Functionally univentricular physiology occurs in the context of a wide range of congenital cardiac developmental disturbances of cardiac chamber looping, atrioventricular septation, atrioventricular valve delamination, as well great arterial development. These complex defects manifest most often in well-circumscribed lesions such as hypoplastic left heart syndrome (HLHS), tricuspid atresia, double inlet left ventricle, and pulmonary atresia. Clinical presentation may be with cyanosis, high pulmonary blood flow and heart failure, or critical obstruction to systemic or pulmonary artery outflow. Depending on the specific hemodynamic characteristics of the underlying congenital heart disease lesion, pulmonary vascular development may be profoundly affected. For example, pulmonary artery size, branching order, and concomitant pulmonary vascular biology may be significantly altered and to varying degree depending on the specific nature of the individual congenital heart disease. In this review, we plan to outline normal developmental biology of the pulmonary arteries, how this is altered in patients with functionally univentricular physiology, and how reparative surgery and transcatheter management of the individual lesions may affect outcomes. Principally, we focus on pulmonary vascular biology in patients who have received Fontan surgery (Fig 1). Reference Asrani, Asrani, Freese, Phillips, Warnes, Heimbach and Kamath1 Finally, we discuss potential therapeutic strategies and future directions.
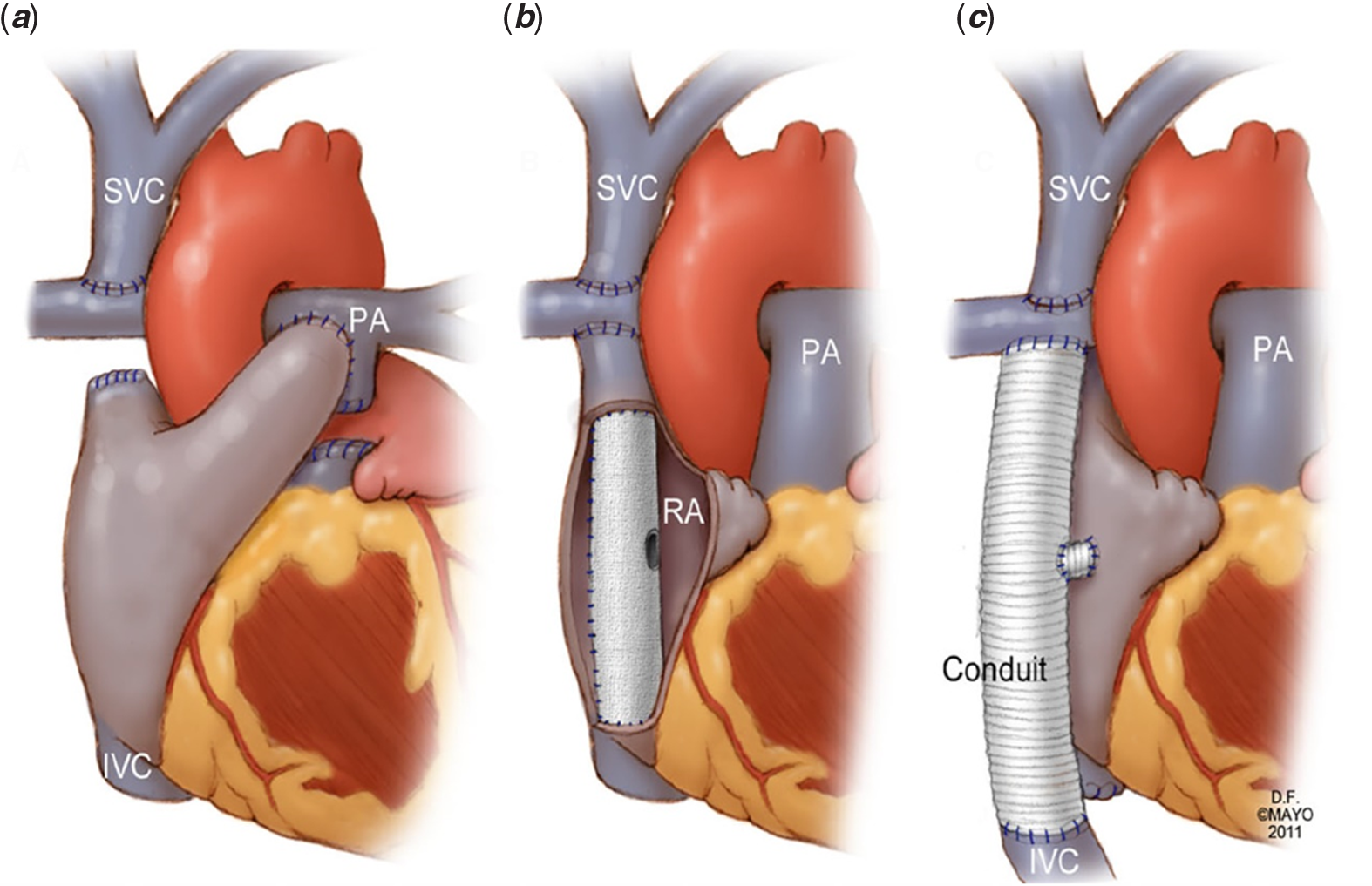
Figure 1. Variations in Fontan Anatomy. The Fontan operation in its three best-known forms: (a) Atriopulmonary Fontan; (b) Lateral Tunnel Fontan; (c) Extracardiac Fontan. Republished from Asrani SK et al, Hepatology 2012: 56(3); 1160–11,6911 with permission.
