Introduction
Pasture-based milk production systems have been shown to correspond to consumer demands and to be cost-effective (Dillon et al., Reference Dillon, Hennessy, Shalloo, Thorne and Horan2008). However, trends towards increasing herd size and fragmented farming areas often limit the applicability of full-time grazing systems. In such situations, part-time grazing systems with indoor complementation of fresh herbage and concentrate, known as ‘cut-and-carry’ system (Cameron et al., Reference Cameron, Chagunda, Roberts and Lee2018), are of interest. These systems allow to maintain high proportions of fresh herbage in the diet even when the area suitable for grazing is limited.
Exclusive herbage feeding has restrictions regarding energy density and nutrient utilization (Kolver and Muller, Reference Kolver and Muller1998), especially in higher-yielding cows. Particularly, an excess of rumen degradable nitrogen (N) in relation to fermentable energy can enhance environmental pollution (Verite et al., Reference Verite, Remond and Journet1984; Kristensen et al., Reference Kristensen, Søegaard and Kristensen2005). Inefficient N use and increased urinary N excretion are common especially when feeding autumn herbage (Peyraud and Delagarde, Reference Peyraud and Delagarde2013), whereas other seasonal periods might be less detrimental. Intensive grazing systems managed under continuous stocking make use of young herbage which is associated with particularly excessive dietary crude protein (CP) contents (van Vuuren and Meijs, Reference van Vuuren, Meijs, van der Meer, Unwin, van Dijk and Ennik2013). Additionally, young herbage is highly digestible and exclusive grazing may lower the reticulo-ruminal pH to borderline levels (Graf et al., Reference Graf, Kreuzer and Dohme2005) and, following abrupt changes to grass feeding or at high dry matter (DM) intake in mid-lactation, subacute ruminal acidosis may develop (compiled by Rafferty et al., Reference Rafferty, Fahey, Grace, Donaldson, Whelan, Lynch, Pierce and Mulligan2019). In contrast, the fresh herbage fed indoors is usually more mature than intensively grazed herbage and the lower energy density requires extra fermentable energy to meet the cow's demand. Therefore, supplementary feeding is common. It can be assumed that possible trends towards a lower reticulo-ruminal pH from supplemented easily fermentable carbohydrates are compensated by the more mature herbage.
There is a lack of specific full-time and part-time grazing system comparisons across the grazing season. Existing studies focus either on grazing systems v. indoor feeding of conserved feed (Hofstetter et al., Reference Hofstetter, Frey, Gazzarin, Wyss and Kunz2014) or systems using the same herbage quality indoors and on pasture with focus on energy expenditure for grazing activity (Dohme-Meier et al., Reference Dohme-Meier, Kaufmann, Görs, Junghans, Metges, van Dorland, Bruckmaier and Münger2014). These studies also did not address the characteristic challenges of pasture-based moderate-concentrate feeding systems, which include the unfavourably low nitrogen use efficiency (NUE) with its adverse environmental consequences on one hand and the potential challenges of part-time grazing on animal behaviour and ruminal metabolism on the other hand. It is especially unknown whether, or not these challenges are differently severe in these systems and this throughout the grazing period. This is important also to decide how to address best the conflict between maximizing the use of local resources (fresh herbage) and at the same time offering only a few concentrate ingredients to ruminants which may represent human foods (Delaby and Peyraud, Reference Delaby and Peyraud2009). To increase NUE and to reduce losses of easily volatile urine nitrogen of grazing cows, energy supplementation e.g. by maize silage and ground maize was recommended (Almeida et al., Reference Almeida, Dall-Orsoletta, Oziemblowski, Michelon, Bayer, Edouard and Ribeiro-Filho2020) with the disadvantage of feeding possible food to ruminants. Supplementary feeding with food industry by-product-based concentrates, as proposed by Condren et al. (Reference Condren, Kelly, Lynch, Boland, Whelan, Grace, Rajauria and Pierce2019), maybe a way to avoid this conflict.
In this study, therefore, the following hypotheses were tested: (1) the NUE is higher in a part-time grazing system with indoor feeding of fresh herbage and concentrates compared to a full-time grazing system performed under continuous stocking. (2) Seasonal changes such as variable herbage composition and progressing stages of lactation affect NUE and grazing behaviour of grazing dairy cows less in part-time than in a full-time grazing system. (3) In a full-time grazing system, rumination is promoted, and feed intake is more uniform during the day compared to a part-time grazing system with complementary indoor feeding of fresh herbage and concentrates.
Materials and methods
Animals and feeding system
The experiment was conducted in 2015 at the experimental pilot farm of the Vocational Education and Training Centre for Nature and Nutrition Hohenrain (47°10′N, 8°19′E), Switzerland, situated at 620 m a.s.l.
