1. Introduction
The Mesozoic geology of ENE China is characterized by widespread magmatic rock assemblages, rift basins with sedimentary–volcanic rock sequences and crustal- to lithospheric-scale oblique-slip fault systems with both transtensional and transpressional kinematics (Fig. 1; Ren et al. Reference Ren, Tamaki, Li and Zhang2002; Meng, Reference Meng2003; Wu et al. Reference Wu, Lin, Wilde, Zhang and Yang2005; Zhang et al. Reference Zhang, Zhao, Zheng and Dai2010, Reference Zhang, Chen, Yu, Dong, Yang, Pang and Bat2011, Reference Zhang, Chen, Batt, Dilek, A, Sun, Yang, Meng and Zhao2015; Wang et al. Reference Wang, Zheng, Zhang, Zeng, Donskaya, Guo and Li2011). The distribution and the regional orientation of all these geological features collectively form a strong NNE–SSW-aligned tectonic fabric, superimposed on the Precambrian geology and the ESE-running Qinling–Dabie Orogenic Belt, which developed as a result of the continental collision between the Yangtze Craton (YC) and North China Craton (NCC) during Triassic time. The most extensive magmatic rock assemblages in this region formed during Late Jurassic (165–158 Ma) and Early Cretaceous (132–118 Ma) time.
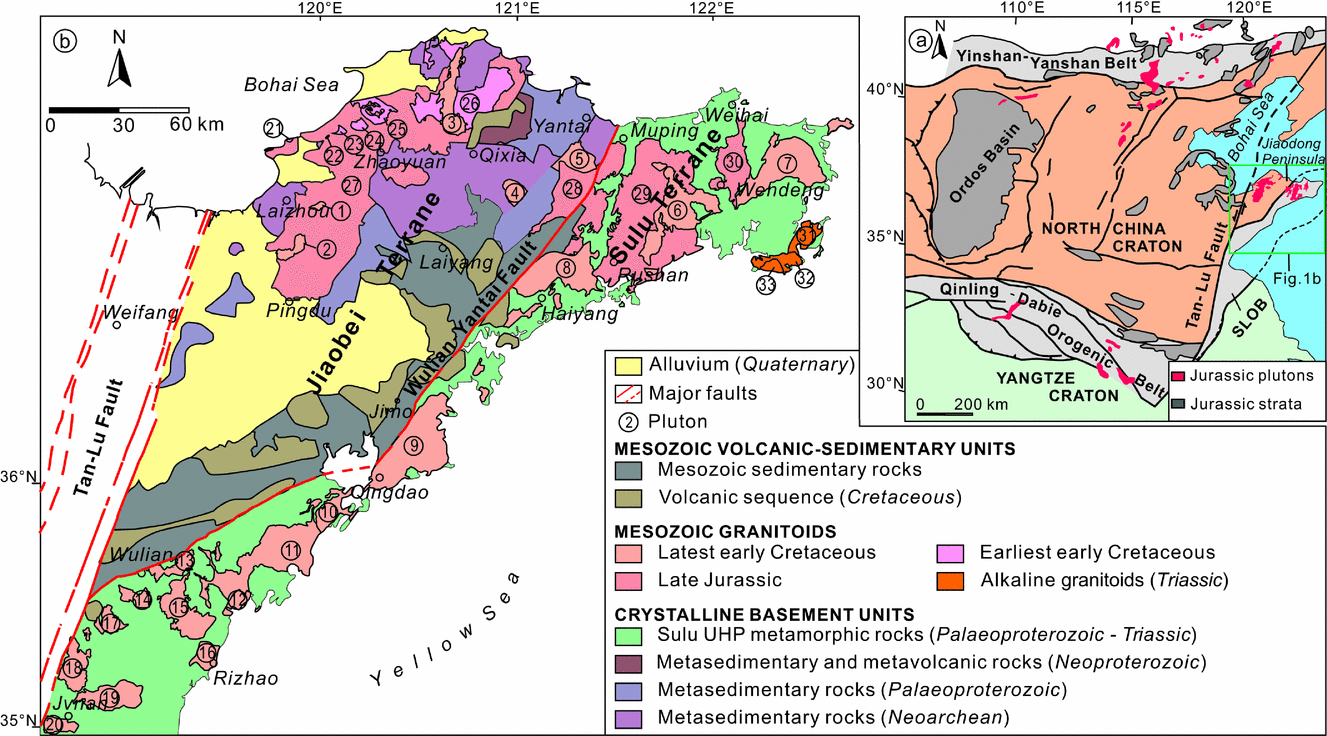
Figure 1. (a) Simplified tectonic map of the Jiaodong Peninsula. Abbreviations: NCC – North China Craton; SLOB – Sulu Orogenic Belt; WYF – Wulian–Yantai Fault; YC – Yangtze Craton. (b) Geological map of the Jiaodong Peninsula, showing the distribution of the Precambrian crystalline basement and Triassic UHP metamorphic rocks, Cretaceous sedimentary–volcanic rift sequence and Mesozoic granitoids. Latest early Cretaceous granitoids: 1, Dazeshan; 2, Gushan; 3, Aishan; 4, Yashan; 5, Yuangezhuang; 6, Sanfoshan; 7, Weideshan; 8, Zhaohushan; 9, Laoshan; 10, Xiaozhushan; 11, Dazhushan; 12, Heshan; 13, Shiwaya; 14, Shichang; 15, Wulianshan; 16, Shijiu; 17, Qizishan; 18, Dadian; 19, Dashan; 20, Banquan. Earliest early Cretaceous granitoids: 21, Sanshandao; 22, Xincheng; 23, Shangzhuang; 24, Congjia; 25, Beijie; 26, Guojialing. Late Jurassic Linglong granitic suite: 27, Linglong; 28, Queshan; 29, Kunyushan; 30, Wendeng. Late Triassic Shidao alkaline complex: 31, Jiazishan; 32, Xingjia; 33, Chashan.
The Late Jurassic granitic intrusions display a strong deformation fabric represented by a predominantly NNE–SSW-aligned mylonitic foliation and ESE–WNW-trending stretching lineations, consistent with ESE–WNW-directed crustal extension in the region (Fig. 2). Generally NNE–SSW-striking, undeformed Early Cretaceous mafic dyke swarms cross-cut this extensional fabric in the granitic plutons, indicating that much of the extensional ductile deformation must have taken place during Late Jurassic time (Deng & Wang, Reference Deng and Wang2016). A large number of NNE–SSW-oriented metamorphic core complexes with middle to lower crustal rocks also indicate that high-magnitude crustal exhumation was part of this regional extension. These structural and spatial relationships suggest that regional extension developed concomitantly with the Late Jurassic granitic magmatism. This inferred tectonic scenario is analogous to those of other extremely attenuated continental domains, where large-scale extension and magmatism kept pace with each other in time and space (Calzia & Rämö, Reference Calzia and Rämö2000; Dilek & Whitney, Reference Dilek and Whitney2000; Dilek, Altunkaynak & Oner, Reference Dilek, Altunkaynak, Oner, Ring and Wernicke2009; Dilek & Sandvol, Reference Dilek, Sandvol, Murphy, Keppie and Hynes2009; Murray, Busby & Verde Ramírez, Reference Murray, Busby and Verde Ramírez2015). The driving force for this syn-extensional magmatism and the melt source and magmatic evolution of the granitic plutons in East China have been a topic of debate and recent investigations. Part of this strong interest in the Late Jurassic granites stems from the fact that their distribution is spatially associated with some of the largest gold deposits in mainland China (Fig. 1; Deng et al. Reference Deng, Yang, Ge, Wang, Zhang, Gao, Zhou and Jiang2006, Reference Deng, Wang, Yang, Zhou, Gong, Yuan, Xu, Guo and Liu2008; Guo, Santosh & Li, Reference Guo, Santosh and Li2013; Yang et al. Reference Yang, Deng, Goldfarb, Zhang, Gao and Wang2014a, Reference Yang, Deng, Guo, Wang, Li and Li2016a, Reference Yang, Deng, Wang, Guo, Li, Groves, Danyushevskiy, Zhang, Zheng and Zhaoc; Deng & Wang, Reference Deng and Wang2016).

