Introduction
As an ancient and widespread practiced cropping system, the advantages of intercropping were realized in both low-input agroforestry and high-input agriculture systems. Higher yields in intercropping systems result from resource complementarity between the intercropped crop species in the temporal and spatial patterns of resource acquisition via niche separation, facilitative interactions between components, and/or reduction in the negative impacts of pests, diseases and weeds (Zhang et al., Reference Zhang, Mallik and Zeng2013; Bottrell and Schoenly, Reference Bottrell and Schoenly2018). However, a delicate balance between complementarity v. competition shifts constantly, depending on the intercropped species/verities choice, the suitable crop combinations, the stage of plant development, spatial configuration and the growing conditions (Letourneau et al., Reference Letourneau, Armbrecht, Rivera, Lerma, Carmona, Daza, Escobar, Galindo, Gutiérrez, López, Mejía, Rangel, Rangel, Rivera, Saavedra, Torres and Trujillo2011).
On the other hand, as changing exudation processes in the rhizosphere, intercropping influences the plant–microbe symbiotic relationships, thereby enabling alternative beneficial (e.g. N-fixing bacteria and mycorrhizal fungi) and/or harmful (e.g. soil-borne parasites and diseases) interactions (Yu, Reference Yu1999; Philippot et al., Reference Philippot, Raaijmakers, Lemanceau and van der Putten2013; Zhang et al., Reference Zhang, Mallik and Zeng2013). Resilient in the soil, root-knot nematodes (RKNs, Meloidogyne spp.) persist in a population reservoir, causing future outbreaks of vegetables (e.g. tomato and cucumber) and fruit trees injuries and cause large losses of crop production and grower's income. Management of RKNs is a great challenge due to their adaptation to a wide range of temperatures that allow them to have a ubiquitous distribution. Although chemical nematicides are the most effective means of controlling RKNs, they are suggested to be the last resort when other actions cannot prevent them, because of their adverse environmental effects. In contrast, from an evolutionary perspective, the sustainable method is to manage pests below the threshold levels, i.e. at low population numbers below biological and/or economically injurious levels, rather than to eradicate them (Green et al., Reference Green, Stenberg and Lankinen2020). Available plant resources, such as plants resistant against RKNs intercropping with host crops, are feasible and low-cost means to create the rhizosphere unfavourable for the development and reproduction of RKNs (Maina et al., Reference Maina, Karuri, Rotich and Nyabuga2020). For instance, it was found that tomato/castor intercropping can serve as an effective way of controlling M. incognita in temperate tomato: the populations in soils decreased by 21.6% and by 37.9% in the field and pot experiment, respectively, compared with those of monoculture at 35 days after inoculation; owing to the fatty acids involved in root extrudes (Dong et al., Reference Dong, Li, Huang, Lu, Li, Yao, Liu and Zuo2018). In the tropics and subtropics, fruit trees, e.g. macadamia, cocoa, coffee and guava, are severely attacked by at least one Meloidogyne species (e.g. M. incognita, M. arenaria or M. javanica) (Daneel, Reference Daneel, Fourie, Spaull, Jones, Daneel and Waele2017). The control becomes more difficult as the short-generation times of RKNs in warm climates lead to rapid population build-up all-year round (Perry et al., Reference Perry, Moens and Starr2009).
Sacha Inchi (Plukenetia volubilis L.), a promising evergreen recurrent woody oilseed crop, is increasingly grown in tropical China and southeast Asia in recent years. Nowadays, Sacha Inchi plantation was dominated by large-scale monoculture farming system in tropical dry-land, presumably negatively impacting on local biodiversity and soil health. But until now, no studies of intercropping of Sacha Inchi exist (Kodahl, Reference Kodahl2020). Most pressingly, Sacha Inchi plants are highly sensitive to RKNs, which greatly stagnate plant growth and even lead to massive crop failure (i.e. plant death) in the commercial plantations in south American and eastern Asia. The RKNs species infesting Sacha Inchi plant was identified in eastern Asia, i.e. M. javanica, based on the morphology, esterase isozyme phenotypes and molecular characterization (Wang et al., Reference Wang, Xie, Cui and Dong2014). Thus, it is urgent to develop agricultural management strategies to reduce the population density of M. javanica in the rhizosphere of Sacha Inchi plants to the lower level of burst threshold. Among them, if properly designed, a Sacha Inchi-based intercropping system is one of the most efficient practices to reduce the incidence and severity of RNKs (Maina et al., Reference Maina, Karuri, Rotich and Nyabuga2020). However, relay intercropping systems, selection of the suitable companion crops is still critical (Letourneau et al., Reference Letourneau, Armbrecht, Rivera, Lerma, Carmona, Daza, Escobar, Galindo, Gutiérrez, López, Mejía, Rangel, Rangel, Rivera, Saavedra, Torres and Trujillo2011).
