Introduction
This article is the second in a series of papers on the mineralogical description and crystal chemistry of minerals containing rare earth elements (REE) as species-defining cations (henceforth – ‘REE minerals’) from polymineralic nodules found at the Mochalin Log deposit, Chelyabinsk Oblast, South Urals, Russia (55°48′42″N, 60°33′46″E). A brief outline of the history of studies, the general data on geology and mineralogy of this deposit, as well as the description of two new isostructural gatelite-group minerals, ferriperbøeite-(La) and perbøeite-(La) are given in the first paper of this series (Kasatkin et al., Reference Kasatkin, Zubkova, Pekov, Chukanov, Škoda, Polekhovsky, Agakhanov, Belakovskiy, Kuznetsov, Britvin and Yu2020b) and references therein.
In the present article we describe two new isostructural minerals, radekškodaite-(La) [pronounced: ra dek shko da ait; Russian Cyrillic: радекшкодаит-(La)], ideally (CaLa5)(Al4Fe2+)[Si2O7][SiO4]5O(OH)3, and radekškodaite-(Ce) [Russian Cyrillic: радекшкодаит-(Ce)], ideally (CaCe5)(Al4Fe2+)[Si2O7][SiO4]5O(OH)3. Radekškodaite-(La), the member of this pair discovered first, was named in honour of Dr. Radek Škoda (born 1979), Associate Professor at the Department of Geological Sciences, Faculty of Science, Masaryk University, Brno, Czech Republic. Dr. Škoda is known for his contribution to the mineralogy and geochemistry of REE – see, e.g. Škoda and Novák (Reference Škoda and Novák2007), Škoda et al. (Reference Škoda, Novák and Cícha2011, Reference Škoda, Cempírek, Filip, Novák, Veselovský and Čtvrtlík2012, Reference Škoda, Plášil, Jonsson, Čopjaková, Langhof and Galiová Vašinová2015, Reference Škoda, Plášil, Čopjaková, Novák, Jonsson, Galiová Vašinová and Holtstam2018), Breiter et al. (Reference Breiter, Čopjaková and Škoda2009), Čopjakova et al. (Reference Čopjaková, Škoda, Vašinová Galiová and Novák2013, Reference Čopjaková, Škoda, Vašinová Galiová, Novák and Cempírek2015), Hönig et al. (Reference Hönig, Čopjaková, Škoda, Novák, Dolejš, Leichmann and Vašinová Galiová2014), Plášil and Škoda (Reference Plášil and Škoda2017) and Plášil et al. (Reference Plášil, Petříček, Locock, Škoda and Burns2018). In addition, once the new phase was discovered during routine scanning electron microscope (SEM) energy-dispersive spectroscopy studies, Dr Škoda made an assumption that it might be the first member of a well-known ‘ET’ (epidote–törnebohmite) polysomatic series including one epidote and two törnebohmite modules (that we propose below to name ET2) in contrast with all the other members of the series based on 1:1 alternation (ET). Subsequent structural investigations have now superbly confirmed his hypothesis. The Levinson suffix-modifier -(La) in the mineral name reflects the predominance of La among REE. Radekškodaite-(Ce) was named as an analogue of radekškodaite-(La) with Ce predominant over each of the other REE.
Both new minerals and their names have been approved by the Commission on New Minerals, Nomenclature and Classification of the International Mineralogical Association (IMA): IMA2018-107 [radekškodaite-(La), Kasatkin et al., Reference Kasatkin, Pekov, Zubkova, Chukanov, Polekhovsky, Belakovskiy, Ksenofontov, Agakhanov, Kuznetsov and Pushcharovsky2018] and IMA2019-042 [radekškodaite-(Ce), Kasatkin et al., Reference Kasatkin, Zubkova, Pekov, Chukanov, Škoda, Nestola, Agakhanov, Belakovskiy and Kuznetsov2019b]. The type specimens are deposited in the systematic collection of the Fersman Mineralogical Museum of the Russian Academy of Sciences, Moscow with the catalogue numbers 96279 [radekškodaite-(La)] and 96698 [radekškodaite-(Ce)].
Occurrence and general appearance
Polymineralic nodules containing both new minerals were found in the 1980s by local collectors at the historical dump no. 2 (Fig. 1) within the Mochalin Log valley and then deposited in the collection of one of the authors (A.M.K.). In 2017 they were sent to the senior author for routine electron microprobe analysis (EMPA) that revealed the novelty of two REE-bearing phases and started the complex investigation into them as new mineral species.