Figure 2. (a) Detailed structural map, and (b) cross-section of the Linglong granites in the NW Jiaodong Peninsula. Abbreviations: SSDF – Sanshandao Fault; JJF – Jiaojia Fault; HXF – Hexi Fault; HJF – Houjia Fault; LDF – Linglong Detachment Fault. Extensional deformation structures displayed by the granitic rocks include: N–S-oriented foliation-mylonitic fabric, WNW–ESE-trending stretching lineation, NE–SW-striking and ESE-dipping low-angle detachment fault, and brittle normal faults. Sample locations with the available geochronology data are also shown. Structurally controlled gold deposit sites are highlighted by yellow circles.
Several overarching questions remain regarding this Late Jurassic granitic magmatism in ENE China: (1) the nature of the tectonic setting and the geochemical affinity of this granitic magmatism; and (2) whether the Late Jurassic magmatism was responsible for continental extension by thermally weakening the crust or, if the regional extension was the main driver, facilitating a heat source and partial melting leading to magmatism. Some of the current models envision a post-collisional origin of the Late Jurassic granitic plutons, suggesting that partial melting of the overthickened crust beneath the NCC and the Triassic orogenic belt might have produced the necessary magmas (Hou et al. Reference Hou, Jiang, Jiang, Ling and Zhao2007; Zhang et al. Reference Zhang, Zhao, Zheng and Dai2010; Yang et al. Reference Yang, Fan, Santosh, Hu, Wilde, Lan, Lu and Liu2012, Reference Yang, Santosh, Shen and Li2014b). Although the nature and the compositions of the partially melted crust vary in these models, the implicit assumption is that the tectonic collapse of a collision-produced, overthickened crust and its anatectic melting must have caused the magmatism and the extensional deformation during Late Jurassic time. However, this post-collisional magmatism model is not compatible with the spatial and temporal features of the Late Jurassic magmatism in ENE China and with other well-documented examples of post-collisional magmatic events in different orogenic belts (Dilek & Moores, Reference Dilek and Moores1999; Zhu et al. Reference Zhu, Sun, Gu, Ogasawara, Jiang and Honma2001; Wang et al. Reference Wang, Chung, O'Reilly, Sun, Shinjo and Chen2004; Dilek, Reference Dilek, Dilek and Pavlides2006; Dilek & Altunkaynak, Reference Dilek and Altunkaynak2007; Seltmann et al. Reference Seltmann, Konopelko, Biske, Divaev and Sergeev2011; Laurent et al. Reference Laurent, Janousek, Magna, Schulmann and Mikova2014). Granitic plutons in ENE China are much more extensive in their areal coverage beyond the collision zone and occur at an almost perpendicular angle to it, and their emplacement time is almost 60–80 Ma after the latest Triassic collision in the region.
In this study we have examined one of the largest Late Jurassic granitic plutons, the Linglong granites, in the Jiaodong Peninsula in order to document their compositional and geochemical characters, crystallization ages, melt source and petrogenesis, and the tectonic boundary conditions that played a significant role in controlling the nature of this magmatic event. We present new geochronological data (LA-ICP-Ms U–Pb zircon dating results), whole-rock major- and trace-element geochemistry and whole-rock Sr–Nd and in situ zircon Hf isotope data from the Linglong granites to better constrain the mode, tempo and petrogenetic-geochemical evolution of the Late Jurassic magmatism within the framework of regional tectonics.
2. Basement geology and the Mesozoic magmatic record of the Jiaodong Peninsula
The crystalline basement in the Jiaodong Peninsula includes parts of the Jiaobei and Sulu terranes, which are bounded by the NE–SW-oriented, oblique-slip Wulian–Yantai Fault that marks the suture between the YC and the NCC in the east (Fig. 1; Jiang et al. Reference Jiang, Chen, Guo and Chang2012; Wang et al. Reference Wang, Yang, Deng, Santosh, Zhang, Liu, Li, Huang, Zheng and Zhao2014). The Sulu Terrane is juxtaposed against the YC to the ESE along a NE-striking transpressional fault system. The YC includes Archaean (3.8 Ga) and Neoproterozoic (1120–850 Ma) greenstone and metasedimentary rock assemblages (Fig. 1; Fan, Reference Fan1995; Zhang et al. Reference Zhang, Zheng, Wu, Zhao, Gao and Wu2006), intruded by Palaeozoic kimberlites and lamproites (Zhang et al. Reference Zhang, Sun, Lu, Zhou, Zhou, Liu and Zhang2001).
The Jiaobei Terrane, situated in the southern margin of the NCC (Fig. 1; Tang et al. Reference Tang, Zheng, Wu, Gong and Liu2008; Zhang et al. Reference Zhang, Zhao, Zheng and Dai2010), mainly includes the Cordilleran-type (Dilek & Moores, Reference Dilek and Moores1999) Linglong metamorphic core complex (MCC), comprising a footwall that is intruded by deformed granites and separated from a hanging wall to the east by a master detachment zone (Fig. 2). The hanging wall is composed of Archaean, Palaeoproterozoic and Neoproterozoic tectonic units. The Archaean Jiaodong Group contains tonalite–trondhjemite–granodiorite (TTG) gneiss (2.9–2.7 Ga), amphibolite (2.5 Ga) and mafic granulite (2.4 Ga) (Tang et al. Reference Tang, Zheng, Wu, Gong and Liu2007; Jahn et al. Reference Jahn, Liu, Wan, Song and Wu2008). Palaeoproterozoic metasedimentary rocks (2484–2381 Ma) of the Fenzishan and Jingshan groups directly overlie the Archaean TTG gneiss (Wang et al. Reference Wang, Qiu, McNaughton, Groves, Luo, Huang and Liu1998). Neoproterozoic metasedimentary marble, slate and quartzite of the Penglai Group rest on the Palaeoproterozoic metasedimentary rock units (Faure et al. Reference Faure, Lin, Monie and Bruguier2004). The occurrence of 3.3 Ga detrital zircons in the metasedimentary rocks of the Fenzishan Group and 3.4–3.0 Ga inherited zircons in the Mesozoic intrusions (Wang et al. Reference Wang, Qiu, McNaughton, Groves, Luo, Huang and Liu1998) suggests that the Jiaobei Terrane also includes Palaeoarchaean continental crust (Fig. 3). Migmatites intercalated with Linglong granites, the Guojialing granitoids and the Aishan granitoids constitute the footwall of the Linglong MCC. The Linglong detachment fault is marked by a 030–040°-striking, c. 4 km wide (at the surface) ductile shear zone, which includes c. 0.5–1.0 km thick mylonitic–ultramylonitic rocks (Fig. 2).