Due to numerous biologically active ingredients including allicin, polysulphides and organosulfur, Allium species (garlic (A. sativum L.), onion (A. cepa L.), Chinese leek (A. tuberosum Rottler ex Sprengel), etc.) have successfully been incorporated into the agricultural systems owing to their function as strong nematicides, antimicrobes and repellents against a range of pests and diseases (Yu, Reference Yu1999; Zhang et al., Reference Zhang, Mallik and Zeng2013; Ji et al., Reference Ji, Li, Meng, Dong, Zhang and Qiao2019). For instance, it was found that garlic root exudates had a significant inhibitory effect on Phytophthora blight (Phytophthora capsici) of pepper plants (Khan et al., Reference Khan, Cheng, Xiao, Khan and Ahmed2011). Interestingly, allicin derived from garlic extracts can simultaneously efficiently control the populations of M. incognita J2s and fungal pathogens (grey mould caused by Botrytis cinerea) in tomato under laboratory and greenhouse conditions, besides it served as plant growth stimulators (Ji et al., Reference Ji, Li, Meng, Dong, Zhang and Qiao2019). Moreover, the main mechanism suppressing RKNs may not be solely direct caused by inhibitory effect from biologically active ingredients, but be indirectly, i.e. derived from plant-mediated anti-nematode microbial consortia (Adam et al., Reference Adam, Westphal, Hallmann and Heuer2014; Topalovic et al., Reference Topalovic, Hussain and Heuer2020). Revealing the shifts of microbial consortia in soils may be helpful to understand the potential microbiota associated with RKNs suppressiveness. Currently, most research focused on the short-season crops and the persistent integration of the above- (i.e. plant traits) and below-ground (e.g. RNKs and soil-borne pathogens) of the target crop into the sustainable agricultural systems are lacking, especially for tropical woody crops. In view of the closely-related work in some vegetables, Allium species are expected to be an ideal companion crop for relay intercropping with Sacha Inchi plants, mainly resulting from the reduction of the severity of RKNs.
Therefore, using a Sacha Inchi/Chinese leek intercropped system that has been set up in the field, the objective of this study was to investigate how a relative long-term (3–4 years) intercropping system would affect plant traits and soil environment (soil nutrients and microorganisms), and thus had in general positive effects in Sacha Inchi plantation. We expected that compared with the Sacha Inchi monoculture, (1) Sacha Inchi/Chinese leek intercropped system influenced soil nutrient contents and shifted soil microbial community (including potential soil-borne pathogens), leading to the improved crop productivity and soil quality in Sacha Inchi plantations; (2) the constrained M. javanica populations in the intercropping system can improve plant growth, survival and/or seed yield of Sacha Inchi plants, which may be due to the direct inhibitory effect from biologically active ingredients of Chinese leek plants and/or the indirect effect from indigenous microbe induced-suppressiveness.
Materials and methods
Study site and experimental design
The study was carried out in Xishuangbanna Tropical Botanical Garden (21°56′N, 101°15′E, 560 m a.s.l), Chinese Academy of Sciences, southwest China. The climate at Xishuangbanna is dominated by the southwest monsoon with three distinct seasons (a wet season from May to October, a cool dry season from November to April and a hot dry season from February to April). The rain-fed field experiments were arranged with 2 m × 44 m sized plots, in a 1.5-year-old P. volubili plantation (seeding plants; ca. total 0.6 ha) at intra- and inter-row spacing of 2.0 and 2.0 m in 2014. The characteristics of the top layer of bulk soil (0–20 cm) were measured in June 2014: pH 5.42, organic carbon 5.65% (w/v), total nitrogen 0.34 g/kg, available N 46 mg/kg, available P 14.1 mg/kg and available K 22 mg/kg. Compound fertilizer (150 kg/ha) was spread in early June each year and weed was done monthly, based on our previous work (Yang et al., Reference Yang, Jiao, Geng, Cai and Cai2014; Cai et al., Reference Cai, Xie and Xu2021). All P. volubilis plants were supported to a height of 1.6 m using steel wire. To avoid edge effects, only the central plants (at least 4 m2 away from the edge) in the plantation were selected.
