Introduction
Charophyte paleobiogeography has been studied widely in the past two decades. Bhatia (Reference Bhatia2006) suggested that the living Eurasian species Lychnothamnus barbatus (Meyen, Reference Meyen1827) von Leonhardi, Reference von Leonhardi1863 dispersed from Western Europe (late Miocene) eastward to other parts of Europe and Central Asia in the Pliocene, with hypothesized dispersals to the Himalayas in the late Pliocene and to Australia in the Pleistocene. Martín-Closas and Wang (Reference Martín-Closas and Wang2008) studied the paleobiogeography of the well-documented, long-lasting fossil record of Cretaceous charophyte Atopochara trivolvis Peck, Reference Peck1941 and concluded that the species probably originated in Eastern Europe, later achieving a global distribution. Some charophyte species have dispersed much faster than others. For example, Sanjuan and Martín-Closas (Reference Sanjuan and Martín-Closas2015a) studied the Eocene–Oligocene Nitellopsis (Tectochara) merianii Braun and Unger in Unger, Reference Unger1852 comb. Grambast and Soulié-Märsche, Reference Grambast and SouliéMärsche1972–Nitellopsis obtusa (Desvaux in Loiseleur, Reference Loiseleur Deslongschamps1810) Groves, Reference Groves1919 and Lychnothamnus stockmansii (Grambast, Reference Grambast1957) Soulié-Märsche, Reference Soulié-Märsche1989–Lychnothamnus major (Grambast and Paul, Reference Grambast and Paul1965) Soulié-Märsche, Reference Soulié-Märsche1989 lineages, and they surmised that the L. stockmansii–L. major lineage achieved a Eurasian distribution soon after its first appearance in the late Eocene, and the N. (T.) merianii–N. obtusa lineage expanded to Eastern Europe from Spain about 10 million years after its first appearance.
From a paleogeographical perspective, Europe and Western Asia were separated from Northeastern Asia by the Turgai Straits from the Middle Jurassic (Martin and Averianov, Reference Martin and Averianov2004; Joyce and Rabi, Reference Joyce and Rabi2015) to the end of the late Eocene (McKenna, Reference McKenna1975; Martin and Averianov, Reference Martin and Averianov2004; Bosboom et al., Reference Bosboom, Mandic, Dupont-Nivet, Proust, Ormukov and Aminov2017). The Turgai Strait, also known as the West Siberian Sea, was a large shallow saline water body that connected the Arctic Ocean and Tethys Sea and separated the Asian and European continents. The dispersal of charophytes from Asia to Europe in the middle to late Eocene appears to have occurred before the retreat of the Turgai Strait in both the Tarim area and the Siberian Basin by the end of the late Eocene. Our recovery of late Paleocene charophyte fossils in Central Asia (western China) adds greatly to the paleobiogeographic story of intercontinental dispersals and distributions of charophyte taxa in the Paleogene. We use charophyte data to discuss potential agents of dispersal and paleoenvironments and to form the basis to explain the expansion and colonization of various areas by charophytes.
Geological background
The Junggar Basin is one of the largest sedimentary basins in Xinjiang, northwestern China. It is surrounded by the Zaier and Hala'alate Mountains to the northwest, the Aletay and Kelamaili Mountains to the northeast, and the Tian Shan and Bogda mountains to the south (Tang et al., Reference Tang, Zhang, Pe-Piper, Piper, Guo and Li2020). The basin includes many fossiliferous horizons from the Cretaceous and Paleogene. The latest Paleocene South Gobi fossil area (46°5′19″N, 88°11′9″E) is situated in an unnamed early Cenozoic lithological unit located in the northeastern part of the Junggar Basin in the Gurbantünggüt Desert (Fig. 1). This horizon is known primarily for its fossil vertebrate assemblage (Ni et al., Reference Ni, Li, Stidham, Li, Lu and Meng2016), but fossils of charophytes also have been discovered in this area. This fossiliferous unnamed geological unit comprises a set of sediments consisting of reddish-brown mudstones interbedded with a few grayish-green or yellowish-gray fluvial sandstone beds or lenses (Ni et al., Reference Ni, Li, Stidham, Li, Lu and Meng2016). The fossils of charophytes were recovered from the sandy mudstone unit (at the height of ~7.5 m in Fig. 2).
