Introduction
Eosinophils are granulocytes present in bone marrow are derived from pluripotent progenitors and represent 5% of the total leucocytes in the peripheral blood circulation. These leucocytes secrete diverse granules composed of cationic eosinophil proteins, eosinophil peroxidases and eosinophil-derived neurotoxins. Eosinophils are activated and recruited into the tissue in response to stimuli such as the cytokine interleukin 5 (IL-5) and chemokines (Rosenberg et al., Reference Rosenberg, Dyer and Foster2013; Obata-Ninomiya et al., Reference Obata-Ninomiya, Domeier and Ziegler2020). In response to allergic insult or some types of infections, eosinophils are recruited to the sites of inflammation secreting their granules, contributing as a source of multiple cytokines, such as IL-2, IL-4, IL-5, IL-10, IL-12, IL-13, IL-16, IL-18, transforming growth factor-β1 and lipid mediators, in addition to being able to induce tissue damage and dysfunction (Rothenberg and Hogan, Reference Rothenberg and Hogan2006). In allergic processes, an increase in eosinophils in the peripheral blood is typical as in helminth infections. These cells are known to promote effector immunity against these parasites (Weller and Spencer, Reference Weller and Spencer2017). However, studies suggest that in some specific helminths, eosinophils may subsidize the permanence and favour the longevity of parasites in the host, but the role of eosinophils in these infections remains undefined (Swartz et al., Reference Swartz, Dyer, Cheever, Ramalingam, Pesnicak, Domachowske, Lee, Lee, Foster, Wynn and Rosenberg2006; Rosenberg et al., Reference Rosenberg, Dyer and Foster2013).
During cell development in the bone marrow, eosinophils have properties shared with basophils, and their differentiation occurs due to the presence of transcription factors PU.1, CCAAT/enhancer-binding protein and GATA-binding factor 1 (GATA-1). Eosinophils have a high affinity palindromic (or double) site of GATA-1 located in the gene regulatory region. In mice, it is possible to perform the deletion of the GATA-1 transcription factor interrupting eosinophilic cell maturation, resulting in complete or selective deficiency for eosinophil differentiation. In this sense, these mice are essential for studying eosinophil-related functions and pathologies (Yu et al., Reference Yu, Cantor, Yang, Browne, Wells, Fujiwara and Orkin2002; Rothenberg and Hogan, Reference Rothenberg and Hogan2006).
In murine models, the protective role of eosinophils has been shown to prevent secondary infection by Trichinella spiralis, and in humans with Schistosomiasis, an increase in eosinophils after treatment with praziquantel has been correlated with resistance to reinfection (Hagan et al., Reference Hagan, Wilkins, Blumenthal, Hayes and Greenwood1985; Huang et al., Reference Huang, Gebreselassie, Gagliardo, Ruyechan, Luber, Lee, Lee and Appleton2015; Huang and Appleton, Reference Huang and Appleton2016). However, in mice infected with Schistosoma mansoni, ablation of eosinophil had no significant impact on disease development during infection (Swartz et al., Reference Swartz, Dyer, Cheever, Ramalingam, Pesnicak, Domachowske, Lee, Lee, Foster, Wynn and Rosenberg2006). In eosinophil genetically deficient mice, it was shown that T. spiralis larvae died in large numbers showing that death of the parasites was correlated with a reduced recruitment of T-helper 2 (Th2) cells and a concomitant increased activity of the inducible nitric oxide synthase and synthesis of nitric oxide by macrophages (Fabre et al., Reference Fabre, Beiting, Bliss, Gebreselassie, Gagliardo, Lee, Lee and Appleton2009; Gebreselassie et al., Reference Gebreselassie, Moorhead, Fabre, Gagliardo, Lee, Lee and Appleton2012).
Toxocariasis is a zoonosis whose primary aetiological agent is Toxocara canis. It is a neglected, cosmopolitan disease with a 19% seroprevalence worldwide (Rostami et al., Reference Rostami, Riahi, Holland, Taghipour, Khalili-Fomeshi, Fakhri, Omrani, Hotez and Gasser2019). Humans are infected by accidental ingestion of eggs containing infective T. canis larvae, which penetrate the mucosa and migrate to various tissues. The immunological mechanism capable of eliminating T. canis larvae remains unclear. However, studies showed that Th2 and Th17 immune responses are triggered during infection, and there is an increase in innate immunity cells, especially eosinophils (Resende et al., Reference Resende, Gazzinelli-Guimarães, Barbosa, Oliveira, Nogueira, Gazzinelli-Guimarães, Gonçalves, Amorim, Oliveira, Caliari, Rachid, Volpato, Bueno, Geiger and Fujiwara2015; Leal-Silva et al., Reference Leal-Silva, Vieira-Santos, Oliveira, Padrão, Kraemer, da Paixão Matias, de Almeida Lopes, Loiola Ruas, de Azevedo, Nogueira, Rachid, Caliari, de Castro Russo, Fujiwara and Bueno2021). We have recently demonstrated that ST2−/− receptor deficiency reduced the parasite load combined with reduced eosinophils during T. canis infection in mice (Leal-Silva et al., Reference Leal-Silva, Vieira-Santos, Oliveira, Padrão, Kraemer, da Paixão Matias, de Almeida Lopes, Loiola Ruas, de Azevedo, Nogueira, Rachid, Caliari, de Castro Russo, Fujiwara and Bueno2021), suggesting that eosinophils may be relevant in the framework of T. canis infection.
Studies regarding immune responses in toxocariasis are scarce, and the role of eosinophils during this infection has not yet been defined. In this context, the present study aimed to determine the role of eosinophils during the immunopathology caused by T. canis infection using a murine experimental model. Our results revealed that eosinophils favour T. canis larvae tropism during infection, increasing the tissue damage with induction of hepatic and pulmonary granulomas and brain haemorrhage in mice. We concluded that eosinophil cells are deleterious in the context of T. canis infection in mice.