Figure 3. Histogram of inherited zircon U–Pb ages from the Sulu Terrane, Yangtze Craton and Jiaobei Terrane. Data sources: Sulu Terrane, Tang et al. (Reference Tang, Zheng, Wu, Gong and Liu2008); Yangtze Craton, Xie et al. (Reference Xie, Gao, Liu and Gao2009), Zhou et al. (Reference Zhou, Wilde, Liu and Han2012); Jiaobei Terrrane, Wan et al. (Reference Wan, Song, Liu, Wilde, Wu, Shi, Yin and Zhou2006).
The Sulu Terrane is widely considered as the ENE extent of the Qinling–Dabie Orogenic Belt, which developed as a result of the collision between the YC and NCC around 240–220 Ma (Li et al. Reference Li, Xiao, Liu, Chen, Ge, Zhang, Sun, Cong, Zhang, Hart and Wang1993; Liu et al. Reference Liu, Xu, Liou and Song2004). The crystalline basement of the Sulu Terrane is composed mainly of UHP metamorphic rocks, including granitic gneiss, coesite-bearing eclogite, marble and quartzite (Fig. 1; Yang et al. Reference Yang, Chung, Wilde, Wu, Chu, Lo and Fan2005a; Tang et al. Reference Tang, Zheng, Wu, Gong and Liu2008; Liu, Gerdes & Liu, Reference Liu, Gerdes and Liu2013), with Neoproterozoic protolith ages (780–740 Ma) (Fig. 3; Li et al. Reference Li, Xiao, Liu, Chen, Ge, Zhang, Sun, Cong, Zhang, Hart and Wang1993). Local outcrops of eclogite, granulite and amphibolite with Palaeoproterozoic protolith ages of 2.0–1.8 Ga also occur in the Sulu Terrane (Fig. 3; Liu et al. Reference Liu, Tsujimori, Chu, Zhang and Wooden2006; Tang et al. Reference Tang, Zheng, Wu, Gong and Liu2008). The presence of coesite-bearing eclogite in the Sulu Terrane indicates that the protoliths of these UHP rocks were subducted to depths greater than 200 km beneath the NCC during middle Late Triassic time (Zheng, Reference Zheng2008).
The spatial, temporal and geochemical distribution of Mesozoic igneous rock groups in the Jiaodong Peninsula points to three main episodes of magmatism with distinct melt sources and tectonic regimes that were responsible for their formation (Goss et al. Reference Goss, Wilde, Wu and Yang2010; Jiang et al. Reference Jiang, Chen, Guo and Chang2012). The Shidao alkaline complex in the southeastern edge of the Sulu Terrane has an emplacement age of 215–200 Ma, and consists of potassic to ultrapotassic pyroxene-syenite, quartz-syenite and associated mafic dykes (Yang et al. Reference Yang, Chung, Wilde, Wu, Chu, Lo and Fan2005a). Magmas of this alkaline complex were produced by partial melting of the subducted Yangtze Craton lithospheric mantle during the exhumation of the UHP rocks in the Sulu Terrane (Yang et al. Reference Yang, Chung, Wilde, Wu, Chu, Lo and Fan2005a, b).
The second stage of Mesozoic magmatism in the Jiaodong Peninsula is represented by the Late Jurassic – Early Cretaceous granitoids (160–110 Ma) and their volcanic counterparts (Fig. 1; Sun et al. Reference Sun, Ding, Hu and Li2007; Ma et al. Reference Ma, Jiang, Hofmann, Dai, Hou, Zhao, Chen, Li and Jiang2014). The Late Jurassic granitoids (160–141 Ma) occur both in the Jiaobei and Sulu terranes and are composed of garnet-granite, biotite-granite, amphibole-bearing biotite-granite and muscovite-granite (Yang et al. Reference Yang, Wu, Chung, Wilde, Chu, Lo and Song2005b; Jiang et al. Reference Jiang, Chen, Guo and Chang2012). The Early Cretaceous granitoids are subdivided into two groups based on their spatial relationships (Fig. 1): the first group with ages of 132–123 Ma, which crops out mainly in the Jiaobei Terrane and consists of quartz-monzonite, porphyritic granodiorite and monzogranite with large K-feldspar phenocrysts (Hou et al. Reference Hou, Jiang, Jiang, Ling and Zhao2007; Zhang et al. Reference Zhang, Zhao, Zheng and Dai2010); and the second group with ages of 118–110 Ma (Goss et al. Reference Goss, Wilde, Wu and Yang2010) which occurs mainly in the Sulu Terrane, and includes monzogranite and syenogranite of I-type affinity and local alkali-feldspar granite of A-type affinity (Yang et al. Reference Yang, Deng, Goldfarb, Zhang, Gao and Wang2014b). The contemporaneous volcanic rocks (130–110 Ma) of the Cretaceous granitoids are formed of alkali basalt, basaltic trachyandesite, latite and trachyte. Basaltic rocks represent the partial melting products of a metasomatized, enriched lithospheric mantle, whereas the synchronous felsic rocks represent the anatectic melting products of the lower/middle continental crust (Fan et al. Reference Fan, Guo, Wang, Lin and Zhang2001). Underplating of basaltic melts beneath the Jiaobei Terrane likely provided the necessary heat source for this partial melting event.
The Late Jurassic granitic rocks in the Jiaobei Terrane (Figs 1, 2) are traditionally called the Linglong granites, which host the majority of gold resources in the NW part of the Jiaodong Peninsula. The Linglong granites intrude the crystalline Precambrian basement and consist mainly of biotite-granite with light grey to light flesh-pink colour and a medium- to coarse-grained granular texture (Fig. 4a). The granitic rocks are composed of quartz (35–40%), plagioclase (30–35%), K–feldspar (20–25%), biotite (5–10%) and amphibole (<2%), with accessory minerals of sphene, zircon and apatite (Fig. 4b, c). These rocks display a strong ductile deformation fabric represented by the recrystallized and foliated quartz aggregates between the K-feldspar and plagioclase grains (Fig. 4d). Stretched quartz grains show undulatory extinction and the biotite grains display a monoclinic symmetry and a well-developed cleavage (Fig. 4e). Dynamically recrystallized quartz grains form a well-developed foliation.