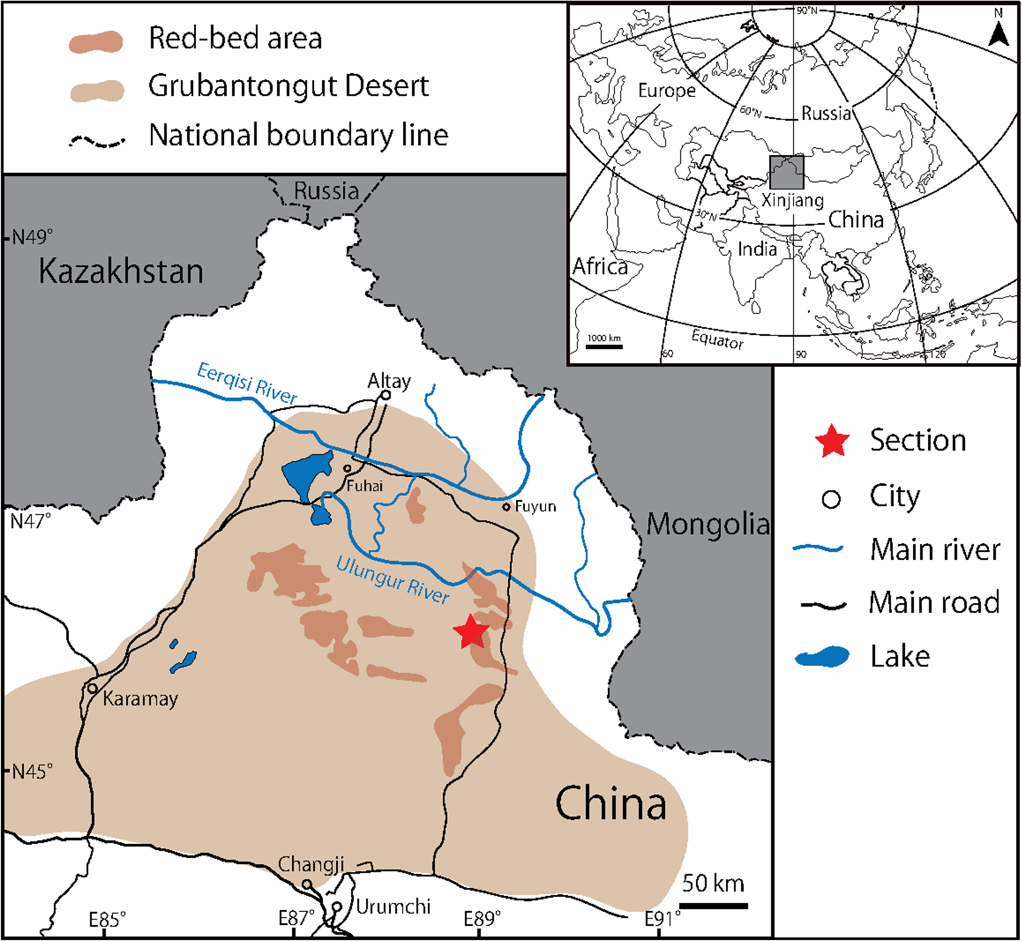
Figure 1. Geographic location. Maps showing location of the South Gobi area in the Northeastern Junggar Basin in Xinjiang, China.

Figure 2. Correlation and age of charophyte assemblages. Charophytes and mammalian fossils from the South Gobi area are correlated with the Subeng and Nomogen III faunas from the Erlian Basin in Inner Mongolia, China. The age of the South Gobi fossil assemblage is estimated as late Gashatan Asian Land Mammal Age, roughly equivalent to the Clarkforkian North American Land Mammal Age. Modified from Ni et al. (Reference Ni, Li, Stidham, Li, Lu and Meng2016).
