Introduction
At the landscape scale, agroecosystems are a mosaic of habitats (Evans et al., Reference Evans, Pocock, Brooks and Memmott2011; Pocock et al., Reference Pocock, Evans and Memmott2012; Evans et al., Reference Evans, Pocock and Memmott2013) within which there are cultivated and uncultivated compartments (Woodward & Bohan, Reference Woodward and Bohan2013; Derocles et al., Reference Derocles, Le Ralec, Besson, Maret, Walton, Evans and Plantegenest2014). The cultivated areas mainly include annual crops, which are harvested seasonally, leading to habitat instability (Rodriguez & Hawkins, Reference Rodriguez and Hawkins2000; Gurr et al., Reference Gurr, Wratten and Luna2003). In contrast, uncultivated habitats (e.g., field edges, hedges, and woods) are more stable and host a large diversity of plants (Benton et al., Reference Benton, Vickery and Wilson2003). The different characteristics of these two compartments influence the structure and distribution of communities they host in time and space as well as the interactions among organisms (Thompson, Reference Thompson1994; Begon et al., Reference Begon, Townsend and Harper2006). The exploitation of resources at different trophic levels depends on the degree of trophic specialization of species (Chaplin-Kramer et al., Reference Chaplin-Kramer, O'Rourke, Blitzer and Kremen2011). Generalist predators or parasitoids species can use a diversity of resources in the mosaic of crops and non-crop habitats. Specialist species, however, are only able to exploit a few patches of resources, dispersed in this mosaic (Tscharntke et al., Reference Tscharntke, Bommarco, Clough, Crist, Kleijn, Rand, Tylianakis, van Nouhuys and Vidal2007). Cultivated habitats are abundant but temporary resources for phytophagous insects and their associated natural enemies, while uncultivated habitats can act as refuges for these organisms because they are more stable environments. These habitats provide alternative prey for predators or hosts for parasitoids (Alhmedi et al., Reference Alhmedi, Haubruge, D'Hoedt and Francis2011; Rusch et al., Reference Rusch, Valantin-Morison, Roger-Estrade and Sarthou2012). Many studies have demonstrated the importance of semi-natural habitats and their alternative resources in agroecosystems in determining the abundance and diversity of pest natural enemies (Tscharntke et al., Reference Tscharntke, Bommarco, Clough, Crist, Kleijn, Rand, Tylianakis, van Nouhuys and Vidal2007; Ricci et al., Reference Ricci, Franck, Toubon, Bouvier, Sauphanor and Lavigne2009, Reference Ricci, Franck, Bouvier, Casado and Lavigne2011) and their efficiency for biological control (Langer & Hance, Reference Langer and Hance2004).
The ability to use alternative resources depends on the degree of specialization of the various species involved. Specialist species are more dependent on the availability of a particular prey or host than generalist species. Consequently, specialists may exploit resources in only one type of habitat (cultivated or uncultivated; Gagic et al., Reference Gagic, Petrović-Obradović, Fründ, Kavallieratos, Athanassiou, Starý and Tomanović2016) and are prone to local extinction when their main food disappears (Pyke et al., Reference Pyke, Pulliam and Charnov1977; Holt & Lawton, Reference Holt and Lawton1993; Devictor et al., Reference Devictor, Clavel, Julliard, Lavergne, Mouillot, Thuiller, Venail, Villeger and Mouquet2010). However, adaptation of specialist species to resources leads to improved performance (Devictor et al., Reference Devictor, Clavel, Julliard, Lavergne, Mouillot, Thuiller, Venail, Villeger and Mouquet2010) on these resources, which could lead to a greater efficiency in limiting crop pests. Nevertheless, in some studies, generalist species were demonstrated to be more effective biocontrol agents than specialists (Stiling & Cornelissen, Reference Stiling and Cornelissen2005). Species able to exploit at least a small variety of resources can be more efficient than highly specialized ones, as they are able to use resources in non-crop areas. This could ensure population conservation during crop-free periods and increase parasitism pressure when the pest colonizes the crop.
The sub-family Aphidiinae (Hymenoptera: Braconidae) includes about 400 species of aphid parasitoids (Godfray & Shimada, Reference Godfray and Shimada1999; Sanchis et al., Reference Sanchis, Michelena, Latorre, Quicke, Gardenfors and Belshaw2001). Most parasitize only one or two aphid species. However, some are able to parasitize more than a dozen aphid species (e.g., Aphidius ervi (Haliday, 1834), Aphidius matricariae (Haliday, 1834), Diaeretiella rapae (M'intosh, 1855), Ephedrus plagiator (Nees, 1811), Lysiphlebus fabarum (Marshall, 1896) or Praon volucre (Haliday, 1833) (Starý, Reference Starý2006)). These generalist species may be suitable candidates for regulating pest aphids in conservation biological control strategies as they are theoretically able to exploit both pest and non-pest species.