In 2014, 25 and 23 dairy cows had been randomly assigned to two feeding systems: a full-time grazing system with mineral supplementation only (system F) and a part-time grazing system with indoor feeding of fresh herbage plus mineral and concentrate supplementation (system P), respectively. The mineral mix was offered to the system F cows at ad libitum access in a covered cask located near the milking parlour. It contained, per kg, 282 g sodium, 100 g magnesium, 12.2 g zinc oxide, 2.5 g copper sulphate, 156 mg calcium iodate and 50 mg sodium selenite. The concentrate was supplemented individually to system P cows in the amount covering energy requirements for maintenance of the individual plus extra for 24 kg milk/day (Agroscope, 2015) assuming average contents of crude protein (CP) and acid detergent fibre (ADF) of 209 and 234 g/kg DM, respectively, for the fresh herbage. In addition to the vitamin-trace element mix included in the concentrates (Table 1), system P cows received about 120 g of a complementary 4 : 5 mix of mineral feed and sodium chloride feed dispersed on top of the fresh herbage fed indoors. The mineral feed contained, per kg, 110 g calcium, 120 g magnesium, 110 g phosphorus, 900 000 IU vitamin A, 150 000 IU vitamin D3, 2000 mg vitamin E, 5 g zinc oxide, 4 g magnesium (II) sulphate, 1 g copper (II) sulphate, 0.5 g iron (II) sulphate, 100 mg calcium iodate anhydride, 40 mg sodium selenite and 20 mg cobalt carbonate-monohydrate. From each system, six cows were selected for the present experiment: two Brown Swiss and two Swiss Fleckvieh each, as well as either two Holstein-Friesian (system P) or two Holstein Friesian × Jersey crossbreds (system F). These animals had been selected by forming homogeneous groups regarding lactation stage, milk yield and lactation number. At the start of the experiment, the system F and system P cows selected were 70 ± 15 and 51 ± 37 days in milk (means ± standard deviations), produced 23.4 ± 4.4 and 28.4 ± 2.9 kg/day of energy-corrected milk (ECM), weighed 556 ± 46 and 582 ± 70 kg (difference in average owing to using crossbreds in F and Holstein in P), and had calved 3.4 ± 1.5 and 2.0 ± 1.1 times, respectively. The cows stayed with their entire system group during the entire experiment. The two herds grazed on adjoining pastures.
Table 1. Composition of the balanced concentrate offered to the system P cows in spring and summer (n = 6), and of the concentrate with high NEL:APD ratio in summer and autumn (n = 5)
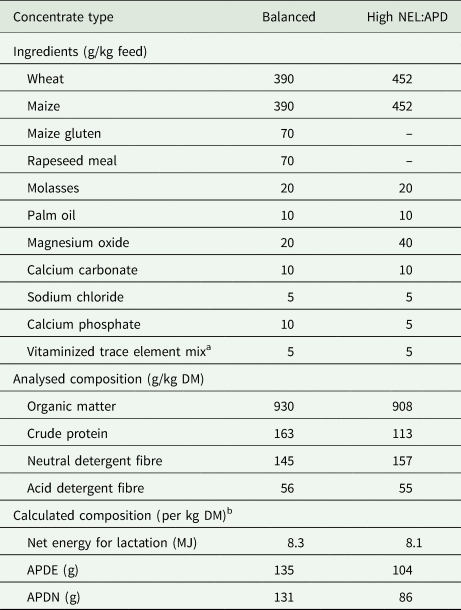
NEL, net energy for lactation; APD, absorbable protein at the duodenum; APDE/APDN, APD calculated as the sum of microbial protein from energy supply and rumen undegradable protein/sum of microbial protein from rumen degradable protein and rumen undegradable protein.
a Contained per kg: 3 mg vitamin A, 0.037 mg vitamin D3, 9 mg vitamin E, 10 mg iron sulphate monohydrate, 10 mg copper sulphate pentahydrate, 20 mg zinc oxide, 50 mg manganese oxide, 0.6 mg iodine, 0.6 mg cobalt carbonate monohydrate, 0.15 mg sodium selenite.
b As indicated by the manufacturer.