Fig. 1. Radek Škoda (left) and Anatoly V. Kasatkin (right) at historical dump No. 2 where nodules containing radekškodaite-(La) and radekškodaite-(Ce) were collected. The memorial plate between them says that Mochalin Log was visited in 1912 by Academician A.E. Fersman. August 2018. Photo: A.M. Kuznetsov.
As has been mentioned by Kasatkin et al. (Reference Kasatkin, Zubkova, Pekov, Chukanov, Škoda, Polekhovsky, Agakhanov, Belakovskiy, Kuznetsov, Britvin and Yu2020b), one of the most characteristic features of REE minerals from Mochalin Log is that in the majority of samples the gross Ce:La ratio varies widely but is most frequently close to 1:1 so that both Ce- and La-dominant members may occur in the same specimen. Therefore, in the text below, the absence of a particular Levinson suffix means the presence of Ce- and La-dominant mineral species together.
Radekškodaite-(La) occurs in polymineralic nodules composed mainly of allanite–ferriallanite, bastnäsite, fluorbritholite-(Ce) and törnebohmite. The new mineral is typically intergrown with ferriperbøeite-(La) (Kasatkin et al., Reference Kasatkin, Zubkova, Pekov, Chukanov, Škoda, Polekhovsky, Agakhanov, Belakovskiy, Kuznetsov, Britvin and Yu2020b) or is overgrown by it and often contains small inclusions of allanite–ferriallanite (Fig. 2). Other minerals associated closely with radekškodaite-(La) include albite, alexkuznetsovite-(Ce) (Kasatkin et al., Reference Kasatkin, Zubkova, Pekov, Chukanov, Škoda, Agakhanov, Belakovskiy and Pushcharovsky2020a), alexkuznetsovite-(La) (Kasatkin et al., Reference Kasatkin, Zubkova, Pekov, Chukanov, Škoda, Agakhanov, Belakovskiy and Pushcharovsky2019a), biraite-(Ce), cerianite-(Ce), REE-bearing epidote, ferriperbøeite-(Ce), heulandite-Ca, hollandite, lanthanite-(La), monazite, nontronite, perbøeite, perrierite, rhabdophane-(La), thorianite and thorite.

Fig. 2. Radekškodaite-(La) (Rsk) associated with ferriperbøeite-(La) (Fpb), fluorbritholite-(Ce) (Brt), allanite-(La) (All), törnebohmite-(La) (Tnb) and perrierite-(La) (Per). Black grains are quartz. The red circle shows the place from which the new mineral was extracted for the structural investigation. Polished section, specimen no. ML 60-2. SEM (back-scattered electron) image.
Radekškodaite-(Ce) occurs in polymineralic nodules composed mainly of bastnäsite, perbøeite-(Ce) and törnebohmite-(Ce) (Fig. 3). Other minerals present in the association with radekškodaite-(Ce) are allanite-(Ce), ancylite-(Ce), cerianite-(Ce), ferriallanite-(Ce), heulandite-Ca, lanthanite-(La), magnetite, perrierite-(Ce), quartz, thorianite and thorite.

Fig. 3. (a) Polished section of a nodule showing zones of brown radekškodaite-(Ce) (Rsk), black perbøeite-(Ce) (Prb), orange–yellow bastnäsite-(Ce)/-(La) (Bst) and greenish törnebohmite-(Ce) (Tnb). Size of the sample: 3.9 cm × 1.4 cm; (b) SEM (back-scattered electron) image on a fragment from (a), specimen no. ML 85-2. Black grains are quartz.
According to the distribution scale of RΕΕ minerals found at the Mochalin Log deposit (Kasatkin et al., Reference Kasatkin, Zubkova, Pekov, Chukanov, Škoda, Polekhovsky, Agakhanov, Belakovskiy, Kuznetsov, Britvin and Yu2020b), radekškodaite-(La) should be considered as rare and radekškodaite-(Ce) as very rare: among 300 nodules with REE-bearing minerals investigated by us, radekškodaite-(La) was found in 13 and radekškodaite-(Ce) only in two. These new minerals were not found in association with each other.
Both new minerals form isolated anhedral grains. Those of radekškodaite-(La) reach 0.35 mm × 0.75 mm, but typically they are much smaller. Although radekškodaite-(Ce) is rarer, its grains are bigger (up to 1 mm × 2 mm in the holotype specimen).
Physical properties and optical data
Both new minerals are greenish-brown, translucent in thin fragments, with brown streak and vitreous lustre. They are non-fluorescent under ultraviolet light. One direction of good cleavage and one direction of imperfect cleavage are observed. Based on the structure data (see below) we assume that good cleavage could be on {100}. Both minerals are brittle with an uneven fracture (observed under the SEM). The Vickers’ hardness (load 150 g) is equal to 871 kg mm–2 (range 804–919, n = 5) for radekškodaite-(La) and to 862 kg mm–2 (range 800–922, n = 5) for radekškodaite-(Ce); both values correspond to ca. 6½ on the Mohs scale. Density could not be measured due to lack of pure material in grains suitable for measurements. Density values calculated using the empirical formulae and the unit-cell parameters from single-crystal X-ray diffraction (XRD) data are 4.644 g cm–3 for radekškodaite-(La) and 4.651 g cm–3 for radekškodaite-(Ce).
Both new species are weakly pleochroic in marsh green hues with the absorption scheme Z > Y > X. Some areas found in grains of radekškodaite-(La) have a weak pleochroism in brownish tints with the same absorption scheme. Both minerals are optically biaxial (+). Radekškodaite-(La) has α = 1.790(7), β = 1.798(5), γ = 1.825(8) (589 nm), 2V = 60(10)° and 2Vcalc = 58°. Radekškodaite-(Ce) has α = 1.798(6), β = 1.806(6), γ = 1.833(8) (589 nm), 2V = 65(10)° and 2Vcalc = 58°. The 2V values are estimated by the curvature degree of the isogyre on the sections perpendicular to the optical axes. In both cases dispersion of optical axes is weak, r < v. Optical orientation was not determined for either mineral due to the anhedral shape of the grains.
Optical properties of radekškodaite-(La) were also examined in reflected light. The mineral is dark grey, very weakly anisotropic with whitish internal reflections. The bireflectance is very weak, ΔR = 0.2% (589 nm). The reflectance values have been measured in air by means of the MSF-21 microspectrophotometer (LOMO company, St. Petersburg, Russia) with the monochromator slit width of 0.4 mm and beam diameter of 0.1 mm. SiC (Reflectionstandard 474251, No. 545, Germany) was used as a standard. The reflectance values (R max/R min) are given in Table 1.
Table 1. Reflectance data (R, %) of radekškodaite-(La).