However, recent reports questioned the actual host range of generalist Aphidiinae. Several studies revealed the existence of cryptic species (i.e., morphologically indistinguishable but genetically different species) among the previously known generalists A. matricariae (Derocles et al., Reference Derocles, Plantegenest, Rasplus, Marie, Evans, Lunt and Le Ralec2016), Binodoxys communis (Haliday, 1833) (Desneux et al., Reference Desneux, Starý, Delebecque, Gariepy, Barta, Hoelmer and Heimpel2009), L. fabarum (Barahoei et al., Reference Barahoei, Madjdzadeh and Mehrparvar2011), and E. plagiator (Tomić et al., Reference Tomić, Tomanović, Kavallieratos, Starý, Athanassioud, Tomić and Lucic2005). Other studies revealed the existence of genetically differentiated populations exploiting different aphid host species, suggesting intraspecific host specialization. For example, Derocles et al. (Reference Derocles, Plantegenest, Rasplus, Marie, Evans, Lunt and Le Ralec2016) found genetic differentiation within generalist species, such as D. rapae and P. volucre. In parasitoid species, specialization reduces the number of exploited aphid species and can potentially lead to speciation (Ravigné, Reference Ravigné, Thomas, Lefevre and Raymond2010).
In this study, we assessed the extent of ecological specialization and reproductive isolation in one of the apparently most generalist Aphidiinae species, D. rapae in which genetic structuration was recently detected. For this, we tested the ability of several parasitoid strains to parasitize aphids from cultivated vs. uncultivated habitats as well as their reproductive compatibility. We chose three aphid species from cultivated plants. Two were from Brassicaceae, the cabbage aphid Brevicoryne brassicae (Linnaeus, 1758) and the green peach aphid Myzus persicae (Sulzer, 1776), frequently parasitized by D. rapae in western France. The other one was the grain aphid Sitobion avenae (Fabricius, 1775) from Poaceae, more rarely parasitized in this area. On uncultivated plants, we focused on Chenopodium album (Linnæus, 1753) (Chenopodiaceae). In agricultural areas, this plant is found both in fields and edges, throughout the year, and harbors three potential hosts aphid species Hayhurstia atriplicis (Linnaeus, 1761), Aphis fabae (Scopoli, 1763), and M. persicae (Starý, Reference Starý2006). Starý & González (Reference Starý and González1991) suggested that H. atriplicis could thus play a role as a reservoir for D. rapae in the absence of B. brassicae. In addition, Derocles et al. (Reference Derocles, Plantegenest, Rasplus, Marie, Evans, Lunt and Le Ralec2016) showed that there is genetic differentiation between parasitoids developing on B. brassicae or M. persicae and those parasitizing H. atriplicis. We therefore added H. atriplicis and A. fabae to the range of tested aphid species.
We tested the hypothesis that uncultivated plants of the genus Chenopodium could serve as reservoirs for D. rapae. The ability of D. rapae to develop on non-pest species in wild plants from semi-natural habitats could improve its efficiency in controlling B. brassicae the main pest of cultivated Brassicaceae, by promoting its early presence on Brassica crops (Le Guigo et al., Reference Le Guigo, Maingeneau and Le Corff2012a ). The efficiency of D. rapae to control B. brassicae was recently shown to depend on a quasi-synchronicity between aphid colonization of the plant and parasitoid arrival (Neuville et al., Reference Neuville, Le Ralec, Outreman and Jaloux2016). However, C. album could only act as a reservoir for D. rapae if there is no ecological specialization or reproductive isolation between individuals developing on Brassica and Chenopodium aphids. To test these hypotheses, we established four strains of D. rapae isolated from three different aphid host species and compared their performances on the selected aphid host species using host switching experiments. We also examined cross-breeding among parasitoid strains from different aphid host species but from the same geographical area, and compared it to cross-breeding among individuals from the same aphid host species but from different geographical areas.
Material and methods
Parasitoids
The aphid parasitoid D. rapae is reported to parasitize nearly 100 aphid species around the world (Singh & Singh, Reference Singh and Singh2015). Four strains of D. rapae were established from parasitized aphids of its main aphid host species (Němec & Starý, Reference Němec and Starý1984), collected in two geographic areas, western France and Portugal. Three strains were isolated from broccoli fields. Two (one from each locality) were from the cabbage aphid, B. brassicae, and one was from the green peach aphid, M. persicae, in western France. The last strain was isolated from the aphid H. atriplicis collected on C. album, in a semi-natural habitat (an herbaceous bank next to a cultivated field) in western France. The sampling locations are shown in table 1. For the three French strains, the maximum distance between the collection sites was approximately 100 km. Each D. rapae strain was reared in the laboratory on the aphid species and plant of collection.
Table 1. Diaeretiella rapae strains included in this study: name, collection aphid host and plant, collection location, collection year, and number of males and females for breeding.

Dr/Mp: D. rapae from M. persicae; Dr/Bb (Fr): D. rapae from B. brassicae (France); Dr/Bb (Por): D. rapae from B. brassicae (Portugal); Dr/Ha: D. rapae from H. atriplicis.