The same experimental schedule was maintained throughout the entire experiment. After changing diets from winter indoor feeding to fresh herbage, animals had been on pasture and receiving fresh herbage indoor, 2 weeks before the start of sampling. The experiment included three 15-day sampling periods (one per season) where alkane capsules were administered from day 1 to day 14 and data collection was performed in the last 10 days taking place on 20 April to 4 May (spring), 29 June to 13 July (summer) and 28 September to 10 October (autumn). Botanical composition of pastures (used for grazing) and leys (used for harvesting fresh herbage to be fed indoors) are displayed in Table 2. Pastures and leys were fertilized with 165 and 190 kg N/ha and year, respectively. The pastures were managed under a continuous stocking system on one paddock in spring and two paddocks each in summer and autumn for the system F cows. The average grazing height (cm) was 6.7, 5.6 and 7.1 cm at a daily pasture allowance of 0.23, 0.40 and 0.50 ha per cow in spring, summer and autumn, respectively. System F cows grazed from 07.00 to 17.00 h and from 18.00 to 06.00 h in spring and autumn. In the summer assessment, these periods were restricted to 07.00 to 12.00 h and 18.00 to 06.00 h to minimize heat stress. The pastures grazed by the system P cows were managed under a slightly modified continuous stocking system alternating daily between two paddocks in spring and summer as well as four paddocks in autumn at an average grazing height of 8.4, 8.7 and 10.1 cm and a pasture allowance of 0.08, 0.08 and 0.14 ha per cow in spring, summer and autumn, respectively. During spring and autumn, system P cows grazed from 07.30 to 16.00 h and received fresh herbage indoors from 16.15 to 07.15 h. During summer, system P cows grazed from 07.30 to 10.30 h and additionally received fresh herbage at 12.00 h. The fresh herbage was generally harvested at a more mature growth stage as the grazed swards, aiming at use at the beginning of panicle emergence of plants. After harvesting the herbage, it was supplied directly at the barn, removing refusals after 14 h. The selected cows were milked within their respective system group (system P from 05.15 to 05.45 h and from 16.15 to 16.45 h; system F from 06.15 to 06.45 h and from 17.30 to 18.00 h), all in the same milking parlour. The system P cows received concentrate indoors in portions of <1 kg from an automatic feeding station. The concentrate allowance was adapted to milk yield every 2 weeks. An amount of 0.4 kg concentrate was allocated per kg milk/day exceeding 24 kg/day. The composition of concentrates used is shown in Table 1. During spring a balanced concentrate regarding the ratio of net energy for lactation (NEL) and absorbable protein at the duodenum (APD) relative to requirements for milk production was offered (Agroscope, 2015). In summer, additionally a concentrate with a higher NEL : APD ratio (1 : 1) was offered, whereas in autumn only the concentrate with the higher NEL : APD ratio was used, anticipating a decreasing NEL : APD ratio in fresh herbage in the course of the season.
Table 2. Botanical compositiona of pastures (n = 5) and leys (n = 2) measured in spring, summer and autumn (proportion of total number of plants; arithmetic means and standard deviation)

a Plants representing less than 2% of total number of plants were not listed.
Data recording and sampling
The botanical composition of the experimental pastures and the leys used for harvesting fresh herbage was assessed once per season either within or shortly after (autumn) the sampling weeks following Daget and Poissonet (Reference Daget and Poissonet1969). Climatic parameters were measured in spring, summer and autumn by using a stationary meteorological station (Lufft Opus 200, G. Lufft Mess- und Regeltechnik GmbH, Fellbach, Germany) situated at a distance of <1 km from the pastures.
The cows were weighed after morning milking on a mobile balance placed in the milking parlour on days 5 and 15 of each seasonal period. Milk yield was recorded at each milking in each seasonal period. Milk composition was analysed on 3 days between days 7 and 13 from proportionately pooled evening and morning milk samples. Herbage intake was estimated by the double n-alkane method (Mayes et al., Reference Mayes, Lamb and Colgrove1986; Berry et al., Reference Berry, Scheeder, Sutter, Kröber and Kreuzer2000). From days 1 to 13, 6.5 cm long gelatine capsules with 1.7 cm diameter (Capsula GmbH, Ratingen, Germany; HGK 17-60sl) were administered orally after each milking. The capsules, filled manually, contained 5.00 ± 0.01 g dried apple pomace carrying 100 mg/g of the alkane C32 (n-dotriacontane, C32H66, Minakem Beuvry Production SAS, Beuvry-la-Forêt, France). From days 8 to 14, daily spot samples of faeces from each cow were collected by either spontaneous or stimulated defaecation indoors between 05.00 and 06.30 h. Morning sampling had been found to provide the most accurate intake estimates (Berry et al., Reference Berry, Scheeder, Sutter, Kröber and Kreuzer2000). After mixing, subsamples of 200 and 30 g were stored at −20°C for later drying or analysis of N content in fresh faeces, respectively. Approximately 500 g fresh weight of herbage were collected daily from days 6 to 12. Pasture samples were hand-plucked by two people mimicking the cow's selection (Berry et al., Reference Berry, Scheeder, Sutter, Kröber and Kreuzer2000). Animals were followed while grazing for >10 min between 07.00 and 09.00 h and between 18.00 and 19.00 h (full-time grazing cows only). Samples from individual animals were pooled by system and day. Herbage fed indoors was sampled daily between 16.30 and 17.00 h (grazing period) by sampling randomly at ten spots in the barn with a core sampler, unifying the subsamples to one sample per day. All samples were immediately stored at −20°C until further analysis. Concentrates were sampled at least once per season and stored frozen until further analysis. Individual concentrate intake was recorded daily.
Eating and locomotive behaviour of each cow were recorded on six consecutive days (starting on day 5 and day 6, respectively), using noseband sensors and 3D-accelerometers mounted on the right hind leg (Rumi Watch, Itin + Hoch GmbH, Liestal, Switzerland). Sensors were monitored with the software Rumi Watch Manager 2 (2.0.5.0). Sensor recordings were assigned to the activities of eating, ruminating and idling as well as walking, standing and lying, with the software Rumi Watch Converter (V.0.7.3.2). The Rumi Watch system has been validated against visual observation by Zehner et al. (Reference Zehner, Umstätter, Niederhauser and Schick2017) for rumination and eating behaviour, and by Alsaaod et al. (Reference Alsaaod, Niederhauser, Beer, Zehner, Schuepbach-Regula and Steiner2015) for locomotive behaviour. Cows had been accustomed to the nosebands and accelerometers for at least 1 day in advance.
