INTRODUCTION
The Kudoidae Meglitsch, 1960 is a family of myxosporean, spore-forming parasites that infect a large range of fishes. They are most widely known for their impacts on meat quality in aquaculture caused by post-mortem myoliquefaction. Myxosporeans are morphologically simple organisms that have few distinctive features on which to characterize species. Families within the Multivalvulida Shulman, 1959 were traditionally distinguished by the number of polar capsules and spore valves. However, Whipps et al. (Reference Whipps, Grossel, Adlard, Yokoyama, Bryant, Munday and Kent2004) found that phylogenetic analysis of 18S and 28S rDNA of myxosporeans within the Multivalvulida showed that clades were not consistent in the number of spore valves. The analysis found that species of the families Pentacapsulidae Naidenova & Zaika, 1970, Hexacapsulidae Shulman, 1959 and Septemcapsulidae Hsieh & Chen, Reference Hsieh and Chen1984 all fell within the Kudoidae. In order to maintain monophyly of Kudoa Meglitsch, 1947, they proposed that the related families found within the Kudoa clade should be synonymized with Kudoidae and that the diagnosis of the genus Kudoa should be amended to accommodate myxosporeans possessing 4 or more polar capsules. Presently there are only 2 genetically monophyletic families in the Multivalvulida, Trilosporidae Shulman 1959 with 3 genera, Trilospora Noble, 1939, Trilosporoides Køie, 2005, and Unicapsula Davis, 1924, and the Kudoidae with a single genus, Kudoa.
As a result of these taxonomic changes, Kudoa contains approximately 69 species reported globally from a broad range of fish families and tissue sites. At present there is no real understanding of the mechanisms that have underpinned this distribution. The goal of this study is to explore factors that may correlate with patterns of genetic relatedness. In so doing we propose 2 new species of Kudoa, K. chaetodoni n. sp. and K. lethrini n. sp. and characterize a third, Kudoa sp. from Sillago ciliata (Fig. 1), all isolated from the brain of their hosts. At this stage, we are not formally proposing Kudoa sp. as new because the 18S rDNA sequence data from this species has 100% homology with another already characterized species, Kudoa yasunagaiHsieh & Chen, Reference Hsieh and Chen1984, despite significant morphological differences.

Fig. 1. Stylized diagrams of new species in apical and lateral view. (A and B) Kudoa chaetodoni n. sp.; (C and D) Kudoa lethrini n. sp.; (E and F) Kudoa sp. from Sillago ciliata. Scale bar=10 μm.
Recognizing patterns of morphological and biological characters that match molecular relationships will increase our understanding of the biology of these organisms and make identification of species, and control strategies (if warranted), more practical than relying solely on molecular data. Tissue tropism, geographical distribution, and host-specificity are evaluated in this study to determine whether a combination of these biological characteristics together with morphology can characterize the clades inferred from molecular phylogenetic analysis.
MATERIALS AND METHODS
Collection and dissection
Teleost fish were collected from 3 locations along the Queensland coastline of Australia: North Stradbroke Island, Heron Island, and Lizard Island. Fish were collected either by speargun, seine net, or by line fishing then examined using routine necropsy procedures for myxosporean parasites.
Fish were euthanized by immersion in clove oil or by pithing. To obtain brain tissue for examination, a cut was made along the dorsal surface of the mid-sagittal section starting from the premaxillary region to the end of the cranium. The cranium was prized open and the brain removed. A subsample (approximately 3 mm3) of brain tissue was placed on a glass microscope slide with a drop of saline, macerated with scalpel blades and covered with a glass cover-slip. Subsamples of gut wall cysts were also examined. When the tissue was found to contain myxosporean spores by observation using light microscopy at 400× magnification, remaining tissue was preserved by freezing (for morphological analysis), in 90% ethanol (for DNA extraction), and in 10% neutral buffered formalin (for histology). Where material was limited, priority went to frozen samples from which both morphological information and DNA could be recovered.
Histology
Tissue samples preserved in 10% formalin were sectioned using standard histological methods. Alternating slides were stained either with haematoxylin and eosin (H & E) or Giemsa and eosin.
The slides were examined using compound light microscopy at 100×, 200×, and 400× magnification. Digital photographs of myxosporean spores were taken at all magnifications using a Panasonic Wv-CP610 CCD camera attached through a capture device to Snappy Video Snapshot (Play Inc.) image capture software.