Aphids
Aphid strains used for parasitoid rearing and experiments were established from field-collected individuals or were part of a laboratory clone collection (table 2). Aphids were all maintained on their plant species of collection. Six aphid species were used in the experiments; we chose five species that D. rapae is known to parasitize in France and a sixth aphid species not established as part of the D. rapae host range. These included: B. brassicae, which is the main host of D. rapae and a Brassicaceae pest (Němec & Starý, Reference Němec and Starý1984; Wilson & Lambdin, Reference Wilson and Lambdin1987; Pike et al., Reference Pike, Starý, Miller, Allison, Graf, Boydston, Miller and Gillespsie1999); M. persicae and H. atriplicis from which the parasitoid D. rapae was collected in the field; and three other species, A. fabae, S. avenae, and Acyrthosiphon pisum (Harris, 1776). Aphis fabae and S. avenae are part of the theoretical host range of D. rapae, while A. pisum is not (except for a few records in South America, Singh & Singh, Reference Singh and Singh2015). B. brassicae, H. atriplicis, S. avenae, and A. pisum are specialist aphids, on Brassicacae, Chenopodiaceae, Poacae, and Fabacae, respectively. M. persicae and A. fabae are more generalist species (Blackman & Eastop, Reference Blackman and Eastop2006). For these two last species, individuals were collected from plants where another D. rapae host could be found. This was to test the hypothesis that the parasitoid could use different aphid host species on the same plant species. Thus, M. persicae like B. brassicae was collected on Brassicaceae, and A. fabae like H. atriplicis was collected on Chenopodiaceae (table 2).
Table 2. Aphid species included in this study: species name based on morphological identification, collection plant, collection location, and collection year.

Three clones of S. avenae and A. pisum were used because intraspecific variability in host suitability for aphidiinae parasitoids is known for both species (Le Ralec, unpublished data; Simon et al., Reference Simon, Baumann, Sunnucks, Hebert, Pierre, Le Gallic and Dedryver1999; Peccoud et al., Reference Peccoud, Ollivier, Plantegenest and Simon2009). As this variability is partially due to symbiotic bacteria (Hamiltonella defensa) in A. pisum (Dion et al., Reference Dion, Polin, Simon and Outreman2011), we chose three aposymbiotic clones. This was not necessary with B. brassicae populations in western France because they are composed of very few genotypes (Le Guigo et al., Reference Le Guigo, Rolier and Le Corff2012b ), and no protective symbiotic bacteria against parasitoids have ever been found in this species (Henry et al., Reference Henry, Maiden, Ferrari and Godfray2015).
Insect rearing
Aphids and parasitoids were reared under laboratory conditions, at a temperature of 19 ± 2.0 °C, 60 ± 10% relative humidity and a L16: D8 photoperiod in separate climatic chambers. B. brassicae and M. persicae were reared separately on broccoli plants (Brassica oleracea var. italica cv Marathon). The three S. avenae clones were reared separately on wheat (Triticum aestivum cv. Boston) and the three A. pisum clones on alfalfa plants (Medicago sativa). Both H. atriplicis and A. fabae were reared on goosefoot plants (C. album) obtained from seeds collected in the field.
Host suitability assessments
The ability of each D. rapae strain to parasitize the six aphid species was evaluated. The non-host aphid species A. pisum was tested to ensure parasitism events did not result from experimental non-choice conditions, regardless of the aphid species. Newly emerged females of each of the four parasitoid strains were fed with honey and water for 24–48 h, and then placed in isolation with a single male of the same strain for 24 h. Mated females had no contact with an aphid prior to the test. Each parasitoid female was tested only once. For each aphid species and clone, ten third instar nymphs were placed on a young plant of the species they had been reared on. After infestation, plants were covered with a Cellophane® bag (29 cm × 15 cm) for 1 h to allow the aphids to settle. They were then exposed to a single D. rapae female for 24 h. The female was then removed and the aphids were kept in climatic-controlled chambers for 20 days. For each aphid species, clone and parasitoid strain, ten replicates were carried out. After 10 days, mummified aphids were counted. Experiments were carried out in two distinct sessions (with 10 days between each session). The number, sex ratio (number of females/total number of individuals), and development time of the parasitoids that emerged from the mummies were recorded.
Reproductive compatibility
To assess reproductive compatibility among D. rapae strains, originating from various hosts, we performed crosses among the four parasitoid strains. Because Aphidiinae are haplodiploid, the absence of females in the progeny indicates reproductive incompatibility, as 100% male progeny reflects the absence of oocyte fertilization. We used this feature to detect possible reproductive isolation between the strains. To avoid previous mating, mummies were isolated until emergence. Males and females were isolated for 24 h after emergence and supplied with water and honey, and then couples were randomly paired. Ten females from each strain were allowed to mate with ten males of the four possible strains. Five females were proposed to oviposit on aphids belonging to the species of female rearing and five of them on aphids belonging to the species of male rearing. Each mated couple was used only once. We used the same experimental design as above (host suitability experiment) to obtain progeny and check the sex ratio as the proportion of females. The number of mummies, as well as the number and sex of emerged adults were recorded.
Statistical analysis
First, the influence of the aphid species (aphid of collection (rearing) or not), the D. rapae strain and the session on the parasitism rate was assessed using a generalized linear model (GLM) assuming a binomial error and a logit link function. Pairwise comparisons between each strain–aphid pair were performed with the function ‘esticon’ using the ‘doBy’ package (Højsgaard, Reference Højsgaard2004). The effect on the parasitism rate of the aphid species in which the female and male in each cross-breeding have developed was tested with the same method. Second, we determined the effects of aphid host species (aphid of collection (rearing) or not), D. rapae strains and session on the development time using an analysis of variance (ANOVA) followed by Tukey's tests. Finally, the effects of aphid host species (aphid of collection (rearing) or not) and D. rapae strains on the sex ratio in both experiments were examined using ANOVA followed by Tukey's tests. To ensure the accuracy of our model, we verified the normality of the distribution of residuals. All analyses were carried out with R version 3.1.1 (R Development Core team, 2013).