Data for wavelengths recommended by the IMA Commission on ore microscopy (COM) are marked in boldtype.
The Gladstone-Dale compatibility index (1 – K p/K c) is 0.036 for radekškodaite-(La) and 0.022 for radekškodaite-(Ce) using their empirical formulae and the unit-cell parameters determined from single-crystal X-ray data. Both values are rated as excellent (Mandarino, Reference Mandarino1981).
Radekškodaite-(La) and radekškodaite-(Ce) do not react with cold hydrochloric and nitric acids.
Raman spectroscopy
The Raman spectra (Figs 4 and 5) of radekškodaite-(La) and radekškodaite-(Ce) were obtained from polished sections by means of a Horiba Labram HR Evolution spectrometer. This dispersive, edge-filter-based system is equipped with an Olympus BX 41 optical microscope, a diffraction grating with 600 grooves per millimetre, and a Peltier-cooled, Si-based charge-coupled device (CCD) detector. After careful tests with different lasers (473, 532 and 633 nm), the 633 nm He–Ne laser with the beam power of 10 mW at the sample surface was selected for spectra acquisition to minimise analytical artefacts. A Raman signal was collected in the range of 100–4000 cm–1 with a 100x objective and the system operating in the confocal mode; beam diameter was ~1 μm and the lateral resolution ~2 μm. No visual damage of the analysed surface was observed at these conditions after the excitation. Wavenumber calibration was done using the Rayleigh line and low-pressure Ne-discharge lamp emissions. The wavenumber accuracy was ~0.5 cm–1, and the spectral resolution was ~2 cm–1. Band fitting was done after appropriate background correction, assuming combined Lorentzian–Gaussian band shapes using the Voight function (PeakFit; Jandel Scientific Software).

Fig. 4. Raman spectra of radekškodaite-(La) and radekškodaite-(Ce) excited by a 633 nm laser in the 100–1250 cm-1 region. The measured spectrum is shown by dots. The curve matched to dots is a result of spectral fit as a sum of individual Voigt peaks shown below the curve.

