitz2007) and checked with PBDAT (Ludwig, Reference Ludwig1991). All isotopic ratios are corrected for mass fractionation, blank and initial common Pb, the latter after the model of Stacey & Kramers (Reference Stacey and Kramers1975). Ages and uncertainties were calculated with the decay constants of Jaffey et al. (Reference Jaffey, Flynn, Glendenin, Bentley and Essling1971), and are reported at the 2σ level; ‘concordia ages’ were calculated with Isoplot (see Ludwig, Reference Ludwig1999).
Monazite U–Th–Pb EMPA chemical dating method
The EMPA chemical dating of monazite (Montel et al. Reference Montel, Foret, Veschambre, Nicollet and Provost1996; Suzuki & Adachi, Reference Suzuki and Adachi1991) was done with the Cameca SX100 electron microprobe at the University of Oviedo (Spain) using polished grain mounts. The samples were coated with graphite. The analyses followed the protocols of Scherrer et al. (Reference Scherrer, Engi, Gnos, Jakob and Liechti2000) for U, Th, Pb and rare earth elements (REEs), in addition to Si, P, Y, Al and Ca. The chemical age calculations were done with the program provided with the Cameca SX100 geochronology option, which is based on Williams, Jercinovic & Terry (Reference Williams, Jercinovic and Terry1999) and Montel et al. (Reference Montel, Foret, Veschambre, Nicollet and Provost1996), and with the procedure of Cocherie & Albarede (Reference Cocherie and Albarede2001). The error on each age determination is reported at the 2-sigma level. To assure quality control we have used our own monazite standard with a U–Pb ID-TIMS age of 1083 ± 1 Ma (Valverde-Vaquero et al. Reference Valverde-Vaquero, Carrión, Reyes Andrés, Martín Rubí and Paradas Herrero2005) and we have dated the Jefferson Mountain (370 ± 15 Ma EMP age) and the Iveland (951 ± 33 Ma EMP age) monazite standards, for further details see Fernández González et al. (Reference Fernández González, Cuesta Fernández, Rubio-Ordóñez and Valverde-Vaquero2009). Cumulative plots and weighted average ages were done with Isoplot 3.0 (Ludwig, Reference Ludwig1999).