Introduction
The introduction and establishment of gypsy moth, Lymantria dispar (L.) (Lepidoptera: Erebidae), is often referred to as one of the most destructive ecological disturbances in North American temperate forests (e.g., Sharov et al. Reference Sharov, Leonard, Liebhold, Roberts and Dickerson2002; Invasive Species Specialist Group 2009). Gypsy moth outbreaks in forest stands can open up the canopy (e.g., Gale et al. Reference Gale, DeCecco, Marshall, McClain and Cooper2001), reduce tree growth (e.g., Muzika and Liebhold Reference Muzika and Liebhold1999), weaken and kill trees (e.g., Davidson et al. Reference Davidson, Gottschalk and Johnson1999), alter nutrient cycling and other ecosystem processes (Lovett et al. Reference Lovett, Canham, Arthur, Weathers and Fitzhugh2006), and change stand structure and composition (Jedlicka et al. Reference Jedlicka, Vandermeer, Aviles-Vasquez, Barros and Perfecto2004). These effects can cause temporary and (or) lasting changes in habitat for other forest organisms (e.g., Kasbohm et al. Reference Kasbohm, Vaughan and Kraus1994; Schowalter and Whitmore Reference Schowalter and Whitmore2002).
Although its effects on trees and forests have been well studied, there has been little research on the impacts of gypsy moth on native forest insects. Although the presence of gypsy moth likely has an impact on native insect communities (e.g., Scriber Reference Scriber2004; Summerville and Crist Reference Summerville and Crist2008), most of such studies have examined nontarget effects of gypsy moth management on native species (Butler and Kondo Reference Butler and Kondo1993; Butler et al. Reference Butler, Chrislip and Kondo1995, Reference Butler, Chrislip, Kondo and Townsend1997; Sample et al. Reference Sample, Butler, Zivkovich, Whitmore and Reardon1996; Wagner et al. Reference Wagner, Peacock, Carter and Talley1996; Rieske and Buss Reference Rieske and Buss2001; Rastall et al. Reference Rastall, Kondo, Strazanac and Butler2003; Boulton et al. Reference Boulton, Otvos, Halwas and Rohlfs2007; Schweitzer et al. in press) as well the effects of introduced natural enemies and pathogens (Boettner et al. Reference Boettner, Elkinton and Boettner2000; Kellogg et al. Reference Kellogg, Fink and Brower2003; Hajek et al. Reference Hajek, Strazanac, Wheeler, Vermeylen and Butler2004; Ellis et al. Reference Ellis, Walter, Tooker, Ginzel, Reagel and Lacey2005). Wagner and Van Driesche (Reference Wagner and Van Driesche2010) listed gypsy moth management as a major factor in the decline of threatened species of forest Lepidoptera in the northeastern United States of America. Except for Sample et al. (1996), none of these studies have considered the impacts of gypsy moth itself, despite the fact that its presence and (or) numerical dominance in a forest stand may have important direct and indirect effects on native species.
The establishment of gypsy moth in North American forests has brought it into association with a diverse assemblage of native Lepidoptera (Schaffner and Griswold Reference Schaffner and Griswold1934; Rose and Lindquist Reference Rose and Lindquist1982; Wagner et al. Reference Wagner, Giles, Reardon and McManus1997; Schweitzer et al. Reference Schweitzer, Minno and Wagner2011) as well as their host plants and natural enemies. The presence of large gypsy moth populations may affect the natural and non-native enemy communities by attracting or augmenting native parasitoids and predators (Redman and Scriber Reference Redman and Scriber2000; Barber et al. Reference Barber, Marquis and Tori2008) and species that have been introduced for its control (Smith and Lautenschlager Reference Smith and Lautenschlager1978; Simons et al. Reference Simons, Reardon and Ticehurst1979; Nealis et al. Reference Nealis, Carter, Kenis, Quednau, van Frankenhuyzen, Mason and Huber2002). By feeding on common host trees gypsy moth may compete directly with native species, especially during periods of high abundance, when it may remove almost all foliage from a stand (e.g., Davidson et al. Reference Davidson, Gottschalk and Johnson1999). Although defoliated host trees usually produce a second flush of leaves, summer-feeding caterpillars in some habitats and with certain life-history traits may starve during gypsy moth outbreaks (Schweitzer Reference Schweitzer2004). In addition, spring feeding by gypsy moth induces changes in host plant foliage quality (Schultz and Baldwin Reference Schultz and Baldwin1982; Havill and Raffa Reference Havill and Raffa1999); this may indirectly affect the growth and survival of species feeding later in the season as well as in subsequent years (Scriber et al. Reference Scriber, Weir, Parry and Deering1999; Redman and Scriber Reference Redman and Scriber2000).
Competition between native and introduced species, especially those with outbreak populations, may be an important community-structuring factor (Denno et al. Reference Denno, Peterson, Gratton, Cheng, Langellotto, Huberty and Finke1995). Indirect interactions may be especially important in these situations (White et al. Reference White, Wilson and Clarke2006; Gandhi and Herms Reference Gandhi and Herms2010). Damage by spring-feeding outbreak species within natural forest herbivore communities can affect later feeding species through indirect, plant-mediated interactions (e.g., Haukioja and Niemelä Reference Haukioja and Niemelä1979; Hunter Reference Hunter1987; Neuvonen et al. Reference Neuvonen, Hanhimäki, Suomela and Haukioja1988; Dankert et al. Reference Dankert, Herms, Parry, Scriber, Haas and Raman1997; Wold and Marquis Reference Wold and Marquis1997). Where the impact of gypsy moth on native communities has been explicitly addressed, some evidence was found linking indirect effects of high gypsy moth populations with reduced abundance or fitness of some Lepidoptera (Sample et al. Reference Sample, Butler, Zivkovich, Whitmore and Reardon1996; Redman and Scriber Reference Redman and Scriber2000; Work and McCullough Reference Work and McCullough2000).