Fig. 5. Raman spectra of radekškodaite-(La) and radekškodaite-(Ce) excited by a 633 nm laser in the 2900–3600 cm-1 region. The measured spectrum is shown by dots. The curve matched to dots is a result of spectral fit as a sum of individual Voigt peaks shown below the curve.
The spectra of both new minerals are similar; the small differences are mainly in the ratios of band intensities and probably are of an orientational nature. In the Raman spectra of radekškodaite-(La) and radekškodaite-(Ce), three bands of O–H stretching vibrations are observed, which corresponds to the number of independent sites occupied by OH groups. Bands at 3438, 3337 and 3225 cm–1 in the spectrum of radekškodaite-(La) and 3384, 3325 and 3216 cm–1 in the spectrum of radekškodaite-(Ce) correspond to O–H-stretching vibrations of the O2–H, O1–H and O3–H groups, respectively (the assignment was made based on H⋅⋅⋅A distances equal to 2.968, 2.718 and 2.710 Å, respectively, in radekškodaite-(La) and 2.994, 2.77 and 2.73 Å, respectively, in radekškodaite-(Ce): see below the description of their crystal structures). Bands in the 1000–1100 cm–1 range correspond to stretching vibrations of the Si–O–Si fragments in Si2O7 groups, those in 850–1000 cm–1 region are due to stretching vibrations of apical Si–O bonds, and those in 650–690 cm–1 area to Al–O⋅⋅⋅H bending vibrations. Bands in the 290–630 cm–1 range correspond to mixed modes and overlapping bands of (Al,Fe3+,Mg)–O stretching vibrations, as well as bending vibrations of silicate groups, while those with frequencies below 300 cm–1 correspond to lattice modes involving REE–O, Ca–O and Fe2+–O stretching vibrations and librational vibrations of silicate groups.
The Raman spectra of both new minerals are close to those of västmanlandite-(Ce) (Holtstam et al., Reference Holtstam, Kolitsch and Andersson2005) and ferriperbøeite-(La) (Kasatkin et al., Reference Kasatkin, Zubkova, Pekov, Chukanov, Škoda, Polekhovsky, Agakhanov, Belakovskiy, Kuznetsov, Britvin and Yu2020b) in the range of 300–1000 cm–1 but significantly differ from them in the regions of stretching vibrations of the Si–O–Si fragments (1000 to 1100 cm–1), O–H stretching vibrations (above 3200 cm–1) and stretching vibrations involving REE and bivalent cations forming low-force-strength bonds (below 300 cm–1). In particular, in the spectra of radekškodaite-(La) and radekškodaite-(Ce), the vibrational bands of stretching vibrations of the Si–O–Si fragments are significantly weaker than in the ferriperbøeite-(La) spectrum, which reflects a lower Si2O7:SiO4 ratio in their composition.
The band at 687 cm–1 in the Raman spectrum of radekškodaite-(La) and the one at 690 cm–1 in the Raman spectrum of radekškodaite-(Ce) are close to the strong band in the Raman spectrum of allanite-(Ce) 689 cm–1 (Andò and Garzanti, Reference Andò and Garzanti2014; Č3) and can be tentatively assigned to the epidote-type module.
Chemical composition
Chemical data for both new minerals were obtained using a Cameca SX-100 electron microprobe (WDS mode, acceleration voltage of 15 kV, a beam current of 20 nA and a 3 μm beam diameter). The spectral interference of FKα and CeMζ were manually corrected using empirically determined correction factors. H2O was not determined directly due to the scarcity of pure material and was calculated by stoichiometry on the basis of O28(OH,F)3 taking into account that bond-valence sums for the O(1), O(2) and O(3) sites are close to 1 (see below). Both the crystal structure and Raman spectroscopy data confirm the presence of OH groups and the absence of carbonate and borate groups in both new species. Attempts to determine the valence state of iron using Mössbauer spectroscopy were unsuccessful due to a lack of pure material: small but abundant inclusions of allanite–ferriallanite and ferriperbøeite-(La) in radekškodaite-(La) and those of perbøeite-(Ce) in radekškodaite-(Ce) significantly affect the reliability of the data. For this reason, the Fe3+:Fe2+ ratio was determined on the basis of the charge balance requirement.
Analytical data are given in Table 2. Contents of other elements with atomic numbers higher than that of carbon are below detection limits.
Table 2. Average chemical composition of radekškodaite-(La) and radekškodaite-(Ce) (wt.%).

S.D. = standard deviation.
*Apportioned from total Fe measured as FeO content of 5.36 wt.% for radekškodaite-(La) and 5.07 wt.% for radekškodaite-(Ce) in accordance with bond-valence sums for the cationic sites and charge-balance requirement for the empirical formulae.
**For total iron calculated as FeO.
***Calculated by stoichiometry.
The empirical formulae calculated on the basis of O28(OH,F)3 are: radekškodaite-(La), (Ca0.98Th0.01La2.75Ce2.01Nd0.16Pr0.09)Σ6.00(Al3.28Fe3+0.63Fe2+0.57Mg0.34Mn0.15Ti0.03)Σ5.00Si7.00O28[(OH)2.91F0.09]; and radekškodaite-(Ce), (Ca0.79Mn0.16Th0.01Ce2.39La2.20Nd0.30Pr0.14)Σ5.99(Al3.43Fe2+0.62Fe3+0.52Mg0.42Ti0.01)Σ5.00Si7.00O28[(OH)2.92F0.08].
The idealised formulae are as follows. Radekškodaite-(La): (CaLa5)(Al4Fe2+)[Si2O7][SiO4]5O(OH)3 which requires CaO 3.52, FeO 4.50, La2O3 51.11, Al2O3 12.79, SiO2 26.38, H2O 1.70, total 100 wt.%. Radekškodaite-(Ce): (CaCe5)(Al4Fe2+)[Si2O7][SiO4]5O(OH)3 which requires CaO 3.50, FeO 4.49, Ce2O3 51.29, Al2O3 12.75, SiO2 26.28, H2O 1.69, total 100 wt.%.
X-ray crystallography
Powder XRD data for radekškodaite-(La) (Table 3) were collected with a Rigaku R-AXIS Rapid II single-crystal diffractometer equipped with a cylindrical image plate detector (radius 127.4 mm) using Debye-Scherrer geometry, CoKα radiation (rotating anode with VariMAX microfocus optics), 40 kV, 15 mA and exposure of 15 min. Angular resolution of the detector is 0.045°2θ (pixel size is 0.1 mm). The data were integrated using the software package Osc2Tab (Britvin et al., Reference Britvin, Dolivo-Dobrovolsky and Krzhizhanovskaya2017). The powder XRD data for radekškodaite-(Ce) (Table 4) were collected with a Supernova Rigaku-Oxford Diffraction diffractometer equipped with a Pilatus 200 K Dectris detector and an X-ray micro-source (MoKα radiation) with spot size of ~0.12 mm. The detector-to-sample distance was 68 mm. Data reduction was performed using CrysAlisPro (Rigaku-Oxford Diffraction). A standard phi scan mode as implemented in the powder power tool of CrysAlisPro was used for the powder data collection. Parameters of monoclinic unit cells refined from the powder data are for radekškodaite-(La): a = 8.958(6), b = 5.730(3), c = 25.13(2) Å, β = 116.68(5)° and V = 1153(2) Å3; and for radekškodaite-(Ce): a = 8.9982(6), b = 5.6720(4), c = 25.192(2) Å, β = 116.912(9)° and V = 1146.5(2) Å3.
Table 3. Powder X-ray diffraction data for radekškodaite-(La).