In June 2014, seedlings with both green sprouts aboveground (12 cm in plant height) and elongated bulbs underground (ca. 0.6 cm in diameter) of Chinese leek were purchased from the nearby farmers and transplanted directly into nearly half of land area in the Sacha Inchi plantation. A completely randomized design was used consisting of eight replications (plots) within each treatment (Sacha Inchi monoculture and Sacha Inchi/Chinese leek strip-intercropping). In the intercropping plots, two rows of Chinese leek plants were planted at each side of a plot, respectively, at inter-row spacing of 10.0 cm; 0.75 m away from the P. volubilis trunk. The aboveground tissues of Chinese leek were not harvested, except for the last year of the experiment.
Measurements of plant traits
The phenology (i.e. flowering and fruiting of Sacha Inchi plants; yellowing and withering of leaves and aboveground parts of Chinese leek) and growth was monitored monthly or trimonthly in 2016 and 2017. The numbers of the dead individual Sacha Inchi plants were recorded in each cropping group each year. Mature capsule fruits (one seed per pod) of Sacha Inchi plants were harvested manually and repeatedly five times in each survived individual plant throughout the fruit ripening period; fruit dry mass per plant was measured at each harvest. At each harvest, subsamples of mature fruits were peeled and seed dry mass (size) was weighted. Seed yield in each harvested date and the total seed yield (per plant) throughout a growing season were then calculated as we described previously (Yang et al., Reference Yang, Jiao, Geng, Cai and Cai2014; Geng et al., Reference Geng, Chen, Yang, Jiao, Zhang and Cai2017). Stem diameter of Sacha Inchi plants at 5 cm above soil level in each group and the average plant height of Chinese leek plants in the intercropping system were measured with a calliper to assess plant growth; the dead Sacha Inchi plants were excluded. Fine roots (diameter <1 mm) of Sacha Inchi plants were harvested for the measurements of specific fine-root length (SRL, m fine root/g fine root mass). Fine-root vitality was assessed by the modified reduction of 2,3,5-triphenyl tetrazolium chloride method; the details were supplied in the online Appendix Information. When Chinese leek plants start to flower, all flowering stalks were cut manually twice in each month to conserve the nutrition for the vegetative growth. In late September 2017, subsamples of aboveground tissues of Chinese leek were harvested and weighted (fresh mass); the yield and economic output were then estimated.
Soil traits in the rhizosphere and RKN population densities
We only sampled the rhizosphere of the survived Sacha Inchi plants, i.e. the soil tightly adhering to the roots (approximately 0–2 mm from root surface), for the measurements of physico-chemical, RKNs and microbial traits. Because of the heterogeneous distribution of soil microorganisms in the field, five soil cores were collected from four points with 6 m away from each other and one core was collected from the centre of each plot. Five soil cores in each quadrate were then mixed thoroughly. Rhizosphere soils were carefully removed using a sterile knife. Soil physico-chemicals (water content, pH, organic matter, total nitrogen, total potassium, etc.) were analysed using routine methods (see the online Appendix Information for further detail). Three to four replications were used in the monoculture or the intercropping treatment. Subsamples for DNA extraction were transported to the laboratory on ice and stored at −80°C.
Individual samples consisting of ten soil cores were taken from the rhizosphere soil from each plot in each season in 2016 and 2017. RKN juveniles (J2) were extracted from two ca. 150 g soil subsamples using the sieving and centrifugation-flotation method. The extracted nematodes were heat killed and fixed in 4% formaldehyde solution (Hooper, Reference Hooper1970). Thereafter, nematodes were transferred to a counting dish, counted and identified (M. javanica) via morphological characteristics under a compound microscope and PCR-based molecular approach, as previously described (Wang et al., Reference Wang, Xie, Cui and Dong2014). The population levels (J2) were expressed as the numbers of M. javanica per 100 g fresh soil. Meanwhile, roots of the survived Sacha Inchi plants per plot were randomly collected in dry and wet seasons in 2017. The washed roots were mixed and chopped; and eggs were extracted from two 10 g-subsamples by macerating them for 10 min in a blender containing a 0.5% (v/v) sodium hypochlorite solution. Resistance was evaluated in terms of numbers of eggs per gram fresh root (Eggs/Gram roots).