Our objective was to investigate the ecological impacts of gypsy moth on the diversity and structure of native caterpillar assemblages in northern temperate forests. We focused on north-central Ontario, the northern edge of the current distribution of gypsy moth in Canada, as well as the area of most recent infestation and the northern edge of the range of its preferred host, red oak (Quercus rubra L.) (Fagaceae). No extensive survey of Lepidoptera had previously been carried out in these forests. In 2006, at the beginning of this study, gypsy moth had reached outbreak levels in some, but not all, of the oak-dominant forests of this region, and thus we were able to compare sites with and without histories of gypsy moth outbreak. We predicted that past gypsy moth outbreaks would have had long-term ecological impacts on the natural enemy community and (or) host foliage in a forest stand and caused loss of diversity within native assemblages and notable differences in the composition of the caterpillar community at sites with different outbreak histories.
Materials and methods
Study sites
We sampled caterpillars from 10 study sites (2–8ha in area) within red oak-dominated stands in north-central Ontario (Table 1, Fig. 1). Five sites were in stands with a history of moderate to severe defoliation by gypsy moth (GM history); these were paired with five similar sites in stands where gypsy moth damage had never been observed (no GM history). Canadian Forest Service survey data and Ontario Forest Resource Inventory maps were used to identify areas with and without gypsy moth outbreak history and individual stands, respectively. Canadian Forest Service survey data are based on regular aerial and ground surveys of affected stands conducted each year. The first high populations of gypsy moth observed at the study sites occurred between 1993 and 1996 (Evans et al. Reference Evans, Jones, Smith, Rowlinson and Francis1997; Nealis et al. Reference Nealis, Roden and Ortiz1999); a second period of moderate to severe defoliation occurred from 2001 to 2003 (Hopkin and Scarr Reference Hopkin and Scarr2003). Efforts were made to ensure that other ecological and geophysical variables were as similar as possible in all plots (Table 1). All sites are located within ecodistrict 411 in the Algonquin - Lake Nipissing ecoregion (Ecological Stratification Working Group 1995) (Fig. 1).
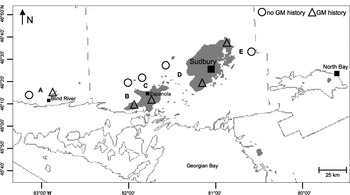
Fig. 1. Locations of paired sites within (Δ) and outside of (○) recorded areas of heavy gypsy moth defoliation (shaded) in north-central Ontario, Canada. The map is based on aerial- and ground-survey data from the Canadian Forest Service Forest Insect and Disease Survey (1950–1991) and the Canadian Forest Service Forest Health Monitoring Unit (1993–2005). The figure is modified from a map prepared by B. Biggs and R. Fournier, Great Lakes Forestry Centre, Canadian Forest Service, Sault Ste. Marie, Ontario (2006).
Table 1. Characteristics of 10 northern temperate forest stands surveyed for macrolepidopteran caterpillar communities in north-central Ontario, Canada, during the summers of 2006 and 2007.

*Dominant species: RO, red oak, Quercus rubra L.; RM, red maple, Acer rubrum L.; SM, sugar maple, Acer saccharum Marsh.; LTA, largetooth aspen, Populus grandidentata Michx.; TA, trembling aspen, Populus tremuloides Michx.; WAS, white ash, Fraxinus americana L.; WB, white birch, Betula papyrifera Marsh.; EWP, eastern white pine, Pinus strobus L.
† Values are given as the mean ± SE.
‡ Percent canopy openness, a measure of the amount of light coming through the canopy, was determined using a densiometer at 50 points in each stand in mid-July 2006.
§ Determined using Canadian Forest Service Forest Health survey maps, available at the Great Lakes Forestry Centre, Sault Ste. Marie, Ontario.
Caterpillar sampling and rearing
Caterpillars were collected bimonthly from May through August in 2006 and 2007 using the burlap-band sampling technique, a method known to be effective in monitoring gypsy moth populations (Weseloh Reference Weseloh1987) as well as populations of native species from various other families of Lepidoptera (Wagner et al. Reference Wagner, Peacock, Carter and Talley1995; Butler and Strazanac Reference Butler and Strazanac2000; Raimondo et al. Reference Raimondo, Strazanac and Butler2004). Burlap bands were wrapped around the trunks of 30 dominant or co-dominant red oak trees at each site. Another 20 bands were placed on each of 10 co-dominant trees of two common species in the stand: either red maple (Acer rubrum L.) or sugar maple (A. saccharum Marsh.) (Aceraceae), largetooth (Populus grandidentata Michx.) or trembling aspen (P. tremuloides Michx.) (Salicaceae), white ash (Fraxinus americana L.) (Oleaceae), or white birch (Betula papyrifera Marsh.) (Betulaceae). Banded trees were located within areas of approximately 1.5–3.5 ha. Bands were 50 cm in width and were tied onto the trunk at approximately 1.3m above ground, then folded in two over a piece of twine. Banded trees were chosen haphazardly at the beginning of May 2006. The same 50 trees at each site were used in both years, though approximately 10 trees at one site (Wavy) were replaced in July 2006 because of windstorm damage.