Voucher specimens of Kudoa ciliatae (Giemsa and Eosin and H & E-stained histological sections) were deposited to the Queensland Museum, South Brisbane, Australia with Accession numbers G464887–G464894.
Morphological analysis of brain myxosporeans
Morphological measurements of spores from fresh and frozen material followed the guidelines proposed by Lom and Arthur (Reference Lom and Arthur1989) for species descriptions of Myxosporea. Additional measurements were required to fully characterize spores, including spores with an uneven number of spore valves (see Fig. 2). Where a significant number of spores showed plasticity in valve number, measurements were made for each morphotype present. Length (lateral) measurements were taken from all spores present since valve number could not be determined from this elevation. A minimum of 30 measurements of each character was taken from digital images of spores at 400× magnification calibrated against a stage micrometer slide. An additional 30 measurements were taken from formalin-preserved spores of the Kudoa sp. from Sillago ciliata and used for comparison with morphometrics provided by Hsieh and Chen (Reference Hsieh and Chen1984) for Kudoa yasunagai. The ratio of the different morphotypes in each sample was noted.

Fig. 2. Schematic diagram of spore measurements. (A) Apical view of spore with uneven number of valves: W, width; T, thickness; SR, suture radius. (B) Lateral view of spore with lateral apical extensions: L, length; PCL, polar capsule length; PCW, polar capsule width; WH, wing height. (C) Valve measurements: VW, valve width; VB, valve breadth; APCL, apical polar capsule length.
18S rDNA sequencing
DNA of Kudoa spp. was extracted from 5 fish host tissues: Chaetodon unimaculatus Bloch, 1787 brain, Gymnocranius audleyi Ogilby, 1916 brain, Lethrinus harak Forsskål, 1775 brain, Sillago ciliata Cuvier, 1829 and S. maculata Quoy & Caimard, 1824 brain, and S. ciliata gut wall cyst. The gut wall cyst contained spores identified as Kudoa ciliatae and was included for sequencing because the available 18S rDNA sequence on GenBank was much shorter (872 base pairs) than the 1580 average length of 18S rDNA from other myxosporean species and thus would not allow robust sequence analyses.
DNA was extracted from 2 mm3 sections of tissues using QIAgen DNeasy Kit (QIAGEN Inc., Valencia, California) as per manufacturer's instructions. Myxosporean 18S ribosomal DNA (rDNA) was amplified using PCR primers specific to 18S rDNA sequences conserved among members of the Order Multivalvulida and universal primers (Table 1). PCR fragments were amplified using primer combinations: 18e-Kud1r and Kud6F-18g. Reaction mixtures (25 μl) were made up of 15·9 μl of autoclaved Millipore water, 2·5 μl of 10× Hotmaster Taq Buffer (Eppendorf, Hamburg, Germany), 2·0 μl of 10 mm dNTPs, 1·25 μl of 10 μm forward primer, 1·25 μl of 10 μm reverse primer, 0·1 μl of 5U Hotmaster Taq (Eppendorf) and 2 μl of template DNA (undetermined concentration as contains both host and myxosporean DNA). PCR reactions were performed in a cp2-01 thermocycler (Corbett Research, Australia) with an initial denaturation at 94°C for 2 min, followed by 35 cycles of denaturation at 94°C for 20 sec, annealing at 50°C for 20 sec and extension at 65°C for 60 sec; with a final extension at 65°C for 10 min.
Table 1. Primer sequences and positions used to amplify/sequence 18S ribosomal DNA

* Relative to 18e.
Twenty μl of each of the PCR products were isolated on a 1·4% agarose TA gel stained with 0·2 μg/ml ethidium bromide run at 70 volts for 1 h.
Amplified PCR products were cut from the gel and purified using the Perfectprep® Gel Cleanup Kit (Eppendorf).
Sequencing reactions were performed in both directions following standard sequencing protocol for ABI BigDye® Terminator (Applied Biosystems) as described by Gunter et al. (Reference Gunter, Whipps, Cribb and Adlard2006).
Additional sequencing primers (see Table 1) were designed for this study based on conserved regions of already acquired sequence data using Primer3 (Rozen and Skalersky, Reference Rozen and Skalersky2000).