Reticulo-ruminal pH was measured in 10-min intervals by an indwelling, wireless data recording and transfer system (smaXtec animal care GmbH, Graz, Austria; Gasteiner et al., Reference Gasteiner, Guggenberger, Häusler and Steinwidder2012). Loggers were administered to the cows on day 2 in spring (recording in spring and summer, where time after administration was still within the 90-day period where the producer states an accuracy of ± 0.2 in pH) and in autumn. The data recorded was transferred to a receiving station installed in the milking parlour and transmitted to a server. Means per hour and day were calculated over a 7-day period.
Laboratory analyses
Previously frozen herbage samples and subsamples of faeces were dried in a ventilated oven at 60°C for 48 h and milled through a 1-mm screen. The faeces samples were pooled per animal and season. The seven herbage samples per season and system were analysed individually to determine the alkane contents, whereas the seven herbage samples were pooled to three samples per season and system across days 6−12 for analysis of proximate contents and gross energy following the Association of Official Analytical Chemists (AOAC) (1995). The other feeds and the faeces samples were subjected to these analyses as well. DM and total ash were analysed with a thermo-gravimetric device (model TGA-500, Leco, St. Joseph, MI, USA), and N (faeces: non-dried) (AOAC no. 968.06) on a C/N analyser (Leco Analyser Type CN 2000, Leco Corporation, St. Joseph, MI, USA). Feed was also analysed for (ash-corrected) contents of neutral (NDF) and acid detergent fibre (ADF) (AOAC no. 973.18) using the Fibertec System M (Tecator, 1020 Hot Extraction, 1021 Cold Extraction, Flawil, Switzerland). Heat-stable α-amylase was used for NDF analysis, but no sodium sulphite (van Soest et al., Reference van Soest, Robertson and Lewis1991). A bomb calorimeter device (Calorimeter System C700 with Cooler C7002, IKA, Staufen, Germany) was used to determine the gross energy contents. Feeds and faeces were analysed for n-alkanes (C31, C32 and C33), as described by Berry et al. (Reference Berry, Scheeder, Sutter, Kröber and Kreuzer2000), on a gas chromatograph (HP-6890, Hewlett Packard, Waldbronn, Germany) equipped with a flame ionisation detector and an SPB-1 column from Supelco (Buchs, Switzerland). Milk gross compositional analysis was performed on a MilkoScan FT6000 (Foss, Hillerød, Denmark) at Suisselab AG (Zollikofen, Switzerland).
Calculations and statistical analysis
Feed and faecal CP was calculated as 6.25 × N. Contents of APD in herbage were differentiated into rumen non-degradable protein plus microbial protein either from energy supply (APDE) or from rumen degradable protein (APDN) (Agroscope, 2015). The content of APD was calculated based on measured CP content, calculated degradability of the CP and calculated fermentable organic matter. The content of NEL was calculated from the calculated contents of digestible organic matter and digestible CP via equation as described by Agroscope (2015) for unknown herbage composition. The ECM (kg) was calculated according to Agroscope (2015) as [(0.038 × fat (g/kg) + 0.024 × protein (g/kg) + 0.017 × lactose (g/kg)) × kg milk]/3.14. For the calculation of DM intake (DMI) and faecal output from alkane measurements, the equations proposed by Mayes et al. (Reference Mayes, Lamb and Colgrove1986) were applied. Alkane concentrations determined in faeces were corrected using the recovery rates identified by Berry et al. (Reference Berry, Scheeder, Sutter, Kröber and Kreuzer2000). As the six system P cows were kept in a larger cow herd on this treatment, the amounts of fresh herbage fed indoors and herbage from pasture could not be quantified separately via the n-alkane method. To estimate total herbage and N intake of system P cows, the alkane content and the chemical composition of the herbage fed indoors was used.
Nitrogen intake was calculated from the estimated DMI and the corresponding N content of the entire diet. Faecal N output was estimated using the results from the alkane method and faecal N content. Urine N output was estimated assuming 26 g N/kg of body weight (BW) change (Gibb et al., Reference Gibb, Ivings, Dhanoa and Sutton1992; Estermann et al., Reference Estermann, Wettstein, Sutter, Erdin and Kreuzer2003). Milk N output was calculated from milk yield and milk protein content. To account for initial system group differences in BW, all intake-related variables were statistically analysed and presented per 100 kg of BW.