The geological age of the deposit was determined through mammalian biostratigraphy (Ni et al., Reference Ni, Li, Stidham, Li, Lu and Meng2016). Mammalian fossils in the unit include species of insectivorans (Bumbanius ningi Missiaen and Smith, Reference Missiaen and Smith2008 and Asionyctia guoi Missiaen and Smith, Reference Missiaen and Smith2005), Glires (Tribosphenomys minutus Meng and Wyss, Reference Meng and Wyss2001 and Neimengomys qii Meng et al., Reference Meng, Ni, Li, Beard, Gebo, Wang and Wang2007), plesiadapiforms (Subengius mengi Smith, Van Itterbeeck, and Missiaen, Reference Smith, Van Itterbeeck and Missiaen2004), arctostylopids (Anatolostylops sp. aff. Anatolostylops zhaii Wang et al., Reference Wang, Meng, Ni and Beard2008), deltatheroidan marsupials (Gurbanodelta kara Ni et al., Reference Ni, Li, Stidham, Li, Lu and Meng2016), and multituberculates (Mesodmops cf. M. tenuis Missiaen and Smith, Reference Missiaen and Smith2008). Among them, seven species (all except Anatolostylops sp. aff. A. zhaii) were reported as also occurring in the well-constrained late Paleocene Subeng Fauna from the Erlian Basin of Inner Mongolia, China (Ni et al., Reference Ni, Li, Stidham, Li, Lu and Meng2016). In addition, the specimens of Anatolostylops sp. aff. A. zhaii (an arctostylopid) from the South Gobi Fauna are much larger than those of Palaeostylops iturus Matthew and Granger, Reference Matthew and Granger1925 from the late Paleocene Subeng Fauna, and they approach the size of Anatolostylops zhaii from the early Eocene Nomogen III Fauna of Inner Mongolia. Therefore, the mammalian fossils and the South Gobi deposit are thought to be from the latter part of the Paleocene (lacking any Eocene biostratigraphic indicators). The Paleocene–Eocene Boundary in the Erlian Basin lies within or just below the “Gomphos” bed, and the absence of the common Gomphos (Glires, Mammalia) in the South Gobi area also points to an age older than the Eocene (Ni et al., Reference Ni, Li, Stidham, Li, Lu and Meng2016 and references therein) (Fig. 2). Therefore, this latest Paleocene South Gobi Fauna (and charophyte flora) can be correlated to the late Gashatan Asian Land Mammal Age, which is roughly equivalent to the Clarkforkian North American Land Mammal Age, with the age estimate of ~56–57 Ma (Fig. 2).
Materials and methods
Approximately 20 tons of sedimentary matrix (composing one massive sample) was collected from the latest Paleocene fossiliferous layer in Section A (Section, Fig. 1). The sample was screen washed with a minimum mesh size of 0.2 mm to maximize fossil recovery. For the semi-quantitative analyses, ~100 gyrogonite specimens were measured for each species (e.g., Gyrogona lemani capitata Grambast and Grambast-Fessard, Reference Grambast and Grambast-Fessard1981). When there were fewer than 100 specimens available for a species (e.g., Lychnothmanus vectensis (Groves, Reference Groves, Reid and Chandler1926) Soulié-Märsche, Reference Soulié-Märsche1989 and Peckichara torulosa var. varians (Dollfus and Fritel, Reference Dollfus and Fritel1919) Sanjuan, Vicente, and Eaton, Reference Sanjuan, Vicente and Eaton2020), all the specimens were measured. Morphometric data from specimens of G. lemani capitata and L. vectensis were compiled into statistical charts, but charts were not made for P. torulosa var. varians because there are too few specimens. The specimens were measured using the ZEN 2 system stored with a Zeiss stereomicroscope (Stemim 508) at the Nanjing Institute of Geology and Paleontology, Chinese Academy of Sciences. Specimens were imaged using a Zeiss Evo MA25 scanning electron microscope at the Key Laboratory of Vertebrate Evolution and Human Origins of the Chinese Academy of Sciences, Beijing.
Repository and institutional abbreviation
All specimens in this study are deposited in the Institute of Vertebrate Paleontology and Paleoanthropology (IVPP), Chinese Academy of Sciences, Beijing, China.
Systematic paleontology
Division Charophyta Migula, Reference Migula and Rabenhorst1897
Class Charophyceae Smith, Reference Smith1938
Order Charales Lindley, Reference Lindley1836
Family Characeae Agardh, Reference Agardh1824
Subfamily Charoideae Braun in Migula, Reference Migula and Rabenhorst1897
Genus Peckichara Grambast, Reference Grambast1957
Type species
Peckichara varians Grambast, Reference Grambast1957 from the Paleocene to lowermost Eocene (Sparnacian local stage) of the Paris Basin, France.
Peckichara torulosa var. varians (Dollfus and Fritel, Reference Dollfus and Fritel1919) Sanjuan, Vicente, and Eaton, Reference Sanjuan, Vicente and Eaton2020
Figure 3.1–3.7
- Reference Dollfus and Fritel1919
Chara torulosa Dollfus and Fritel, p. 249, fig. 9.
- Reference Grambast1957
Peckichara torulosa; Grambast, p. 15, pl. 7, figs. 1–6.
- Reference Grambast1957
Peckichara varians; Grambast, p. 14, pl. 8, figs. 1–8.
- Reference Sanjuan, Vicente and Eaton2020
Peckichara torulosa var. varians; Sanjuan, Vicente, and Eaton, p. 7, pl. 3, A–F.