DNA extraction and sequencing
Total microbial genomic DNA was extracted only in the wet season (late July) in 2016, when plant growth is fastest and M. javanica population level is highest. DNA amplification and sequencing were performed as previously described (Cai et al., Reference Cai, Zhang, Yang and Wang2018; Cai et al., Reference Cai, Wang, Bhadra and Gao2020). The details were supplied in the online Appendix Information. Briefly, the hypervariable V4-V5 regions of 16S rRNA genes were amplified using the barcode primers 515F and 907R, and the fungal ITS region was amplified by ITS1 and ITS2 primers. The optimized sequences were clustered into operational taxonomic units (OTUs) using the USEARCH program (version 7.1) at the 97% similarity level for both bacteria and fungi. Subsequently, analyses with the richness estimators (Chao 1) and abundance-based coverage estimators (Ace), Good's coverage estimator and α diversity (within samples) estimators (Shannon and Simpson index) were performed, and the rarefaction curve was generated using the Mothur software package. The taxonomy assignment of each sample was conducted using the silva of the bacterial ribosomal database (version 123) and ITS fungi unite database (Release 6.0, http://unite.ut.ee/index.php); the confidence level was 0.70. The Mothur software package was used to generate the Venn diagrams that compared the phylogenetic structure of the libraries. The heat maps were also generated to graphically display the abundance of species in different samples using the vegan package in R (version 2.4-1).
Statistical analysis
One- or two-way analysis of variance (ANOVA) followed by Tukey's test was used to test for significant differences in the plant and soil traits, indices of richness and α diversity and the relative abundance of phyla between two groups (i.e. Sacha Inchi monoculture v. Sacha Inchi/Chinese leek intercropped system), using SPSS 22.0 (SPSS Inc., Chicago, IL, USA).
The β-diversity was calculated in QIIME based on the weighted and unweighted UniFrac distance (Lozupone and Knight, Reference Lozupone and Knight2005). For visualization of compositional differences, the UniFrac distance matrix was used to perform the principal coordinate analysis scaling (PCoA) in R vegan package.
Results
Plant phenology and growth
The climate factors (average monthly temperature and precipitation) did not vary greatly between 2016 and 2017 (Figs 1(a) and (b)). Both Sacha Inchi and Chinese leek plants grow fast in the wet season; small Chinese leek plants (plant height <40 cm) did not lead to light competition aboveground for Sacha Inchi plants (ca. 1.6 m in flat canopy height). As a recurrent tropical woody crop, Sacha Inchi plant blooms and fruits continuously; massive flowering occurred in the wet season, but the peak values of fruit (seed) maturation were obtained in the cool and dry season (from December to April). In general, the seasonal pattern of fruit (seed) maturity of Sacha Inchi plants throughout two growing seasons (Figs 1(c) and (d)) and plant growth (stem diameter; Figs 1(e) and (f), P = 0.092–0.204) did not differ greatly between the monoculture and intercropping systems. Whereas in the intercropping system, a clear temporal divergence of resource acquisition was observed between two crops: during the fruit-maturing periods of Sacha Inchi plants, the aboveground tissues of Chinese leek plants were completely withered (Figs 1(g) and (h)).

Fig. 1. Climatic variables and plant growth and phenology (n = 8–10) of P. volubilis (PV) and A. tuberosum (AT) plants in the experimental periods.
Plant and soil physicochemical traits
Compared with monoculture, intercropping did not significantly affect SRL (Fig. 2(a); P = 0.146) and fine-root vitality (Fig. 2(b); P = 0.223) in the wet season in 2016. Intercropping did not affect seed yield per plant of the survived plant in 2016 (Fig. 2(c); P = 0.153) or in 2017 (Fig. 2(d); P = 0.243), but increased the survival rate (Figs 2(e) and (f); P < 0.001). Thus, the enhanced seed yield per unit area of Sacha Inchi plants was dominantly attributed to its higher survival rate (Table S1). Based on the estimated economic input and output in the farming systems, much higher practical benefits (increased by 54.8% in net income) were obtained in the intercropping system relative to monoculture (Table S1).