During sampling visits all macrolepidopteran caterpillars found on, underneath, and just above or below the bands were collected into individual transparent plastic rearing cups (8cm diameter, plastic soufflé cups, Solo Cup Company, Mississauga, Ontario), provided with host-tree foliage, and identified using appropriate literature (Wagner et al. Reference Wagner, Giles, Reardon and McManus1997; Troubridge and Lafontaine Reference Troubridge and Lafontaine2004; Wagner Reference Wagner2005; Opler et al. Reference Opler, Lotts and Naberhaus2009). From late May through mid-July, high populations of the forest tent caterpillar, Malacosoma disstria Hübner (Lepidoptera: Lasiocampidae), and gypsy moth were found at three stands. During each sampling visit, total numbers of these abundant species were recorded but, to keep rearing work to a sustainable level, only two caterpillars and (or) two pupae (maximum) of each species were collected from each tree. In 2007 all forest tent caterpillars and gypsy moth caterpillars were counted. In 2006 all gypsy moth caterpillars were counted, but the sizes of forest tent caterpillar populations at the three sites with high populations were estimated using the differences between collected and observed numbers from 2007.
All specimens were reared in the laboratory at 21°C under a natural light:dark photoperiod. Rearing cups were checked daily and caterpillars were provided with fresh foliage as needed. Foliage was obtained from oak, aspen, and maple trees on the University of Toronto campus and rinsed with distilled water to remove potential pathogens and air-dried before being used for feeding. Changes in condition of each specimen were noted (e.g., pupation, adult emergence, death). Pupae requiring diapause were overwintered outside and were brought inside in late winter to induce emergence. Successfully emerged adult Lepidoptera were killed and pinned, and their larval identifications were confirmed or corrected. Voucher specimens were deposited in the Forest Entomology laboratory at the University of Toronto.
Data analyses
Over 111 species of macrolepidoptera were collected and reared (Table 2, Appendix). Species names and classifications follow Troubridge and Lafontaine (Reference Troubridge and Lafontaine2004) and Lafontaine and Schmidt (Reference Lafontaine and Schmidt2010). Collections yielded 75 (2006) and 58 species (2007). Twenty-four species were shared among years and, of these, 13 were present at sites with and without gypsy moth history (Table 3). In addition to using the entire data matrix of individuals (identified at least to family) collected at all 10 sites over both sampling years, we also performed selected analyses after dividing the community into two groups based on larval phenology. Each species was classified as an early- (May and June) or late-season (July and August) feeder depending on collection dates as well as on available life-history information. Sixty-nine species were classified as early-season and 42 as late-season feeders. This division was considered useful because gypsy moth may have a greater effect on late-season feeders than on early-season feeders; caterpillars feeding later in the summer develop after the peak period of gypsy moth activity and are exposed to any gypsy moth-induced changes in the local ecology (Work and McCullough Reference Work and McCullough2000; Schweitzer Reference Schweitzer2004).
Table 2. Numbers of species and larval abundances of families and subfamilies of macrolepidoptera collected during the summers of 2006 and 2007 from burlap bands on 500 hardwood trees in northern temperate forest stands in north-central Ontario, Canada, with (n = 5) and without (n = 5) history of gypsy moth (GM) outbreak.

Note: Values in parentheses are larval abundances.
*Specimens that were not identified to at least family level were not included in analyses.
Table 3. Pooled abundances of gypsy moth and 12 native macrolepidopteran species collected consistently over 2 years from burlap bands on 500 hardwood trees in northern temperate forest stands in north-central Ontario, Canada, with (n = 5) and without (n = 5) a history of gypsy moth (GM) outbreak.

*Correlations between log-transformed abundances of gypsy moth and each species within each site. Values in parentheses are 95% confidence intervals. Values where the bounds of the bootstrapped 95% confidence intervals do not overlap zero are shown in boldface type.
† Abundances are based on observed numbers, not collected specimens (see Methods).
Correlations were calculated between the observed total numbers of gypsy moth and the number of individuals collected at each site for each of the other 12 species collected at sites of each type in both years. Pearson's correlation coefficients were calculated using log10(abundance +1)-transformed data for each site and are presented with bootstrapped 95% confidence intervals to assess significance. The log10(abundance+1) transformation was chosen for this as well as for all further analyses of species abundance data because of the large differences in abundance between rare and common species.
Species diversity
Community diversity and evenness were examined using rank-abundance plots and rarefaction analysis. We used the entire species data matrix for these analyses, including morphospecies as well as individuals that we were able to assign to a genus but not to a species. Rank-abundance plots were created for pooled data from sites with and without gypsy moth history for 2006 and 2007. We used Estimate-S (Colwell Reference Colwell2005) to calculate sample-based rarefaction curves (Gotelli and Colwell Reference Gotelli and Colwell2001) for each site over both years. Each of the 50 trees at each site from which collections were made was considered a sample in these calculations. Curves were rescaled to the number of individuals collected, as recommended for studies concerned with species richness (Gotelli and Colwell Reference Gotelli and Colwell2001; Buddle et al. Reference Buddle, Beguin, Bolduc, Mercado, Sackett and Selby2005). We used the rarefaction calculations to find the Mao Tau estimate of the expected number of species (Colwell et al. Reference Colwell, Mao and Chang2004) for each site at a sample size of 50 individuals, the lowest number of individuals collected from any site over both years. This allowed comparisons of species richness between sites despite apparent differences in sampling effort (i.e., the different number of individuals collected from each sample tree). As a predictor variable of interest, gypsy moth abundance was included neither in the data used to calculate the rarefaction curves nor in the response data for any of the subsequent analyses.