*For the calculated pattern, only reflections with intensities ≥1 are given.
**For the unit-cell parameters calculated from single-crystal data.
The eight strongest reflections are given in boldtype.
Table 4. Powder X-ray diffraction data for radekškodaite-(Ce).

*For the calculated pattern, only reflections with intensities ≥1 are given.
**These data were calculated from the structure determined by single-crystal X-ray data.
The eight strongest reflections are given in boldtype.
Single-crystal X-ray studies of both new minerals were carried out using an Xcalibur S diffractometer equipped with a CCD detector for grains analysed by electron microprobe and then extracted from the polished sections. A full sphere of three-dimensional data was collected. Data reduction was performed using CrysAlisPro Version 1.171.37.35 (Agilent Technologies, 2014). The data were corrected for Lorentz factor and polarisation effects.
The crystal structure of radekškodaite-(La) was solved by direct methods and refined with the use of the SHELX-97 software package (Sheldrick, Reference Sheldrick2008) to R = 0.0554 for 2837 unique reflections with I > 2σ(I). The crystal structure of radekškodaite-(Ce) was refined with a model of radekškodaite-(La) as the starting one and using the SHELX software package (Sheldrick, Reference Sheldrick2015) to R = 0.0769 for 3050 unique reflections with I > 2σ(I). The H atoms of the OH groups of both minerals were located from the difference-Fourier synthesis and their positions were restricted to keep O–H distances of 0.85(1) Å.
The crystal data, data collection information and structure refinement details for radekškodaite-(La) and radekškodaite-(Ce) are given in Table 5, atomic coordinates, thermal displacement parameters of atoms and site occupancies in Table 6, selected interatomic distances in Table 7 and hydrogen bonds in Table 8. Bond-valence calculations are given in Table 9. The crystallographic information files for both minerals have been deposited with the Principal Editor of Mineralogical Magazine and are available as Supplementary material (see below).
Table 5. Crystal data, data collection information and structure refinement details for radekškodaite-(La) and radekškodaite-(Ce).

*w = 1/[σ2(Fo 2) + (0.0391P)2 + 14.1116P]; P = {[max of (0 or Fo 2)] + 2F c2}/3 for radekškodaite-(La)
w = 1/[σ2(Fo 2) + (0.0165P)2 + 72.0691P]; P = {[max of (0 or Fo 2)] + 2F c2}/3 for radekškodaite-(Ce)
Table 6. Coordinates, equivalent displacement parameters (U eq, in Å2) and anisotropic displacement parameters of atoms (excepting H) and site occupancy factors (s.o.f.) for radekškodaite-(La) and radekškodaite-(Ce).