Developmental biology and structure of the normal pulmonary vascular bed
Pulmonary arteries originate within the early embryonic mesenchymal “soup”. Endothelial precursor cells together with neural crest-derived cells proliferate and weave smooth muscle cells into a primitive but stable vessel wall. Reference Deruiter, Groot, Rammos and Poelmann2 Ensuing cell sub-specialisation within and around the vessel wall is locally directed through signalling matrix proteins. These matrix proteins include among other molecules, platelet-derived growth factor-BB, insulin-like growth factor-1, and transforming growth factor-B. Reference Stenmark, Dempsey, Badesch, Frid, Mecham and Parks3 These molecules direct cell migration, adhesion, and cell proliferation and specialisation. Pulmonary arterial growth occurs in geographic and temporal proximity with airway development. In humans, the pre-acinar pulmonary arteries, i.e., the pulmonary arteries located prior to the respiratory region of the lung, are evident by 20 weeks of foetal life. Reference Hislop and Reid4 By this time, cardiac development or in the case of congenital heart disease, its mal-development, is already well established and the presence of its associated hemodynamic disturbance, such as found in functionally univentricular physiology, may profoundly impact the neuro-broncho-vascular bundle formation and patterning.
In humans, pulmonary arteries branch consecutively and progressively at approximately 90° angles up to 17 divisions when traced from the hilum peripherally to the pleural surfaces. Along this branching order, the pulmonary arteries become progressively less muscularised and also contain progressively less elastin. Reference Townsley5,Reference Davies, McBride, Murray, Wilcox, Shallal and Reid6 Indeed, at the pre-acinar and acinar levels, smooth muscle no longer occupies the vessel circumference, instead, and increasingly, the vessel wall is formed exclusively by thin squamous endothelial cells. Pulmonary capillaries do not consistently arise from the most distal branching order, but may arise from higher order pulmonary artery branches. Thus, there is considerable heterogeneity (Fig 2). Reference Horsfield7

Figure 2. Variable origin of pulmonary capillary networks. Pre-capillary arteries may branch at right angles from a small parent artery then give rise to a capillary network after some distance (a and b). Alternatively, small arteries may abruptly end in a capillary network (c and d). Capillary networks may also emerge directly from the parent artery (e). In human and rat lung, extra-alveolar pulmonary arteries 100 um or more in diameter may give rise directly to capillary networks. Republished from Horsfield E, Circ Res 1978:42(5);593–77 with permission.
Pulmonary veins follow a similar branching order to pulmonary arteries with around 15 branching generations. Reference Townsley5 They follow a mixed pattern of drainage, i.e., in part they are associated with the airways, but they are also partly divergent from the airways as the larger order vessels approximate the left atrium. Pulmonary veins differ from pulmonary arteries in that they are thinner walled, but paradoxically are more muscular than elastic, and contain more extracellular matrix in large part due to an expanded collagen content.
The adventitia of the pulmonary arteries is integrated with the surface of the adjacent airways, but only in the respiratory portion of the pulmonary arteries is there uncoupling from the airways due to the buffering effect of the lymphatic vessels. This means that the mechanical distension of the airways during normal ventilation has more effect on the distal pulmonary arteries and veins in particular. Reference von Hayek8 The anatomic basis for pulmonary artery compliance and maintenance of low resistance is more complex as will be discussed later. Suffice to say that at the anatomic level, the integration between density and patterning of branching order, the thickness of the muscular media, the preponderance of elastic fibres, and neural regulation provides baseline tonicity to pulmonary vascular compliance (Fig 3). Reference Reid9

Figure 3. Muscularity of the Pulmonary Vascular Tree. Schematic showing the graded changes in the media of pulmonary arterial wall, moving from muscular arteries to pre-capillary non-muscular vessels. The top panel illustrates the gradual loss of musculature. The middle panel shows the transition from vascular smooth muscle cells (M) to intermediate cells (I) and finally to pericytes (P). The bottom panel represents cross-sections, highlighting the partial circumferential coverage of the media. Republished from Reid LM, Chest 1986:89(2);279–889 with permission.