Fig. 2. Fine-root traits, plant survival and total seed yield of P. volubilis plants in P. volubilis monoculture (PV) and P. volubilis/A. tuberosum intercropping (PV_AT) groups. Different letters indicate the significant difference at the P < 0.05 level. SRL, specific fine-root length. Fine-root traits were measure in 2016.
There was no significant season × treatment interaction for the measured physical-chemical properties in the rhizosphere of Sacha Inchi plants (all P > 0.05), except for the available N and K (Fig. 3), implying that the responsiveness to intercropping did not differ greatly among seasons. Compared with monoculture, intercropping decreased soil water content only in the dry season (Fig. 3(a); P = 0.012), while pH value did not vary greatly in each season (Fig. 3(b); all P > 0.05). Although organic matter and total N content were slightly lower in the Sacha Inchi/Chinese leek intercropping system than those in monoculture, no significant differences were found (Figs 3(c) and (d); all P > 0.05). Intercropping increased total K and available P and K contents (Figs 3(e), (g) and (h); all P < 0.05), but decreased available N content (Fig. 3(f); P = 0.007), relative to monoculture.

Fig. 3. Soil physicochemical properties in the rhizosphere of P. volubilis plants in monoculture (PV) and P. volubilis/A. tuberosum intercropping (PV_AT) groups in 2016. Different small and capital letters indicate significant differences between the different cropping systems within each season (C) and between different seasons (S), respectively, at the P < 0.05 level. *P < 0.05, **P < 0.01, ***P < 0.001, ns, no significance.
Populations of root-knot nematodes
In the nearby (ca. 70 km away) Sacha Inchi monoculture plantation, M. javanica populations in the rhizosphere was much higher than those in our studied plantation; the estimated threshold level for the survival of Sacha Inchi plant is about 600–750 no. J2/100 g soil (Fig. S1). A strong seasonal pattern was found in the populations of M. javanica in the rhizosphere of Sacha Inchi plants in both 2016 and 2017 in both monoculture and intercropping groups, with the highest (170–270 no. J2/100 g soil) and lowest (16–25 no. J2/100 g soil) population density occurring in the wet and cool season, respectively (Figs. 4(a) and (b)). Compared with monoculture, without great differences in the cool or dry season, intercropping significantly suppressed the M. javanica populations in the rhizosphere in the wet season in both 2016 (decreased by 36.2%; P = 0.008) and 2017 (decreased by 27.5%; P = 0.011) (Figs 4(a) and (b)), and decreased the numbers of eggs per gram fresh root of Sacha Inchi plant in 2017 (Fig. 4(c); P = 0.012).
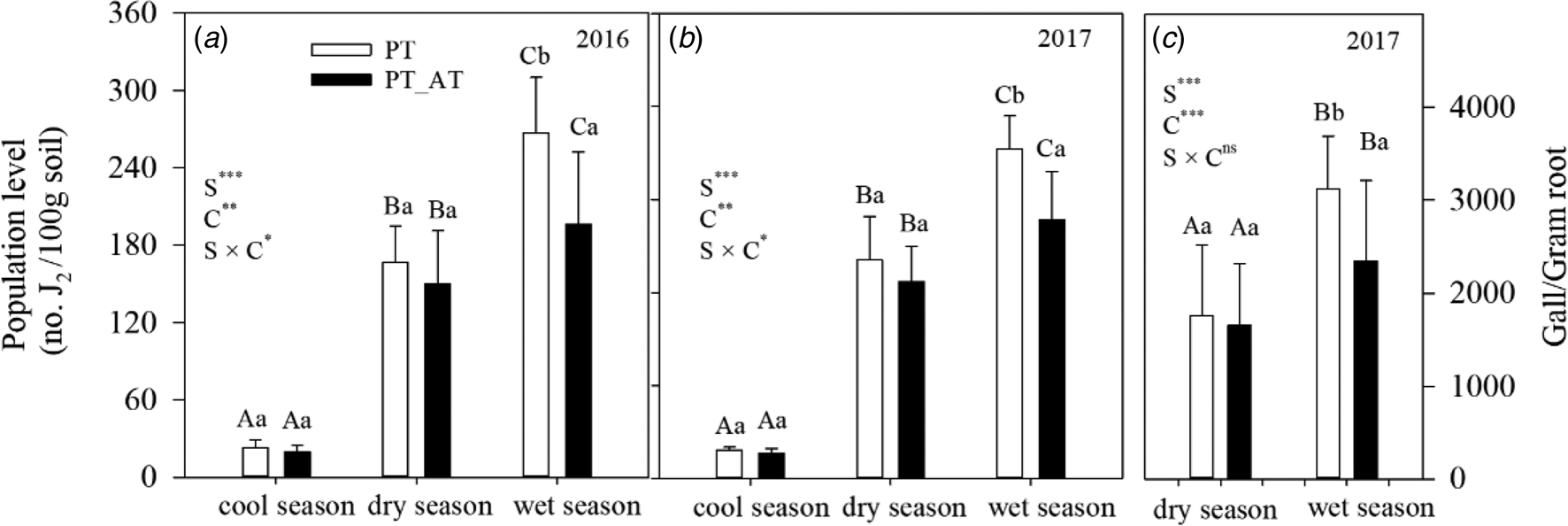
Fig. 4. Seasonal dynamics of the population densities of M. javanica in the rhizosphere (panel a and b) and eggs per gram root (Eggs/Gram roots) (panel c) of P. volubilis plants in monoculture (PV) and P. volubilis/A. tuberosum intercropping (PV_AT) groups. Different small and capital letters indicate significant differences between the different cropping systems within each season (C) and between different seasons (S), respectively, at the P < 0.05 level. *P < 0.05, **P < 0.01, ***P < 0.001, ns, no significance.