Figure 4. Hand-sampled images and photomicrographs of some of the representative Linglong granites under cross-polarized light. (a) Homogeneous, medium- to fine-grained biotite-granite composed mainly of plagioclase, K-feldspar, quartz and biotite. (b) K-feldspar with a plagioclase grain. (c) K-feldspar grain including biotite. (d) K-feldspar and plagioclase grains with recrystallized and foliated quartz aggregates between them, showing a strong ductile deformation fabric. (e) Foliated granite with stretched quartz showing undulatory extinction and a biotite fish displaying a monoclinic symmetry and a well-developed cleavage, indicating a top-to-the-right extensional deformation fabric. (f) Deformed granite with dynamically recrystallized quartz forming a well-developed foliation, K-feldspar and accessory zircon crystal. Ap – apatite; Bt – biotite; Kfs – K-feldspar; Pl – plagioclase; Q – quartz; Zr – zircon.
3. Sampling and analytical methods
Following our detailed field and structural observations, we picked 15 representative samples of the Linglong granites from underground adits in the Sizhuang, Cundong, Wang'ershan, Taishang and Luoshan gold deposit sites in the Jiaojia and Linglong regions. In order to avoid any potential hydrothermal alteration imprint on the rocks, we collected all the samples far from the gold lodes, the locations of which are shown in Figure 2. We ensured that the samples for geochemical analyses would display no secondary alteration effects in thin-sections (Fig. 4).
Whole-rock major- and trace-element geochemistry analyses were carried out at the Research Institute of Uranium Geology, Beijing, China using a Philips PW2404 X-Ray Fluorescence Spectrometer (XRF) and an ELEMENT-1 plasma mass spectrometer (Finnigan-MAT Ltd.), respectively. Analytical uncertainties were better than ±1% for major elements and ±5% for rare Earth elements. The detailed analytical procedures are presented in Wang et al. (Reference Wang, Yang, Deng, Santosh, Zhang, Liu, Li, Huang, Zheng and Zhao2014).
Whole-rock Sr–Nd isotopic data were collected using a Finnigan MAT-261 multicollector mass spectrometer at China University of Geosciences, Wuhan. The detailed analytical procedures for this isotope work are described in Wang et al. (Reference Wang, Yang, Deng, Santosh, Zhang, Liu, Li, Huang, Zheng and Zhao2014). The mass fractionation corrections used for Sr and Nd were 86Sr/88Sr=0.1194 and 146Nd/144Nd=0.7219. Throughout the analytical procedures the Sr standard NBS-987 yielded 87Sr/86Sr=0.710236±16 (2σ), and the Nd standard La Jolla yielded 143Nd/144Nd=0.511862±5 (2σ). Total analytical Sr and Nd blanks were <1 ng and <50 pg, respectively.
U–Pb zircon dating and trace-element analyses were carried out synchronously using a GeoLas Pro laser ablation system (Coherent Inc., America) equipped with a 193 nm ComPex102 ArF excimer laser coupled to an Agilent 7500a (Agilent Inc., America) quadrupole plasma mass spectrometer at the School of Resources and Environmental Engineering, Hefei University of Technology. Helium was used as the carrier gas to transport the ablated material through 3 mm i.d. PVC tubing and was combined with Ar in a 30 cm3 mixing chamber before entering the ICP-MS for isotopic measurement. Analyses were carried out with a beam diameter of 32 μm and 6 Hz repetition rate. All measurements were performed using zircon 91500 as the external standard for U–Pb dating, Mud Tank as the isotopic monitoring sample and NIST 610 as the external standard for the trace-element concentration calculation combined with the internal standard using 91Zr (Liu et al. Reference Liu, Gao, Hu, Gao, Zong and Wang2010). Common Pb was corrected following Andersen (Reference Andersen2002). Isotopic ratios and elemental concentrations were processed using ICP-MS DataCal program (Liu et al. Reference Liu, Hu, Gao, Günther, Xu, Gao and Chen2008, Reference Liu, Gao, Hu, Gao, Zong and Wang2010) with 1σ errors, and weighted mean age calculations were made by the ISOPLOT program of Ludwig (Reference Ludwig2003) with 2σ error.
In situ Hf isotope analyses of zircons were carried out on the same spots that were analysed for U–Pb dating. Hf isotopic compositions were determined by a Neptune Plus MC-ICP-MS (Thermo Fisher Scientific, Germany) equipped with a GeoLas 2005 excimer ArF laser ablation system (Lambda Physik, Göttingen, Germany) at the State Key Laboratory of Geological Processes and Mineral Resources, China University of Geosciences, Wuhan. Instrumental conditions, analytical procedures and data acquisition were as described by Wang et al. (Reference Wang, Yang, Deng, Santosh, Zhang, Liu, Li, Huang, Zheng and Zhao2014) and Hu et al. (Reference Hu, Liu, Gao, Liu, Zhang, Tong, Lin, Zong, Li, Chen, Zhou and Yang2012). Offline selection and integration of analytical signals and mass bias calibrations were performed using ICP-MS DataCal program (Liu et al. Reference Liu, Gao, Hu, Gao, Zong and Wang2010). A decay constant of 1.865×10–11 a–1 for 176Lu (Scherer, Munker & Mezger, Reference Scherer, Munker and Mezger2001) was adopted in this work. The measured U–Pb ages and the chondritic reservoir present-day 176Hf/177Hf ratio = 0.282772 and 176Lu/177Hf = 0.0332 (Blichert-Toft & Albarede, Reference Blichert-Toft and Albarede1997) were used to calculate the initial 176Hf/177Hf ratio (ε Hf(t)). Single-stage Hf model ages (T DM1) were determined with present-day 176Hf/177Hf = 0.283250 and 176Lu/177Hf = 0.0384 (Griffin et al. Reference Griffin, Pearson, Belousova, Jackson, van Achterbergh, O‘Reilly and Shee2000), and two-stage Hf model ages (T DM2) were calculated using a 176Lu/177Hf ratio of 0.015 (Griffin et al. Reference Griffin, Wang, Jackson, Pearson, O'Reilly, Xu and Zhou2002).
4. Zircon geochronology
The results of LA-ICP-MS U–Pb zircon dating of eight biotite-granite samples from the Linglong granites are provided in online Supplementary Table S1 (available at https://journals.cambridge.org/geo). The weighted 206Pb/238U ages and selected cathodoluminscence (CL) images of the zircons are presented in Figure 5. Zircons from the biotite-granite samples are short and prismatic or stubby, with lengths and length/width ratios ranging over 100–220 μm and 1:1–3:1, respectively. CL images reveal that the majority of zircons have well-developed oscillatory zoning, suggesting their magmatic origin (Hoskin & Schaltegger, Reference Hoskin and Schaltegger2003), and some zircons contain inherited cores (Fig. 5). The analysed zircon grains show large variations in Th (6–1213 ppm) and U (14–1171 ppm) contents, with their Th/U ratios mostly ranging over the range 0.4–2.09, except for a few grains with Th/U ratios <0.1. In this study we have used the 206Pb/238U ages for rocks younger than 1000 Ma, and the 207Pb/206Pb ages for rocks older than 1000 Ma.

Figure 5. LA-ICP-MS U–Pb concordia diagrams for zircon grains from the Linglong granites. Insets show the representative CL images with U–Pb ages of the analysed zircon grains.
Previous SHRIMP/LA-ICP-MS U–Pb zircon geochronological studies of the Linglong granites have yielded relatively consistent, Late Jurassic ages ranging over 150–160 Ma (Qiu et al. Reference Qiu, Groves, McNaughton, Wang and Zhou2002; Zhang et al. Reference Zhang, Zhao, Zheng and Dai2010; Ma et al. Reference Ma, Jiang, Dai, Jiang, Hou, Pu and Xu2013). These existing Late Jurassic ages are in agreement with the new ages of the majority of dated zircon grains we have obtained in this study (Fig. 5). Our zircon geochronology work provides more tightly constrained age data for the timing of the emplacement of the Linglong granites and their record of inherited zircons.
4.a. Sample XC10D217B2
Twenty-eight zircon spots were analysed in sample XC10D217B2, yielding concordant ages ranging from 2310±59 to 129±4 Ma (Fig. 5b). Nineteen of the 28 analyses, ranging from 174±6 to 154±5 Ma, form a coherent group on the concordia diagram yielding a weighted mean age of 161±2 Ma (2σ, MSWD = 0.95) (Fig. 5a). Four analyses have yielded the youngest and concordant ages between 134±4 Ma and 129±4 Ma. The remaining five analyses of inherited zircons have given ages ranging from 2310±59 to 190±6 Ma.
4.b. Sample XC12D010B7
Twenty-six analyses from sample XC12D010B7 have yielded concordant ages of 744±20 to 150±7 Ma (Fig. 5d). Twenty-two of these concordant ages range between 178±10 and 150±7 Ma, with a weighted mean age of 161±3 Ma (2σ, MSWD = 1.6) (Fig. 5c). The other four concordant ages, 744±20, 226±7 Ma, 201±6 Ma and 197±6 Ma, are from inherited zircons.
4.c. Sample WES10D214B1
Fifty-eight zircon spots were analysed for this sample, giving ages ranging from 2547±26 Ma to 154±3 Ma (Fig. 5f). Twenty-three analyses have revealed ages of 176±3 Ma to 154±3 Ma, forming a coherent group with a weighted mean age of 161±2 Ma (2σ, MSWD = 3.3) (Fig. 5e). The other 29 analyses have provided old and concordant ages ranging from 2547±26 Ma to 180±3 Ma, defining three age clusters at 260 Ma, 220 Ma and 180 Ma.
4.d. Sample WES10D228B2
Sixty zircon spots were analysed in this sample, yielding ages ranging from 832±22 Ma to 156±3 Ma. Nineteen analyses have given young ages of 168±4 Ma to 156±3 Ma, with a mean age of 161±2 Ma (2σ, MSWD = 1.4) (Fig. 5g). The remaining 41 analyses define concordant ages with a wide scatter of ages ranging from 832±22 Ma to 181±5 Ma (Fig. 5h).
4.e. Sample TS12D008B2
Twenty-seven analyses from sample TS12D008B2 have given concordant ages ranging from 180±3 Ma to 138±3 Ma (Fig. 5j). Eighteen of these analyses are in the range of 169±3 Ma to 151±2 Ma, with a weighted mean age of 160±3 Ma (2σ, MSWD = 6.4) (Fig. 5i). Eight analyses have revealed younger ages varying between 149±2 Ma to 136±1 Ma and the remaining three analyses reflect an inherited age of 180±3 Ma.
4.f. Sample TS12D017B1
Twenty-five zircon spots were analysed from this sample, giving concordant ages ranging from 2490±30 Ma to 135±1 Ma (Fig. 5l). Two of these analyses have yielded young concordant ages of 135±1 Ma. Twenty-two of the remaining analyses have provided concordant ages between 168±2 Ma and 152±2 Ma, with a weighted mean age of 158±2 Ma (2σ, MSWD = 5.5) (Fig. 5k). One analysis has revealed an inherited age of 2490±30 Ma.
4.g. Sample LS13D003B1
We carried out 18 U–Pb isotope analyses on this sample. The concordant or near-concordant ages range from 174±6 to 152±5 Ma (Fig. 5n), producing a coherent group on the concordia diagram with a weighted mean age of 160±3 Ma (2σ, MSWD = 1.3) (Fig. 5m).
4.h. Sample LS13D042B3
We have a total of 19 analyses from this sample, giving concordant ages ranging from 2500±60 Ma to 153±4 Ma (Fig. 5p). Fourteen of these analyses have yielded young and concordant age groups of 175±6 Ma to 153±4 Ma, with a weighted mean 206Pb/238U age of 159±3 Ma (2σ, MSWD = 1.3) (Fig. 5o). The remaining five analyses have provided old and concordant ages ranging from 2500±60 Ma to 214±6 Ma.
4.i. Summary of zircon geochronology
The analysed samples show a significant age peak around 160 Ma (Fig. 5). Most of the dated zircon grains from each sample have weighted mean ages varying between 161±3 Ma and 158±2 Ma, which we interpret as the emplacement age of the Linglong granites (online Supplementary Table S1; Fig. 5). However, these samples commonly contain inherited zircons with ages ranging over 180–300 Ma, as well as some Proterozoic and Archaean ages which characterize the ages of the crystalline basement rocks in NCC (Fig. 6). There is also a younger cluster of ages between 149±2 Ma and 129±4 Ma from zircon grains that display clear zoning (Fig. 7), Th/U ratios of 0.1–0.7 and similar REE patterns to the zircons dated at c. 160 Ma. These REE patterns are distinctively left-leaning and show positive Ce anomalies (Fig. 8) and indicate, together with the zoning textures and Th/U ratios of the analysed zircons, a magmatic origin of the grains. These magmatic zircons represent a major igneous event during latest Jurassic time through earliest Cretaceous history of the eastern part of the NCC. The existence of a 130 Ma granitoid (Guojialing granodiorite suite) in the study area has been reported earlier (Yang et al. Reference Yang, Chu, Liu and Zhai2003, Reference Yang, Fan, Santosh, Hu, Wilde, Lan, Lu and Liu2012).