Statistical analyses were performed with R (R Core Team, 2017) using the packages lme4, lmerTest and lsmeans for the data analysis of linear mixed models. Figures were produced using the package ggplot2. The following random intercept Model 1 was used for the statistical evaluation of all variables except behavioural traits:

where the grazing system (G; full-time and part-time), season (S; spring, summer, autumn) and their interaction (G × S) were considered as fixed effects, and animal (A) was set as a random intercept. The breed was not considered due to the small number of representatives per system, but differences were considered by equal allocation to the system. Model 2, used for the behavioural characteristics, additionally included day within season and system as a random intercept. Model assumptions for analysis of variance were inspected graphically. If P < 0.05, effects were considered as statistically significant, and if 0.05 ≤ P < 0.10, effects were considered as trends. The chemical composition of fresh herbage was analysed with Model 3 using a linear model with the season, herbage type (pasture v. indoor herbage) and their interaction as fixed effects. Multiple comparisons among the least-square means were performed by contrast analysis considering P < 0.05 as significant. Due to health problems, one system P cow was excluded from all statistical analyses and one system F cow was excluded for the analyses in spring. Noseband sensor data from one cow per system were missing due to misfit of the noseband or injury. For these animals only pedometer data were available.
Results
Sward composition and climate
The leys used for the production of herbage fed indoors had a lower proportion of grasses and a higher proportion of legumes than the pastures (Table 2). The pasture herbage, collected when accompanying the cows of the two system groups, had similar contents of organic matter, CP, NDF and ADF (data not shown). In contrast, the fresh herbage fed indoors had lower CP and NDF contents than the pasture herbage (Table 3). The extent of the differences varied to some extent among seasons (interactions, both P < 0.01). The NEL content of the herbage fed indoors was lower by 0.35 MJ/kg DM than that of the pasture herbage across all seasons. It was lowest in energy in summer, and it had a lower APDN-to-APDE ratio than the pasture herbage in spring and autumn (data not shown).
Table 3. Chemical composition (per kg of DM) of the pastures (‘Pasture’, n = 6 per period) and indoor fed herbage (‘Indoor’, n = 3 per period) during the grazing season

DM, dry matter; OM, organic matter; CP, crude protein; NDF, neutral detergent fibre; ADF, acid detergent fibre; NEL, net energy for lactation; APD, absorbable protein at the duodenum; APDE/APDN: cf. footnote to Table a,b,c Least-square means carrying no common superscript differ significantly within type at P < 0.05 (tested by contrast comparison).
y,zLeast-square means carrying no common superscript differ significantly within season at P < 0.05 (tested by contrast comparison).
1Calendar weeks 17/18, 27/28, and 38/39, respectively.
2d.f. = calculated by Satterthwaite's approximation for degrees of freedom.
3Per kg of wet weight.
4Calculated based on regressions for unknown herbage composition (Agroscope, 2015).
5Presented as arithmetic means.
In the spring measurement period, the ambient temperature was 12.5 ± 2.6°C (mean ± standard deviation), relative humidity was 75.7 ± 17.2% and precipitation was 10.2 ± 38.8 mm (data not shown in table). The corresponding climate data measured in summer were 24.3 ± 3.5°C, 61.6 ± 7.2% and 1.0 ± 2.3 mm, and those obtained in autumn were 11.2 ± 1.9°C, 86.3 ± 8.1% and 1.2 ± 2.4 mm.
DMI and animal performance
In spring, the herbage DMI per 100 kg of BW was higher (P < 0.05) by 35% in system F compared to system P, whereas this was not the case in summer and autumn (interaction, P = 0.003; Table 4). Across seasons, there was only a weak trend (P = 0.097) for a higher herbage DMI per 100 kg of BW in system F compared to system P. Concentrate DMI of the system P cows was 3.5, 2.9 and 1.0 kg/day in spring, summer and autumn, respectively. Total DMI per 100 kg of BW did not differ between systems but was lower (P < 0.05) in autumn compared to summer by 0.27 kg DMI/100 kg BW across systems. Across the seasons, the system F cows tended (P = 0.085) to be lighter by 72 kg on average than the system P cows. However, the BW of the cows developed differently in the two systems over the seasonal periods investigated. Accordingly, an increase (P < 0.05) was observed from summer to autumn in system F cows, whereas this happened in system P cows from spring to autumn (system × season, P = 0.009). The BW was highest in autumn in both systems (season effect, P < 0.001). BW-corrected daily yields of ECM, milk protein and fat were highest in spring (P < 0.001), and decreased from spring to summer by 29, 31 and 28%, respectively. Feed efficiency (kg ECM/kg total DMI) did not differ between the systems. It was highest (P < 0.05) in spring at 1.79 kg ECM/ kg total DMI.
Table 4. Feed intake, performance, reticulo-ruminal pH and behaviour of the cows of the two pasture-based systems in different periods of the grazing season

a,b,cLeast-square means carrying no common differ significantly within grazing system at P < 0.05 (tested by contrast comparison).
y,zLeast-square means carrying no common differ significantly within season at P < 0.05 (tested by contrast comparison).
1d.f. = calculated by Satterthwaite's approximation for degrees of freedom.
2Assessed according to Edmonson et al. (Reference Edmonson, Lean, Weaver, Farver and Webster1989).