Phylogenetic analysis
DNA sequence information was edited using BioEdit (Hall, Reference Hall1999) and aligned with other Kudoa 18S sequences available from Genbank (see Table 2) using ClustalW (Thompson et al. Reference Thompson, Higgins and Gibson1994). The alignment was then checked and adjusted by eye.
Table 2. Species isolates used for 18S rDNA sequence analysis

Unicapsula sp., a multivalvulidan in the family Trilosporidae was used as an outgroup as it represents the closest known relative external to the Kudoidae.
Neighbour joining, parsimony, and maximum likelihood analyses were conducted using PAUP* 4.0b10 (Swofford, Reference Swofford2002) and Bayesian analysis conducted using Mr Bayes 3.0B4 (Heulsenbeck and Ronquist, Reference Heulsenbeck and Ronquist2001). Neighbour-joining and parsimony analyses were given bootstrap confidence values based on 1000 replicates. Parsimony analysis employed a heuristic search with 50 repetitions of random sequence addition and tree bisection and reconnection branch swapping.
Optimum evolutionary models were used for maximum likelihood analyses as determined by Modeltest 3.7 (Posada and Crandall, Reference Posada and Crandall1998). Two models were utilized: GTR+I+G as determined by Akaike information criterion analyses of the sequence data and (TrN+I+G) selected by hierarchical likelihood ratio tests.
Bayesian analysis was conducted with 2 million a generations of Markov chain Monte Carlo analysis, a set at 4 simultaneous chains with a burnin of 3000 trees and saving current trees to file every 100 generations.
RESULTS
Characterization of brain myxosporean species
Phylum Myxozoa
Class Myxosporea
Order Multivalvulida
Family Kudoidae Meglitsch, 1960
Genus Kudoa Meglitsch, 1947
- Type host:
Chaetodon unimaculatus Bloch, 1787, Teardrop butterflyfish (Teleostei, Chaetodontidae) adult.
- Location in the host:
Brain. Pseudocysts sometimes visible as amorphous areas (up to 1 mm in diameter) that were paler than surrounding brain tissue.
- Type locality:
Turtle Beach (14° 39′S; 145° 27′E), Lizard Island, Great Barrier Reef, Queensland, Australia.
- Type Material:
Hapantotype G464863 (Giemsa and eosin-stained histological section) deposited in the Queensland Museum, South Brisbane, Australia.
- Etymology:
specific name refers to the genus of host fish in which it was found.
- Prevalence:
One of 7 (1 of 4, Lizard Island; none of 3 from North Wistari Reef, Capricorn-Bunker Group).
Description
Spores. (Figs 1A, B, 3A–D). Spores stellate in apical view, radiating from centre with 7–9 equal shell valves and polar capsules (in hapantotype, 8 SV spores slightly dominate 9 SV spores (ratio of 51·3:47·4) and 1·3% of spores have 7 SV), pyramidal in lateral view. Suture lines between valves not visible; shell distal margins tapering smoothly to point. Polar capsules pyriform, in anterior portion of shell valve and occupy approximately 62% of total length of shell valve in apical view. Spore measurements are shown in Table 3.
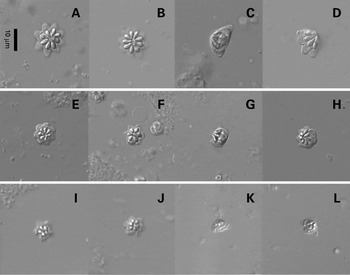
Fig. 3. Phase-contrast micrographs of fresh spore preparations. Kudoa chaetodoni n. sp. (A) 8-spore valve morphotype apical view. (B) 9-spore valve morphotype apical view. (C) Side view. (D) Oblique view. Kudoa lethrini n. sp. (E) 7-spore valve morphotype apical view. (F) 6 spore valve morphotype. (G) Side view. (H) Oblique view. Kudoa sp. I=5 spore valve morphotype apical view. (J) 6-spore valve morphotype apical view, K=side view, L=oblique view.
Table 3. Spore measurements in μm (with standard deviation) of myxosporeans isolated from brain tissue
(SV=spore valve; PCL=polar capsule length; PCW=polar capsule width; n/a=measurement not appropriate for that morphotype. See Fig. 2 for measurement schematics.)

- Type host:
Gymnocranius audleyi Ogilby, 1916, Collared large-eye bream (Teleostei, Lethrinidae) adult.