Pulmonary arterial development in the presence of congenital heart disease
The haemodynamics associated with congenital heart disease presents a variegated canvas in which prenatal and post-natal pulmonary artery development, morphology, and physiology are profoundly directed.
In lesions with high pulmonary artery blood flow and or pulmonary hypertension, contractile myofilament volume and density increases more rapidly than normal. Reference Haworth10 The brick-shaped smooth muscle cells within the pulmonary artery media do not dilate as normal during postnatal life. By the 3rd post-natal day, there is abnormally high myofilament concentration within the smooth muscle cells and an excessive amount of collagen deposition. These changes extend to the lung periphery where normally there is progressive medial thinning as described above. Together with these pro-constrictive changes, there is premature development of neural innervation. Reference Edvinsson, Gulbenkian, Wharton, Jansen and Polak11 These neural fibres characteristically contain dominating pro-constrictor molecules. The net impact of these changes are: i) regionally heterogeneous anatomic and physiologic variations, i.e., different lung segments may be differentially affected, ii) that there is a pro-constriction milieu and iii) that a disproportionate amount of resistance occurs for the total histopathologic cell and matrix burden observed due to the altered local neuro-biochemical environment.
In functionally univentricular physiology lesions with low pulmonary blood flow, such as pulmonary atresia or severe pulmonary stenosis and where an arterial shunt was required, the pulmonary artery growth potential is reduced and branching order may be significantly reduced from normal. The vessel wall itself is significantly altered in more than 70% of cases. There is elastic fragmentation, increased ground substance, intimal thickening, and fibrosis in one-third of patients (Fig 4). Reference Chowdhury, Bishnoi and Ray12 This is particularly the case of patients palliated with an arterial shunt.

Figure 4. Photomicrograph of pulmonary artery biopsy of a patient with a functionally univentricular heart. This image demonstrates microscopic changes in the pulmonary vascular bed as a result of Fontan circulation. There is widespread elastic fibre fragmentation and lamellar loss with areas of ground substance accumulation, loss of smooth muscle, and patchy areas of fibrosis (elastic van Gieson stain; original magnification × 200). Republished from Chowdhury et al Ann Thorac Surg 2009;589–9612 with permission.
Structural intervention on the pulmonary arteries
Surgical or transcatheter pulmonary artery enlargement is frequently needed in the modified natural history of functionally univentricular physiology patients. It may be necessary at the time of bidirectional Glenn or at Fontan surgery in up to half of cases and very frequently during heart transplantation. Central pulmonary artery hypoplasia and left pulmonary artery stenosis and hypoplasia are particularly common in hypoplastic left heart syndrome. The stenosis may be due to technical surgical factors such as the pulmonary artery confluence being placed to the left or right of the midline. Frequently, mid left pulmonary artery stenosis is caused by compression the ascending neo-aorta anteriorly and the left bronchus and descending aorta posteriorly. Diminished flow down the left pulmonary artery may precipitate hypoplasia of the left pulmonary artery more globally. Though intervention on the pulmonary arteries, whether surgical or transcatheter, does not appear to affect late survival, little data exists on the long-term effects of pulmonary artery rehabilitation on morbidity and quality of life. MRI-based studies have demonstrated in vivo that zones of narrowing within the non-pulsatile pulmonary artery circuit precipitate profound energy losses proportional to the degree of narrowing. These energy losses are significantly amplified during exercise as cardiac output rises. More recently, such stenoses and flow aberrations have also been demonstrated to be strongly associated with end-organ disease such as liver fibrosis Reference Trusty, Wei and Rychik13 and also with objectively measured functional capacity (e.g., peak oxygen consumption). Reference Nathan, Loukas and Moko14
The Fontan circulation and the pulmonary vascular bed
The Fontan operation is an effective palliation of complex congenital heart disease, and it involves a re-routing of systemic venous return directly into the pulmonary vascular bed. As a result, pulmonary flow supply is dependent on non-pulsatile flow through the lungs to achieve ventricular filling. Reference Gewillig15 In the absence of an effective subpulmonary ventricle, the systemic venous pressure (i.e., central venous pressure) becomes the driving force required to overcome pulmonary vascular impedance necessary to maintain systemic ventricular preload. The pulmonary vascular impedance is dependent on pulmonary artery compliance, pulmonary capillary capacitance (pulmonary vascular resistance), and pulmonary wedge pressure, which is a surrogate for ventricular filling pressure. Reference Gewillig and Brown16,Reference Egbe, Connolly and Miranda17