Microbial diversity and community composition
With good quality sequences generated from the 16S rRNA sequencing (bacteria) and ITS sequence reads (fungi) obtained for all samples (Table S2), the rarefaction curves showed that adequate sequencing depth was achieved (Fig. S2). From the 16S rRNA sequencing data, there are 620 and 420 specific OTUs in monoculture and the intercropping system, respectively; 1579 OTUs (60.3%) were shared within the two groups (Fig. S3(A)). Meanwhile, from the ITS sequence data, 524 and 458 specific OTUs were obtained in the monoculture and the intercropping group, respectively; only 448 OTUs (31.3%) were shared within the two groups (Fig. S3(B)). Thus, the microbes formed two spatially separable niches exhibiting distinct and overlapping microbial communities.
Compared with monoculture, intercropping decreased the richness (estimated by Ace and Chao1) of fungi (P < 0.050), but not bacteria (P > 0.050) (Figs 5(a) and (b)). No significant difference in α-diversity (Shannon and inverse Simpson diversity indices) of fungi or bacteria was found between the monoculture and the intercropping system (Figs 5(c) and (d); all P > 0.050). At the phylum level, Proteobacteria (>81%) was the dominant bacteria in both monoculture and intercropping groups, followed by Firmicutes, Actinobacteria, and Acidobacteria and Chloroflexi (Fig. S4). Compared with monoculture, intercropping increased the relative abundances in Firmicutes (P = 0.042) and Actinobacteria (P = 0.033), but decreased those in Acidobacteria (P = 0.030) and Proteobacteria (P = 0.046). Whereas for the three-dominant fungal phylum, Ascomycota (>81%) was the predominant in both monoculture and intercropping groups. Intercropping did not significantly affect the relative abundance of Ascomycota, but increased Zygomycota and decreased Basidiomycota relative to monoculture (Fig. S4). At the genera level in the intercropping system v. monoculture, Bacillus was the most abundant bacterial genera (17.5 v. 11.3%), followed by Gaiellales (4.5 v. 3.4%), Nitrospira (3.3 v. 3.6%), Lactococcus (2.4 v. 3.4%), Massilia (1.3 v. 3.4%), Acidobacteriaceae_Subgroup_1_uncultured (0.01 v. 3.3%) and Lysobacter (2.1 v. 0.03%) (Fig. 6(a)). Their relative abundances differed significantly between these two groups (one-way ANOVA, all P < 0.05), except for Nitrospira. Meanwhile, for the fungal genera in the intercropping system v. monoculture, Nectriaceae_unclassified (22.3 v. 16.5%) was the most abundant, followed by Fusarium (10.8 v. 15.9%), Chaetomiaceae_unclassified (15.5 v. 10.3%), Humicola (10.7 v. 8.0%), Mortierella (11.1 v. 3.7%), Gibberella (2.9 v. 3.8%) and Ascomycota_unclassified (2.5 v. 3.9%) (Fig. 6(b)). Intercropping greatly decreased the relative abundances of Fusarium and Gibberella, but increased those of Nectriaceae_unclassified, Chaetomiaceae, Humicola and Mortierella (all P < 0.05), relative to monoculture.