- Other host:
Lethrinus harak Forsskål, 1775, Thumbprint emperor (Teleostei, Lethrinidae) adult.
- Location in the host:
Brain
- Type locality:
North Wistari Reef (23° 27′S; 151° 55′E), Capricorn-Bunker Group, Great Barrier Reef, Queensland, Australia.
- Other localities:
Lizard Island (14° 40′ S; 145° 27′ E), Great Barrier Reef, Queensland, Australia.
- Type material:
Hapantotype G464864 (Giemsa and eosin-stained histological section); parahapantotypes G464865–G464866 (Giemsa and eosin-stained histological sections) and G464867–464870 (H&E-stained histological sections) deposited in the Queensland Museum, South Brisbane, Australia.
- Etymology:
specific name refers to the family of host fish in which it was found.
- Prevalence:
Gymnocranius audleyi – 4 of 7 from North Wistari Reef, Capricorn-Bunker Group; Lethrinus harak – 3 of 6 from Lizard Island.
Description
Spores. (Fig. 1C, D, 3E–H). Spores stellate in apical view, radiating from centre with 7 equal shell valves (95·1:4·9 ratio of spores with 7 shell valves to those with 6 shell valves), cup shape in lateral view. Suture lines between valves not visible; shell distal margins rounded at base and lateral apical extensions taper smoothly to point in flower petal-like form. Polar capsules pyriform, in anterior portion of shell valve and occupy approximately 55% of total length of shell valve in apical view. Spore measurements are shown in Table 3.
- Hosts:
Sillago ciliata Cuvier, 1829, Sand whiting (Teleostei, Sillaginidae) adult and S. maculata Quoy & Gaimard, 1824, Winter whiting adult.
- Location in the host:
Brain.
- Localities:
One Mile (27° 29′S; 153° 24′E) and Amity Point (27° 23′S; 153° 26′E), North Stradbroke Island, Queensland, Australia.
- Material:
Vouchers G464871–G464876 (Giemsa and eosin stained histological sections) and G464877–G464882 (H&E-stained histological sections) deposited in the Queensland Museum, South Brisbane, Australia.
- Prevalence:
Sillago ciliata – 9 of 35 (8 of 19 from One Mile, 1 from 2 at Amity Point, North Stradbroke Island; none of 1 from Shark Bay, Heron Island; none of 12 from Casuarina Beach, Lizard Island); Sillago maculata – 2 of 9 (from One Mile).
Description
Spores. (Fig. 1E, F, 3I–L). Spores stellate in apical view, radiating from centre with 5 or 6 equal shell valves (in voucher specimen, 5 polar capsule spores dominate 6 polar capsule spores (ratio of 86·2:13·8 in Sillago ciliata and 67·3:32·7 in Sillago maculata)), pyramidal in lateral view. Suture lines between valves not visible; shell distal margins tapering smoothly to point. Polar capsules pyriform, in anterior portion of shell valve and occupy approximately 45% of total length of shell valve in apical view. Spore measurements are shown in Table 3.
Morphometric data of brain myxosporean species
The morphometric data from each of the myxosporean species from brain tissue is shown in Table 3. Measurements of Kudoa chaetodoni n. sp. from Chaetodon unimaculatus that had 8 SV and 9 SV morphotypes were not significantly different. Kudoa sp. from Sillago ciliata differed significantly between the 5 SV and 6 SV morphotype with the 6 SV morphotype having a larger width and thickness, but smaller valve breadth.
Histology and spore ratios
Histological sections of brain tissue stained with Giemsa and eosin revealed multiple different spore morphotypes i.e. spores with different numbers of spore valves, originating from a single plasmodium in both Kudoa chaetodoni n. sp. and Kudoa sp. from Sillago ciliata (Fig. 4). Histological sections from Kudoa lethrini n. sp. did not reveal the minor morphotype (6 SV).

Fig. 4. Giemsa and eosin-stained histological section of pseudocyst. (A) Kudoa chaetodoni n. sp. (B) Kudoa lethrini n. sp. (C) Kudoa sp.
Kudoa chaetodoni n. sp. had an almost equal proportion of 8 SV and 9 SV morphotypes. K. lethrini n. sp. had almost complete domination of the 7 SV morphotype over 6 SV. Kudoa sp. had 5 SV dominate over 6 SV at 6:1 in Sillago ciliata and 2:1 in Sillago maculata.