The remaining statistical analyses were conducted in R (R Development Core Team 2007) using packages vegan (Oksanen et al. Reference Oksanen, Kindt, Legendre and O'Hara2006) and nlme (Pinheiro et al. Reference Pinheiro, Bates, DebRoy and Sarkar2007). We used mixed effects modeling in tests of the effects of gypsy moth (either outbreak history or abundance) and sampling year on various measures of species diversity. Mixed effects models were chosen because by modeling site identification as a random effect, they can account for the fact that the data include two observations (i.e., sample years) from each of the 10 sites (Lindstrom and Bates Reference Lindstrom and Bates1990; Zuur et al. Reference Zuur, Ieno, Walker, Saveliev and Smith2009). The first set of mixed effects models tested the effects of gypsy moth history (yes or no) and sampling year (2006 or 2007) on five response variables: (1) log10 gypsy moth abundance, (2) log10 abundance of forest tent caterpillars, (3) log10 abundance of all other species, (4) observed species richness, and (5) rarefied estimates of species richness. Forest tent caterpillar abundance was included as a response variable because a strong positive correlation was detected between it and gypsy moth at the study sites. The second set of models tested the effects of log10 gypsy moth abundance and sampling year on response variables 2 through 5. We followed model-fitting procedures (Zuur et al. Reference Zuur, Ieno, Walker, Saveliev and Smith2009) including fitting the full model, finding the optimal random structure, and finding the optimal fixed structure. The fit of these models to the data was assessed using diagnostic graphical methods, including plots of fitted values versus standardized residuals as well as normal QQ plots for fixed and random residual error.
Community structure
We used nonmetric multidimensional scaling (NMDS) to explore patterns in community structure between sampling years and sites with different gypsy moth history. NMDS is a distance-based ordination method that represents each community as a point in a scatterplot such that distances between points approximate observed community dissimilarities (Oksanen Reference Oksanen2007). It is a robust technique (Minchin Reference Minchin1987) and is commonly used to investigate patterns in communities of forest arthropods (e.g., Hilt et al. Reference Hilt, Brehm and Fiedler2006; Summerville et al. Reference Summerville, Bonte and Fox2007). We used Bray-Curtis distance, a measure considered more appropriate for community data than are other common distance measures (Legendre and Gallagher Reference Legendre and Gallagher2001), to quantify observed community dissimilarities. We carried out NMDS using the function metaMDS in R, which uses several random starting configurations and selects among similar solutions with the smallest stresses, thus yielding a solution based on a global instead of a local minimum. A Sheppard diagram was used to assess the fit of the final solution.
One NMDS was carried out with the full data matrix of species and a second with the reduced matrix of 12 shared species (Table 3, not including gypsy moth). Axis scores from the full NMDS were correlated with and graphed against transformed species abundance data to determine which species were best described by variation in the axes (Legendre and Gallagher Reference Legendre and Gallagher2001). Examination of these results indicated that most species other than the 12 shared species had little to no relationship with either axis, thus we present only the results of the ordination using the reduced species matrix. This also reduces the number of rare species in the data matrix, eliminating large numbers of zeroes in the data, which can cause difficulties in accurately calculating distance matrices and also problems with the results of ordinations (Legendre and Legendre Reference Legendre and Legendre1998). As a means of graphically demonstrating the relationship between gypsy moth abundance (as compared with gypsy moth history) and community structure, the sizes of points in the NMDS graph are scaled in proportion to the abundance of gypsy moth at each site.
Finally, we tested the hypothesis that current gypsy moth abundance was related to patterns in community structure, using analysis of dissimilarities, which calculates a permutational multivariate analysis of variance using distance matrices. It is analogous to distance-based redundancy analysis, and also has been equated to a nonparametric multivariate analysis of variance without the need to meet the stringent assumptions of multivariate normality (McArdle and Anderson Reference McArdle and Anderson2001). The analysis was conducted on the log-transformed species data and was run through 1000 permutations using a Bray-Curtis distance matrix and the entire community as well as the early- and late-season groups as the response matrices. In addition, because of the strong positive correlation between gypsy moth and forest tent caterpillar, we repeated the entire community as well as the early-season analyses after removing forest tent caterpillar from the species matrix. The full models tested the effects of gypsy moth abundance, sample year, and the interaction between the two terms on each of the five species matrices. None of the interaction terms in these five models were significant; therefore, in accordance with the principle of parsimony, the final models tested only the additive effects of gypsy moth abundance and sample year on the community structure.
Results
Lepidopteran community
Over the 2-year study we collected a total of 6923 individuals representing more than 111 species (Table 2, Appendix). We collected more noctuine (Erebidae) species (58) than any other family or subfamily, followed by Geometridae (21) and lymantriines (Erebidae) (10). This trend held true in both sampling years and at sites with and without a history of gypsy moth outbreak. There were no large differences in family- or subfamily-level species richness across site types or years, except that most species of Saturniidae and Notodontidae were collected only at sites without histories of gypsy moth outbreak (Table 2).