Figure 6. Histogram of inherited U–Pb zircon ages from the analysed Linglong granite samples.

Figure 7. Cathodoluminescence (CL) images of Linglong zircon grains with ages over the range 129–149 Ma. Yellow circles highlight the sites of LA-ICP-MS dating, and numbers show the sequence of the dating spots.

Figure 8. Chondrite-normalized rare Earth element (REE) patterns of zircon grains from the Linglong granites.
5. Whole-rock major- and trace-element geochemistry
Our major- and trace-element data for the Linglong granites are presented in Table 1. The analysed rocks show low LOI (loss on ignition) values of 0.28–2.99 wt% which indicate the lack of any significant post-magmatic hydrothermal alteration, consistent with our petrographic observations of thin sections.
Table 1. Major (wt%) and trace (ppm) element compositions of a representative suite of the Linglong granites.

The representative Linglong granite samples have SiO2 contents of 69–74 wt% and total alkali (K2O + Na2O) concentrations of 8–9 wt%, defining them as ‘granite’ in the (K2O + Na2O) v. SiO2 diagram (Fig. 9a). They have relatively high K2O contents of 3–5 wt% and K2O/Na2O ratios of 0.6–1.2, and they are metaluminous to weakly peraluminous with an aluminium saturation index (i.e. A/CNK = molecular Al2O3/(CaO+Na2O+K2O)) of 0.95–1.05 (Fig. 9b). Their Mg no. values are low, ranging over 21–51 (average = 31; Table 1). The major oxides FeO+MgO, Al2O3 and CaO correlate negatively with SiO2 (Fig. 9c–e); the MgO contents do not show any linear pattern against the SiO2 values.

Figure 9. Selective major-element diagrams for the classification of the Linglong granites. (a) Total alkali v. silica (TAS) diagram (after Middlemost, Reference Middlemost1994). (b) A/NK (molar Al2O3/(Na2O+K2O) v. A/CNK (molar Al2O3/(CaO+Na2O+K2O)) diagram (after Maniar & Piccoli, Reference Maniar and Piccoli1989). (c) (FeO + MgO) v. K2O diagram. (d–f) Al2O3, CaO and MgO v. SiO2, respectively. Published data for the Linglong granites (J. H. Yang, unpub. Ph.D. thesis, Institute of Geology and Geophysics, Chinese Academy of Sciences, 2000; Hou et al. Reference Hou, Jiang, Jiang, Ling and Zhao2007) and TTGs (Shan et al. Reference Shan, Zhai, Wang, Zhou, Santosh, Zhu, Zhang and Wang2015) are also included.
The Linglong granites are characterized by low total REE contents (ΣREE = 68–136 ppm). In the chondrite-normalized REE diagram (Fig. 10a) they show enrichment in light rare Earth elements (LREE) with the (La/Yb)N ratios varying over 8–30, and they display flat HREE patterns. They exhibit negligible negative to medium-positive Eu anomalies, with variable Eu/Eu* ratios of 0.8–2.1. In the primitive mantle-normalized spider diagram (Fig. 10b) all the samples appear enriched in large-ion lithophile elements (LILEs), such as Rb (56–86 ppm), Ba (1417–2690 ppm) and Sr (455–980 ppm), but relatively depleted in high-field-strength elements (HFSEs) such as Nb (3–8 ppm), Ta (0.1–0.5 ppm) and Y (3–13 ppm). They are also enriched in LREEs and Pb, but have low compatible element concentrations with Cr varying over 0.4–22.3 ppm, Ni varying over 0.5–5.4 ppm and V varying over 12–67 ppm.

Figure 10. (a) Chondrite-normalized rare Earth element (REE) patterns, and (b) primitive mantle-normalized trace-element spider diagrams for the Linglong granite samples, analysed in this study. Chondrite normalizing values are from Sun & McDonough (Reference Sun, McDonough, Saunders and Norry1989), and primitive mantle values are after McDonough & Sun (Reference McDonough and Sun1995). TTG field is shown for comparison (data from Shan et al. Reference Shan, Zhai, Wang, Zhou, Santosh, Zhu, Zhang and Wang2015).
6. Whole-rock Sr–Nd isotopes
Two representative samples of the Linglong granites were analysed for Rb–Sr and Sm–Nd isotopic compositions (Table 2). Our results, together with the extant data available in the literature, are displayed in Figure 11. Collectively, the Linglong granitoids have 87Sr/86Sr ratios of 0.709010–0.716132 and (87Sr/86Sr)i ratios of 0.708257–0.713731, calculated for the initial age of 160 Ma. The measured 143Nd/144Nd ratios vary over 0.511493–0.511651 and the calculated ε Nd(t) values range from –22.4 to –10.9, with the two-stage Nd model ages varying over 1755–3669 Ma.
Table 2. Rb–Sr and Sm–Nd isotopic compositions for a representative suite of the Linglong granites.

Note: ε Nd = [(143Nd/144Nd)S/(143Nd/144Nd)CHUR–1]×10000 (CHUR – chondritic uniform reservoir), fSm/Nd = (147Sm/144Nd)S/(147Sm/144Nd)CHUR–1, T DM2 = (1/λ Sm)ln(1+A), A = {(143Nd/144Nd)S–(143Nd /144Nd)DM–[(147Sm/144Nd)S–(147Sm/144Nd)C](eλt–1)}/[(147Sm/144Nd)C–(147Sm/144Nd)DM]◦ (147Sm/144Nd)DM = 0.2136, (147Sm/144Nd)DM = 0.513151(Miller & O'Nions, Reference Miller and O'Nions1985); (147Sm/144Nd)CHUR=0.1967, (143Nd/144Nd)CHUR=0.512638 (Lugmair & Marti, Reference Lugmair and Marti1978).