Materials and methods
Parasites
Adult T. canis worms were collected from the feces of naturally infected puppies and were treated with anthelmintics (Drontal Puppy, Brazil) at a dosage of 1 mL kg−1 in the Zoonoses Control Center (Belo Horizonte, Minas Gerais, Brazil). The adult worms were placed in water and taken to the Laboratory of Immunology and Genomics of Parasites at the Federal University of Minas Gerais for processing. The eggs were isolated from the uteri of female adult worms by mechanical maceration, purified by filtration using 100 μm nylon strainers, placed in culture flasks with 50 mL of 0.2 m sulphuric acid and maintained in a Bio-Oxygen Demand incubator at 26 C. The eggs remained in the culture until their embryonic period, which took approximately 6 weeks (Leal-Silva et al., Reference Leal-Silva, Vieira-Santos, Oliveira, Padrão, Kraemer, da Paixão Matias, de Almeida Lopes, Loiola Ruas, de Azevedo, Nogueira, Rachid, Caliari, de Castro Russo, Fujiwara and Bueno2021).
Experimental design
Eight-week-old female mice of wild-type (WT) BALB/c and mice genetically deficient for the transcription factor GATA1−/− were maintained in an animal facility of the Department of Parasitology of the Federal University of Minas Gerais under controlled conditions of temperature (24 ± 1 C) and lighting (12-h light–dark cycle), and were provided with filtered water and commercial food (Nuvital Nutrients, Brazil) ad libitum.
Each of the two WT and GATA1−/− mice strains was randomly divided into four experimental groups: 0 days post-infection (dpi) (control), 3, 14 and 63 dpi. Each group used a total of 12 mice, divided into two groups containing six mice for immunological and histological analyses and six for parasitological and biochemical analyses (Fig. 1). All mice were euthanized with a lethal injection of xylazine/ketamine (8.5 and 130 mg kg−1).

Fig. 1. Characterization of the experimental design of Toxocara canis infection. WT and GATA1−/− mice were infected with 1000 eggs of T. canis and euthanized with 0, 3, 14 and 63 dpi.
Toxocara canis infection
For Toxocara infection, before inoculation, the fully embryonated eggs were incubated with a 5% sodium hypochlorite solution in an incubator [37 C and 5% carbon dioxide (CO2)] for 1 h 40 min to disrupt the outer layer of the eggs and facilitate in vivo larval hatching. After incubation, eggs were resuspended and washed with phosphate-buffered saline (PBS) (0.4 m NaCl and 10 mm NaPO4) three times. All mice, except the 0 dpi group (control), were inoculated by oral gavage with 0.2 mL of the solution containing 1000 embryonated eggs.
Parasitological analysis
Parasite burdens from infected mice were evaluated by counting the total number of larvae recovered from the liver, lungs and brain. Tissues were collected, punctured with surgical scissors and placed in a modified Baermann-Moraes apparatus for 4 h in PBS at 37 C and 5% CO2. The larvae recovered from the pellet of the device were fixed in 10% formalin and quantified by light microscopy.
Analysis of leucocytes and liver enzymes
For the total and differential leucocyte counts, blood samples of the mice were collected by cardiac puncture, placed in tubes with ethylenediamine tetraacetic acid (EDTA) and were analysed in an automatic haematology counter (Bio-2900 Vet, Bioeasy, USA) for a global leucocyte count. Blood smears stained with Panotic (Laborclin, Brazil) were used for differential cell count under microscopy.
The blood was centrifuged, and the plasma was collected to quantify the liver enzyme activity, aspartate aminotransferase (AST), using a colorimetric enzymatic assay employing a commercial kit (Bioclin Quibasa, Brazil) according to the manufacturer's instructions.
Bronchoalveolar lavage (BAL)
BAL was collected from mice with the assistance of a 1.7 mm catheter inserted into the trachea, and then 2 mL PBS was added through the catheter to collect the BAL fluid. The lavage fluid was centrifuged at 300 g for 10 min, and the supernatants were collected to estimate protein and haemoglobin levels by using a commercial kit according to the manufacturer's instructions. The pellets were used to quantify total and differential cellularity using light microscopy as previously described (Oliveira et al., Reference Oliveira, Matias, Kraemer, Gazzinelli-Guimarães, Santos, Amorim, Nogueira, Freitas, Caliari, Bartholomeu, Bueno, Russo and Fujiwara2019).
Cytokine profile
The right lobe of the mice lung tissue was removed to determine tissue cytokine concentration. First, 100 mg of tissue was weighed and then homogenized (TissueLyser LT-Qiagen, Germany) in 1 mL of extraction solution with protease inhibitors (0.4 m NaCl, 0.05% Tween 20, 0.5% bovine serum albumin, 0.1 mm phenylmethylsulphonyl fluoride, 0.1 mm benzethonium chloride, 10 mm EDTA and 20 IU aprotinin A). The homogenate was centrifuged at 800 g for 10 min at 4 C and the supernatant was used to determine the cytokines IL-1β, tumour necrosis factor-α, IL-6, IL-4, IL-33, IL-13, IL-5, IL-10 and IL-17A by enzyme-linked immunoassay (ELISA) (R&D Systems, USA) according to the manufacturer's protocol.
Neutrophil myeloperoxidase (MPO) and macrophage N-acetylglucosaminidase (NAG) assays
The indirect activity of macrophages and neutrophils was assessed by using the concentrations of NAG and MPO, respectively, in the liver, lungs and brain homogenates according to the method previously described (Barcelos et al., Reference Barcelos, Talvani, Teixeira, Vieira, Cassali, Andrade and Teixeira2005; Nogueira et al., Reference Nogueira, Gazzinelli-Guimarães, Barbosa, Resende, Silva, de Oliveira, Amorim, Oliveira, Mattos, Kraemer, Caliari, Gaze, Bueno, Russo and Fujiwara2016). After the tissues were homogenized (TissueLyser LT-Qiagen, Hilden, Germany), the homogenate was centrifuged at 800 g for 10 min at 4 C, and the pellet was used to determine NAG and MPO activity. Absorbance was expressed by using a VersaMax ELISA microplate reader (Molecular Devices, USA) according to each assay, and the results were expressed as optical densities.
Histopathological analysis
The left lung, right liver lobe and left half of the brain were collected from mice to analyse the histological changes. The organs were fixed in a 4% formalin solution, gradually dehydrated in ethanol before being cleared in xylene and embedded in paraffin blocks that were cut 4–5 μm thick and fixed on the microscope slide. The tissue slides were stained with haematoxylin and eosin to assess tissue damage under microscopy, and all histopathological analyses were subjected to a blind study..