Gypsy moth was present at some level at all sites over both years and made up 55% (3861) of all individuals collected (Appendix). Over both years, an additional 15756 gypsy moth caterpillars were observed on the banded trees but not collected. Of the remaining 3062 individuals collected, the four most abundant species of Lepidoptera were forest tent caterpillar (1377) and Orgyia leucostigma (J.E. Smith) (Erebidae: Lymantriinae) (338), Abagrotis alternata (Grote) (Erebidae: Noctuinae) (170), and Morrisonia latex Guenée (Erebidae: Noctuinae) (78). An additional 489 forest tent caterpillars were observed but not collected in 2007. More than three-quarters of the species encountered in the collections were uncommon, represented by five or fewer individuals. Gypsy moth abundance was significantly correlated with 3 of the 12 commonly collected species; gypsy moth exhibited a positive correlation with the forest tent caterpillar and negative correlations with O. leucostigma and Catocala ilia (Cramer) (Erebidae: Noctuinae) (Table 3).
Species diversity
Several patterns are apparent in the rank abundance distribution curves (Fig. 2). In 2006, sites with no gypsy moth history displayed a relatively even pattern of species abundance, whereas sites with gypsy moth history were dominated by a few very abundant species. In addition, sites without gypsy moth history contained a greater number of species than those with gypsy moth history. However, in 2007 these differences were not present; both site types exhibited a high dominance and reduced number of species.
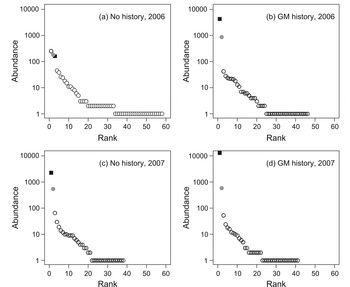
Fig. 2. Rank-abundance distributions of pooled collections of macrolepidoptera from sites in north-central Ontario, Canada, with (n = 5) and without (n = 5) histories of gypsy moth outbreak, collected using burlap bands on 50 hardwood trees at each stand from May though August in 2006 and 2007; the relative positions of gypsy moth and forest tent caterpillars in each distribution are indicated by solid squares and grey circles, respectively.
The identities of the most abundant species are also of interest. In 2006 the sites without gypsy moth history were dominated by O. leucostigma (250), forest tent caterpillar (183), and gypsy moth (159), while sites with gypsy moth history were dominated by gypsy moth (4298) and forest tent caterpillar (765). In 2007, sites without and with gypsy moth history were both dominated by gypsy moth (2258 and 12902, respectively) and forest tent caterpillar (540 and 581, respectively).
All rarefaction curves for each of the 10 sites over the 2 sampling years demonstrate steep slopes and fail to reach an asymptote (Fig. 3), indicating that the sampling did not capture all species at any of the sites. Comparisons between sites and site types are therefore made with caution, even after data standardization using rarefaction to account for the different number of individuals collected in each sample. Rarefaction curves from 2006 show some separation between sites with and without gypsy moth history; the three sites with the lowest rarefied-species richness are sites with histories of gypsy moth outbreak. In the rarefaction curves from 2007, however, sites of both types are mostly clustered together with lower rarefied estimates of species richness than in the previous year.

Fig. 3. Sample-based rarefaction curves, rescaled to the number of individuals collected, for bimonthly collections of macrolepidoptera from burlap bands on 50 hardwood trees at each of 10 northern temperate forest stands in north-central Ontario, Canada, with (solid lines) and without (broken lines) histories of gypsy moth outbreak in 2006 (a) and 2007 (b); the arrow indicates the smallest number of individuals collected at one site in both years (50).
Total species abundance, richness, and rarefied estimates of species richness from all 10 sites over both years are presented in Table 4. Gypsy moth abundance was significantly higher in 2007 than in 2006 (P < 0.001) and marginally significantly higher at sites with histories of outbreak than those without (P = 0.047) (Table 5). None of the other four response variables were significantly related to gypsy moth history (forest tent caterpillar abundance: P = 0.706; other-species abundance: P = 0.119; observed richness: P = 0.305; estimated richness: P = 0.685). Although the abundance of forest tent caterpillar was not significantly related to sampling year (P = 0.110), pooled other-species abundance as well as observed and estimated species richnesses were significantly lower in 2007 than in 2006 (other-species abundance: P = 0.001; observed richness: P = 0.005; estimated richness: P = 0.020) (Table 5).
Table 4. Characteristics of collections of macrolepidoptera, made over two summers, from burlap bands on 500 hardwood trees in northern temperate forest stands in north-central Ontario, Canada, with (n = 5) and without (n = 5) a history of gypsy moth (GM) outbreak, including the abundances of gypsy moth, forest tent caterpillar, and all other species combined, as well as observed species richness and rarefied estimates of species richness.

*Abundances are based on observed numbers, not collected (see Methods).
† Observed species richness does not include gypsy moth.
‡ Calculated using sample-based rarefaction to estimate the number of species for each site at a sample size of 50 individuals, the lowest number of individuals collected from any site over both years. Values are given as the mean ± 95% CI.
Table 5. Mixed effects models of the additive effects of gypsy moth (GM) outbreak history and sampling year on five characteristics of communities of macrolepidoptera collected from burlap bands on 50 hardwood trees at each of 10 northern temperate forest stands in north-central Ontario, Canada, with different histories of GM outbreak over 2 sampling years (2006 and 2007).

Gypsy moth abundance was positively correlated with forest tent caterpillar abundance (P = 0.036) but not with the pooled abundance of all other species collected (P = 0.215), observed species richness (P = 0.341), or rarefied-species richness (P = 0.114) (Table 6). In these models, sampling year was not significantly related to total species abundance (P=0.060), forest tent caterpillar abundance (P = 0.412), observed-species richness (P = 0.089), or rarefied-species richness (P = 0.677) (Table 6). Diagnostic graphing indicated reasonable homogeneity and normality of residuals in both sets of mixed effects models, although models with gypsy moth abundance and estimated species richness as response variables demonstrated some heterogeneity in the spread of residuals. This is likely due to the bivariate distribution possessed by both of these response variables, even after transformation.