Sequence alignment
The sequence of Kudoa ciliatae 18S rDNA derived in this study was almost identical to that of sequence from GenBank (see Kent et al. Reference Kent, Andree, Bartholomew and El-Matbouli2001, GenBank Accession number AF378348), but the new sequence provided an extra 700 base pairs (to the existing 880 bp) and resolved a number of indeterminate bases in the original sequence. Therefore, the sequence generated in this study was used for phylogenetic analyses and submitted to GenBank (Accession number DQ519390).
Phylogenetic analysis
Figure 5 shows a maximum likelihood analysis. Most clades were consistent in all analyses except for the placement of K. ciliataeLom, Rohde & Dykova, Reference Lom, Rohde and Dykova1992, K. dianae Dykova, Avila & Fiala, 2002, K. paniformis Kabata and Whitaker, 1981, and K. miniauriculata Whitaker, Kent & Sakanari, J. A., 1996. Kudoa ciliatae was either found branching before the clade of K. alliaria Kovaleva, Shulman & Yakovlev, 1979, K. clupeidae Hahn, 1917, K. dianae, K. funduli Hahn, 1915, K. miniauriculata, K. paniformis, and K. rosenbuschi Gelormini, 1943 (in maximum likelihood analyses); or forming a clade with K. ovivora Swearer & Robertson, D. R., 1999 (Bayesian, parsimony, and neighbour joining analyses). Kudoa dianae, K. paniformis, and K. miniauriculata always formed a clade but with different topologies depending on the analysis. The clades including these species always had low bootstrap confidence levels or low clade credibilities.

Fig. 5. Maximum likelihood analysis tree using the GTR+I+G model determined by the Alkaiki Information Criterion of kudoid 18S rDNA sequences. Bootstrap confidence levels greater than 50 shown at nodes. Symbols indicate the tissue type and geographical region from which each species was isolated. Where species have been sequenced from multiple hosts, see Table 2 for full host names.
The 5 species infecting the brain formed a single clade. The 2 species that infect cardiac tissue, K. hypoepicardialis Blaylock, Bullard & Whipps, 2005 and K. shiomitsui Egusa & Shiomitsu, 1983, also formed a clade. There were 3 distinct groups containing multiple species that infect skeletal muscle and a further 2 skeletal muscle species, K. thalassomi Adlard, Bryant, Whipps & Kent, 2005 and K. quadricornis Whipps, Adlard, Bryant & Kent, 2003, that fell outside all other groups. The smooth muscle representatives, K. ciliatae and K. dianae, did not form a clade. Kudoa ovivora, the only representative from gonadal tissue, did not form a clade with any small group of species or tissue type.
Only 2 clades of more than 1 species were associated with a single fish family, the Merlucciidae (Kudoa alliaria Kovaleva, Shulman & Yakovlov, 1979 and K. rosenbuschi Kovaleva, Shulman & Yakovlov, 1979); and Scombridae (K. grammatorcyni Adlard, Bryant, Whipps & Kent, 2005 and K. scomberomori Adlard, Bryant, Whipps & Kent, 2005). There are 10 fish families that have more than 1 Kudoa infection where the Kudoa species do not form clades.
Patterns in the analysis of the geographical distribution (Fig. 5) amongst the species varied depending on the scale considered. Two clades, 1 from Argentina and 1 from Australia, were associated with a single country. Resolution improved when looking at divisions based on the Atlantic/East Pacific and Indo/West Pacific giving 8 separate clades.
DISCUSSION
Characterization of new species
The morphological and molecular studies revealed 3 morphologically novel species from brain tissue. Histological examination of each of the species shows spore valve number plasticity comparable to that reported for K. monodactyli Gunter, Whipps, Cribb & Adlard, 2006 and Kudoa permulticapsula Whipps, Adlard, Bryant & Kent 2003.