Reticulo-ruminal pH
Across seasons, the daily average reticulo-ruminal pH did not differ between systems (Table 4). This was different within the seasons. System P cows had the lowest pH in spring, whereas in system F cows pH was lowest in summer (interaction, P < 0.001). During the daytime, a continuous pH decline was observed for system F cows, whereas in system P cows, the pH decreased markedly after they had received fresh herbage indoors, especially in spring and autumn, where fresh herbage was offered only once per day (Fig. 1). In summer the pH decline was less abrupt and less pronounced. The daily minimum pH differed among seasons (P < 0.001), and the difference between systems was greater in spring and autumn than in summer (interaction, P < 0.001; Table 4). The range in average pH (min to max) was particularly broad for system F cows in spring.

Fig. 1. Reticulo-ruminal pH in the course of the day for lactating cows in the full-time grazing system and the part-time grazing system with complementary indoor feeding of fresh herbage and concentrate measured in spring, summer and autumn (arithmetic means ± standard deviation). The grey shaded area is the period when the part-time grazing cows had access to fresh herbage indoors. The full-time grazing cows did not receive fresh herbage indoors.
Feeding and locomotive behaviour
On average, the system F cows spent more (P = 0.027) time eating than system P cows (697 v. 611 min/day) (Table 4). All variables describing rumination behaviour per day were affected (P < 0.001) by season, but not by system. The animals ruminated most intensively in summer and least in autumn (rumination time, number of ruminating mastication and boli per day: 464 v. 375 min, 29 v. 23 × 103 and 501 v. 383 in summer and autumn, respectively; P < 0.05). Also, rumination chews per bolus were affected by season (P < 0.05), with a higher number of chews per bolus (P < 0.05) in summer compared to autumn. Within seasons, system differences differed in extent (interaction, P = 0.006). Rumination chews per bolus remained constant for system P cows whereas the number of chews slightly increased for system F cows in autumn compared to summer (interaction, P = 0.006). System F cows spent walking twice as long (P < 0.001) as the system P cows (104 v. 49 min/day). In turn, system P cows spent more time lying (P = 0.028) than system F cows (585 v. 481 min/day). In autumn, for cows in systems F and P, lying time was longer ( + 85 and + 110 min, respectively; P < 0.05) and standing time was shorter (−78 and −110 min, respectively; P < 0.05) than in summer (season, P < 0.001).
Nitrogen balance
Despite the presence of interaction (P = 0.048), no significant difference in total dietary N intake per 100 kg BW between system group means within the three seasons was observed (Table 5). However, total dietary N intake was higher (P = 0.018) by 25% in system F cows than for system P cows (89.0 v. 70.9 g N/day consumed per 100 kg BW, respectively). The N intake from herbage per 100 kg BW was greater (P = 0.001) for system F cows as for system P cows; this in spring and autumn and across all seasons (system, P = 0.001). The N intake from herbage increased (P < 0.05) by 30% between spring and autumn in system P cows, whereas the herbage N intake for system F cows only weakly changed (interaction, P = 0.009). The estimated faeces N output per 100 kg BW remained constant with the season for system P cows, whereas N output via faeces was lower for system F cows (P < 0.05) by 18% in summer compared to autumn (interaction, P < 0.001). Urinary N excretion (g/100 kg BW and day) was calculated to be higher (P < 0.05) in summer and autumn (40.0 and 45.7, respectively) compared to spring (21.3) (P < 0.001). It was greater (P = 0.001) for system F cows than for system P (43.3 v. 28.0 g N/100 kg BW and day). The N output via milk protein, corrected for BW, was higher (P < 0.05) in spring than summer and autumn (25.2 v. 17.2 and 16.2 g/100 kg BW and day) in both systems. In both systems, NUE was higher (P < 0.05) in spring compared to summer and autumn. In spring, the NUE was also higher (P < 0.05) in system P than system F cows, whereas this was not significant in summer and autumn (interaction, P = 0.033). Milk urea N (MUN) content was overall higher (P < 0.001) in system F than system P cows (22.4 v. 11.9 mg/dl). Along with the increase (P < 0.001) in MUN taking place from spring to autumn, this system difference increased (interaction, P < 0.001).
Table 5. Nitrogen balance of the cows of the two pasture-based systems in different periods of the grazing season

BW, body weight.
a,b,cLeast-square means carrying no common differ significantly within grazing system at P < 0.05 (tested by contrast comparison).
y,zLeast-square means carrying no common differ significantly within season at P < 0.05 (tested by contrast comparison).
1d.f. = calculated by Satterthwaite's approximation for degrees of freedom.
2Estimated based on the assumption that urine N = N intake – milk N – faecal N – N in BW change and assuming ± 26 g N/kg BW change as calculated by Estermann et al. (Reference Estermann, Wettstein, Sutter, Erdin and Kreuzer2003).
Discussion
Feed quality, dry matter intake and performance
Overall, the fresh herbage used in the present experiment had a relatively high quality. Herbage composition showed the typical changes along the grazing period where fibre contents increase in summer and CP in autumn (Delagarde et al., Reference Delagarde, Peyraud, Delaby and Faverdin2000). Therefore, the evaluation periods chosen were suitable to reflect the different seasons occurring in temperate climates. The leys had higher proportions of legumes compared to pasture swards, especially in spring and summer, which is typical for temporary grassland using mixed swards. Despite that, the fresh herbage fed indoors had lower CP and NDF contents and increased ADF contents (only in summer). The differences resulted most likely from the more mature growth stage of the herbage used for feeding indoors and less so from the respective botanical composition. Using fresh herbage with different botanical composition and growth stage contributed to the system difference typical for farm practice, but their effects on the variables investigated cannot be separated from other factors of influence.