Results
Assessment of host suitability
The four strains differed in the range of aphid species they parasitized and in the parasitism rates obtained on these species (fig. 1). No mummies were obtained on S. avenae and A. pisum; these two species were removed from the statistical analysis. Parasitoid strains (GLM: χ2 = 14.162, df = 3, P = 0.0026), aphid species (GLM: χ2 = 284.571, df = 3, P < 0.001), and the interactions between parasitoid strains and aphid species (GLM: χ2 = 248.991, df = 9, P < 0.001) significantly influenced the parasitism rate. Higher parasitism rates were obtained on the rearing aphid host species of each strain compared with the other aphid species (GLM: χ2 = 247.6, df = 1, P < 0.001). Session had no significant effect (GLM: χ2 = 0.307, df = 1, P = 0.58) on the parasitism rate. Overall, mummies were produced on only four aphid species, and the number of aphid species with at least one mummy varied from two to four depending on the parasitoid strain (fig. 1). All the strains parasitized B. brassicae and M. persicae. Two strains parasitized H. atriplicis and one strain parasitized A. fabae. The D. rapae strain reared on H. atriplicis produced offspring on four aphid species. The three other strains only successfully parasitized B. brassicae and M. persicae, although a single mummy was obtained from the Portuguese strain on H. atriplicis. The strain reared on H. atriplicis produced significantly less progeny than the three other strains reared on B. brassicae and M. persicae (fig. 2).

Fig. 1. Total number of mummies produced per aphid species by the various Diaeretiella rapae strains. (a) Average number of mummies per female with standard deviation; the sex ratio is expressed as the female ratio and the mean development time in days with standard deviation for each pair of parasitoid strains and aphid species (b). Dr/Mp: D. rapae from Myzus persicae; Dr/Bb(Fr): D. rapae from Brevicoryne brassicae (France); Dr/Bb(Por): D. rapae from B. brassicae (Portugal); Dr/Ha: D. rapae from Hayhurstia atriplicis. The data were analyzed with a generalized linear model for parasitism rate and ANOVA for development time with pairwise comparisons with ‘esticon’ and Tukey's tests as post hoc tests, respectively. Results from the three clones of A cythosiphon pisum and S itobion avenae were pooled as no mummies were obtained. Significant differences are indicated with different letters (P < 0.05) and with asterisks (***P < 0.001).
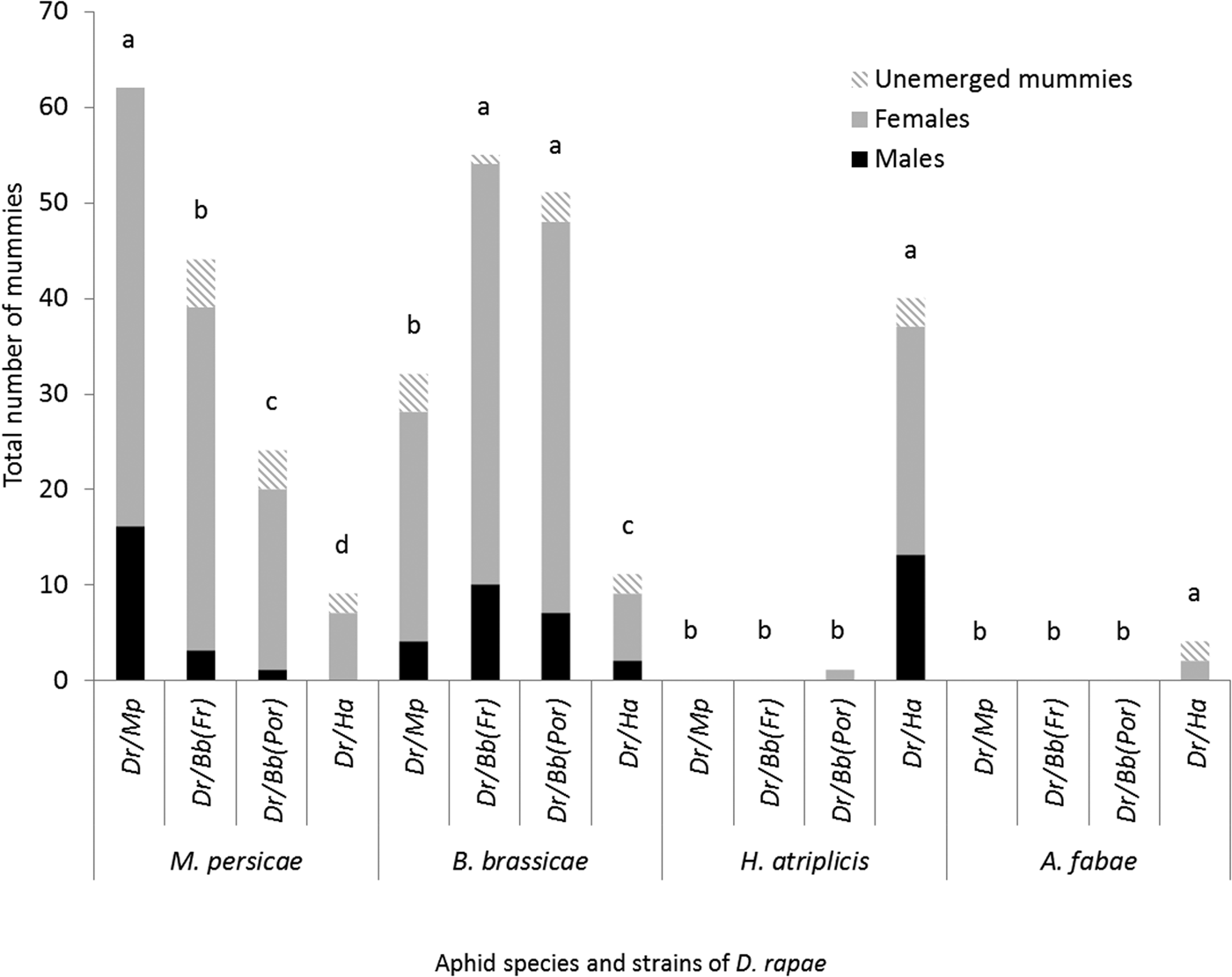
Fig. 2. Total number of adult female and male offspring, as well as unemerged mummies, produced per aphid species by the various Diaeretiella rapae strains. The data were analyzed with a generalized linear model with pairwise comparisons with an ‘esticon’ post hoc test. Different letters show significant differences (P < 0.05).