Kudoa chaetodoni n. sp. is distinct from all other known species of multivalvulidan myxosporeans in having spores containing mainly 8 or 9 spore valves. This is the first myxosporean described from Chaetodon unimaculatus. Two multivalvulidans have been reported from chaetodontids previously, Kudoa muscularis (Cheung et al. Reference Cheung, Nigrelli and Ruggieri1983) from the muscle of Chaetodon collare Bloch, 1787 from the Philippine Islands and Kudoa sp. from muscle of a C. xanthurus Bleeker, 1857 of unknown provenance (Voelker, Reference Voelker1978). Molecular data are not available for these species. Kudoa chaetodoni n. sp. is most closely related to other species that infect brain tissue differing from Kudoa sp. from Sillago ciliata and K. yasunagai by 0·1% (2 bp), from K. lethrini n. sp. by 0·9% (14 bp) and from K. neurophila Grossel, Dykova, Handlinger & Munday, 2003 by 1·5% (23 bp).
Kudoa lethrini n. sp. is the second myxosporean species that has 7 spore valves. It is distinct from the other species, Kudoa yasunagai, in having lateral apical extensions at the distal edge of each valve similar to those of K. miniauriculata, a 4 SV species that infects the muscle of Sebastes paucispinis Ayres, 1854 (see Whitaker et al. Reference Whitaker, Kent and Sakanari1996). The measurements of spores of K. lethrini n. sp. are distinct from those of K. yasunagai but this comparison is compromised by the fact that the measurements given by Hsieh and Chen (Reference Hsieh and Chen1984) for K. yasunagai were taken from spores preserved in formalin which shrinks spores (Parker and Warner, Reference Parker and Warner1970). This is the first record of Kudoa from the family Lethrinidae. The other 7 SV species, K. yasunagai was recorded from Lateolabrax japonicus Cuvier, 1828 (Lateolabracidae) and Paralichthys olivaceus Temminck & Schlegel, 1846 (Paralichthyidae).
In 18S rDNA sequence, K. lethrini n. sp. is most closely related to Kudoa sp. from Sillago ciliata and K. yasunagai, only differing by 0·8% (12 bp). K. lethrini n. sp. differs from the other brain-inhabiting species by 0·9% (14 bp) from K. chaetodoni n. sp., and 1·2% (19 bp) from K. neurophila.
Kudoa sp. from Sillago ciliata differs from all but 1 (K. neurophila) of the 4 known 5 SV species of Kudoa by being localized in the brain (see Table 4). Its spores are distinctly larger than those of K. neurophila and K. shulmaniNaidjenova & Zaika, Reference Naidjenova and Zaika1970, and distinctly smaller than those of K. cutaneaKovaljova & Gajevskaja, Reference Kovaljova and Gajevskaja1984 and K. muscularisCheung, Nigrelli & Ruggieri, Reference Cheung, Nigrelli and Ruggieri1983. The polar capsule length is similar to those of K. neurophila and K. muscularis, larger than that of K. shulmani, and smaller than that of K. cutanea. The polar capsule width is smaller than that of K. neurophila and K. cutanea but larger than that of K. muscularis.
Table 4. Kudoa sp. data collated with the other known 5 spore valve Kudoa species
(PCL=Polar capsule length, PCW=Polar capsule width.)

Kudoa sp. from Sillago ciliata differs in 18S rDNA sequence from K. chaetodoni n. sp. by 0·1% (2 bp), K. lethrini n. sp. by 0·8% (12 bp), and K. neurophila by 1·4% (21 bp). However, the 18S rDNA sequence was identical to that of K. yasunagai (which has 7 SV) from the brain of Paralichthys olivaceus.
The morphometric data from the 7 SV K. yasunagai compared with that from Kudoa sp. from Sillago ciliata (see Table 5) shows significant differences, particularly in the proportions that reflect spore shape. Finding identical 18S rDNA sequences for myxosporeans that have dramatically different morphology and occur in widely disparate hosts is a surprising and unique outcome. Hsieh and Chen (Reference Hsieh and Chen1984) mentioned the existence of exceptional spores with 6 or 8 shell valves in the original description of K. yasunagai, which blurs the distinction, at least on polar capsule count, between it and Kudoa sp. from Sillago ciliata, which has both 5 SV and 6 SV morphotypes. Although spore valve number does not reflect directly the genetic affinities between groups of kudoid species (see Whipps et al. Reference Whipps, Grossel, Adlard, Yokoyama, Bryant, Munday and Kent2004), to identify Kudoa sp. as K. yasunagai on the basis of the 18S rDNA sequence would be unsatisfactory as it would imply that almost no aspect of the morphology of kudoid spores was a reliable indicator of identity. Thus, we take the conservative approach and hypothesize that the morphological difference between these forms is indeed an indication of species-level separation and that the 18S rDNA is incapable of distinguishing some combinations of species of Kudoidae. The obvious next step is to investigate gene regions that offer higher rates of mutation and would thus provide higher resolution.