Table 6. Mixed effects models of the additive effects of sampling year and gypsy moth (GM) abundance on four characteristics of communities of macrolepidoptera collected from burlap bands on 50 hardwood trees at each of 10 northern temperate forest stands in north-central Ontario, Canada, with different histories of GM outbreak over 2 sampling years (2006 and 2007).

Community structure
Visual interpretation of the ordination plot suggests that much of the variation on the first axis was due to sampling year (Fig. 4). Three sites from 2006 (Loon, Wavy, and Wanapitei), however, are found closer to the sites from 2007 than to sites from their own year; these three sites had the highest gypsy moth abundances in 2006 (Table 4, Fig. 4). Furthermore, although sites with and without gypsy moth history are intermingled in 2007, a cluster of sites on the left of the plot is made up of all 2006 sites with no gypsy moth history as well as the sites with gypsy moth history that had low abundances of gypsy moth in 2006 (Granary and Cutler). Correlations between transformed species abundances and site scores for the first two axes of the ordination indicate that variation along the first axis (i.e., between sampling years) was related to interannual shifts in abundance of C. ilia, forest tent caterpillar, Orthosia rubescens (Walker) (Erebidae: Noctuinae), and to a lesser extent O. leucostigma, M. latex, A. alternata, and Satyrium calanus (Lepidoptera: Lycaenidae). Variation on the second axis was most related to the abundances of A. alternata, M. latex, and secondarily to Lithophane innominata (J.B. Smith) (Erebidae: Noctuinae), and O. rubescens. The final stress of the NMDS was 16.87. The NMDS solution fit the observed Bray-Curtis distances very well (linear R 2 = 0.84).
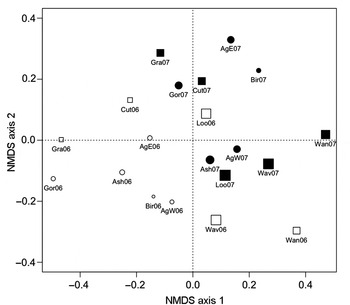
Fig. 4. NMDS ordination of communities of macrolepidoptera collected from burlap bands on 50 hardwood trees at each of 10 northern temperate forest stands in north-central Ontario, Canada, with (squares) and without (circles) histories of gypsy moth outbreak in 2006 (open symbols) and 2007 (solid symbols); the size of the symbols is proportional to the abundance of gypsy moth at each site.
Analysis of dissimilarities detected significant effects of sampling year (P = 0.001) and gypsy moth abundance (P = 0.050) on patterns in community structure when the entire set of species was used as the response matrix (Table 7). However, although the effects of sampling year remained significant (P = 0.001) when forest tent caterpillar was removed from the response matrix, the effect of gypsy moth abundance was no longer significant (P = 0.204). Analyses performed after dividing the community into groups according to larval phenology revealed a marginally significant effect of gypsy moth abundance on late-season species (P = 0.049) but not on early-season species (P = 0.136). When forest tent caterpillar was removed from the early-season species matrix, the response of the community to gypsy moth abundance became even less significant (P = 0.456). The effect of sampling year on community structure in all of these models was very strong (P < 0.01 in all cases). However, none of the models explained more than about a quarter of the variance in community structure (R 2 of the residuals ranged from 0.72 to 0.75).
Table 7. Results of an analysis of dissimilarity procedures testing for the effects of sampling year and log10(gypsy moth abundance) on five different divisions of community structure of macrolepidoptera collected over 2 sampling years at 10 northern temperate forest stands in north-central Ontario, Canada, with (n = 5) and without (n = 5) histories of gypsy moth (GM) outbreak.

Discussion
Current gypsy moth abundance, but not outbreak history, had an impact on native caterpillar communities in this study. We observed a significant increase in gypsy moth abundance from 2006 to 2007, concurrently with a decrease in native caterpillar species abundance, richness, and evenness. Gypsy moth abundance was not significantly related to these reductions in native diversity; however, there was a significant effect of gypsy moth abundance on late-season caterpillar community structure. In contrast, the gypsy moth outbreak history of a study site was not a good predictor of community diversity or structure. This is in contrast to our original hypothesis that sites with differing histories of gypsy moth outbreak would demonstrate differences in native caterpillar communities as a result of long-term ecological impacts (e.g., changes in abundance and species of natural enemies). Instead, the results suggest that the differences observed in the community are due to the presence of a highly abundant folivore and not to permanent shifts in the native community resulting from the introduction of an invasive species.
By 2007, gypsy moth was dominant at many of the study sites, commonly accounting for more than three-quarters of the total number of individuals collected. Temperate macrolepidopteran communities are generally described by a log-normal or log-series species-abundance distribution (Summerville and Crist Reference Summerville and Crist2008) in which a few dominant species represent up to 30% of the sample, while the remainder is comprised of less common species. In our study this is reflected in the rank-abundance curve for sites with no gypsy moth history from the first sampling year: less than a quarter of the community was taken up by the dominant species (Fig. 3). However, the rest of the rank-abundance plots show dominance by gypsy moth of up to 96% of the sample, representing a considerable shift in the dominance structure of the community.