The development time between egg laying to adult emergence differed significantly depending on the parasitoid strains (ANOVA: F(3, 287) = 27.85, P < 0.001), aphid species (ANOVA: F(3, 287) = 10.51, P < 0.001), and the interaction between these factors (ANOVA: F(4, 287) = 3.39, P = 0.009) (fig. 1). The strain reared on H. atriplicis required 1 or 2 more days to complete its development compared with the strains reared on M. persicae and B. brassicae (fig. 1). Finally, for all strains, the progeny sex ratio ranged from 0.7 to 1 (fig. 1), and neither the aphid species (ANOVA: F(3, 63) = 0.95, P = 0.42) or the strains (ANOVA: F(3, 63) = 2.14, P = 0.1) had a significant effect.
Reproductive compatibility
The overall sex ratio of the progeny was approximately 0.8 females, except for crosses involving individuals reared on H. atriplicis mated with a partner from another strain. In that case, only males were obtained (fig. 3). The parasitism rates of the four D. rapae strains on the different aphid species observed in this experiment were similar to those obtained in the previous experiment (host suitability assessment) (fig. 3). In all cross-breedings, the parasitism rate was significantly higher on the female's rearing aphid host species compared with the other aphid species (GLM: χ2 = 209.71, df = 1, P < 0.001; fig. 3). Mummies were only produced on H. atriplicis when the female belonged to the H. atriplicis strain.
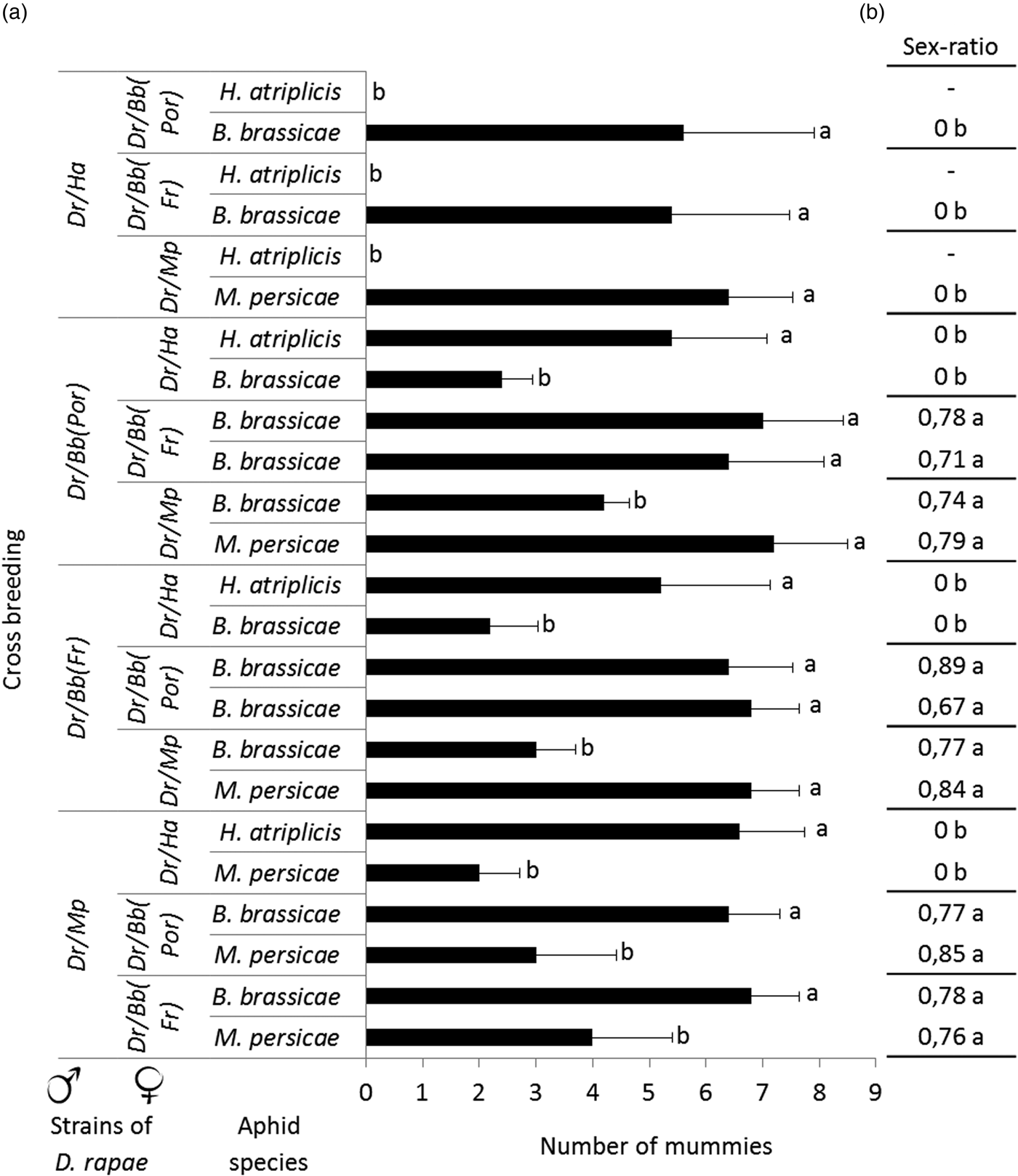
Fig. 3. Average numbers of mummies produced (with standard deviation) (a) and offspring sex ratio (as the female ratio) (b) for each aphid species tested and for all types of cross-breeding. Dr/Mp: Diaeretiella rapae from M yzus persicae; Dr/Bb: D. rapae from B revicoryne brassicae; Dr/Bb (Por): D. rapae from B. brassicae (Portugal); Dr/Ha: D. rapae from H ayhurstia atriplicis. The data were analyzed with a generalized linear model for parasitism rate with pairwise comparisons with an ‘esticon’ post hoc test. To analyse the sex ratio, we used ANOVA with Tukey's tests; different letters indicate significant differences (P < 0.05).
Discussion
The aphid parasitoid D. rapae is known to parasitize 98 aphid species (Singh & Singh, Reference Singh and Singh2015). However, previous studies found local variations in its host range (Le Ralec et al., Reference Le Ralec, Ribule, Barragan and Outreman2011). In the present study, we investigated the level of host (aphid species) specialization of four strains reared from three aphid species and two geographical origins. An increased level of specialization was observed in the three D. rapae strains collected on the aphid species, which infest crops (B. brassicae from