Figure 11. Whole-rock ε Nd(t) v. (87Sr/86Sr)i diagram for the Linglong granites. The published data for other rock suites recalculated for 160 Ma are also included. Data source: Linglong (Zhang et al. Reference Zhang, Zhao, Zheng and Dai2010; Jiang et al. Reference Jiang, Chen, Guo and Chang2012; Yang et al. Reference Yang, Fan, Santosh, Hu, Wilde, Lan, Lu and Liu2012; Ma et al. Reference Ma, Jiang, Dai, Jiang, Hou, Pu and Xu2013); Kunyushan (Zhang et al. Reference Zhang, Zhao, Zheng and Dai2010; D. Wang, unpub. M.Sc. thesis, China Academy of Geological Sciences); Neoarchaean basement of the Jiaobei Terrane (Yang et al. Reference Yang, Chu, Liu and Zhai2003); Neoproterozoic basement of the Sulu Terrane (Tang et al. Reference Tang, Zheng, Wu, Gong and Liu2008); Triassic Shidao alkaline complex in Sulu UHP units (Gao et al. Reference Gao, Chen, Xie and Qian2004). CHUR – chondritic uniform reservoir; DM – depleted mantle.
7. Zircon Hf isotopes
Our results of the zircon Lu–Hf isotope analyses are presented in online Suuplementary Table S2 (available at https://journals.cambridge.org/geo). The 176Lu/177Hf ratios are mostly <0.002, indicating the low accumulation of radiogenic Hf after the formation of zircons in the rocks. The initial 176Hf/177Hf ratios can be used as a robust reference to evaluate the source characteristics of these zircons (Wu et al. Reference Wu, Li, Zheng and Gao2007). The fLu/Hf values range between –1.00 and –0.88, which are lower than the fLu/Hf values of mafic (−0.34; Amelin, Lee & Halliday, Reference Amelin, Lee and Halliday2000) and sialic crust (–0.72; Vervoort et al. Reference Vervoort, Patchett, Gehrels and Nulman1996). The two-stage model age (T DM2) can therefore be used to constrain the timing of the extraction of the source materials from the depleted mantle or when these source materials were residual in the crust (Blichert-Toft & Albarede, Reference Blichert-Toft and Albarede1997). A total of 215 zircon grains were analysed, yielding variable ε Hf(t) values of –39.1 to 6.4 and T DM2 ages of 894–3710 Ma.
7.a. Sample XC10D217B2
Fourteen zircon grains from sample XC10D217B2 were chosen for in situ Hf isotope analysis (online Supplementary Table S2). Twelve analyses of the Late Jurassic zircons (164–156 Ma) yielded negative ε Hf(t) values varying from –39.1 to –18.4 (average –25.3) with T DM2 ranging over 2377–3665 Ma (average 2806 Ma) (Fig. 12). The other two grains were inherited zircons with ages of 1755 Ma and 2310 Ma, and yielded a ε Hf(t = 1755 Ma) value of –6.9 and a ε Hf(t = 2310 Ma) value of 6.4, with T DM2 of 2852 Ma and 2463 Ma, respectively.

Figure 12. ε Hf(t) v. U–Pb zircon age diagram for the Linglong granites and other rock suites in the Jiaobei Terrane.
7.b. Sample XC12D010B7
Thirteen zircon grains from sample XC12D010B7 were chosen for in situ Hf isotope analysis (online Supplementary Table S2). Eleven analyses of the Late Jurassic zircons (169–150 Ma) yielded negative ε Hf(t) values varying from –26.0 to –19.4 (average –23.0) with T DM2 over the range 2436–2845 Ma (average 2806 Ma) (Fig. 12). The other two inherited zircon grains with ages of 201 Ma and 744 Ma yielded a ε Hf(t = 201 Ma) value of –20.0 and a ε Hf(t = 744 Ma) value of –9.3, with T DM2 of 2501 Ma and 2239 Ma, respectively.
7.c. Sample WES10D214B1
Fifty-eight zircons from sample WES10D214B1 were chosen for in situ Hf isotope analysis (online Supplementary Table S2). Thirty analyses of the Late Jurassic zircons (176–154 Ma) yielded negative ε Hf(t) values varying from –20.2 to –1.5 (average –14.4) with T DM2 over 1303–2486 Ma (average 2124 Ma) (Fig. 12). The other 28 inherited zircons with ages 180–921 Ma yielded εHf(t) values of –38.7 to 2.3 with T DM2 ages of 1204–3710 Ma.
7.d. Sample WES10D228B2
Sixty-one zircon grains from sample WES10D228B2 were chosen for in situ Hf isotope analysis (online Supplementary Table S2). Nineteen analyses of the Late Jurassic zircons (168–156 Ma) yielded negative ε Hf(t) values varying from –18.1 to –4.3 (average –13.7) with T DM2 of 1489–2356 Ma (average 2079 Ma) (Fig. 12). The other 42 inherited zircons with ages of 181–832 Ma yielded ε Hf(t) values of –33.3 to 6.2 with T DM2 ages of 894–3464 Ma.
7.e. Sample TS12D008B2
Fifteen in situ Hf isotope analyses were carried out on the Late Jurassic zircons (180–141 Ma) from sample TS12D008B2 (online Supplementary Table S2). The results show negative ε Hf(t) values varying from –29.3 to –21.0 (average –24.6) with T DM2 ages of 2524–3065 Ma (average 2762 Ma) (Fig. 12).
7.f. Sample TS12D017B1
Sixteen zircons from sample TS12D017B1 were chosen for in situ Hf isotope analysis (online Supplementary Table S2). Fifteen analyses of the Late Jurassic zircons (183–143 Ma) yielded negative ε Hf(t) values varying from –27.2 to –20.8 (average –23.9) and T DM2 ages of 2520–2927 Ma (average 2717 Ma) (Fig. 12). One inherited zircon with an age of 2490 Ma yielded an ε Hf(t) value of 5.7 with a T DM2 age of 2644 Ma.
7.g. Sample LS13D003B1
Seventeen spots of in situ Hf isotope analyses were carried out on the Late Jurassic zircons (164–152 Ma) from sample LS13D003B1. The results show negative ε Hf(t) values varying from –27.4 to –24 (average –25.5) with T DM2 ages of 2729–2943 Ma (average 2818 Ma) (Fig. 12).
7.h. Sample LS13D042B3
Twenty-one zircon grains from sample LS13D042B3 were chosen for in situ Hf isotope analysis (online Supplementary Table S2). Eighteen analyses of the Late Jurassic zircons (178–145 Ma) yielded negative ε Hf(t) values varying from –26.9 to –22.3 (average –25.2) with T DM2 ages of 2620–2908 Ma (average 2802 Ma) (Fig. 12). The remaining three grains are inherited zircons with ages of 188 Ma, 214 Ma and 2500 Ma, and yielded ε Hf(t) values of –25.5, –23.2 and 5.1 with T DM2 ages of 2842 Ma, 2714 Ma and 2691 Ma, respectively.
7.i. Summary of the zircon Hf isotope analyses
Zircon populations with different age spectra show distinct Hf isotopic characteristics. The majority of the magmatic zircons with ages of 150–179 Ma have variable ε Hf(t) values ranging from –39.1 to –1.5 (dominantly between –29.0 and –10.8), and show T DM2 ages of 1303–3605 Ma (Fig. 13). The inherited zircons with continuous ages of 180–300 Ma show ε Hf(t) values mostly ranging from –18.7 to –6.9 (excluding three spots with relatively low ε Hf(t) values of –38.7, –36.1 and –35.9 and two spots with positive ε Hf(t) values of 0.8 and 6.2), and have corresponding T DM2 ages of 1677–2360 Ma (excluding three spots with relatively ancient T DM2 ages of 3710 Ma, 3542 Ma and 3506 Ma and two spots with younger T DM2 ages of 1204 Ma and 894 Ma). The scattered Carboniferous–Proterozoic inherited zircons show ε Hf(t) values mainly varying from –16.6 to –1.8 and T DM2 ages of 1832–2381 Ma (Fig. 13). Three inherited zircons with ages of c. 2500 Ma have positive ε Hf(t) values of 5.1–6.4 and T DM2 ages of 2461–2691 Ma (Fig. 13).