The optimal candidate for Fontan palliation is one with well-developed pulmonary arteries, low pulmonary vascular resistance, and low pulmonary artery wedge pressures. Reference Gewillig and Brown16,Reference Choussat, Fontan, Besse, Shinebourne and Anderson18–Reference Ridderbos, Bonenkamp and Meyer21 However, even patients with low pulmonary vascular resistance at the time of Fontan palliation tend to develop increased pulmonary vascular resistance over time. This is attributed to different pathophysiologic processes. These physiologic changes bring about significant changes to the biology of the pulmonary vasculature over time. Reference Mitchell, Campbell and Ivy22,Reference Khambadkone, Li, de Leval, Cullen, Deanfield and Redington23 Prior animal studies have shown that pulsatile flow, particularly in setting of hypoxemia, is necessary in order for the pulmonary vascular endothelium to release vasodilatory cytokines. Reference Hakim24 Furthermore, non-pulsatile flow has been shown to be maladaptive in a number of ways. Endothelial dysfunction is common in patients post Fontan, and this has recently been shown to correlate with pulmonary vascular disease. Reference Egbe, Miranda, Anderson and Borlaug25 Patients with Fontan circulation have elevated levels of von Willebrand factor, which suggests endothelial injury and dysfunction as this factor is only found in vascular endothelium. Reference Binotto, Maeda and Lopes26 Ex vivo pulmonary vascular models and in vivo animal models have shown that non-pulsatile flow decreases production of endothelial nitric oxide synthase. Reference Zongtao, Huishan and Zengwei27,Reference Nakano, Tominaga, Nagano, Okabe and Yasui28 Recent catheterisation studies showed abnormal pulmonary flow reserve to acetylcholine stimulation, consistent with endothelial dysfunction. Reference Latus, Lederle, Khalil, Kerst, Schranz and Apitz29 Post-mortem studies in patients with Fontan circulation have shown significantly altered histology, with a thin media and a thickened intima. Reference Ridderbos, Wolff and Timmer30 This further suggests abnormal endothelial function in the setting of abnormal endothelial structure, all in the setting of abnormal gross anatomy and physiology.
Immediately after Fontan, the significant changes in anatomy bring about significant changes in flow, thereby altering the physiology of both pulmonary and systemic circuits. Reference Gewillig15 The non-pulsatile pulmonary vascular bed now is a bottleneck in the overall circulation, thereby decreasing preload to the systemic circuit. Reference Gewillig and Brown16,Reference Gewillig, Cools and Van De Bruaene31 Post-operatively prior to extubation, even with normal pulmonary pressures, some studies have shown benefits from administration of pulmonary vasodilators. Reference Cai, Su and Shi32,Reference Gamillscheg, Zobel and Urlesberger33 In the early years post-Fontan, exercise capacity has been shown to be decreased, suggesting the functional limitations of the Fontan circulation are not just due to chronic complications but also in part due to immediate and inherent changes in physiology. Reference Wolff, van Melle and Bartelds34 Long-term, these physiologic changes actually determine the anatomy in that pulmonary artery size decreases late post-Fontan surgery. This is relevant as smaller pulmonary artery size and maldistribution of blood flow are significantly associated with poorer exercise capacity and oxygen saturation. Reference Ridderbos, Bonenkamp and Meyer21,Reference Alsaied, Sleeper and Masci35
Resting and exercise haemodynamics of the pulmonary vasculature in the Fontan circulation
This combination of abnormal anatomy, physiology, and biology creates a tenuous milieu in the pulmonary vascular bed, which is unable to respond to stressors (Fig 5). In contrast to pulsatile physiology, the low cardiac output state can mask significant pulmonary vascular disease at rest. Reference Egbe, Miranda, Anderson and Borlaug25 This was previously suggested in patients with Fontan circulation who subsequently underwent heart transplantation (without concurrent lung transplantation). Reference Mitchell, Campbell and Ivy22 In that cohort, the only patients who did not have a post-transplant increase in pulmonary vascular resistance were those who had failure early post-Fontan. Reference Mitchell, Campbell and Ivy22 Thus, with longer duration of Fontan physiology, more pulmonary vascular disease ensues. Accordingly, it has been shown that responsiveness to pulmonary vasodilators increases with increased time since surgery. Reference Khambadkone, Li, de Leval, Cullen, Deanfield and Redington23 Even subtle, transient declines in pulmonary vascular function in response to stress can cause marked changes to cardiac output, central venous pressure, and venous congestion. Reference Egbe, Connolly and Miranda17 More recently, it was shown that resting assessment for pulmonary vascular disease is insensitive, and invasive exercise haemodynamics can significantly increase the likelihood of identifying early or occult pulmonary vascular disease (Fig 6). Reference Egbe, Miranda, Anderson and Borlaug25 In summary, flow of the Fontan circulation is dependent on pulmonary vascular reserve. Reference Gewillig, Brown and Eyskens20

Figure 5. Relationship between cardiac output and pulmonary vascular resistance. This figure highlights that except at very poor ventricular function, the major determinant of cardiac output is the pulmonary vascular bed. Republished from Gewilig M and Brown SC, Heart 2016;102:1081–108,616 with permission. PVR = pulmonary vascular resistance.