For the analysis of liver lesions, ten random images per mouse were captured at 20× magnification. The score was based on four grades for liver parenchymal damage: grade 0, no inflammatory cells; grade 1, regions of the liver parenchyma with small inflammatory foci and reduced number of inflammatory cells with small areas of necrosis; grade 2, the liver parenchyma showed inflammatory foci with a moderate number of cells, perivascular inflammatory infiltrate and around the ducts with small areas of necrosis dispersed throughout the parenchyma; grade 3, the liver parenchyma often had larger inflammatory foci, diffuse inflammatory infiltrate, exuberant perivascular inflammation and around the ducts, areas of necrosis dispersed throughout the parenchyma (Leal-Silva et al., Reference Leal-Silva, Vieira-Santos, Oliveira, Padrão, Kraemer, da Paixão Matias, de Almeida Lopes, Loiola Ruas, de Azevedo, Nogueira, Rachid, Caliari, de Castro Russo, Fujiwara and Bueno2021). Hepatic and pulmonary granuloma counts were also obtained.
For the airway inflammation score, ten random images per mouse were captured at 20× magnification and analysed for perivascular inflammation, peribronchial inflammation, parenchymal damage and haemorrhage (Gazzinelli-Guimarães et al., Reference Gazzinelli-Guimarães, Gazzinelli-Guimarães, Nogueira, Oliveira, Barbosa, Amorim, Cardoso, Kraemer, Caliari, Akamatsu, Ho, Jones, Weatherhead, Bottazzi, Hotez, Zhan, Bartholomeu, Russo, Bueno and Fujiwara2018). For the analysis of cellular lesions of the whole brain, the score was based on the intensity of the lesion: 0: none; 1: minimum; 2: light; 3: moderate; 4: intense. All semi-quantitative analyses of the slides were conducted under microscopy coupled with a digital image capture system (Motic 2.0, Xiam, China).
Statistical analysis
For statistical analysis, Prism 8.0 software (GraphPad Inc., USA) was used. To detect possible outliers, the Grubb test was used. To verify data distribution, the Shapiro–Wilk test was used. An analysis of variance (ANOVA) test was used for the comparison between the control groups (0 dpi) and each time of infection, followed by the Tukey multiple comparisons post-test for parametric data or the non-parametric Kruskal–Wallis test followed by the Dunn's post-test. All values were considered significant when the P value was ≤0.05.
Results
Tissue eosinophilia induces granuloma formation but does not control the T. canis larval migration in mice liver
In this study, we demonstrate the role of eosinophils in the immunopathology of T. canis infection. After passing through the intestine, Toxocara larvae migrates to the liver tissue. No significant differences were observed between the parasite load in the liver and the AST enzyme throughout the infection (Fig. 2A and B). However, the uninfected GATA1−/− mice showed a basal increase in NAG at 0 dpi persisting during the course of infection (Fig. 2C). The neutrophilic activity was reduced in the acute phase of infection, and remains until the chronic phase compared to WT mice (Fig. 2D). In the score analysis, granulomas were observed only in WT mice at 14 and 63 dpi, and no difference was observed between strains in the total inflammation score (Fig. 2E and F).
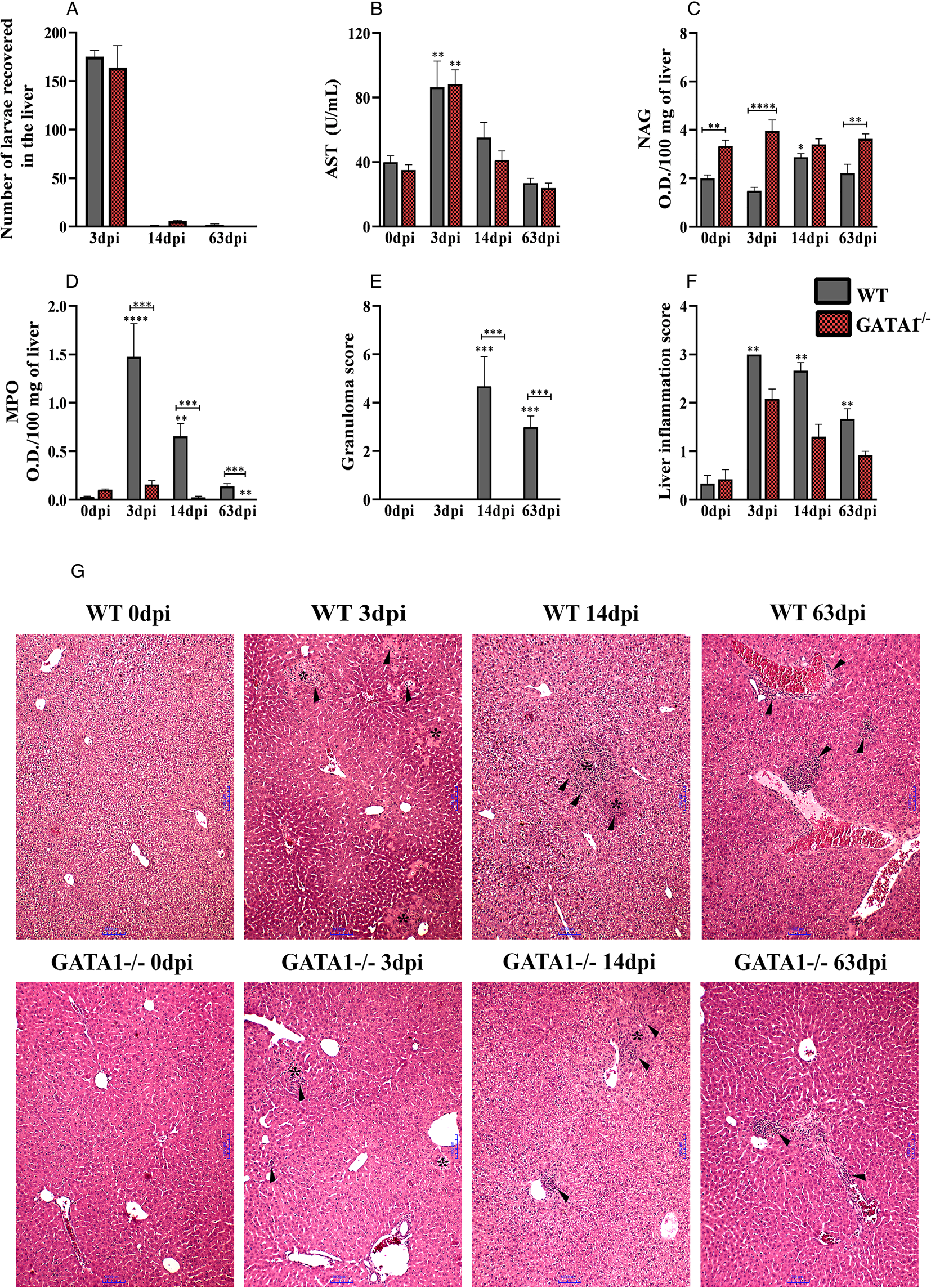
Fig. 2. Liver alterations in WT and GATA1−/− mice infected with T. canis. (A) The number of larvae recovered from the liver; (B) analysis of the enzymatic activity of plasma AST; (C) NAG activity in the liver; (D) MPO activity in the liver; (E) number of granulomas; (F) analysis of liver inflammation by score and (G) representative haematoxylin and eosin staining of liver sections, foci of inflammatory infiltration (arrowheads), area of necrosis (*). Bar = 1000 μm. Statistical comparisons were carried out between each strain with its specific uninfected group (0 dpi) represented by the asterisk without the bar and between the strains at the same time of infection represented by the asterisk with the bar. The results represent the mean ± s.e.m., *P < 0.05, **P < 0.01, ***P < 0.001, ****P < 0.0001. One-way ANOVA test and Kruskal–Wallis test followed by Dunn's test were used.