Figure 13. ε Hf(t) v. U–Pb zircon age diagram for the Linglong granites and the basement units in the Jiaobei Terrane, Yangtze Craton and Sulu Terrane. Data source: Linglong (Zhang et al. Reference Zhang, Zhao, Zheng and Dai2010; Jiang et al. Reference Jiang, Chen, Guo and Chang2012; Yang et al. Reference Yang, Fan, Santosh, Hu, Wilde, Lan, Lu and Liu2012; Ma et al. Reference Ma, Jiang, Dai, Jiang, Hou, Pu and Xu2013); Jiaobei Terrane (Wan et al. Reference Wan, Song, Liu, Wilde, Wu, Shi, Yin and Zhou2006); Yangtze Craton (Xie et al. Reference Xie, Gao, Liu and Gao2009); Sulu Terrane (Tang et al. Reference Tang, Zheng, Wu, Gong and Liu2008).
8. Petrogenesis of the Linglong granites
The Linglong granites have been previously classified as adakite-like intrusions (Zhang et al. Reference Zhang, Zhao, Zheng and Dai2010; Ma et al. Reference Ma, Jiang, Dai, Jiang, Hou, Pu and Xu2013). However, the Linglong biotite-granite samples we have investigated in this study have high K2O/Na2O ratios of 0.6–1.2 and high K2O contents of 3–5 wt%, and relatively low Mg no. (21–51, average = 31), Cr (0.4–22.3 ppm, average = 11.9 ppm) and Ni (0.5–5.4 ppm, average = 2.2 ppm) abundances, in contrast to the characteristic geochemical features of typical adakites (Moyen, Reference Moyen2009; Defant & Drummond, Reference Defant and Drummond1990). These samples all define a dominantly calc-alkaline trend in a Na–K–Ca plot (Fig. 14a), rather than common trondhjemitic affinities of typical adakites. Furthermore, the Linglong granites also have some geochemical features that distinguish them from I-, S-, M- and A-type granites: high Ba (1417–2690 ppm) and Sr (455–980 ppm) concentrations; high light REE contents (>62.51 ppm) (Fig. 14b); a lack of pronounced negative Eu anomalies; low heavy REE values (<7.69 ppm); and low Y and Yb contents with high Sr/Y (57–296) and (La/Yb)N (8–95) ratios. These geochemical characteristics suggest that the Linglong granites represent high Ba–Sr granites (Fowler et al. Reference Fowler, Henney, Darbyshire and Greenwood2001; Ye et al. Reference Ye, Li, Li and Zhang2008; Peng et al. Reference Peng, Wilde, Fan and Peng2013).

Figure 14. (a) Na–K–Ca plot and (b) Sr–Rb–Ba plot (after Tarney & Jones, Reference Tarney and Jones1994) for the analysed Linglong granite samples. The extant data for the Linglong granites in the published literature are also included (J. H. Yang, unpub. Ph.D. thesis, Institute of Geology and Geophysics, Chinese Academy of Sciences, 2000; Hou et al. Reference Hou, Jiang, Jiang, Ling and Zhao2007). Fields of high Ba–Sr and low Ba–Sr granites are based on the data from Fowler et al. (Reference Fowler, Henney, Darbyshire and Greenwood2001). Fields of adakite are based on Qian et al. (Reference Qian, Chung, Lee and Wen2003).
Several petrogenetic models have been proposed in the literature for the origin of high Ba–Sr granites: (1) partial melting of subducted oceanic crust that is younger than 25 Ma at the time of subduction (Defant & Drummomd, Reference Defant and Drummond1990, Reference Defant and Drummond1993; Tarney & Jones, Reference Tarney and Jones1994); (2) crystal fractionation of mantle-derived mafic magmas, which are contaminated by melts produced by partial melting of subducted pelitic sediments (Fowler et al. Reference Fowler, Henney, Darbyshire and Greenwood2001); (3) mixing of enriched, mantle-derived mafic melts with felsic magmas derived from partial melting of the continental lower crust (Tarney & Jones, Reference Tarney and Jones1994); and (4) partial melting of a thick mafic lower crust (Petford & Atherton, Reference Petford and Atherton1996; Ye et al. Reference Ye, Li, Li and Zhang2008; Choi et al. Reference Choi, Rajesh, Seo, Park, Oh, Pak and Kim2009).
We have evaluated these four models based on the regional geological constraints and our new geochemical and isotopic data. The first model, which suggests that the high Ba–Sr Linglong granites might have formed by partial melting of a subducted oceanic crust younger than 25 Ma, is not plausible because the Mianlue oceanic lithosphere subducted beneath the NCC was Palaeozoic–Triassic in age (Yang et al. Reference Yang, Deng, Dilek, Qiu, Ji, Li, Taylor and Yu2015). Further, the Palaeo-Pacific oceanic plate subducted westwards beneath the eastern margin of Asia during Early–Middle Jurassic time was also older than Jurassic age (Wu et al. Reference Wu, Lin, Wilde, Zhang and Yang2005; Jiang et al. Reference Jiang, Jiang, Ling and Ni2010). We also think that it is unlikely that the Linglong granites may have formed by crystal fractionation of mantle-derived mafic magmas, because the positive Eu and Sr anomalies displayed by the analysed samples suggest that these rocks did not undergo any significant feldspar fractionation. Finally, there are no basaltic rocks that are spatially ad temporally associated with the Linglong granites, and no mafic enclaves have ever been reported from these granites. These field observations indicate that the Linglong granites were unlikely to have been generated by mixing of enriched mantle-derived mafic and lower crust-derived felsic magmas.
Experimental studies have demonstrated that <40% partial melting of basaltic rocks could produce the intermediate–acidic magmas with high Ba–Sr contents at P–T conditions of 1000–1100°C and 8–32 kPa (Peacock et al. Reference Peacock, McCann, Sothcott and Astin1994; Rapp & Watson, Reference Rapp and Watson1995). The Mg nos of the Linglong granite samples varying over the range 21–51 are consistent with the derivation of their magmas from a mafic source (Petford & Atherton, Reference Petford and Atherton1996; Tate & Johnson, Reference Tate and Johnson2000; Wang et al. Reference Wang, Wyman, Xu, Zhao, Jian, Xiong, Bao, Li and Bai2006). This inference is also compatible with the low Cr and Ni contents of the high Ba–Sr Linglong granites (Table 1). The presence of residual garnet and possibly amphibole in the magma source of the Linglong granites is suggested by the strong fractionation between their LREE and HREE ((La/Yb)N = 8–95), high Sr/Y ratios and low Y and Yb contents (Defant & Drummond, Reference Defant and Drummond1990, Reference Defant and Drummond1993). We therefore posit that magmas of the Linglong granites were likely derived from partial melting of a thick lower crust (at least 50 km thick) with residual garnet and amphibole contents, rather than plagioclase (Sen & Dunn, Reference Sen and Dunn1994; Rapp & Watson, Reference Rapp and Watson1995).
Experimental studies have also shown, however, that partial melting of mafic lower crustal rocks commonly produces Na-rich granitic magmas (Rapp, Watson & Miller, Reference Rapp, Watson and Miller1991; Rapp & Watson, Reference Rapp and Watson1995) but does not produce high-K calc-alkaline rocks (Patino, Reference Patino, Catro, Fernandez and Vigneresse1999) such as the Linglong granites. Furthermore, the relatively wide-ranging ε Hf(t) values of –39.1 to –1.5 (dominantly between –29.0 and –10.8) obtained from the Linglong magmatic zircons suggest involvement of less-evolved material in the genesis of their magmas, and not entirely by partial melting of an ancient mafic source. We think that the regional geology may provide some answers to these seemingly conflicting aspects of model no. 4. The Linglong granites contain a significant number of inherited zircons with ages varying over the range 200–240 Ma, and the Sulu Terrane includes a K-rich alkaline complex. We infer that the addition of magmas derived from re-melting of the Late Triassic juvenile cumulate rocks preserved in the UHP metamorphic rocks (240–220 Ma), which mainly consist of granitic gneiss, coesite-bearing eclogite, marble and quartzite, and from partial melting of the Sulu alkaline rocks (215–200 Ma), could have given the Linglong magmas a more juvenile character and could have provided the necessary potassium to these magmas.
We therefore conclude that re-melting of the Late Triassic juvenile mafic magmatic rocks together with partial melting of the thick, ancient mafic lower crust beneath the Jiadong Peninsula must have contributed significantly to the petrogenesis of the high-K magmas of the Linglong granites during Late Jurassic time. The Linglong magmas must also have interacted with and assimilated the pre-existing Archaean TTGs and Neoproterozoic (832–563 Ma), Palaeozoic (496–254 Ma) and Triassic (247–201 Ma) magmatic rocks in the Jiadong Peninsula during their ascent, in order to obtain all the inherited magmatic zircons with these age spans as documented in this study. However, it is difficult to constrain the impact of this crustal contamination and assimilation on the isotopic systematics of the Linglong granites based on the currently available data.
9. Tectonic model
One of the widely accepted interpretations for the origin of the Late Jurassic Linglong granites is post-collisional magmatism induced by lithospheric-scale extension (Hou et al. Reference Hou, Jiang, Jiang, Ling and Zhao2007; Yang et al. Reference Yang, Fan, Santosh, Hu, Wilde, Lan, Lu and Liu2012, Reference Yang, Santosh, Shen and Li2014b). The collision between the NCC and YC took place during Middle Triassic time (c. 240 Ma) (Li et al. Reference Li, Xiao, Liu, Chen, Ge, Zhang, Sun, Cong, Zhang, Hart and Wang1993; Liu et al. Reference Liu, Xu, Liou and Song2004). The Late Triassic alkaline and mafic magmatism (215–200 Ma) across this orogenic belt has been interpreted as a post-collisional event, triggered by a tectonic collapse of the overthickened continental crust (Yang et al. Reference Yang, Chung, Wilde, Wu, Chu, Lo and Fan2005a, Reference Yang, Wu, Chung, Wilde, Chu, Lo and Songb, Reference Yang, Deng, Guo, Guo, Wang, Chen and Wang2016b). There is no geological record of any magmatic event in the Jiaodong Peninsula during 205–165 Ma (Zhang, Reference Zhang, Zhai, Windley, Kusky and Meng2007; Yang et al. Reference Yang, Deng, Goldfarb, Zhang, Gao and Wang2014a). Any post-collisional magmatism in the region must therefore have occurred during Late Triassic time and not during Jurassic time as the many current models for the origin of the Linglong granites suggest.
Regional geodynamic models and tectonic interpretations from the NW Pacific Ocean and the adjacent land propose that the initiation of a low-angle subduction of the Palaeo-Pacific plate beneath eastern Asia at c. 180 Ma may have placed the current Jiadong Peninsula in the upper plate of an active continental margin (Maruyama et al. Reference Maruyama, Isozaki, Kimura and Terabayashi1997; Zhou & Li, Reference Zhou and Li2000; Li & Li, Reference Li and Li2007; Jiang et al. Reference Jiang, Jiang, Ling and Ni2010; Ma et al. Reference Ma, Jiang, Dai, Jiang, Hou, Pu and Xu2013). Slab dehydration and partial melting of the Palaeo-Pacific slab subducting at a low-angle produced high-Mg adakitic magmas of the c. 177 Ma Xinglonggou and other granitoids in the eastern NCC (Fig. 15a).