Figure 6. Relationship of Pulmonary Vascular Reserve and End-Organ Function. When pulmonary vascular reserve, as defined by ΔmPAP/ΔCO, is worse then there are stronger associations with endothelial dysfunction and end-organ dysfunction (liver stiffness, renal dysfunction, volume overload, and exercise intolerance). Republished from Egbe AC et al J Am Coll Cardiol 2020;76(23):2755–6325 with permission. CO = cardiac output; mPAP = mean pulmonary arterial pressure.
While inability to augment Fontan flow has long been implicated as a determinant of exercise capacity, prior investigations of the limitations of exertion have focused on stroke volume limitation and chronotropic incompetence as they are easier to measure. Chronotropic incompetence is certainly prevalent amongst adults with Fontan circulation, and may be more likely depending on underlying anatomy and post-operative alteration in autonomic function. Reference Powell and Veldtman36 However, in patients with relatively normal exercise capacity, chronotropic incompetence is still common, implying that inability to augment heart rate is not the only limitation to exercise. Reference Powell, Chin and Alsaied37 Alternatively, inability to augment stroke volume seems to be a major cardiac factor limiting exercise. Reference Powell, Chin and Alsaied37,Reference Claessen, La Gerche and Van De Bruaene38 This inability to augment stroke volume is due not only to diastolic filing impairments of the systemic atrium and ventricle and impaired venous return, but also likely in large part due to decreased pulmonary vascular reserve. Reference Egbe, Miranda, Anderson and Borlaug25,Reference Gewillig, Cools and Van De Bruaene31,Reference Powell, Chin and Alsaied37,Reference Senzaki, Masutani and Ishido39,Reference Opotowsky, Halpern, Kulik, Systrom and Wu40 The physiology behind diastolic dysfunction in the Fontan circulation is complex and research is ongoing to better understand all the contributing factors. Reference Budts, Ravekes, Danford and Kutty41 In many ways, it may mirror what is seen in the chronically underfilled left ventricle of mitral stenosis, which in turn becomes fibrotic with impaired diastolic properties. Reference Liu, Ting and Yang42 Thus, with faster heart rates, there is less filling, and therefore the chronotropic incompetence observed in Fontan patients paradoxically may help augment ventricular preload during exertion.
Determinants of pulmonary artery pressure
Diastolic filling pressures of the systemic atrium and ventricle are relevant as this is the exit pressure for the pulmonary vascular bed, and has been identified as a contributor to Fontan failure. Reference Gewillig15,Reference Choussat, Fontan, Besse, Shinebourne and Anderson18,Reference King, Ayer and Celermajer43 In light of the multiple contributors to abnormal pulmonary vascular function as above, one must keep in mind that pulmonary artery pressures may be elevated due to both pre- and post-capillary mechanisms. Of note, for calculation of pulmonary vascular resistance, pulmonary artery wedge pressure rather than ventricular end-diastolic pressure has been shown to more accurately assess pulmonary vascular function, and this correlates more strongly with outcomes. Reference Miranda, Hagler, Taggart, Borlaug, Connolly and Egbe44 Traditionally, post-capillary mechanisms have not been considered to contribute to pulmonary vascular resistance. However, post-mortem studies in adults with acquired heart disease have shown vascular remodelling in the post-capillary vascular bed (i.e., pulmonary veins). Reference Fayyaz, Edwards and Maleszewski45 In addition, clinical studies in adults with acquired heart disease have shown that the post-capillary pressures are actually the major determinant for the pulmonary artery pressure, even if there is a distinct pre-capillary component. Reference Tedford, Hassoun and Mathai46 Thus, it is possible that with longer duration of Fontan circulation, impaired pulmonary vascular reserve is due to worsened pulmonary vascular endothelial function from non-pulsatile flow (pre-capillary), worsened pulmonary venous compliance due to remodelling (functionally pre-capillary) from progressive diastolic dysfunction of the systemic atrium and ventricle (post-capillary).