To estimate DMI, the double n-alkane method was used, which was repeatedly considered suitable for this purpose before (Malossini et al., Reference Malossini, Bovolenta, Piasentier, Piras and Martillotti1996; Reeves et al., Reference Reeves, Fulkerson, Kellaway and Dove1996; Smit et al., Reference Smit, Taweel, Tas, Tamminga and Elgersma2005; Pérez-Ramírez et al., Reference Pérez-Ramírez, Peyraud and Delagarde2012; Vance et al., Reference Vance, Ferris, Elliott, Hartley and Kilpatrick2013). The present estimates of DMI were lower than expected when compared to the amounts theoretically necessary to cover NEL requirements for actual milk production and maintenance (Agroscope, 2015; data not shown). Nevertheless, similar intake levels were estimated by the double n-alkane method for grazing dairy cows by Vance et al. (Reference Vance, Ferris, Elliott, Hartley and Kilpatrick2013), Munoz et al. (Reference Munoz, Letelier, Ungerfeld, Morales, Hube and Perez-Prieto2016) and Heublein et al. (Reference Heublein, Dohme-Meier, Südekum, Bruckmaier, Thanner and Schori2017). In contrast, authors from studies performed under rotational grazing and a high herbage allowance (Dohme-Meier et al., Reference Dohme-Meier, Kaufmann, Görs, Junghans, Metges, van Dorland, Bruckmaier and Münger2014; Hofstetter et al., Reference Hofstetter, Frey, Gazzarin, Wyss and Kunz2014; Munoz et al., Reference Munoz, Letelier, Ungerfeld, Morales, Hube and Perez-Prieto2016) reported a higher herbage DMI per 100 kg metabolic BW ( + 1.2 kg DMI per 100 kg BW on average). The effect of grazing systems on DMI is controversially discussed (Pulido and Leaver, Reference Pulido and Leaver2003; Briske et al., Reference Briske, Derner, Brown, Fuhlendorf, Teague, Havstad, Gillen, Ash and Willms2008). Still, it could be assumed that, under the conditions of the present experiment, a higher DMI could have been achieved when applying a rotational grazing system. When corrected for BW, total DMI, milk yield and feed efficiency was similar in the two systems tested. This shows that the assumingly positive concentrate effect on absolute milk yield in this study was not real but resulted from a higher total intake owing to a higher BW of the system F cows. As BW related DMI did not change over the season, but BW of the system P cows increased over the season (and thus with progressing lactation), the increase in DMI was obviously mostly used for BW gain. In system F, the omission of supplementary feeding did not result in a reduced BW corrected milk yield, but in a lower BW compared to supplemented cows. Together, this may explain why no system differences were found in feed efficiency calculated as kg DMI per kg ECM and per kg BW. The seasonal changes in performance were confounded with the progressing stage of lactation, with the latter likely being the main cause for the decline in milk yield from spring to autumn. Consistent with this outcome, and corresponding to the highest feed quality, feed efficiency was best in spring.
Feeding and locomotive behaviour as well as reticulo-ruminal pH
In accordance with Bargo et al. (Reference Bargo, Muller, Kolver and Delahoy2003), Dohme-Meier et al. (Reference Dohme-Meier, Kaufmann, Görs, Junghans, Metges, van Dorland, Bruckmaier and Münger2014) and Heublein et al. (Reference Heublein, Dohme-Meier, Südekum, Bruckmaier, Thanner and Schori2017), the indoor feeding of fresh herbage and concentrate resulted in a substantial reduction in grazing time, indicating a lower need and thus motivation to graze. As the concentrate level was low compared to levels reviewed by Bargo et al. (Reference Bargo, Muller, Kolver and Delahoy2003), the herbage feeding indoors likely had an additional substantial effect in substituting pasture grass intake. In this case, also less time is required for walking in search of feed and eating bouts might be larger compared to those when grazing on areas of low sward height typical for intensive continuous stocking. The large difference in walking time between system P and system F cows (about twice as long), and the lack of differences in standing time indicate that system P cows consumed feed very fast indoors. Related to the critical limit of 40% of the time spent lying (Sambraus, Reference Sambraus1978), lying times of system F cows were short, especially in spring (30% of total time). This might have been the consequence of the long time needed for grazing in this system.