Table 5. Comparison of morphometrics of Kudoa yasunagai and Kudoa sp. from Sillago ciliata from formalin preserved spores

The paucity of morphological characters (at light microscope level) drives us to rely on molecular characters to establish individual taxa. However, this study has uncovered the likely limit of resolution in 18S rDNA by showing that morphologically distinct forms (Kudoa sp. from Sillago ciliata and K. yasunagai) shared 100% homology in that region. In contrast, analysis of 18S rDNA of Kudoa thyrsites Gilchrist, 1924 (see Whipps and Kent, Reference Whipps and Kent2006) shows that species identified as K. thyrsites can have significant variation in 18S rDNA correlated with the provenance of the isolate. We consider it likely that the variation in 18S rDNA shown by K. thyrsites isolates reflects the presence of a complex of species. The greatest reported difference between K. thyrsites 18S rDNA sequences (0·9%, 14 base pairs) was between that from Scomber scombrus Linnaeus, 1758 from England and that from Paralichthys olivaceus from Japan. This is the same level of difference we report here between the 2 morphologically distinct species K. chaetodoni n. sp. (8/9 SV) and K. lethrini n. sp. (7 SV with lateral apical extensions). The contrast between the complex of K. thyrsites and the molecular identity of Kudoa sp. from Sillago ciliata and K. yasunagai shows 2 extremes with regard to the analysis of morphology and genotype in the Kudoidae. This complexity provides a challenge for species identification of kudoids until further molecular data are available.
Tissue tropism and genetic relatedness
The need to correlate genetic relationships with characters other than spore valve number has been clear since Whipps et al. (Reference Whipps, Grossel, Adlard, Yokoyama, Bryant, Munday and Kent2004) showed that this character did not correlate with genetic relatedness within the Kudoidae. Our phylogenetic analyses support that conclusion in that species with different spore valve number such as K. permulticapsula (13 SV) and K. scomberomori (6 SV) are closely related, whereas others with the same number of spore valves (4 SV) such as K. amamiensis Egusa & Nakajima, 1980 and K. iwatai Egusa & Shiomitsu, 1983 are relatively distant.
Abollo et al. (Reference Abollo, Novoa and Figueras2005) noted that since the majority of DNA sequences generated for Kudoa species are from those that infect muscle, it was necessary to include more species from different host tissues in order to assess the importance of tissue specificity. Our study has introduced 3 new isolates infecting the brain of fish hosts. In all the analyses, the 3 brain- dwelling isolates formed a strongly supported clade with the other available species from that tissue, K. neurophila and K. yasunagai. Furthermore, K. hypoepicardialis and K. shiomitsui, the only 2 kudoids known to infect cardiac muscle, also formed a clade in all analyses. This result supports the hypothesis that the tissue tropism of some kudoids correlates with genetic relatedness, but there are also significant departures from it. For example, Kudoa dianae and K. ciliatae which infect smooth muscle are apparently unrelated, although the position of K. dianae had low clade credibility and low bootstrap confidence values. Twenty-seven of the 43 18S species analysed were from skeletal muscle. The phylogenetic analyses revealed 3 major clades of muscle-infecting species and 3 species falling outside these clades. It is possible that this multiplicity of clades results from muscle being the ancestral site of infection. However, multiple skeletal muscle clades could equally reflect a series of separate radiations in this site.
Kudoa iwatai and K. lutjanus Wang, Huang, Tsai, Cheng, Tsai, Chen, Chen, Chiu, Liaw, Chang & Chen, 2005 have both been reported as infecting several tissue sites. K. iwatai was reported to infect brain, eye, intestinal tract, heart, kidney, muscle, mouth, nerve axon, ovary, and peritoneum (Diamant et al. Reference Diamant, Ucko, Paperna, Colorni and Lipshitz2005). Wang et al. (Reference Wang, Huang, Tsai, Cheng, Tsai, Chen, Chen, Chiu, Liaw, Chang and Chen2005) reported that K. lutjanus infects brain, eye, muscle and intestinal organs. Thus, some species have the ability to infect almost any site. The apparent close relationship between K. iwatai and K. lutjanus suggests that there may be an ‘opportunistic’ clade.