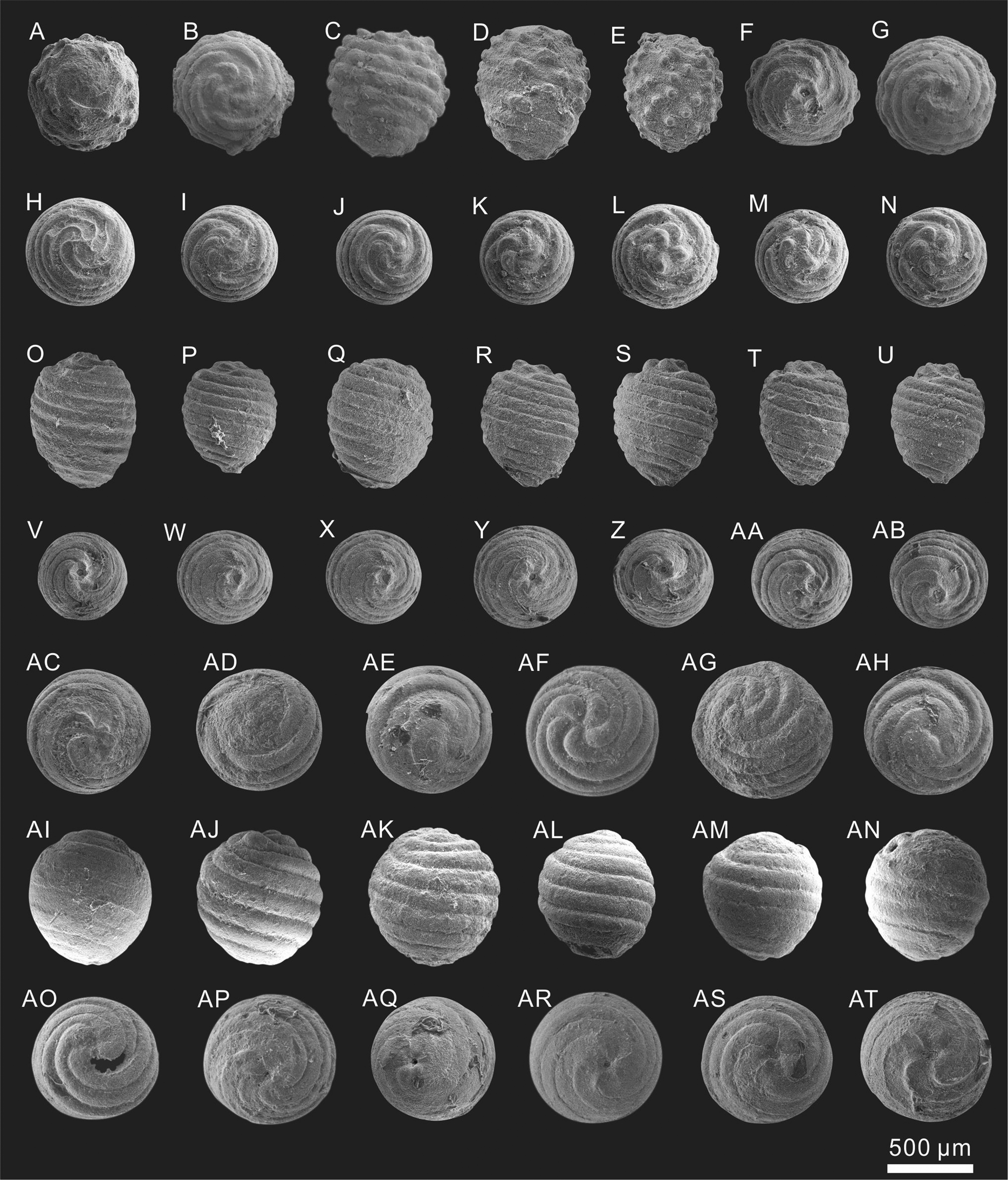
Figure 3. Scanning electron microscope images of fossil charophyte gyrogonites from the latest Paleocene South Gobi area in the Junggar Basin. (1–7) P. torulosa var. varians: (1, 2) apical view; (3–5) lateral view; (6, 7) basal view. (8–28) L. vectensis: (8–14) apical view; (15–21) lateral view; (22–28) basal view. (29–46) G. lemani capitata: (29–34) apical view; (35–40) lateral view; (41–46) basal view.
Holotype
Gyrogonites (with collection number not reported) from marls and lignites in Cramant (Marne), Sarron (Oise) according to Dollfus and Fritel (Reference Dollfus and Fritel1919, fig. 9).
Occurrence
Peckichara torulosa var. varians (originally as P. varians) is known from the Paleocene to the early Eocene of Europe and China (Li et al., Reference Li, Wang, Zhang, Lu and Martín-Closas2016 and references therein).