The histopathological analysis of the liver showed that, in the uninfected mice, both strains retained the hepatocytes with a morphological aspect compatible with normality (Fig. 2G). At 3 dpi, it was observed in some mice in both strains, the larvae dispersed throughout the parenchyma. In WT mice, there were inflammatory foci comprising eosinophils, neutrophils, macrophages and lymphocytes dispersed throughout the parenchyma and around larvae and the blood vessels, in addition to abundant necrosis zones and parenchyma haemorrhage. In GATA1−/− mice, the inflammatory foci comprised of a significant proportion of neutrophils, scarce macrophages and dispersed lymphocytes, and inflammatory infiltrate around larvae, blood vessels, bile ducts and permeating areas of necrosis were also frequently identified. In both strains, the blood vessel congestion induced by infection was observed throughout the hepatic parenchyma. At 14 dpi in WT mice, the presence of granulomas was found, which were in the exudative phase, composed mostly of eosinophils, followed by macrophages, necrotic-exudative and necrotic zones. In both strains of mice, it was possible to identify the presence of inflammatory foci dispersed throughout the hepatic and perivascular parenchyma, consisting of macrophages, low lymphocytes and eosinophils, the latter being observed only in WT mice. In the GATA1−/− group at 14 dpi, the presence of small foci of necrosis near ducts and larger blood vessels, and the presence of fibroblasts and collagen fibre deposition were found, characterizing early tissue fibroplasia. In the chronic phase of infection, in WT mice at 63 dpi, the inflammatory foci were scattered throughout the hepatic and perivascular parenchyma. The presence of granulomas was also found, which were in the exudative and productive phases, consisting of macrophages, giant cells, epithelioid cells, fibroblasts and collagen. While in the GATA1−/− mice at 63 dpi, it was possible to identify only the presence of small inflammatory foci dispersed throughout the liver parenchyma, with macrophages and low lymphocyte numbers, and no exudative phenomena or foci of necrosis were observed. Collectively, these data suggest that eosinophils are primarily associated with the presence of hepatic granulomas during T. canis infection, in addition to influencing neutrophil activity.
Eosinophil deficiency is related to increased neutrophil and reduced pulmonary parasite load leading to secondary pulmonary tissue damage during the acute phase of the T. canis infection in mice
The larvae migrate from the liver to the lung tissue via blood after 3 days of infection (Fig. 3A–I). By analysing the pulmonary parasite load, we observed that GATA1−/− mice showed a reduction in the number of larvae recovered from the lungs at 3 dpi and consequently reduced the concentrations of haemoglobin and protein in BAL when compared to WT mice (Fig. 3A–C). Regarding the leucocytes in BAL (Fig. 3D–H), we observed an increase in the number of total leucocytes in the GATA1−/− mice, due to the increased number of neutrophils. However, at 14 and 63 dpi, there was a reduction in leucocytes in GATA1−/− compared to the WT mice due to the reduced number of neutrophils, macrophages and the absence of eosinophils. In the lung parenchyma, aspects related to tissue inflammation were evaluated (Fig. 4A–H). A reduction in macrophage activity at 3 dpi, haemorrhage score at 63 dpi and granuloma score at 14 and 63 dpi were observed in GATA1−/− mice when compared to WT mice, demonstrating reduced tissue inflammation.

Fig. 3. Number of larvae recovered from lungs and airway inflammation. (A) The number of larvae recovered from the lung; (B) haemoglobin concentration in the BAL; (C) total protein concentration in BAL; (D) number of total leucocytes in BAL; (E) number of eosinophils in BAL; (F) number of neutrophils in BAL; (G) number of macrophages in BAL; (H) number of lymphocytes in BAL and (I) larvae recovered from the lung tissue of WT mice infected with T. canis. Bar = 100 μm. Statistical comparisons were carried out between each strain with its specific uninfected group (0 dpi) represented by the asterisk without the bar and between the strains at the same time of infection represented by the asterisk with the bar. The results represent the mean ± s.e.m., *P < 0.05, **P < 0.01, ***P < 0.001, ****P < 0.0001. One-way ANOVA test and Kruskal–Wallis test followed by Dunn's test were used.