Geographical distribution and genetic relatedness
Genetic relationships did not correlate well with geographical distribution. The only clades associated with a single region are that of K. alliaria and K. rosenbuschi from Argentina and K. grammatorcyni, K. monodactyli, K. permulticapsula, and K. scomberomori all from the east coast of Australia. Otherwise, all the major clades incorporate species from multiple localities, despite sampling being concentrated in a few key regions of the world. Local concentration of data (due to the location of research groups) is likely to result in false geographical patterns but the reverse appears to be the case. There are 8 clades that correspond to the major biogeographical divisions in the Indo/West Pacific and Atlantic/East Pacific but there is so much mixing that this appears to have little significance.
Host specificity and genetic relatedness
Although some kudoids have been recorded from several fish families, and even from different fish orders, most have been recorded from a single host species or family. The findings in this study were consistent with this pattern; all 4 species studied here were found in 1 family; Kudoa chaetodoni n. sp. from a single chaetodontid, K. ciliatae was recorded from 3 sillaginid species, K. lethrini n. sp. was recorded from 2 lethrinid species, and Kudoa sp. from 2 sillaginids. This specificity cannot be considered definitive as sister host families in the geographical regions where infections were recorded are incompletely sampled. Specificity to a single host family, has previously been demonstrated experimentally for the bivalvulidan myxosporean, Myxobolus cerebralis Wolf & Markiw, 1984, by El-Matbouli et al. (Reference El-Matbouli, Hoffmann, Schoel, McDowell and Hedrick1999). This species was able to establish in Salmonidae whereas Adrianichthyidae, Cyprinidae, Poeciliidae, and frogs were refractory to infection.
There are 5 cases of individual fish species being infected by 2 or more kudoids for which molecular data are available for both species. Paralichthys olivaceus is infected by K. shiomitsui (heart), K. thyrsites (muscle), and K. yasunagai (brain); Pempheris ypsilychnus Mooi & Jubb, 1996 is infected by K. amamiensis (muscle), and K. minithyrsites Whipps, Adlard, Bryant, Lester, Findlay & Kent, 2003 (muscle); Scomberomorus commerson Lacepède, 1800 is infected by K. permulticapsula (muscle) and K. scomberomori (muscle); Seriola quinqueradiata is infected by K. amamiensis and K. megacapsula; and Sillago ciliata is infected by K. ciliatae (smooth muscle of the gut wall) and Kudoa sp. (brain). In all 5 cases, relationships reflected site of infection rather than host identity. Kudoa amamiensis and K. minithrysites from Pempheris ypsilychnus are both found in muscle-dwelling clades, but in clades of different origins. Including these examples, at the fish family level, there are 10 examples where tissue tropism relatedness is supported over host identity with the families: Carangidae being infected by K. amamiensis, K. megacapsulaYokoyama & Itoh, Reference Yokoyama and Itoh2005, and K. quadricornis; Clupeidae being infected by K. clupeidae and K. thyrsites; Labridae being infected by K. ovivora and K. thalassomi; Merlucciidae being infected with K. alliaria, K. paniformis, and K. rosenbuschi; Scombridae being infected with K. crumena Iversen & Van Meter, 1967, K. grammatorcyni, K. permulticapsula, K. scomberomori, K. thyrsites; Sparidae being infected by 2 split clade isolates of K. iwatai; and Tetraodontidae being infected with K. dianae and K. shiomitsui.
It is likely that host identity contributes to the parasite distribution observed, but these factors seem to have a lesser role than the tissue they infect. At present there is thus no evidence of any radiation of kudoids in association with fish host taxon.
We thank Dr Jessica Worthington Wilmer and Terrence Miller for assistance with molecular techniques and phylogenetics, Chris Cazier and staff from Veterinary Pathology at the University of Queensland for histological assistance, Dr Chris Whipps for advancing a copy of his then unpublished manuscript on Kudoa thyrsites; Terrence Miller, Dr Matthew Nolan, Abigail Downie and Dr Mal Bryant for assistance with collection of hosts and the staff and facilities at the Australian Museum's Lizard Island Research Station, the University of Queensland's Heron Island Research Station and Moreton Bay Research Station.