In addition to the cardiac limitations to exercise – impaired venous return, stroke volume limitation, diastolic filling impairments, chronotropic incompetence – there are also non-cardiac contributions. The sensation of dyspnoea itself is accentuated in patients with Fontan circulation. Reference Guenette, Ramsook and Dhillon47 Spirometry and ventilator efficiency have been shown to be abnormal in adults post Fontan, and these impairments correlate with functional capacity. Reference Guenette, Ramsook and Dhillon47–Reference Laohachai, Winlaw and Selvadurai49 In addition, central regulation of breathing is likely altered as suggested by the presence of oscillatory ventilation with exercise. Reference Nathan, Loukas and Moko14 Obstructive sleep apnoea is common in these patients and may impact pulmonary vascular function. Reference Boutsikou, Li and Gatzoulis50 While there are no studies evaluating the direct effect of these pulmonary structural abnormalities to pulmonary vascular function, studies in adults with acquired disease suggest that for the vast majority of patients, pulmonary disease does not impact pulmonary vascular haemodynamics as much as filling pressures and lymphatic drainage. Reference Tedford, Hassoun and Mathai46,Reference Reddy, Obokata and Wiley51 However, having said that, reduced lung volumes do appear to affect cardiopulmonary reserve adversely in Fontans. The mechanisms involved however are not clearly defined as yet. Reference Opotowsky, Landzberg and Earing48 Pulmonary artery size seems to correlate with ventilatory function, which suggests the pulmonary vasculature significantly impacts the pulmonary parenchyma.
The liver may also have significant impact on the haemodynamics of the Fontan circulation. It is well-established that patients with liver disease have higher cardiac outputs, driven by lower peripheral arterial resistance. Reference Gordon-Walker, Bove and Veldtman52,Reference Reddy, Melenovsky, Redfield, Nishimura and Borlaug53 While classically a failing Fontan has high central venous pressures and low outputs, those with high pressures and normal outputs may actually have liver disease, which accounts for the output being normal instead of low. Reference Mori, Hebson and Shioda54 This degree of liver dysfunction seems to correspond with a significantly reduced PVR reserve and this may create a spiral of progressive worsening haemodynamics and progressive liver injury. Reference Schmitt, Steendijk and Ovroutski55,Reference Gewillig15,Reference Ridderbos, Hoendermis, Berger and van Melle56, Reference Egbe, Miranda, Anderson and Borlaug25,Reference Mori, Hebson and Shioda54,Reference Ridderbos, Hoendermis, Berger and van Melle56–Reference Hebson, McCabe and Elder59
Pulmonary vascular reserve and outcomes in the Fontan circulation
As discussed above, the critical bottleneck in the Fontan circulation is the pulmonary vascular bed. Reference Gewillig, Cools and Van De Bruaene31 Systemic venous return is non-pulsatile and therefore entirely subject to pulmonary vascular reserve. This inability to augment flow explains functional limitations immediately post-Fontan, and the chronic stresses of the circulation – non-pulsatile flow, endothelial dysfunction, progressive diastolic dysfunction, hypoxemia, impaired lymphatic drainage, higher volume from liver disease – further contribute to impaired pulmonary vascular reserve and functional limitations long-term. Even though this focus on pulmonary vascular reserve is more recent, it should come as no surprise as prior evaluations of the hemodynamic markers of Fontan failure have focused on the downstream effects of impaired pulmonary vascular reserve: elevated central venous pressure, alterations in cardiac output, liver disease, and protein-losing enteropathy. Reference Hebson, McCabe and Elder59–Reference Pundi, Johnson and Dearani61
Elevated central venous pressure correlates with even subtle abnormalities in pulmonary vascular function at rest as well as with pulmonary vascular reserve during exercise (Fig 7). Reference Egbe, Miranda, Anderson and Borlaug25,Reference Egbe, Reddy and Khan62,Reference Yell, Boyer, Bernardi, Klewer and Seckeler63 While studies focusing on outcomes related to pulmonary vascular reserve directly are limited, both elevated central venous pressure and alterations in cardiac output have previously been shown to correlate to poor outcomes. Reference Egbe, Connolly and Miranda17,Reference Egbe, Miranda, Anderson and Borlaug25,Reference Miranda, Borlaug, Hagler, Connolly and Egbe57,Reference Egbe, Reddy and Khan62,Reference Egbe, Connolly, Taggart, Al-Otaibi and Borlaug64 Exercise capacity is established as a predictor of outcomes, and there are multiple studies showing improved functional capacity with pulmonary vasomodulators. Reference Udholm, Aldweib, Hjortdal and Veldtman65–Reference Agnoletti, Gala and Ferroni68 This suggests that pulmonary vascular reserve is indeed the critical bottleneck of the Fontan circulation and potentially the major determinant of long-term outcomes as it has been shown to correlate with flow both upstream (central venous pressure, liver fibrosis, renal function) and downstream (VO2). Reference Egbe, Miranda, Anderson and Borlaug25,Reference Gewillig, Cools and Van De Bruaene31 Further studies are needed directly evaluating pulmonary vascular reserve, measured as the ratio of change in pulmonary artery pressure to the change in cardiac output with exercise, with functional capacity and long-term outcomes. Reference Egbe, Miranda, Anderson and Borlaug25 In particular, as exercise testing allows for earlier identification of occult pulmonary vascular disease, it will be of significant interest whether or not early use of pulmonary vasomodulators could improve long-term outcomes.