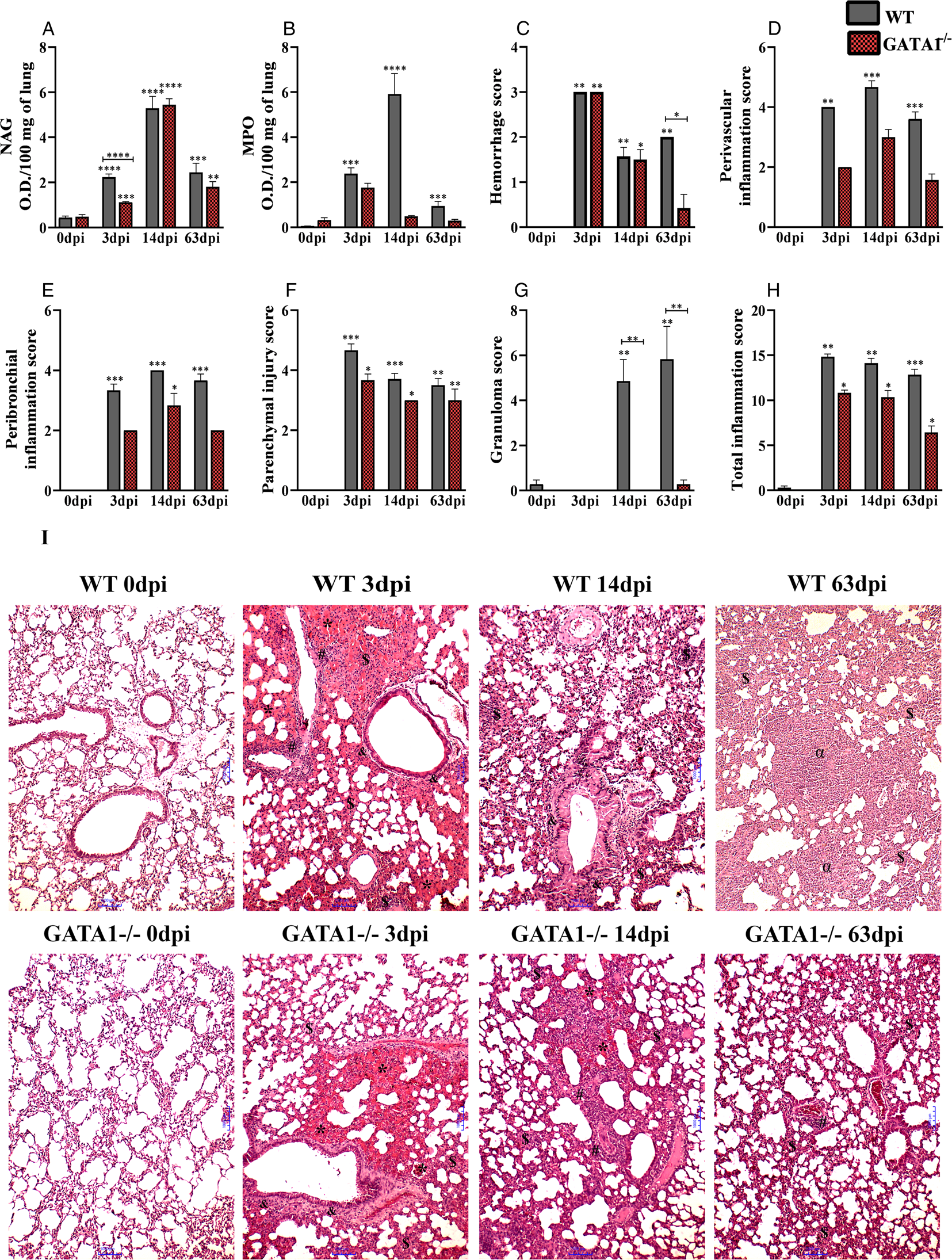
Fig. 4. Inflammation of the lung parenchyma in WT and GATA1−/− mice infected with T. canis. (A) NAG activity in lung tissue; (B) MPO activity in lung tissue; (C) bleeding score; (D) perivascular inflammation score; (E) peribronchial inflammation score; (F) parenchymal injury score; (G) granuloma score; (H) total inflammation score and (I) representation of haematoxylin and eosin staining of lung sections, haemorrhage area (*), parenchymal inflammation ($), airway inflammation (&), vascular inflammation (#), granuloma (α), bar = 1000 μm. Statistical comparisons were carried out between each strain with its specific uninfected group (0 dpi) represented by the asterisk without the bar and between the strains at the same time of infection represented by the asterisk with the bar. The results represent the mean ± s.e.m., *P < 0.05, **P < 0.01, ***P < 0.001, ****P < 0.0001. One-way ANOVA test and Kruskal–Wallis test followed by Dunn's test were used.
The analysis of lungs of the uninfected mice showed compatibility with normality (Fig. 4I). In the WT mice group at 3 dpi, thickening of the interalveolar septa was observed, with the presence of the mixed inflammatory infiltrate mostly composed of eosinophils and neutrophils, macrophages and to a lesser extent by lymphocytes. In some mice, granuloma was observed in the exudative phase evidenced by perivascular oedema and extensive bleeding areas. Most mice had larvae dispersed in the lung parenchyma and often close to the haemorrhagic zones. In the GATA1−/− mice group at 3 dpi, a significant thickening of the interalveolar septa was observed due to the predominantly mononuclear inflammatory infiltrate characterized by macrophages and lymphocytes, with a lower frequency of neutrophils. Exudative phenomena were also evidenced, such as oedema present in the alveolar and perivascular lumen, large areas of haemorrhage and congested vessels. At 14 dpi, we observed interalveolar septal thickening in both lineages due to the presence of inflammatory infiltrate, and the bronchial and bronchiolar epithelium cells showed intense hypertrophy and hyperplasia, and the formation of a mucus plug obstructing the bronchial lumen was frequently observed. In WT mice at 14 dpi, some macrophages presented with a brownish pigment in their cytoplasm suggestive of haemosiderin, and the presence of parenchyma granuloma in the exudative phase was frequently observed. In WT mice at 63 dpi, the presence of diffuse inflammatory infiltrate was observed, and formation of bronchus-associated lymphoid tissue was evidenced in some of them. In all mice, it was possible to identify the presence of granulomas in the productive phase in the lung parenchyma and exudative and vascular phenomena such as small haemorrhagic foci and capillary congestion. While in GATA1−/− mice at 63 dpi, the substantial presence of lymphocytes was observed around the vessels, and in some vessels, the presence of hypertrophy and hyperplasia of bronchial epithelial cells was observed. Sometimes, in some parenchyma regions, the presence of extravasated red blood cells was observed in a small proportion. These results indicate that eosinophils are ineffective at controlling the migration of T. canis larvae in lung tissue and contribute to attenuating the lung inflammation reducing the number of neutrophils and secondary tissue damage. However, the neutrophils may be important to control the infection.