two different areas and M. persicae); whereas the D. rapae strain isolated from the non-pest aphid H. atriplicis was able to parasitize a wider range of aphid species. In addition, this strain appeared reproductively isolated from the three others. These results suggest that specialized cryptic species exist among populations of this presumed generalist parasitoid. The role of H. atriplicis in maintaining D. rapae populations when pest aphids are absent is therefore questionable.
The four strains of D. rapae studied here had different host ranges among the tested aphid species, but also produced varying numbers of offspring on each suitable aphid species. First, the three strains from B. brassicae and M. persicae only parasitized these two aphid host species, regardless of their geographical origin. Next, the strain from H. atriplicis parasitized four of the six aphid species tested. In addition to its host of origin, the strain from H. atriplicis successfully developed on B. brassicae, M. persicae, and A. fabae, the latter species was collected on C. album as well as H. atriplicis. B. brassicae and M. persicae are very common aphids in European agroecosystems (Blackman & Eastop, Reference Blackman and Eastop2000), and constitute an abundant resource during a short period of time for D. rapae on cultivated Brassicaceae (e.g., oil seed rape, cabbage, turnip). In contrast, H. atriplicis is a scarce resource for a parasitoid because it is only present for three months (August to October), on wild Chenopodiaceae (Blackman & Eastop, Reference Blackman and Eastop2000), and in small abundance in agroecosystem. We can hypothesize that it is highly risky for a parasitoid species to specialize on a scarce resource. However, the ability to parasitize other aphid species on the same plant, such as A. fabae or M. persicae, and also aphid species exploiting plants of other families (e.g., B. brassicae and M. persicae on Brassicaceae), could limit the risks associated with specialization. The parasitic success of the most generalist strain (from H. atriplicis) was lower than the parasitic success of the more specialized strains, on the shared aphid host species B. brassicae and M. persicae. This reduction in parasitism efficacy is expected as a result of a trade-off between the ability to exploit numerous aphid species and parasitism efficacy (Devictor et al., Reference Devictor, Clavel, Julliard, Lavergne, Mouillot, Thuiller, Venail, Villeger and Mouquet2010; Le Ralec et al., Reference Le Ralec, Anselme, Outreman, Poirié, Van Baaren, Le Lann and Jacques2010; Gagic et al., Reference Gagic, Petrović-Obradović, Fründ, Kavallieratos, Athanassiou, Starý and Tomanović2016).