Figure 7. Correlations of central venous pressure with pulmonary vascular resistance and pulmonary artery wedge pressure. This figure shows that central venous pressure is much more strongly correlated with pulmonary vascular function, whereas the ventricular filling pressures are less strongly correlated and therefore not the only determinant of central venous pressure. Republished from Egbe AC et al Int J Cardiol 2018;271:312–31,662 with permission. CVP = central venous pressure; PAWP = pulmonary arterial wedge pressure; PVRI = pulmonary vascular resistance index.
The effects of pulmonary vasodilator therapy on pulmonary vascular biology
Khambadkone et al as early as 2003 demonstrated significant acute pulmonary vasoreactivity in response to inhaled nitric oxide late after the Fontan operation. Reference Khambadkone, Li, de Leval, Cullen, Deanfield and Redington23 Since then, a number of studies, including randomised placebo controlled studies with and without cross-over, have been performed. Reference Goldberg, Zak and Goldstein69–Reference Hebert, Mikkelsen and Thilen75 The most frequent primary endpoint was peak VO2. Secondary endpoints included submaximal exercise performance at anaerobic threshold, BNP, NYHA, quality of life, mortality, and Fontan failure. The trials have yielded mixed results, in part due to inadequate subject recruitment and or poor patient adherence. As the physiology of Fontan failure is not limited to the pulmonary vascular bed, and may in fact be a complex mixture of various hemodynamic perturbations such as diastolic dysfunction, pathway obstruction, atrioventricular valve regurgitation, abnormalities of venous compliance, and intravascular volume, it is hardly surprising that trials that do not differentiate specific hemodynamic phenotypes are to a large extent doomed to yield mixed and confusing results. It is entirely possible that accurate characterisation of the hemodynamic phenotype might assist in future treatment strategies.
Bosentan was assessed in three medium-sized trials of 71, 42, and 39 patients, respectively, Reference Hebert, Mikkelsen and Thilen75–Reference Schuuring, Vis and van Dijk77 powered for peak VO2 in two of the three studies. Of the two adequately powered studies, one study Reference Hebert, Mikkelsen and Thilen75 demonstrated a significant increase in mean VO2 from 28.7 to 30.7 ml/kg/min as well as a reduction in BNP and increase in O2 pulse as a surrogate for stroke volume. In the second study of 42 patients, 10 had dropped out, and the primary endpoint of improved peak VO2 was not reached. Reference Schuuring, Vis and van Dijk77 The third bosentan study was smaller with only 16 assigned to bosentan and endpoints included mortality and Fontan failure. Reference Shang, Li and Liu76 Given the relatively small sample size, it is difficult to generalise these findings to larger populations.
Phosphodiesterase inhibitors were assessed in seven trials including sildenafil in five studies Reference Mendoza, Albert and Belda72–Reference Giardini, Balducci, Specchia, Gargiulo, Bonvicini and Picchio74,Reference Tunks, Barker and Benjamin78,Reference Ciliberti and Giardini79 and udenafil in two. Reference Goldberg, Zak and Goldstein69,Reference Goldberg, Zak and Goldstein80 Of the trials, five were acute or very short-term studies, which generally demonstrated acute hemodynamic improvement in mean pulmonary artery pressures and a reduction in transpulmonary gradient. The longer term studies had active drug for 6 weeks and 26 weeks. The primary endpoint of peak VO2 did not change significantly for either of the two studies. In the udenafil trials, exercise parameters at anaerobic threshold improved including a lower VO2 at anaerobic threshold, and a small decrease in VE/VCO2 slope at anaerobic threshold. Though neither trial examined hemodynamic effects of the phosphodiesterase inhibitor, udenafil also did not change vascular function significantly.
One single centre-randomised trial of ambrisentan in Fontans had a significant drop out rate and only 16 of the intended of 21 to detect a significant change in peak VO2. Reference Cedars, Saef and Peterson71
Table 1 summarises the key trials assessing the effects of pulmonary vasodilators.