Fig. 5. Richness index and α diversity index for the microbe communities of the rhizosphere samples based on 16S rRNA (bacteria) and ITS sequencing data (fungi) in the rhizosphere of P. volubilis plants in monoculture (PV) and P. volubilis/A. tuberosum intercropping (PV_AT) groups. Ace: abundance-based coverage estimator. Mean values (means ± s.d., n = 3) followed by different letters indicate significant differences between two groups at the P < 0.05 level.

Fig. 6. Colour online. The relative abundance of the most numerous bacterial and fungal communities at the genus level in the rhizosphere of P. volubilis plants in monoculture (PV) and P. volubilis/A. tuberosum intercropping (PV_AT) groups.
Both the weighted and unweighted UniFrac distance revealed that the samples in the intercropping system were clearly diverged from those in monoculture along the first principal coordinate (PCoA1) for both bacterial and fungal communities (Fig. 7; Fig. S5), indicating that these two groups harboured strikingly distinctive microbiota. Based on the 100 most abundant bacterial genera indicated in heat map plot, intercropping showed a different pattern of bacterial or fungal community structure relative to monoculture (Fig. S6).

Fig. 7. Colour online. Principal component analysis (PCA) plots of the OTU-based weighted UniFrac distances showing the variation in the fungal and bacterial communities between samples in monoculture (PV) and P. volubilis/A. tuberosum intercropping (PV_AT) groups.
Discussion
Intercropping enhanced plant survival and differentially affected soil physico-chemical traits
In our intercropping system, owing to the relative high flat canopies of Sacha Inchi plants (ca. 1.6 m) and small size of Chinese leek (plant height <0.40 m), competition for light of Sacha Inchi plants, i.e. shade effect, did not exist, as the aboveground tissues between these two plants were completely spatially separated. In addition, a partially temporal divergence of acquisitive nutrient foraging strategies via niche separation occurred, as the aboveground tissues of Chinese leek were totally withered during the periods of the peak fruit (seed) maturity of Sacha Inchi plants when large resources are needed. Intercropping did not greatly affect the resource acquisitive syndrome of fine-root traits (SRL and vitality), plant growth and total seed yield of the survived Sacha Inchi plants. Such findings highlight the roles of divergence of plant adult stature and phenology in this intercropping system, where different plant functional groups enabled better use of resources and at least did not negatively affect the healthy woody plants. However, interspecific belowground competition for soil resources (nutrition and water) existed to a certain extent, as available N contents across all seasons and water content in the dry season decreased in the rhizosphere of Sacha Inchi plants. Soil pH, organic matter and total N content were not increased by intercropping. Therefore, the intercropping effects on soil total C and N are limited for the target woody crop if the roots of the small companion crop are in far proximity (Abagandura and Kumar, Reference Abagandura and Kumar2020). Higher P and K availabilities were found in the intercropping system compared with monoculture. That maybe due to the evidence coming from the short-status vegetables that high enzyme activity stimulated by root exudates of Allium species increased soil available nutrition contents (Zhou et al., Reference Zhou, Yu and Wu2011; Xiao et al., Reference Xiao, Cheng, Meng, Liu, Li and Dong2013).
On the other hand, less suffered injuries occurred in the intercropping system, which was indicated by the higher survival rate of Sacha Inchi plants relative to monoculture. Most of the dead plants occurred in the dry season (March to April) when water uptake by root systems and seed production were severely inhibited (Geng et al., Reference Geng, Chen, Yang, Jiao, Zhang and Cai2017); the roots of most of them rotted away (Cai's personal observation). These syndromes conferring the possibility that hydraulic failure of plant water transport owing to the combined effects of RNKs and pathogens (e.g. Fusarium oxysporum) that caused root wilt, as the RNKs population here is far below the estimated threshold level of plant survival obtained from the nearby farming land. The RNKs-pathogen complex led to worse symptoms than those produced by RKNs or pathogen alone (Ravichandra, Reference Ravichandra2014). On the other hand, intercropping is indeed a labour-intensive practice for the management of the economic input per unit land. But along with more seed yield harvested per unit area, the additional surplus of green tissues of Chinese leek greatly increased the net income of production, due to their intrinsic tillering ability and thus densely growing plants.