Description
The P. torulosa var. varians gyrogonites from the South Gobi area are medium to large; 688–770 μm high and 583–708 μm wide. Their isopolarity index ranges between 106 and 118. They are prolate spheroidal to subprolate with seven to eight convolutions in lateral view. The spiral cells are flat to convex, 90–120 μm wide, and ornamented by individualized and prominent tubercles. The tubercles are rounded or oval, usually as wide as the spiral cells, and are spaced regularly (Fig. 3.3, 3.5) or irregularly (Fig. 3.4). The surface ornamentation usually disappears near the apex. The apex is rounded to flat, with a slight to moderate thinning and narrowing of the periapical spiral cells. Sometimes well-developed apical nodules are present, and they can vary in size and shape. Some specimens display small, individualized nodules, and others exhibit thick and large nodules, forming an apical rosette. The base is rounded, with a pentagonal funnel and a pentagonal pore (50–60 μm).
Materials
Approximately 10 gyrogonites have been recovered from the South Gobi area.
Remarks
Two varieties of P. torulosa have been proposed by Sanjuan et al. (Reference Sanjuan, Vicente and Eaton2020). The gyrogonites that bear a mid-cellular crest and massive apical nodules have been termed P. torulosa var. torulosa, and those that exhibit isolated or irregularly connected tubercles along the spiral cells and isolated small apical nodules are called P. torulosa var. varians. The fossil population in this study is referred to P. torulosa var. varians due to the presence of individualized, prominent, irregularly rounded or elongated tubercles on the spiral cells.
Genus Lychnothamnus (Ruprecht, Reference Ruprecht1845) von Leonhardi, Reference von Leonhardi1863 emend. Braun in Braun and Nordstedt, Reference Braun and Nordstedt1882
Type species
Lychnothamnus barbatus (originally as Chara barbata) Meyen, Reference Meyen1827 from the Cenozoic of “Plötzensee bei Berlin” (Germany).
Lychnothamnus vectensis (Groves, Reference Groves, Reid and Chandler1926) Soulié-Märsche, Reference Soulié-Märsche1989
Figures 3.8–3.28, 4
- Reference Groves, Reid and Chandler1926
Chara vectensis Groves, p. 172, pl. 2, figs. 2–8.
- Reference Grambast1958
Stephanochara vectensis; Grambast, p. 158.
- Reference Soulié-Märsche1989
Lychnothamnus vectensis; Soulié-Märsche, p. 160.

Figure 4. Morphometric data of Lychnothmanus vectensis (30 gyrogonites) in the South Gobi area, Junggar Basin. (1–4) Frequency distributions: (1) gyrogonite height; (2) gyrogonite width; (3) isopolarity index; (4) number of convolutions. (5) Dispersion graph of gyrogonite height/gyrogonite width.
Holotype
Gyrogonites (V. 18321 and V. 18355) from the Bembridge beds in Gurnard Bay on the Isle of Wight, UK (Groves, Reference Groves, Reid and Chandler1926, pl. 2, figs. 2–8).
Occurrence
Lychnothmanus vectensis was reported first in the late Eocene Bembridge beds in Gurnard Bay on the Isle of Wight, UK (Groves, Reference Groves, Reid and Chandler1926). It was reported later from the late Eocene of the Paris Basin (Riveline, Reference Riveline1986) and the latest Priabonian to earliest Rupelian of the Ebro Basin (Sanjuan and Martín-Closas, Reference Sanjuan and Martín-Closas2014). In the United States, Vicente et al. (Reference Vicente, Sanjuan, Eaton and Villanueva-Amadoz2020) and Sanjuan et al. (Reference Sanjuan, Vicente and Eaton2020) reported Lychnothamnus sp. (probably a form with an affinity to L. vectensis) in the early Eocene of Utah. In China, L. vectensis is known from the Eocene of the Qaidam Basin on the Tibetan Plateau (Li et al., Reference Li, Wang, Cui, Zhang, Wang and Zhang2020), and Lychnothamnus aff. L. vectensis was reported from the Songliao Basin of China by Li et al. (Reference Li, Wang, Zhang, Wan and Martín-Closas2019).
Description
The Lychnothamnus vectensis gyrogonites from the latest Paleocene South Gobi area are medium to large; 638–845 μm high (mean: 737 μm; standard deviation: 50) and 531–660 μm wide (mean: 596 μm; standard deviation: 32). The isopolarity index ranges from 114 to 149 (mean: 124; standard deviation: 8). They are prolate spheroidal to subprolate with 8–10 convolutions visible in lateral view. The spiral cells are generally convex, but also sometimes flat, and their width ranges between 75 and 100 μm. The apex of the periapical spiral cell is rounded and displays marked thinning. Apical nodules usually are present, and sometimes the nodules are comma or crescent shaped. The apical rosettes are usually well developed, with the apical nodules joined completely at the apical center to form a highly protruding cap. The base is rounded, with a funnel and a pentagonal basal pore ~30–40 μm.