Extreme dominance by one species is common during outbreaks of forest caterpillars (Faeth Reference Faeth, Barbosa and Schultz1987; Mason Reference Mason, Barbosa and Schultz1987), and periodic outbreak populations are a well-studied phenomenon in temperate-forest macrolepidoptera (e.g., Hunter Reference Hunter1991; Berryman Reference Berryman1996). Yet in a field dominated by the study of population dynamics of pest species, research on the community impacts of extremely abundant outbreak species is rare. To our knowledge, no empirical studies exist that can be compared with ours to see whether a reduction in richness and abundance is common in native caterpillar communities dominated by an outbreak species. There is some theoretical and empirical evidence that mechanisms such as indirect competition through induced host-plant defenses (Haukioja and Niemelä Reference Haukioja and Niemelä1979; Hunter Reference Hunter1987; Neuvonen et al. Reference Neuvonen, Hanhimäki, Suomela and Haukioja1988; Dankert et al. Reference Dankert, Herms, Parry, Scriber, Haas and Raman1997; Wold and Marquis Reference Wold and Marquis1997) and changes in the natural enemy community (Eveleigh et al. Reference Eveleigh, McCann, McCarthy, Pollock, Lucarotti and Morin2007) caused by outbreak populations can have a negative impact on non-outbreak species. However, given that non-outbreaking species in natural communities have a long history of coevolution with these occasionally extremely abundant species, less abundant species have probably developed some means of ensuring long-term persistence. Recolonization of affected areas after outbreak collapse is likely to be important for maintaining diversity in communities of temperate-forest Lepidoptera, as it is after other types of disturbance (Hilt and Fiedler Reference Hilt and Fiedler2005; Summerville et al. Reference Summerville, Bonte and Fox2007).
We documented some influence of current gypsy moth abundance on the structure of native caterpillar communities. The cluster of sites seen on the ordination (the only sites in this study with low current gypsy moth abundances) indicated that these sites had caterpillar communities that were more similar to each other than to sites with high gypsy moth populations (Fig. 4). The results of the analysis of dissimilarities also indicate that gypsy moth abundance played a role in structuring native caterpillar communities. When the entire community was analyzed, gypsy moth abundance had a weakly significant influence on community structure; however, this effect was no longer significant when forest tent caterpillar was not included in the response matrix. In this case, the strong positive correlation between gypsy moth and forest tent caterpillar seems to have driven the apparent influence of the invasive species on the native species. However, when the community was split into early- and late-season assemblages it became clear that there was some relationship between gypsy moth abundance and community structure independent of the association between the two dominant species, particularly the structure of the late-season species assemblage.
Our results agree with those of the two other studies that have directly investigated impacts of the gypsy moth on native species. In a survey of adult moth populations in northern Michigan during the first gypsy moth outbreaks in that region, Work and McCullough (2000) concluded that particularly high populations of gypsy moth were correlated with reduced abundances of some late-summer native oak specialists (although, in general, the moth communities were not significantly altered by the presence of the invasive species). Similarly, Sample et al. (1996) found lower abundances of certain species of native caterpillars and moths in areas of heavy gypsy moth defoliation. Although gypsy moth populations were high in these studies, trees were not completely defoliated and any competition between gypsy moth and native species would likely have been indirect. For this reason, the authors of both studies speculated that reductions in native species abundances might be due to feeding-induced reductions in host foliage quality or palatability or to changes in the natural enemy community.
Although gypsy moth was the dominant species at many of our study sites, it removed only a moderate amount of foliage, indicating a possible role for indirect interactions in our study. Our ordination results indicate that O. leucostigma, M. latex, and L. innominata were among the late-season species most affected by gypsy moth. Each of these species is broadly polyphagous and shares a number of host trees with gypsy moth, including all of the dominant species at our study sites (Wagner Reference Wagner2005). In addition, native and introduced parasitoids associated with gypsy moth also attack O. leucostigma (Arnaud Reference Arnaud1978; Krombein et al. Reference Krombein, Hurd, Smith and Burks1979). Parasitoids are not likely to play a major role in the interactions between gypsy moth and native species in the study area (Timms et al. Reference Timms, Walker and Smith2011) and we suggest that investigating host plant mediated interactions between these susceptible species and gypsy moth, as well as the relationship between forest tent caterpillar and the invasive species, would be a fruitful avenue of investigation.
Alternative explanations
Our study was aimed at determining whether or not gypsy moth had an impact on native forest caterpillar communities. However, the large unexplained variation in the data highlights the fact that the diversity and community structure of native forest caterpillars are influenced by a more complex set of variables than simply the historical or current abundances of an invasive species. Factors such as forest structure, stand age, and climate have all been shown to be important in studies of the diversity and composition of forest Lepidoptera (Jeffries et al. Reference Jeffries, Marquis and Forkner2006; Reynolds et al. Reference Reynolds, Ayres, Siccama and Holmes2007; Summerville and Crist Reference Summerville and Crist2008; Savilaakso et al. Reference Savilaakso, Koivisto, Veteli and Roininen2009). In our study the abundances of gypsy moth and forest tent caterpillar exhibited negative relationships with the total basal area of the stands, indicating a possible role for forest structure in this case (Timms Reference Timms2010). Redundancy analysis-based variation partitioning also indicated that gypsy moth abundance did independently explain a small fraction of the variation in caterpillar community structure, although site characteristics were able to explain a larger amount of the observed variation (Timms Reference Timms2010). This supports our conclusion that gypsy moth abundance has small but significant effects on native forest caterpillar communities.