Eosinophil influences the pulmonary immune profile during T. canis infection
We analysed the immune response in the lung parenchyma by measuring cytokines from the Th1, Th2, Th17 and regulatory T (Treg) profiles (Fig. 5). Both mice strains show a mixed type of immune response, with increased Th1, Th2 and Th17 cytokines compared to their respective controls. When comparing the two strains, we observed that the IL-13 cytokine increased in the uninfected GATA1−/− mice (0 dpi) compared to WT mice. At 3 dpi, it was observed that the cytokines IL-6, IL-4, IL-5 and IL-13 increased in the GATA1−/− mice compared to WT mice. In the later stages of infection, GATA1−/− mice showed an increased IL-1β, IL-6, IL-4, IL-5, IL-13 and IL-10 cytokines and reduced Th17 response at 14 dpi compared to WT mice. Thus, the results demonstrate that the absence of eosinophils favours secretion of Th2 cytokines in special IL-13 which explains the excessive mucus production. Finally, eosinophil deficiency increases the pro-inflammatory cytokines IL-1β and IL-6 during T. canis infection and may sustain the neutrophilic inflammation and tissue damage in mice.

Fig. 5. Concentration of cytokines present in the lung tissue of WT and GATA1−/− mice during T. canis infection. Radar graphics express the concentration of pulmonary cytokines throughout the infection. The results represent the mean ± s.e.m., *P < 0.05, **P < 0.01, ***P < 0.001, ****P < 0.0001 when compared to the corresponding WT mice groups. Cytokines were underlined when they presented statistical differences with their respective control group (0 dpi). One-way ANOVA test and Kruskal–Wallis test followed by Dunn's test were used.
Eosinophil deficiency changes the peripheral blood leucocytosis during the T. canis infection in mice
To understand the influence of eosinophils on systemic inflammation, the leucocyte profile in peripheral blood throughout the infection was evaluated (Fig. 6). GATA1−/− mice demonstrated a physiological increase in monocytes and neutrophils compared to WT mice, as observed in uninfected mice, but at no time of infection leucocytosis was observed in GATA1−/− mice, while in WT mice leucocytosis was observed at 14 and 63 dpi. At 3 dpi, an increase in lymphocytes and monocytes and a reduction in neutrophils at 14 dpi was observed in GATA1−/− mice compared to WT mice. As expected, no eosinophils were observed in GATA1−/− mice, while in WT mice, there was eosinophilia at 14 and 63 dpi. Thus, larval migration in eosinophil-deficient mice led to an increased liver and lung tissue damage which could be associated with chronic neutrophilic inflammation, which anticipated the peak of systemic leucocytosis in GATA1−/− mice.

Fig. 6. White blood cells of WT and GATA1−/− mice infected with T. canis. Leucogram were shown after 0, 3, 14 and 63 dpi by T. canis. Statistical comparisons were carried out between each strain with its specific uninfected group (0 dpi) represented by the asterisk without the bar and between the strains at the same time of infection represented by the asterisk with the bar. The results represent the mean ± s.e.m., *P < 0.05, **P < 0.01, ***P < 0.001, ****P < 0.0001. One-way ANOVA test and Kruskal–Wallis test followed by Dunn's test were used.
Absence of eosinophils reduced the parasite load and increased neutrophil activity in brain tissue during T. canis infection in mice
We assessed the parasite load, macrophage and neutrophil activity and haemorrhage score in brain tissue (Fig. 7A–D). The results showed that a reduced number of larvae was recovered from the brain of GATA1−/− mice at 63 dpi compared to WT mice, which may be attributed to the increase in neutrophil activity observed at the same time of infection. No difference in macrophage activity and haemorrhage score was observed between the groups.

Fig. 7. Parasite burden and inflammation of brain tissue during T. canis infection. (A) The number of larvae recovered from the brain; (B) MPO activity in brain tissue; (C) NAG activity in brain tissue; (D) haemorrhage score and (E) representation of haematoxylin and eosin staining of brain sections, haemorrhage area (*), bar = 1000 μm. Statistical comparisons were carried out between each strain with its specific uninfected group (0 dpi) represented by the asterisk without the bar and between the strains at the same time of infection represented by the asterisk with the bar. The results represent the mean ± s.e.m., *P < 0.05, **P < 0.01, ***P < 0.001, ****P < 0.0001. One-way ANOVA test and Kruskal–Wallis test followed by Dunn's test were used.
Histopathological analysis of the brains of uninfected mice showed a morphological aspect compatible with normality (Fig. 7E). In WT mice at 3 and 14 dpi, foci of haemorrhage and vacuolization and small inflammatory foci were observed. At 63 dpi, there were more areas of vacuolization, multifocal areas of haemorrhage and inflammatory foci. In GATA1−/− mice, the histopathological parameters were minimal and were characterized by haemorrhage, vacuolization and focal inflammation in all infected groups.
Discussion
This study is the first to explain in detail about the influence of eosinophils on liver, lung and brain tissues, evaluating the immunological and pathological aspects during T. canis infection in mice. This study found that eosinophils do not promote effective immunity against the parasites. On the contrary, the presence of eosinophils favours the survival and tropism of T. canis larvae into the lungs and brain tissues. Eosinophils also mainly trigger the formation of granulomas in toxocariasis which cannot control larval migration, and they mediate a Th17 inflammatory response that generates tissue damage.
T. canis larvae first targets the liver tissue both in dogs being the definitive host and in accidental hosts as well. In experimental models, in the initial stages of infection, the presence of liver damage has been demonstrated, with inflammatory infiltrate with the presence of eosinophils, neutrophils and polymorphonuclear cells, with no significant contribution of lymphocytes (Resende et al., Reference Resende, Gazzinelli-Guimarães, Barbosa, Oliveira, Nogueira, Gazzinelli-Guimarães, Gonçalves, Amorim, Oliveira, Caliari, Rachid, Volpato, Bueno, Geiger and Fujiwara2015), as observed in the mice in this study, except for the presence of eosinophils in GATA1−/− mice. In the late phase of the infection, granulomatous processes are present in the exudative and productive phases (Fan et al., Reference Fan, Lin, Hung, Chang and Su2004; Leal-Silva et al., Reference Leal-Silva, Vieira-Santos, Oliveira, Padrão, Kraemer, da Paixão Matias, de Almeida Lopes, Loiola Ruas, de Azevedo, Nogueira, Rachid, Caliari, de Castro Russo, Fujiwara and Bueno2021). This study showed that eosinophils are the main triggers for the formation of granulomas in T. canis infection. In the eosinophil deficiency, the presence of hepatic granulomas was not observed. Interestingly, we observed that the neutrophil activity was shown to be reduced in GATA1−/− compared to WT mice. Neutrophils and eosinophils have been shown to be involved in adherence to helminths, including T. canis larvae (Huwer et al., Reference Huwer, Sanft and Ahmed1989). Furthermore, high neutrophil counts that followed the migration of larvae through organs and tissues were observed in T. canis infection, probably acting as the first line of defense against T. canis larvae, as proposed for protozoan parasites (Hermosilla et al., Reference Hermosilla, Caro, Silva, Ruiz and Taubert2014; Resende et al., Reference Resende, Gazzinelli-Guimarães, Barbosa, Oliveira, Nogueira, Gazzinelli-Guimarães, Gonçalves, Amorim, Oliveira, Caliari, Rachid, Volpato, Bueno, Geiger and Fujiwara2015).