Materials
Thirty gyrogonites have been recovered from the South Gobi area.
Remarks
The size of the gyrogonites of L. vectensis is normal for each population measured separately (Sanjuan and Martin-Closas, Reference Sanjuan and Martin-Closas2015b). However, the gyrogonite's height range becomes extremely large if all polymorphs from different localities and different latitudes are considered together. Sanjuan and Martin-Closas (Reference Sanjuan and Martin-Closas2015b) showed that the variation in size of the gyrogonites exhibits a latitudinal polarity according to the morphometric analysis carried out in three populations of L. vectensis from the Hampshire, Paris, and Ebro Basins in Europe. The size of the South Gobi population (current latitude: 46°5′19″N) is similar to that of the Paris Basin (current latitude: ~48°N).
In contrast to the type L. vectensis population from the Isle of Wight (Groves, Reference Groves, Reid and Chandler1926; Sanjuan and Martín-Closas, Reference Sanjuan and Martin-Closas2015b), with more prominent apical rosette and apical nodules being separated, the apical nodules of Lychnothamnus aff. L. vectensis in the Songliao Basin population are fused. However, the ornamentation of gyrogonites may change over time, considering the evolutionary trends of other well-supported charophyte anagenetic lineages (e.g., in lineage Peckichara pectinata Grambast, Reference Grambast1971; Vicente and Martín-Closas, Reference Vicente and Martín-Closas2018). Lychnothamnus aff. L. vectensis probably represents an ancestor of L. vectensis s.s.
Genus Gyrogona Lamarck, Reference Lamarck1804 ex Lamarck, Reference Lamarck1822 emend. Grambast, Reference Grambast1956
Type species
Gyrogona medicaginula Larmark, Reference Lamarck1804 from the Oligocene cherts of the Paris Basin, France.
Gyrogona lemani capitata Grambast and Grambast-Fessard, Reference Grambast and Grambast-Fessard1981
Figures 3.29–3.46, 5
- Reference Brongniart1822
Chara lemani Brongniart, p. 322, pl. 17, fig. 4.
- Reference Pia and Hirmer1927
Gyrogonites lemani; Pia, p. 90.
- Reference Grambast and Grambast-Fessard1981
Gyrogona lemani capitata; Grambast and Grambast-Fessard, p. 12. pl. 2, figs. 8–12.

Figure 5. Morphometric data of Gyrogona lemani capitata (99 gyrogonites) in the South Gobi area, Junggar Basin. (1–4) Frequency distributions: (1) gyrogonite height; (2) gyrogonite width; (3) isopolarity index; (4) number of convolutions. (5) Dispersion graph of gyrogonite height/gyrogonite width.
Holotype
Gyrogonites (C121-7) from the Pont-Bernard, commune de Brény (Aisne), France (Grambast and Grambast-Fessard, Reference Grambast and Grambast-Fessard1981, p. 12. pl. 2, figs. 8–12).
Occurrence
Gyrogona lemani capitata was reported in the Lutetian of France, including Pont-Bernard, commune de Brény (Aisne), Cuis (Marne), Pargnan, and Neuilly-Saint-Front (Grambast and Grambast-Fessard, Reference Grambast and Grambast-Fessard1981).
Description
The G. lemani capitata gyrogonites are large, with a height of 701–892 μm (mean: 784 μm; standard deviation: 38) and a width of 634–838 μm (mean: 739 μm; standard deviation: 37). Their isopolarity index ranges between 96 and 119 (mean: 106; standard deviation: 5). The shape is oblate spheroidal to prolate spheroidal, with seven to eight convolutions visible in lateral view. The spiral cells are flat to convex, with a width of 115–130 μm. The apex of the gyrogonite is rounded, with moderate periapical thinning of the spiral cells, and ornamented with apical nodules on convex specimens. The base is rounded, with a pentagonal basal pore ~30–40 μm in diameter.
Materials
More than 100 gyrogonites have been recovered from the South Gobi area.
Remarks
The G. lemani capitata population in this study is smaller in size than that from Pont-Bernard, commune de Brény (Aisne) in Grambast and Grambast-Fessard (Reference Grambast and Grambast-Fessard1981), which is 950–1,250 μm high and 925–1,200 μm wide. However, the isopolarity index and number of convolutions in the two populations are similar, with an isopolarity index of 96–116 and six to eight convolutions for the French population.