The strong influence of sampling year on all of the response variables is also an important aspect of our results; in particular, the abundance and richness of native species in our study were significantly lower in 2007 than in 2006. Short-term temporal variation is commonly observed in communities of Lepidoptera (e.g., Spitzer et al. Reference Spitzer, Rejmanek and Soldan1984; Summerville et al. Reference Summerville, Bonte and Fox2007) and has been attributed to a variety of causes ranging from annual variation in life-history parameters (Spitzer et al. Reference Spitzer, Rejmanek and Soldan1984) to large-scale abiotic factors (Roy et al. Reference Roy, Rothery, Moss, Pollard and Thomas2001). Spring lepidopterans, in particular, have higher variability in population size than late-summer species, possibly because of variation in population synchrony with their hosts and natural enemies (Forkner et al. Reference Forkner, Marquis, Lill and Le Corff2008). Increased interannual variation in population size caused by indirect effects on fecundity is an unexplored potential impact of gypsy moth on native species that would be interesting to address using long-term data sets.
Not all Lepidoptera are readily sampled using burlap bands; the species observed in this study are therefore only a subset of the total macrolepidopteran community in the area, and we may have missed some of the native caterpillars likely to be affected by gypsy moth. For example, we collected very few saturniids and notodontids. Local saturniid populations are of particular conservation concern because they may be negatively affected by a parasitoid introduced for gypsy moth control (Boettner et al. Reference Boettner, Elkinton and Boettner2000; Kellogg et al. Reference Kellogg, Fink and Brower2003; but see Selfridge et al. Reference Selfridge, Parry and Boettner2007). Gypsy moth had been present throughout the study region for 10–15 years prior to our work and it is possible that our study took place too late to detect effects on vulnerable portions of the community. A lack of baseline data makes this difficult to assess, but studies targeting particular groups could be helpful. Alternatively, it is possible that gypsy moth impacts on the community may take longer to appear, as is suggested by the concept of extinction debt (Kuussaari et al. Reference Kuussaari, Bommarco, Heikkine, Helm, Krauss and Lindborg2009). This issue could be addressed through long-term sampling of the caterpillar communities at our study sites.
Although using burlap-band techniques to sample Lepidoptera has limitations, we believe that it is justified in studies like ours. The types of caterpillars sampled by burlap bands are those that share at least one life-history trait with gypsy moth (the tendency to rest on tree trunks during the day). This has implications for shared parasitism and predation; many parasitoids and other natural enemies locate hosts on the basis of general habitat and search image cues (Godfray Reference Godfray1993). Also, in comparison with other methods of collecting forest caterpillars, burlap bands allow for large sample sizes, produce a large number of individuals per unit effort (Wagner et al. Reference Wagner, Peacock, Carter and Talley1996; Raimondo et al. Reference Raimondo, Strazanac and Butler2004), and are more effective at sampling certain families and groups (Wagner et al. Reference Wagner, Peacock, Carter and Talley1995). These considerations are particularly important when sampling forest caterpillar and other communities where many of the species occur at very low abundances (Summerville and Crist Reference Summerville and Crist2008). Finally, using burlap bands is an inexpensive, uncomplicated, and easily replicated technique.
Conclusions
Invasive species are only one of a number of natural and anthropogenic disturbance factors threatening forest-insect biodiversity. To ensure that conservation efforts are effective, it is necessary to fully understand each threat and how it affects particular insect species and communities. Our research has shown that the presence of a highly dominant invasive species has some impact on the structure of native caterpillar communities. However, these impacts are limited to one portion of the native community and do not seem to be the result of ecological changes caused by the history of the invasive species at a site. Future research identifying the mechanisms involved in these relationships would greatly increase our knowledge of biological invasions and help natural-resource managers to make informed conservation decisions. We suggest that long-term studies in invaded areas would be useful for identifying the changes that occur in native communities over time as invasive species become an established part of local food webs. Our study is the first record of the composition of forest caterpillar communities in north-central Ontario. It encompassed a period when gypsy moth populations were first arriving and increasing in the region, making it an important record of community composition during the early stages of gypsy moth establishment. It would be informative to return to these sites to determine changes that may have taken place. This information would be useful for studies on the impacts of invasive forest insects such as gypsy moth, as well as for assessing the impacts of other disturbances such as climate change or forest-harvesting practices.
Acknowledgements
J. Kaknevicius, D. Maguire, E. Ratsep, S. Walker, and a number of volunteers assisted in specimen collection and processing. V. Nealis, P. Kotanen, C. Darling, and J. Malcolm provided suggestions regarding experimental design and interpretation of the results. We are also grateful to S. Walker, C. Buddle, K. Summerville, and two anonymous reviewers for comments that improved the manuscript. This research was supported by a Natural Sciences and Engineering Research Council of Canada (NSERC) postgraduate scholarship to L.L.T. and NSERC Biocontrol Network and Discovery Grants to S.M.S.
Appendix
Table A1. Taxonomic listing of macrolepidopteran larvae and pupae collected from burlap bands on hardwood trees at 10 northern temperate forest stands in north-central Ontario, Canada. Numbers (per host tree and gypsy moth (GM) outbreak history), species abundance (grand total and overall rank), and collection dates are provided for each species. Host trees are identified as follows: AS, white ash (Fraxinus americana); BI, white birch (Betula papyrifera); M, red and sugar maple (Acer rubrum and A. saccharum); RO, red oak (Quercus rubra L.); PO, trembling aspen and largetooth aspen (Populus tremuloides and P. grandidentata).

*Species that do not feed on leaves, but feed on lichen and (or) detritus.
† Individuals of Catocala spp. that were not identifiable to species or morphospecies were not included in analyses.
‡ Specimens that were not identifiable at least to family level were not included in analyses.