*Fixed during the refinement. In radekškodaite-(La) for M(1) Al vs. Fe was refined (e ref 17.50), for M(3) Fe vs. Mg was refined (e ref 23.40). Thus, on the basis of chemical data and e ref the M(1) site was assumed to be occupied by (Al0.49Fe3+0.36Mg0.15) possibly with a minor Ti4+ admixture; M(3) octahedron – by (Fe2+0.57Mg0.18Mn0.14Fe3+0.11); in radekškodaite-(Ce) for the A(1) site Ca vs. Ce was refined (eref 22.89), for M(1) Al vs Fe was refined (eref 15.86). Thus, on the basis of chemical data and e ref the A(1) site was assumed to be occupied by (Ca0.79Mn0.16REE 0.05) and the M(1) site by (Al0.62Fe3+0.23Mg0.15) possibly with a minor Ti4+ admixture.
**U iso.
Table 7. Selected interatomic distances (Å) and Si–O–Si angle (°) for radekškodaite-(La) and radekškodaite-(Ce).
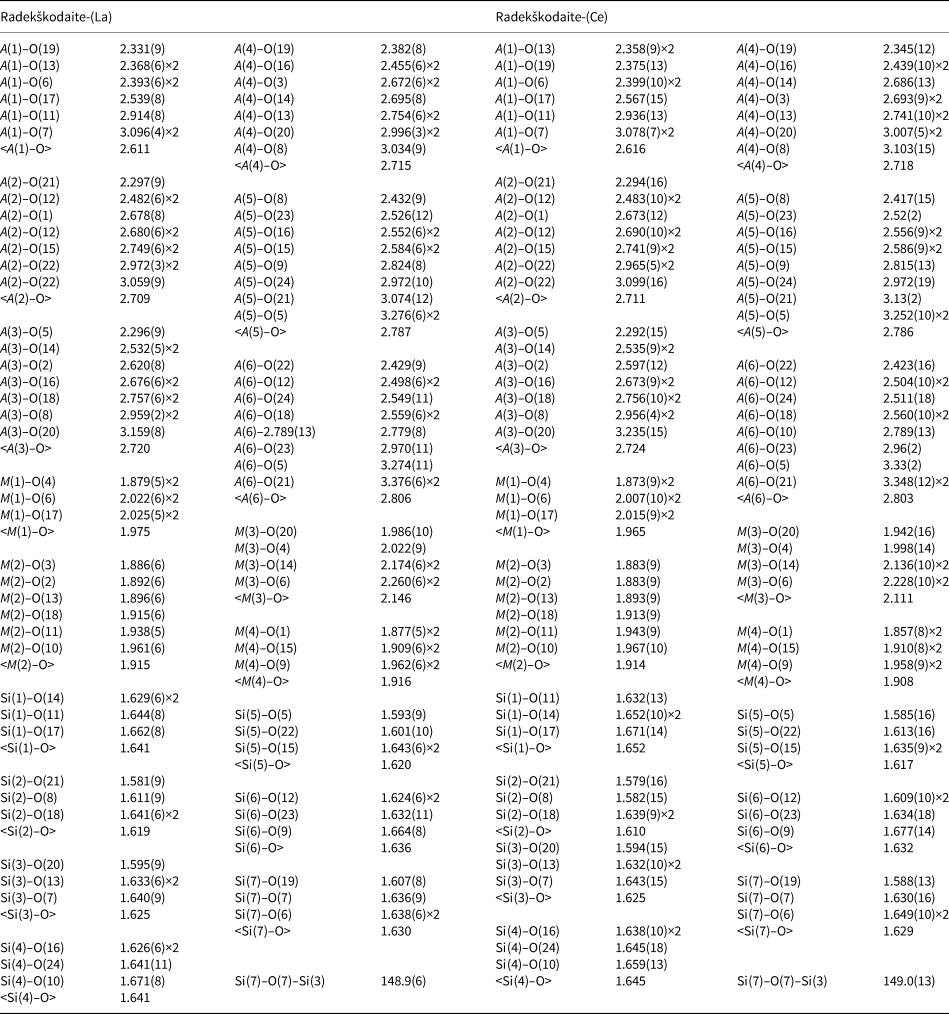
Table 8. Hydrogen-bond lengths (d in Å) and angles (in °)

D – donor; A – acceptor.
Table 9. Bond-valence calculations for radekškodaite-(La) and radekškodaite-(Ce). Parameters were taken from Gagné and Hawthorne (2015) and from Ferraris and Ivaldi (1988) for H bonding.

The values were calculated taking into account the refined occupancies for the M(1–4) and A(1) sites. For Fe cations in the M(3) site the bond-valence parameters of Fe2+ was used. The bond-valence parameters of La3+ were used for A(1–6) sites for radekškodaite-(La) and Ce3+ for radekškodaite-(Ce). For better clarity the split character of the O(23) and O(24) sites was not taken into account and these sites were considered as averaged with no splitting (the cations–O(23)/O(24) distances for bond-valence calculations including H bonding were taken for non-split sites).
Crystal structure
Radekškodaite-(La) and radekškodaite-(Ce) are isostructural and, thus there is one crystal-structure diagram (Fig. 6) for both minerals. They belong to a new structure type related to the members of the recently approved gatelite supergroup (Bonazzi et al., Reference Bonazzi, Holstam and Bindi2019). The latter are considered as iso-topological ET type polysomes within a polysomatic series which has epidote-type and törnebohmite-type structures as end-members (Shen and Moore, Reference Shen and Moore1982). Their structures can be described as a regular alternating 1:1 stacking of slabs with the epidote-type structure (E) and slabs with the törnebohmite-type structure (T) (Bonazzi et al., Reference Bonazzi, Bindi and Parodi2003, Reference Bonazzi, Lepore, Bindi, Chopin, Husdal and Medenbach2014; Holtstam et al., Reference Holtstam, Kolitsch and Andersson2005; Bindi et al., Reference Bindi, Holstam, Fantappiè, Andersson and Bonazzi2018). In the radekškodaite structure, regular alternation of one epidote-type module with two törnebohmite-type modules is observed. This results in the formation of a new polysome that we propose to name the ET2 type. The abbreviations of the structural sites in the text below are given in accordance with the above mentioned nomenclature of gatelite-supergroup minerals where the A sites are occupied by cations in large cavities, mainly Ca and REE, and the M sites have octahedral coordination and are occupied by di- and trivalent cations, namely Al, Fe2+, Fe3+, Mg, Mn2+ and Mn3+. (Bonazzi et al., Reference Bonazzi, Holstam and Bindi2019).