The grain aphid S. avenae was never parasitized, even though this species was previously reported to belong to the D. rapae host range (Pike et al., Reference Pike, Starý, Miller, Allison, Graf, Boydston, Miller and Gillespsie1999; Singh & Singh, Reference Singh and Singh2015). Such an unsuitability of theoretical D. rapae hosts was previously observed for other cereal aphids (Antolin et al., Reference Antolin, Bjorksten and Vaughn2006; Le Ralec et al., Reference Le Ralec, Ribule, Barragan and Outreman2011). Unfortunately, we did not find any D. rapae individuals from cereal aphids to use as an experimental strain. Less surprisingly, no progeny were obtained on the pea aphid A. pisum, used as a non-host control in this study, even in the experimental non-choice conditions.
The highest parasitic success was always obtained on the aphid host species of collection, which was also the rearing host. This is not surprising as strains collected on a given host are expected to be adapted to this aphid species. This variation in performance also indicates some degree of host specialization of the populations in the field (Henry et al., Reference Henry, Roitberg and Gillespie2008). Differences in performance might also result from parasitoid strains being continuously maintained on their aphid species of collection since sampling. Rearing on a single aphid host species was shown to have a positive impact on parasitoid success (Vafaie et al., Reference Vafaie, Fitzpatrick and Cory2013), enhance host recognition (Wickremasinghe & Van Emden, Reference Wickremasinghe and Van Emden1992; Storeck et al., Reference Storeck, Poppy, Van Emden and Powell2000), and induce preference in host choice (Van Emden et al., Reference Van Emden, Storeck, Douloumpaka, Eleftherianos, Poppy and Powell2008).
The observed differences in the set of aphid host species successfully parasitized by the four D. rapae strains are consistent with the observations of Derocles et al. (Reference Derocles, Plantegenest, Rasplus, Marie, Evans, Lunt and Le Ralec2016). Indeed, using molecular phylogenetic analysis, Derocles et al. (Reference Derocles, Plantegenest, Rasplus, Marie, Evans, Lunt and Le Ralec2016) showed a clear genetic differentiation between D. rapae populations collected on B. brassicae and M. persicae and populations sampled on H. atriplicis from the same geographical area. Our results suggest that this molecular variation between D. rapae populations from different aphid species actually reflects host specialization. In addition, the strains from B. brassicae and M. persicae appeared to be reproductively incompatible with the strain from H. atriplicis. While distant populations from France isolated from B. brassicae or M. persicae and Portugal from B. brassicae were able to successfully interbreed, the French population reared on H. atriplicis did not produce female offspring when they mated with another strain. Our data reveal a clear convergence between the observed genetic differentiation, host specialization, and reproductive isolation in the populations of the generalist parasitoid D. rapae included in this study, suggesting the existence of cryptic species among D. rapae. Such cryptic species or specialized biotypes are known in other Aphidiinae. In several studies, morphometric analysis revealed specialized biotypes (Tomić et al., Reference Tomić, Tomanović, Kavallieratos, Starý, Athanassioud, Tomić and Lucic2005; Žikić et al., Reference Žikić, Tomanović, Ivanovic, Kavallieratos, Starý, Stanisavljevic and Rakhshani2009; Barahoei et al., Reference Barahoei, Madjdzadeh and Mehrparvar2011; Tomanović et al., Reference Tomanović, Petrović, Mitrović, Kavallieratos, Starý, Rakhshani, Rakhshanipour, Popović, Shukshuk and Ivanović2014). However, in our case, a morphological re-examination of D. rapae individuals based on the main morphological characteristics usually used to identify Aphidiinae (maxillary palps, number of antennal segments, size, shape, and rib wings and the anterolateral area of the petiole; Kavallieratos et al., Reference Kavallieratos, Lykouressis, Sarlis, Stathas, Segovia and Athanassiou2001; Tomanović et al., Reference Tomanović, Kavallieratos, Starý, Athanassiou, Žikić, Petrović-Obradović and Sarlis2003; Kavallieratos et al., Reference Kavallieratos, Tomanović, Starý, Athanassiou, Fasseas, Petrović, Niketić, Stanisavljević and Veroniki2005; Tomanović et al., Reference Tomanović, Rakhshani, Starý, Kavallieratos, Stanisavljević, Žikić and Athanassiou2007) failed to find any evidence of differentiation between strains.
Derocles et al. (Reference Derocles, Plantegenest, Rasplus, Marie, Evans, Lunt and Le Ralec2016) could not conclude in favor of two different species among D. rapae on the sole basis of molecular variations. Indeed, the analytical approach they applied, which was based on a combination of maximum likelihood trees and the species delimitation method called Bayesian Poisson tree processes model (Zhang et al., Reference Zhang, Kapli and Stamatakis2013), did not separate the two genetic sub-groups (D. rapae populations from H. atriplicis on one side and populations from other species on the other) into distinct species. However, our complementary findings showing that morphologically indistinguishable (based on our morphological reexamination) host-specialized strains are reproductively isolated strongly suggest that there are at least two cryptic D. rapae species. Desneux et al. (Reference Desneux, Starý, Delebecque, Gariepy, Barta, Hoelmer and Heimpel2009) reported similar results and described a new species, Binodoxys koreanus, from two populations previously identified as B. communis. Consequently, our results suggest that a new Diaeretiella species could potentially be described. The evolutionary process leading to reproductive isolation of individuals from the same ancestral species and resulting in two species (Ravigné, Reference Ravigné, Thomas, Lefevre and Raymond2010) involves physical or biological reproductive barriers. In the case of B. communis and B. koreanus (Desneux et al., Reference Desneux, Starý, Delebecque, Gariepy, Barta, Hoelmer and Heimpel2009), the two species originated from distant countries and their recent divergence could be explained by geographical isolation. In the present study, the incompatible strains were collected in the same geographical area. Sympatric speciation, i.e., the emergence of ‘new species’ without geographical isolation (Futuyma, Reference Futuyma and Tilmon2008; Wiley & Lieberman, Reference Wiley and Lieberman2011) can result from micro-habitat differentiation, which leads to a reduction in gene flow between specialized populations.