Concerning reticulo-ruminal pH, several studies (i.e. Graf et al., Reference Graf, Kreuzer and Dohme2005, Al Ibrahim et al., Reference Al Ibrahim, Gath, Campion, McCarney, Duffy and Mulligan2012, Rafferty et al., Reference Rafferty, Fahey, Grace, Donaldson, Whelan, Lynch, Pierce and Mulligan2019) suggested that fast eating of forages followed by long nocturnal periods without feed intake may depress pH to values lower than those found under a more constant eating pattern. However, the diurnal pH dynamics registered in this study (Fig. 1) more reflect the continuous herbage intake of system F cows with a continuous pH decrease during access to pasture. For system P, the decline begins after cows had access to fresh herbage indoors, especially when fresh herbage was supplied only once per day (spring and autumn). This also suggests that the main intake phase in these cows happened indoors and not on pasture. The time spent ruminating, rumination mastication and number of boli were similar between systems thus excluding the presence of indirect effects on pH though influences on rumination. Consistent with this, Heublein et al. (Reference Heublein, Dohme-Meier, Südekum, Bruckmaier, Thanner and Schori2017) reported similar ease of bolus formation and swallowing for non-supplemented and supplemented cows. The intensity of rumination was higher in summer compared to spring and autumn. At similar intake, the high fibre content of summer herbage may have been the cause for this observation.
Nitrogen balance
As expected, the diet consumed by cows grazing full-time was calculated to contain more CP (227 g/kg DM on average) than that of the cows grazing part-time (174 g CP/kg DM). Together with the lower absolute daily milk protein yield, this resulted in a lower NUE in system F than system P cows which confirms previous reports (Mulligan et al., Reference Mulligan, Dillon, Callan, Rath and O'Mara2004; Hoekstra et al., Reference Hoekstra, Schulte, Struik and Lantinga2007). The excessive CP ingested by the system F cows was excreted mainly via urine which was reflected by a concomitantly increased MUN content, confirming its role as an important tool to monitor protein nutrition of dairy cows (Hof et al., Reference Hof, Vervoorn, Lenaers and Tamminga1997). On an average, the MUN of the system F cows was twice as high as that of the system P cows, whereas differences in estimated urine N excretion were smaller. When put into a greater context of annual farm-gate nitrogen balances of the same farm (Akert et al., Reference Akert, Dorn, Frey, Hofstetter, Berard, Kreuzer and Reidy2020), the difference in NUE between system F (0.33) and system P (0.50) was even more pronounced than found in this study. Different from that, on commercial dairy farms, no such differentiation was found as farms practicing system P showed a much higher fertilizer N input than those applying system F. In spring, a high NUE (above 250 g/kg) was found in both grazing strategies in this study. Levels of NUE in the range of 230–300 g/kg were also reported by Miller et al. (Reference Miller, Moorby, Davies, Humphreys, Scollan, MacRae and Theodorou2001) for herbage-based zero-grazing systems with differing grass quality and moderate concentrate supplementation. Different CP levels in concentrate at a relatively high concentrate supplementation rate of 7 kg/day did not increase the NUE (around 260 g/kg; Hynes et al., Reference Hynes, Stergiadis, Gordon and Yan2016). The low NUE in summer/autumn (about 200 g/kg) was manifested in increased calculated urine N excretion levels and MUN contents compared to spring. These changes were expected for autumn with the highest CP content of the diet (system P: 184 g/kg DM; system F: 256 g/kg DM) at low milk N excretion, but not for summer. On a closer look, the high ADF content and, thus presumed, a low soluble carbohydrate content of the summer herbage could have prevented a better N utilization by the rumen microbes.
Conclusion
The investigated common part-time grazing system with indoor complementation of fresh herbage and concentrate resulted in clear differences in intake, reticulo-ruminal pH, locomotive behaviour and a higher NUE (confirming hypothesis 1) compared to the full-time grazing system, and this differently across the grazing period. Consistent with hypothesis 2, NUE was higher in the part-time grazing system, especially in spring, which made better use of fresh herbage along with a limited N intake and excretion compared to the full-time grazing system. On the other hand, cows could be similarly supplemented with energy during milking in the full-time grazing system. This would be particularly efficient in periods with high CP surplus of the pasture grass. Confirming hypothesis 3, grazing fresh herbage over an extended period of the day instead of feeding indoors a large amount of fresh herbage at once can prevent pronounced declines in reticulo-ruminal pH. Management measures counteracting this limitation of the part-time grazing system might consist of offering the fresh herbage in the barn in more than one portion. Although this study is based on a limited number of experimental units, the large variations found between systems in different seasons illustrate that the efficiency of pasture-based milk production systems is highly dependent on the stages of grazing season and lactation. In order to develop recommendations to improve systems, further studies with a larger number of cows and under different climatic conditions are required to further confirm the present findings.
Acknowledgements
The authors are grateful to H.J. Frey, J. Estermann and M. Käch (BBZN Hohenrain), and C. Kunz, M. Mergani and P. Stirnemann (ETH Zurich) and L. Kneubühler (HAFL, Zollikofen). The authors are also grateful to Christoph Kopp for the statistical advice.
Financial support
This study was funded by the Swiss Commission for Technology and Innovation, the Swiss Milk Producers and the Federal Office for Agriculture.
Conflict of interest
The authors declare no conflicts of interest.
Ethical standards
The experiment carried out in the current study is covered by the Lucerne Cantonal Veterinary Office approval number LU 02/15.