Fig. 6. The crystal structure of both radekškodaite-(La) and radekškodaite-(Ce). SiO4 tetrahedra are red. H atoms of OH groups are shown as small blue circles. Alternation of epidote-type slabs (E) and törnebohmite-type slabs (T) is shown. The unit cell is outlined.
The crystal structure of radekškodaite consists of chains of edge-sharing octahedra running along the b axis: single chains of the M(2)- and M(4)-centred octahedra and branched chains with the M(1)-centred octahedra in the central part with the M(3)-centred octahedra attached to them from both sides. The chains are linked via isolated [SiO4] tetrahedra and disilicate groups [Si2O7]. The A(1–6) sites occur in large cavities. The Si–O–Si angles in the disilicate groups of both species are very close to each other: 148.9(6)° in radekškodaite-(La) and 149.0(13)° in radekškodaite-(Ce) (Table 7).
Despite La3+ being a dominant REE in radekškodaite-(La), the Ce3+ scattering curve was used during the structure refinement for the A(1–6) sites of both new minerals because of the significant [dominant in radekškodaite-(Ce)] content of Ce3+ cations, minor amounts of heavier Nd3+ and Pr3+, and very minor amounts of Th4+.
According to the site-occupancy factors refinement and cation–anion distances (Table 7), the A(2–6) sites are occupied by REE cations. The refinement of their site occupancy factors gave the values very close to 1.0 and thus according to chemical data and the results of the refinement these sites are considered as fully occupied by REE cations. The A(1) site in both species is Ca dominant with minor REE admixture. In radekškodaite-(La) the refined A(1) site population is Ca0.955(5)LREE 0.045(5), where LREE implies light rare earth elements. Thus, the total composition of the A sites in radekškodaite-(La) is LREE 5.04Ca0.96, which is in a good agreement with the electron microprobe data (Table 2). In radekškodaite-(Ce) we also assume some Mn occupies the A(1) site due to the lack of cations at A sites and excess of cations at octahedral M sites, but we do not exclude that minor Mn may also occupy the M(3) site. For the A(1) site, Ca vs. Ce was refined (e ref = 22.89), and on the basis of chemical data and e ref, the A(1) site was assumed to be occupied by Ca, Mn and REE in the ratio 0.79:0.16:0.05 (e calc = 22.70). Thus, the total composition of the A sites in radekškodaite-(Ce) is LREE 5.05Ca0.79Mn0.16, which is also in a good agreement with the electron microprobe data (Table 2).
There are four octahedral M sites, M(1–4). For the M(1), M(2) and M(4) sites, Al vs. Fe was refined, and for the M(3) site, Fe vs. Mg was refined. The M(1), M(2) and M(4) sites are Al-dominant. The refined population of the M(2) and M(4) sites is Al0.939(10)Fe0.061(10) / Al0.926(14)Fe0.074(14), respectively, in radekškodaite-(La) and Al0.945(16)Fe0.055(16) / Al0.93(2)Fe0.07(2), respectively, in radekškodaite-(Ce). These sites centre the smallest octahedra with the mean distances of 1.915 Å [M(2)–O] and 1.916 Å [M(4)–O] in radekškodaite-(La) and 1.914 Å [M(2)–O] and 1.908 Å [M(4)–O] in radekškodaite-(Ce). The M(1)-centred octahedron is slightly larger, with the mean M(1)–O distance in radekškodaite-(La)/radekškodaite-(Ce) of 1.975/1.965 Å and the refined number of electrons (e ref) at the M(1) site being 17.50/15.86. This site is assumed to be occupied predominantly by Al with subordinate Fe3+ and Mg cations in the ratio Al:Fe3+:Mg equal to 0.49:0.36:0.15 in radekškodaite-(La) and 0.62:0.23:0.15 in radekškodaite-(Ce). The largest M(3) octahedron with the mean M(3)–O distance of 2.146/2.111 Å is predominately occupied by Fe2+ with subordinate Mg, Mn and Fe3+ in radekškodaite-(La) and by Mg and Fe3+ in radekškodaite-(Ce). According to electron microprobe data and the refined number of electrons in radekškodaite-(La)/radekškodaite-(Ce) (e ref = 23.40/22.22), the atomic ratio in the former is Fe2+:Mg:Mn:Fe3+ = 0.57:0.18:0.14:0.11, while in the latter it is Fe2+:Mg:Fe3+ = 0.63:0.27:0.10. During the refinement, splitting was found for the O(23) and O(24) sites which deviate from the m plane just as it does in the structures of gatelite-supergroup minerals. Bond-valence calculations (Table 9) confirm the above conclusions about distribution of cations between different sites.
Discussion
As noted above, the structures of both new minerals can be described as a regular alternating 1:2 stacking of slabs of epidote-type (E) and törnebohmite-type (T) structures (Fig. 6). The E modules are (001) slabs with the allanite composition CaREEAl2Fe2+[Si2O7][SiO4]X(OH), where X = O2– and/or F–. The T modules are ($\bar{1}$02) slabs with the törnebohmite composition [REE 2Al(SiO4)2(OH)].
Commonly, the REE nodules from Mochalin Log show concentric texture. The arrangement of the mineral assemblage follows a trend of decreasing REE content in minerals from the core to the rim of the nodule. A similar feature was observed in monazite replaced by allanite and REE-enriched epidote during metamorphism in metapelites (Finger et al., Reference Finger, Broska, Roberts and Schermaier1998), in magmatic REE-rich accumulations from aplitic rock at Jamestown, Colorado (Allaz et al., Reference Allaz, Raschke, Persson and Stern2015) and locally in the Bästnas-type deposits in Bergslagen, Sweden (e.g. Andersson, Reference Andersson2004). The most REE-enriched minerals occurring in central parts of the described nodules from Mochalin Log include bastnäsite, britholite, percleveite and torneböhmite, whereas the occurrence of REE-enriched epidote-supergroup members and structurally related silicates is confined to the outermost parts. The following generalised mineral sequence of torneböhmite–epidote polysomatic series from the core of the nodules to their rims is documented: torneböhmite → radekškodaite → perbøeite (ferriperbøeite) → allanite (ferriallanite) → REE-enriched epidote; more commonly, radekškodaite is missing in this scheme. In other words, members of the torneböhmite–epidote series alternate in the following order from the centre towards the margin of the nodule: T → ET2 → ET → E. The typical feature of the Mochalin Log REE assemblage is its enrichment in LREE, especially La and Ce, and very strong depletion in HREE and Y resulting in the situation when the contents of Y and any REE heavier than Sm is below its detection limit by EMPA (Kasatkin et al., Reference Kasatkin, Zubkova, Pekov, Chukanov, Škoda, Polekhovsky, Agakhanov, Belakovskiy, Kuznetsov, Britvin and Yu2020b and references therein). Composition of REE in radekškodaite follows the same trend (Figs 7 and 8). Such similar distribution of REE in many minerals belonging to various structure types indicates that is probably caused by the general geochemistry of the mineral-forming system. The origin of this geochemical feature (which could be named a ‘lanthanum anomaly’) at Mochalin Log is not in the scope of the present work. However, some local differences in distribution of individual lanthanides between coexisting minerals were observed and reflect the affinities of representatives of different structure types to La3+ or REE 3+ with smaller ionic radii. For example, REE enter mainly the A(2)–A(6) sites in radekškodaite and A(2)–A(4) in (ferri)perbøeite, and the average REE–O distance in radekškodaite is ~2% larger than in (ferri)perbøeite (cf. Kasatkin et al., Reference Kasatkin, Zubkova, Pekov, Chukanov, Škoda, Polekhovsky, Agakhanov, Belakovskiy, Kuznetsov, Britvin and Yu2020b). This difference could cause preferential incorporation of La into radekškodaites as compared to (ferri)perbøeite and be responsible for the coexistence of radekškodaite-(La) with (ferri)perbøeite-(Ce).