Figure 15. (a) Simplified tectonic map of ENE China showing the major continental blocks, suture zones and fault systems. Late Mesozoic plutons and rift units, and metamorphic core complexes with extensional directions are also shown. (b, c) Tectonic model for the magmatic evolution of the Late Jurassic Linglong granites. CLM – continental lithospheric mantle; MASH – magma assimilation, storage and homogenization; PPP – Palaeo-Pacific Plate; TF – Tanlu Fault; WYF – Wulian–Yantai Fault. See text for discussion.
The subsequent steepening and rapid rollback of the Palaeo-Pacific plate by 165–160 Ma induced asthenospheric upwelling beneath the upper plate, causing partial melting of the garnet-amphibolite-bearing lower crust of the Jiaobei Terrane and the juvenile crust with alkaline rocks of the Sulu Terrane (Fig. 15c). The advective heating and partial melting of the lower crust across the suture zone created a long-lasting, open-system magmatic front near the crust–mantle boundary, facilitating assimilation, storage and homogenization (MASH) (Fig. 15c; Hildreth & Moorbath, Reference Hildreth and Moorbath1988). Magmas derived from partial melting of the garnet-amphibolite-bearing Jiaobei and the alkaline rocks of the Sulu terranes were mixed and evolved into the high Ba–Sr granitic magmas (Hou et al. Reference Hou, Jiang, Jiang, Ling and Zhao2007) of the 160–158 Ma Linglong granites in this MASH system. The production of the Linglong granites by partial melting of the mafic lower crust marks the onset of a lithospheric-scale continental extension in eastern China by Late Jurassic time that continued into Early Cretaceous time with a strong magmatic imprint (Fowler et al. Reference Fowler, Kocks, Darbyshire and Greenwood2008; Ye et al. Reference Ye, Li, Li and Zhang2008; Peng et al. Reference Peng, Wilde, Fan and Peng2013). This extensional regime led to further cratonic thinning in the NCC, starting with the post-collisional collapse of the Triassic orogenic belt in eastern Asia.
10. Conclusions
Our new LA-ICP-MS U–Pb zircon dating of the Linglong granites constrain their emplacement age between 161±3 Ma and 158±</2>2 Ma during Late Jurassic time. The Linglong granites have high K2O/Na2O ratios and high K2O contents, and have relatively low Mg nos and Cr and Ni values. They contain very high Ba and Sr concentrations and represent high-K, calc-alkaline granites. We therefore classify them as high Ba–Sr granites instead of adakitic granitoids as interpreted in previous studies. The Linglong granite magmas were generated by partial melting of a garnet-amphibolite-bearing and alkaline-rich lower crust of the Jiaobei and Sulu terranes, respectively.
This Late Jurassic magmatic event was induced by slab-rollback-related asthenospheric upwelling beneath East Asia, following the onset of a flat subduction of the Palaeo-Pacific oceanic lithosphere westwards under the continent. Emplacement of the Late Jurassic high Ba–Sr Linglong granites therefore marks the beginning of a lithospheric-scale extension and accompanying magmatism that resulted in significant thinning of the NCC by the end of Early Cretaceous time.
Acknowledgments
We thank Richard J Goldfarb and Ryan Taylor for their help with the fieldwork in the Jiaodong Peninsula. Constructive comments by Jean-François Moyen, Huafeng Zhang and Qingjie Gong on an earlier version of the manuscript were helpful for our interpretations. This study was financially supported by the National Natural Science Foundation of China (grant nos 41230311 and 41572069), the National Science and Technology Support Program (grant no. 2011BAB04B09), the geological investigation work project of the China Geological Survey (grant no. 12120114034901) and 111 Project of China (grant no. B07011). Y Dilek's work in eastern China was supported by research funds from the China University of Geosciences in Beijing. We thank the two anonymous referees of Geological Magazine for their constructive and thorough reviews, and editor M. B. Allen for his editorial handling of and insightful comments on our manuscript.
Supplementary material
To view supplementary material for this article, please visit https://doi.org/10.1017/S0016756816001230