Discussion
The late Paleocene fossil charophytes recorded in the South Gobi area include the oldest global record of Gyrogona lemani capitata and the second-oldest record for Lychnothamnus vectensis. The latest Paleocene G. lemani capitata from the South Gobi area is older than the middle Eocene record from France (Fig. 6). The oldest record of L. vectensis (as Lychnothamnus aff. L. vectensis) is from the Cretaceous–Paleocene transition deposits of the Songliao Basin in northeastern China (Li et al., Reference Li, Wang, Zhang, Wan and Martín-Closas2019), and the oldest European record is from the late Eocene–early Oligocene transition (Fig. 6). In the United States, its oldest record is probably early Eocene (Vicente et al., Reference Vicente, Sanjuan, Eaton and Villanueva-Amadoz2020). These species clearly were present in Asia for some time before their dispersal to North America and Europe. The older age of the American Lychnothamnus aff. L. vectensis relative to those in Europe could suggest a pattern of dispersal from Asia to North America first. The dispersal of L. vectensis to Europe in the late Eocene could have occurred alongside known tetrapod dispersal events between Europe and North America or through a route (according to known mammalian dispersal patterns) from Asia to Europe (Stidham and Ni, Reference Stidham and Ni2014; Stidham et al., Reference Stidham, Townsend and Holroyd2020). The occurrence of these widespread charophyte taxa in Asia (and North America) before their first records in Europe parallels that of a widely distributed bird group known in the middle Eocene of North America (Utah) and Central Asia (Uzbekistan) before their oldest record in the late Eocene of Western Europe (Stidham et al., Reference Stidham, Townsend and Holroyd2020). For the Asia-to-Europe potential dispersal route, given physical distances and known geographic barriers (i.e., the Turgai Strait and Tethys Seaway), the charophyte lineages likely would have dispersed from Central Asia to Eastern Europe across the straits in the middle to late Eocene.

Figure 6. Potential biogeographic dispersal routes of Lychnothmanus vectensis and Gyrogona lemani capitata. Lychnothmanus vectensis: its earliest record is ~300 kyr before the Cretaceous–Paleogene boundary transition, distributed in Xinjiang in the latest Paleocene, in the early Eocene of the United States, and in Spain and France in late Eocene–early Oligocene. Gyrogona lemani capitata: its earliest record is in the latest Paleocene in Xinjiang, then dispersed to France in the middle Eocene. The different starred localities are numbered chronologically. Paleogeographic map of the Earth at ~60 Ma reproduced with permission from “Global Paleogeography and Tectonics in Deep Time ©2016 Colorado Plateau Geosystems Inc.”
Biotic factors could have led to the intercontinental dispersal of charophytes. Birds are known to carry charophyte oospores in their stomachs and externally in mud adhering to their feet and feathers (Proctor, Reference Proctor1962, Reference Proctor1980; Proctor et al., Reference Proctor, Malone and DeVlaming1967; Trabelsi et al., Reference Trabelsi, Sames, Salmouna, Piovesan, Rouina, Houla, Touir and Soussi2015). Through the alimentary tract of common migratory water birds, many freshwater algae probably could be carried readily between lakes 100–150 miles apart, and through the caecum, they may be carried several times this distance (Proctor, Reference Proctor1959). For example, oospores were found in the digestive tracts of 24% of the birds shot in the Camargue, France (including the red-crested pochard, Netta rufina Pallas, Reference Pallas1773), with charophytes, including oospores and vegetative parts, representing 21% of the stomach contents of the ducks (Allouche et al., Reference Allouche, Roux and Tamisier1988). Eurasian teal (Anas crecca Linnaeus, Reference Linnaeus1758) also ingested propagules of charophyte macrophytes, with 23% of the total weight ingested consisting of charophyte oospores (Tamisier, Reference Tamisier1971). Furthermore, Proctor (Reference Proctor1962) found that 34% of the oospores taken from mallards (Anas platyrhynchos Linnaeus, Reference Linnaeus1758) were able to germinate.
Our results show the direction and timing of charophyte dispersal is penecontemporaneous with the intercontinental dispersals of some extinct birds. Those bird records include a group of small-sized pangalliforms in the middle Eocene of Central Asia, Europe, and Africa, along with North America (Stidham et al., Reference Stidham, Townsend and Holroyd2020), ameghinornithid-like birds in the middle Eocene of Inner Mongolia, Eocene and Oligocene of Europe, and early Oligocene of Egypt (Stidham and Smith, Reference Stidham and Smith2015; Stidham and Wang, Reference Stidham and Wang2017 and references therein), and the stem anatids Romainvilliinae from the late Eocene of Xinjiang and the late Eocene and Oligocene of Europe (Stidham and Ni, Reference Stidham and Ni2014). The geographic distributions of those birds imply intercontinental dispersals in the Eocene across the Turgai Strait, demonstrating that the dispersal mechanism of charophytes along with birds is plausible. Furthermore, considering the hypothesized dispersals of the lineages of Lychnothamnus stockmansii–L. major and Nitellopsis (Tectochara) merianii–N. obtusa from Europe to Asia in the late Eocene (Sanjuan and Martín-Closas, Reference Sanjuan and Martin-Closas2015b), the dispersal routes of charophytes were likely bidirectional in the Eocene between Europe and Asia.