Fig. 7. Ternary plot showing distribution of REE at A sites in radekškodaite-(La) and radekškodaite-(Ce).

Fig. 8. Distribution of REE (chondrite-normalised; McDonough and Sun, Reference McDonough and Sun1995) in radekškodaite-(La) and radekškodaite-(Ce).
А mineral corresponding by chemical composition to a Ce-dominant member of ET2-type has been found recently by R. Škoda (pers. comm.) at Nya Bastnäs deposit, Bergslagen, Sweden. It occurs as thin lammellae (up to 10 μm thick) embedded in ferriperbøeite-(Ce). Associated minerals include torneböhmite-(Ce), ferriallanite-(Ce) bastnäsite-(Ce) and cerite-(Ce). The average composition of this mineral is (electron microprobe, wt.%; Fe2+/Fe3+ ratio calculated from charge balance, H2O – by stochiometry): CaO 3.30, La2O3 17.42, Ce2O3 26.37, Pr2O3 2.07, Nd2O3 5.46, MgO 1.97, MnO 0.09, FeO 1.10, Al2O3 8.79, Fe2O3 5.79, SiO2 26.09, F 0.16, H2O 1.60, –O=F –0.07, total 100.14. The empirical formula calculated on the basis of 18 cations and O28(OH,F)3 is: (Ca0.95Ce2.59La1.72Nd0.52Pr0.20Mn0.02)Σ6.00(Al2.78Fe3+1.17Mg0.79Fe2+0.25)Σ4.99Si7O28(OH2.86F0.14) (data kindly provided by R. Škoda). The tiny size of grains precludes the structural investigation of this mineral, however, its structural relation with radekškodaite is very likely.
Supplementary material
To view supplementary material for this article, please visit: https://doi.org/10.1180/mgm.2020.64.
Acknowledgements
Dr Radek Škoda is acknowledged for his valuable help in collecting WDS and Raman data of the new minerals, and for discussion and useful advice. We thank Associate Editor Irina Galuskina, Structure Editor Daniel Atencio, Principal Editor Stuart Mills and two anonymous referees for constructive comments that improved the quality of the manuscript. This study was supported by the Russian Foundation for Basic Research, grant no. 18-05-00332 (in part of XRD structure studies and crystal chemistry). This work was partly performed in accordance with the state task, state registration no. ААА-А19-119092390076-7 for N.V.C. The technical support by the SPbSU X-Ray Diffraction Resource Center in the powder XRD study is acknowledged.