Most aphid species are specialized on a few plant species or families (Ferrari et al., Reference Ferrari, Via and Godfray2008); thus, we hypothesize that habitat choice could be the primary driver of parasitoid specialization (Le Ralec et al., Reference Le Ralec, Anselme, Outreman, Poirié, Van Baaren, Le Lann and Jacques2010). Indeed, plants emit secondary compounds during aphid attack that attract various Aphidiinae parasitoids (Guerrieri et al., Reference Guerrieri, Pennacchio and Tremblay1993; Funk et al., Reference Funk, Filchak and Feder2002; Figueroa et al., Reference Figueroa, Simon, Le Gallic, Prunier-Leterme, Briones, Dedryver and Niemeyer2004; Toneatto et al., Reference Toneatto, Nielsen, Ørgaard and Hauser2010) and thus affect the aphid host species selection behavior of the parasitoid (Rehman & Powell, Reference Rehman and Powell2010). Similarly, after pest attack, Brassicaceae emit secondary compounds that attract D. rapae (Blande et al., Reference Blande, Pickett and Poppy2007; Pope et al., Reference Pope, Kissen, Grant, Pickett, Rossiter and Powell2008). We propose that the secondary compounds Chenopodiaceae emit when infested with aphids are different. These differences in compounds potentially lead to specialization among sections of the population for the specific recognition of these compounds.
According to our results, the presumed generalist species D. rapae could thus comprise at least two biotypes or even distinct species, one developing on aphid species infesting Brassicaceae and one on aphid species on Chenopodiaceae. The synonymy of the species name D. rapae with names referring to Chenopodiaceae, such as Diaeretiella chenopodii or Diaeretiella chenopodiaphidis (Singh & Singh, Reference Singh and Singh2015) should therefore be re-examined. The theoretical D. rapae host range includes almost nearly 100 aphid host species and similar cases of specialization in this parasitoid have been shown for other aphid hosts (see Antolin et al., Reference Antolin, Bjorksten and Vaughn2006; Le Ralec et al., Reference Le Ralec, Ribule, Barragan and Outreman2011). The potential number of specialized sub-taxa or cryptic species could then be greater. This study only included four populations with probably low genetic diversity due to the low number of individuals used to constitute the strains. To fully elucidate the taxonomic status of D. rapae, we need to conduct host switching and cross-breeding experiments on a larger number of populations originating from a wider range of aphid species, plants, and countries.
Finally, according to our findings, the role of H. atriplicis as a reservoir for D. rapae as proposed by Němec & Starý (Reference Němec and Starý1984) and Starý & González (Reference Starý and González1991) is questionable. We found an asymmetry in host range between specialist strains from B. brassicae and M. persicae and a more generalist strain from H. atriplicis, with the former strains being unable to develop on H. atriplicis, a situation not tested by Starý & González (Reference Starý and González1991). This confirms the scarcity of parasitoid exchanges between cultivated and uncultivated compartments (Derocles et al., Reference Derocles, Le Ralec, Besson, Maret, Walton, Evans and Plantegenest2014). Promoting non-pest aphids on non-crop plants as a parasitoid reservoir may not be a suitable way of enhancing natural pest control by D. rapae in conservation biological control. Wild Brassicaceae species in the vicinity of the field are more likely to be the actual reservoir of the corresponding D. rapae sub-taxa in the studied area, as they harbor the pest aphid species B. brassicae or M. persicae (Le Guigo et al., Reference Le Guigo, Maingeneau and Le Corff2012a , Reference Le Guigo, Rolier and Le Corff b ). However, wild Brassicaceae in uncultivated compartments in agroecosystems could also act as a pest reservoir.
Our study is consistent with several recent studies, which demonstrated that populations of Aphidiinae species generally considered as generalists may potentially include specialized sub-groups or even cryptic species, as seems to be the case for D. rapae. This has to be fully considered in ecological studies on interaction networks between plants, aphids, and parasitoids to avoid misinterpretation of resource sharing (Gagic et al., Reference Gagic, Petrović-Obradović, Fründ, Kavallieratos, Athanassiou, Starý and Tomanović2016) and the structure of ecological networks (Derocles et al., Reference Derocles, Evans, Nichols, Evans and Lunt2015). Furthermore, the importance of aphid traits in the evolution of host specificity in Aphidiinae is often highlighted (Raymond et al., Reference Raymond, Plantegenest, Gagic, Navasse and Lavandero2016). Nevertheless, the plant taxa supporting the aphid hosts could also be a key factor driving parasitoid specialization.
Acknowledgements
The authors thank Bernard CHAUBET for technical assistance for maintained insect rearing. This work was supported by the French ‘Ministère de l'Enseignement Supérieur et de la Recherche’ and was founded by the French Research National Agency (PEERLESS project, ANR-12-AGRO-0006).