We explored the sedimentary facies of Asia, North America, and Europe to interpret the paleoenvironments of charophytes when they arrived. Proctor (Reference Proctor1959) mentioned suitable media or environmental conditions are important for Chara zygotes to germinate and colonize. Gyrogona lemani capitata is found in sandstones and sandy mudstones attributed to deposition in intermittent riverine environments in the uppermost Paleocene of the northeastern Junggar Basin, Xinjiang (Ni et al., Reference Ni, Li, Stidham, Li, Lu and Meng2016). It was later found in middle Eocene beds of France. It occurs in marls from Pont-Bernard, commune de Brény (Aisne), Cuis (Marne), Pargnan, and Neuilly-Saint-Front of France, corresponding to sedimentation in shallow lacustrine facies (Grambast and Grambast-Fessard, Reference Grambast and Grambast-Fessard1981). Therefore, it appears that either G. lemani capitata shifted or varied its habitat preferences during its biogeographic history, from fluviatile environments in the South Gobi area to shallow lacustrine belts in the middle Eocene of Europe, or it occupied a wider array of habitats than previously recognized. Lychnothmanus aff. L. vectensis was reported from shallow lacustrine facies of the Songliao Basin (Li et al., Reference Li, Wang, Zhang, Wan and Martín-Closas2019) and in lake delta front facies of the Jiaolai Basin, eastern China. To the west, in the northeastern Junggar Basin, Xinjiang, Lychnothmanus aff. L. vectensis is present in fluviatile sandstones and sandy mudstones. Lychnothmanus aff. L. vectensis recovered from Utah (United States) came from beds of siltstones containing mainly thin thalli, ostracods, gastropods, and trace fossils that were interpreted as representing floodplain environments. Assuming all these findings represent autochthonous populations, these data suggest the species occupied a wide range of habitats in China. When it dispersed to Europe around the Eocene–Oligocene boundary transition, L. vectensis appears to have become restricted in its habitats to shallow lacustrine facies. For example, L. vectensis is in the shallow lacustrine deposits of the Artés Formation, Ebro Basin, Catalonia (Sanjuan and Martín-Closas, Reference Sanjuan and Martin-Closas2015b) and the lacustrine bluish marls of the Argenteuil Formation in Loiret (Centre-Val de Loire) in the Paris Basin, France (Riveline, Reference Riveline1986). In addition, L. vectensis was found in the lacustrine deposits from the Bembridge Marls Member (Bouldnor Formation, Solent Group) in the Hampshire Basin on the Isle of Wight, United Kingdom (Sanjuan and Martín-Closas, Reference Sanjuan and Martin-Closas2015b and references therein).
Conclusions
We studied the taxonomy, paleoecology, and paleobiogeography of a latest Paleocene charophyte flora in the South Gobi area of Xinjiang in China, which includes the taxa Peckichara torulosa var. varians, Lychnothmanus vectensis, and Gyrogona lemani capitata. The dispersal of this flora from Asia to Europe in the middle to late Eocene probably occurred before the retreat of the Turgai Strait in both the Tarim area and the Siberian Basin by the end of the late Eocene and before the “Grande Coupure” in Europe and the “Mongolian Remodelling” event in Asia during the Eocene–Oligocene transition. Waterbirds may have played an important role in facilitating this intercontinental dispersal. In comparison with European Eocene records, it appears that G. lemani capitata and Lychnothmanus aff. L. vectensis had wider paleoecological tolerances in the Paleocene of China, before their migration westward.
Acknowledgments
This work was supported by the National Natural Science Foundation of China (grant numbers 41625005, 41972018, 41730317, 41888101, 41988101, 41902020), the Strategic Priority Research Program of Chinese Academy of Sciences (grant numbers CAS XDB26030400, XDB26030300, XDB26010304, XDA20070203, XDA19050100), and the open project of State Key Laboratory of Paleobiology and Stratigraphy (grant number 193103). We thank G. Wang, R. Li, and Y. Wu for sorting the specimens from the screen-washing concentrates. Thanks to X. Jin for help with SEM imaging. We gratefully acknowledge C. Martín-Closas (University of Barcelona) and an anonymous reviewer for their careful work and thoughtful suggestions that have helped improve this paper substantially.