Table 1. Pulmonary vasodilator trials

Abbreviations: 6MWD = 6 minute walk distance; BNP = brain natriuretic peptide; mPAP = mean pulmonary artery pressures; NYHA = New York Heart Association; VO2 = Oxygen consumption.
Exercise rehabilitation effects on pulmonary vascular haemodynamics
During exercise in normal two ventricular circulations, there is greater pulsatile blood flow in the pulmonary arteries, which triggers a release of vasoactive mediators acting largely through nitric oxide mediated pathways. As discussed, in Fontan circulations, these responses are constrained by the absence of pulsatile flow, relatively poor conduit compliance, less muscularization of the conductance pulmonary arteries, and a significant limitation in diastolic reserve of the single ventricle. Reference Ridderbos, Wolff and Timmer30 These hemodynamic changes are likely to actively contribute to a restricted pulmonary vascular reserve and are expected to contribute greatly to the observed reduction in cardiopulmonary capacity in Fontan patients. Rehabilitation programmes focusing on both aerobic and strength building exercise have had encouraging results. Cordina et al. have demonstrated that it is possible to produce some pulsatility in the central pulmonary arteries during exercise, and significantly better local muscle blood flow suggesting greater cardiac output and better pulmonary blood flow following exercise training programmes. Reference Cordina, Celermajer and d’Udekem81 Fontan patients engaged in regular physical exercise and sport activities not only have better quality of life but also have better peak VO2, and presumably better pulmonary blood flow and cardiac output.
Mechanical circulatory support and the pulmonary vascular bed
When systolic dysfunction predominates, mechanical circulatory support may be of benefit as a bridge to transplantation. If mechanical circulatory support successfully reduces diastolic pressures and increases cardiac output, whilst pulmonary artery pressure remain similar at higher flow rates, the implication is that there is low pulmonary vascular resistance. In patients with elevated pulmonary vascular resistance, or with Fontan pathway obstruction, the benefits of mechanical circulatory support may not be immediately evident, and indeed congestion may not be relieved or may even be worsened immediately after surgical implantation of the device. Further support with pulmonary vasodilators, relief of the obstruction, or even a right heart assist may be necessary until the pulmonary vascular bed adequately remodels.
The effects of orthotopic cardiac transplantation on the pulmonary vascular bed
At the time of transplant assessment, pulmonary vascular disease may be underestimated, and its reversibility may not be possible to demonstrate through conventional cardiac catheterisation techniques. This is because the absolute amount of pulmonary blood flow cannot always be accurately assessed through the Fick method when there are multiple sources of pulmonary blood flow such as aorto-pulmonary collaterals, and the presence of regionalised veno-venous collaterals may further complicate assessment. Reference Downing, Dori, Harris and Glatz82 Concomitant cardiac magnetic resonance image flow studies at the time of cardiac catheterisation may improve the accuracy of such hemodynamic assessments. Pulmonary vascular resistance may also be markedly and adversely affected early after heart transplantation in the intensive care setting by a variety of pulmonary causes, vasopressor effects, fluid overload, and the presence of early restrictive left ventricular physiology of the transplanted heart. Mitchell et al. demonstrated a high prevalence of elevated pulmonary vascular resistance after heart transplantation. Reference Mitchell, Campbell and Ivy22 Assessment and management of the pulmonary vascular bed in terms of anatomy, biology, the impact of diastolic dysfunction of the pre-transplanted, as well as the post-heart transplanted heart, are all essential in attaining good outcomes.
Conclusions and future directions
The biologic and morphologic competence of the pulmonary vascular bed is essential for optimal functioning of the Fontan circulation. However, the maturation, branching, and vasodilatory maturation of the pulmonary arteries are frequently abnormal as a direct consequence of the underlying functionally univentricular haemodynamics. Further, surgical techniques may be associated with proximal as well as mid-level branch pulmonary artery stenosis, particularly in the context of HLHS and the Norwood procedure. There is currently very little data available outlining the influence of morphologic and histologic abnormalities on the biology of the pulmonary arteries. Regardless, it is clear that pulmonary vascular reserve is reduced in Fontan patients. This latter phenomenon is directly associated with abnormal endothelial biology and poor end-organ and patient outcomes. Pharmacologic modulation and structural intervention to the pulmonary vascular bed both have important roles to play in individual patients, though do not provide a panacea for the wider problem of limited pulmonary vascular reserve.
Financial support
This research received no specific grant from any funding agency, commercial or not-for-profit sectors.
Conflicts of interest
None of the authors declare any relevant conflicts of interest.