This study revealed a reduction in the pulmonary parasite load in GATA1−/− mice at 3 dpi and consequently reduced concentrations of haemoglobin and protein. Interestingly, an inverse relationship was detected between the number of neutrophils present in the airways and the number of larvae recovered from the tissues, suggesting that neutrophils may be necessary for controlling the parasite load. In addition, in WT mice at 3 dpi, even after obtaining an increased number of eosinophils, we still observed a high parasite load, demonstrating that eosinophils were not able to combat the T. canis larvae effectively. Unlike other nematodes, in toxocariasis, there seems to be no relation between eosinophils and larval death, and quite possibly, this reflects the evasion capacity of T. canis larvae. It was demonstrated in vitro that eosinophils from humans with toxocariasis in the presence of infective T. canis larvae were able to adhere to the surface of the larvae, form close interdigitations with the cuticular streaks of the larvae, degranulate on the surface of the parasite and the larvae still remained alive, in addition, some larvae have been shown to be able to escape from the areas of dense eosinophil/parasite binding (Fattah et al., Reference Fattah, Maizels, McLaren and Spry1986). In vitro studies have also indicated that neutrophils are not able to kill T. canis larvae (Huwer et al., Reference Huwer, Sanft and Ahmed1989). However, it is known that the in vivo and in vitro responses are different and that leucocytes are plastic and heterogeneous, being able to adapt to microenvironments, modifying their phenotypic properties and functions (Natoli and Ostuni, Reference Natoli and Ostuni2019; Ng et al., Reference Ng, Ostuni and Hidalgo2019). Thus, we cannot rule out the possibility that neutrophils are active and important immune cells involved in T. canis larvae immune response and parasite control.
In addition to a reduction in the formation of hepatic granuloma in infected GATA1−/− mice, a reduction in the formation of pulmonary granulomas was also observed, reaffirming the role of eosinophils in the formation of granulomas. In T. canis infection, the larvae trigger the granuloma formation in the lung tissue, which is mainly composed of eosinophils and later on by macrophages. Granulomas are formed due to the chronic persistence of the antigen or parasite in an attempt to contain it, and their formation involves the interaction between the organism that acts as an antigen and the immune cells of the host. The problem is that their presence usually results in scar tissue formation, which ends up reducing the organ's function (Kayes and Adams Oaks, Reference Kayes and Adams Oaks1978; Sales et al., Reference Sales, Tristão, Rocha, Carlos, Ketelut-carneiro, Oliveira, Souza, Milanezi, Silva and Hube2017; Ariyaratne and Finney, Reference Ariyaratne and Finney2019). Furthermore, inadequate accumulation and activation of eosinophils can result in direct tissue damage through the release of highly cytotoxic granular proteins (Rankin et al., Reference Rankin, Conroy and Williams2000).
Several studies have reported an increase in cytokines Th1, Th2, Th17 and Treg responses in T. canis infection, demonstrating that during infection, there is no polarized immune response (Liao et al., Reference Liao, Fan, Kao, Ji, Su, Lin and Cho2008; Nagy et al., Reference Nagy, Bede, Danka, Szénási and Sipka2012; Resende et al., Reference Resende, Gazzinelli-Guimarães, Barbosa, Oliveira, Nogueira, Gazzinelli-Guimarães, Gonçalves, Amorim, Oliveira, Caliari, Rachid, Volpato, Bueno, Geiger and Fujiwara2015; Leal-Silva et al., Reference Leal-Silva, Vieira-Santos, Oliveira, Padrão, Kraemer, da Paixão Matias, de Almeida Lopes, Loiola Ruas, de Azevedo, Nogueira, Rachid, Caliari, de Castro Russo, Fujiwara and Bueno2021). This study observed that the absence of eosinophils alters the immune profile during infection, inducing an increase in IL-1β, IL-6, IL-4, IL-5, IL-13 and IL-10. The cytokines IL-4, IL-5 and IL-13 are classified as markers of the Th2 type of immune response and influence the eosinophilic response. IL-5 has been identified as a critical regulator in eosinophil development, migration and activation. At the same time, IL-4 and IL-13 are essential for the recruitment of these cells into lung tissue (Foster et al., Reference Foster, Mould, Yang, Mackenzie, Mattes, Hogan, Mahalingam, Mckenzie, Rothenberg, Young, Matthaei and Webb2001), so we believe that the increase in these cytokines in the infected GATA1−/− mice is an immunological mechanism of trying to amplify eosinophilic activity. The cytokine IL-6 in lung tissue is produced from epithelial cells, interstitial fibroblasts, macrophages and other inflammatory cells in response to a variety of stimuli such as environmental particles and inhaled toxic particles, having the function of regulating T cell-mediated responses CD4+ which include the production of cytokines such as IL-4, IL-13, IL-17, IL-21 and suppressor activity in Treg cells (Kaur et al., Reference Kaur, Bansal, Kumar and Bansal2020). IL-10 is expressed by many adaptive immune system cells, including Th1, Th2, Th17, Treg, CD8+ T, B cells and cells of the innate immune system. Moreover, its primary role appears to be as a feedback regulator of various immune responses, not only Th1 cell responses but also Th2 cell responses to parasites (Saraiva and O'Garra, Reference Saraiva and O'Garra2010). Studies have indicated that IL-1β in the presence of type 2 cytokines may also support Th2-mediated immunity (Helmby and Grencis, Reference Helmby and Grencis2004; Ferguson et al., Reference Ferguson, Newland, Gibbs, Tourlomousis, Fernandes dos Santos, Patel, Hall, Walczak, Schramm, Haas, Dunne, Cooke and Zaccone2015). In our previous study with mice infected with T. canis and genetically deficient for the ST2 receptor, which is a ligand of IL-33 and, therefore, has reduced eosinophils, an increase in IL-1β concentration was also observed in later times of infection (Leal-Silva et al., Reference Leal-Silva, Vieira-Santos, Oliveira, Padrão, Kraemer, da Paixão Matias, de Almeida Lopes, Loiola Ruas, de Azevedo, Nogueira, Rachid, Caliari, de Castro Russo, Fujiwara and Bueno2021), suggesting that eosinophils may influence the release of IL-1β. Thus, we observe that the absence of eosinophils increases the secretion of pulmonary cytokines from the innate and the Th2 immune responses.
During T. canis infection, an increase in blood leucocytes is often observed, with an increase in neutrophils, followed by eosinophilia. In mice, the peak eosinophilia is usually observed between 14 and 18 dpi, but it can persist to the chronic phase at 45 dpi (Pecinali et al., Reference Pecinali, Gomes, Amendoeira, Bastos, Martins, Pegado, Bastos, Bozza and Castro-Faria-Neto2005; Margareth et al., Reference Rodolpho, Oliveira, Souza, Dejani, Neris, Correia, Zaia, Faccioli, Melo and Anibal2014; Resende et al., Reference Resende, Gazzinelli-Guimarães, Barbosa, Oliveira, Nogueira, Gazzinelli-Guimarães, Gonçalves, Amorim, Oliveira, Caliari, Rachid, Volpato, Bueno, Geiger and Fujiwara2015). In this study, eosinophilia was observed from 14 dpi, persisting up to 63 dpi in WT mice. On the contrary, in the infected GATA1−/− mice, leucocytosis was not observed at any time of infection, probably due to the absence of eosinophils.
In neuro-toxocariasis, haemorrhage has been shown to be the main histopathological finding, extending for long periods after infection. The presence of activated microglia and focal accumulation of gitter cells has also been related (Janecek et al., Reference Janecek, Beineke, Schnieder and Strube2014), as observed in this study. Interestingly, in addition to lung tissue, the parasite load in brain tissue was also reduced in GATA1−/− mice at 63 dpi, and again we observed an increased neutrophil activity. In the central nervous system, neutrophils are rarely found in the brain parenchyma due to a blood–brain barrier. However, under pathological conditions such as infections, trauma and haemorrhage, a more significant number of neutrophils enter the brain tissue. These cells can cause degranulation, phagocytosis and release the extracellular neutrophil trap, which is composed of histones, enzymes and granules that help eliminate pathogens (Joice et al., Reference Joice, Mydeen, Couraud, Weksler, Romero, Fraser and Easton2009; Liu et al., Reference Liu, Li and Dai2018). However, this process can lead to tissue damage, so neutrophils are often phagocytosed or inhibited by macrophages or lymphocytes after pathogen digestion to minimize tissue damage (Liu et al., Reference Liu, Li and Dai2018). Thus, we believe that neutrophils are important cells in combating T. canis larvae, but further studies are needed regarding the function and mechanism of action of cells, especially in the brain tissue, where the damage caused by these cells can lead to significant morbidity.
In conclusion, this work described the influence of eosinophils on acute and chronic toxocariasis (Fig. 8). The presence of these cells induces susceptibility to infection, alters the pulmonary immune response, cannot reduce the pulmonary and cerebral parasite load and decreases the neutrophilic activity in tissues, which seem to play an important role in the control of T. canis infection. We also observed that eosinophils are the primary triggers in the formation of pulmonary and hepatic granulomas that can lead to damage and loss of tissue function. Thus, we believe that future research investigating the escape mechanisms of T. canis larvae from the immune system is essential to expand therapeutic measures for the disease and dissecting the role of neutrophils in the context of T. canis infection may contribute to understanding the anti-parasite role of this granulocyte subtype.

Fig. 8. Influence of transcription factor GATA-1 on T. canis infection. The presence of eosinophils during T. canis infection contributes to the increase in the pulmonary and cerebral parasite load, to the formation of pulmonary and hepatic granulomas, increases the Th17 response and reduces the number of neutrophils.
Data
All data generated or analysed during this study are included in this published article. The datasets used and/or analysed during the present study are available from the corresponding author upon reasonable request.
Acknowledgements
We thank Michele Silva de Matos and Vanessa Gomes Fraga for their technical assistance and support during the experiments. Figures were prepared using biorender.com. This work was funded by the Coordenação de Aperfeiçoamento de Pessoal de Nível Superior (CAPES).
Author contributions
T. L.-S., C. A. L., F. V.-S., F. M. S. O., L. K., L. L. S. P., C. C. O. A., J. L. N. S., F. S. B. and M. A. R.: carried out sampling and laboratory analyses. T. L.-S., R. C. R., R. T. F. and L. L. B.: conceived the study. All authors contributed to data analysis and interpretation, and preparation of the manuscript. All authors read and approved the final manuscript.
Financial support
This investigation received partial support from Fundação de Amparo a Pesquisa do Estado de Minas Gerais/FAPEMIG, Brazil (grant no. CBB APQ-00766-18), the Brazilian National Research Council (CNPq) (grant nos. 421392/2018-5 and 302491/2017-1) and Pró-Reitoria de Pesquisa of Universidade Federal de Minas Gerais to cover research inputs. T. L.-S. is grateful for the Ph.D. fellowship provided by the Brazilian National Research Council (CNPq), Post-graduation Program in Infectology and Tropical Medicine/Universidade Federal de Minas Gerais. M. A. R., R. C. R., R. T. F. and L. L. B. are Research Fellows from the Brazilian National Research Council (CNPq). The funders had no role in study design, data collection, analysis, decision to publish, publication fees or manuscript preparation.
Conflict of interest
The authors declare there are no conflicts of interest.
Ethical standards
The maintenance and use of mice were carried out following the Brazilian College of Animal Experimentation (COBEA) recommendations, and all efforts were made to minimize animal suffering. The present study was submitted and approved by the Ethics Committee for Animal Experimentation (CEUA) of the Federal University of Minas Gerais, Brazil, through protocol no. 56/2018.