Intercropping suppressed Meloidogyne javanica
In line with the fact that high temperatures are favourable for hatching, the development and invasion of M. javanica (Davila et al., Reference Davila, Allen and Dickson2005), M. javanic populations in the rhizosphere of Sacha Inchi plants in the dry and wet seasons in both monoculture and intercropping groups were much higher than those in the cool season. Besides acting as allelochemicals, the volatile substances or root exudates (mainly allicin and sulphides) released into soil by Allium plants inhibited RKNs directly (Ji et al., Reference Ji, Li, Meng, Dong, Zhang and Qiao2019). Compared with monoculture, intercropping greatly suppressed the populations of M. javanica in the wet season. We presumed that changes in M. javanica populations in the intercropping system may be attributed to the combined effects of the direct allelopathy and induced-suppressiveness effects via modifications of the indigenous microbes in the rhizosphere. Uncovering the perceptible shifts of microbial consortia in the suppressive soils may provide a better understanding of the potential microbiota associated with RKNs suppressiveness. Recently, the most commonly detected antagonistic bacterial and fungal genus in soils with specific suppressiveness to plant-parasitic nematodes was summarized (Topalovic et al., Reference Topalovic, Hussain and Heuer2020). In our current study, among the most abundant bacteria genera, Bacillus and Lysobacter were greatly enriched in the intercropping system where M. javanica populations were suppressed. The spore-forming Bacillus species are almost ubiquitous in nature. It was known that Bacillus species produce nematicidal and fungicidal compounds and were generally isolated from suppressive soils (Zhou et al., Reference Zhou, Yuen, Wang, Wei and Ji2016; Sansinenea, Reference Sansinenea, Singh, Keswani, Reddy, Sansinenea and García-Estrada2019). But for Bacillus isolates in tomato plants, the main mechanism suppressing RKNs was not direct but plant-mediated (Adam et al., Reference Adam, Westphal, Hallmann and Heuer2014). Several species in genus Lysobacter produce a range of metabolites (e.g. extracellular enzymes) with activity against microbes (bacteria and fungi) and RKNs (Exposito et al., Reference Exposito, Postma, Raaijmakers and Bruijn2015; Zhou et al., Reference Zhou, Yuen, Wang, Wei and Ji2016). As for the fungi, the relative abundances of Humicola and Mortierella were largely increased in the intercropping system relative to monoculture. Humicola spp. can produce conidia that adhere to the cuticle of juveniles of RKNs and thereafter penetrate and kill them before root invasion (Wang et al., Reference Wang, Zhang and Jiang2016). Serving as nematophagous fungi, the hyphae of Mortierella spp. was observed inside the gelatinous matrix and hatched eggs of RKNs (Orion et al., Reference Orion, Kritzman, Meyer, Erbe and Chitwood2001). By developing a high-throughput screening method of generalist pathogens against RNKs, a new fungal isolate (i.e. Mortierella globalpina) was recently found, which is pathogenic against RKNs via hyphal adhesion and penetration of the cuticle layer, and subsequently digestion of its cellular contents (DiLegge et al., Reference DiLegge, Manter and Vivanco2019). Overall, our study suggests how the anti-nematode microbial consortia can derive from indigenous (resident) microbiomes, providing primary information for managing the microbial communities against RKNs in our studied intercropping system.
Intercropping shifted microbial composition and structure
No differences were found in diversities of both bacterial and fungal communities between the intercropping and monoculture system, probably implying that the rhizosphere of Sacha Inchi plants in the intercropping system was not conducive to the establishment and maintenance of a large microbial repository (Cai et al., Reference Cai, Zhang, Yang and Wang2018). Intercropping significantly decreased the populations (i.e. richness) of fungi, rather than bacteria. Richness may not be sufficient to predict soil health, but it may be a prerequisite to soil health (Philippot et al., Reference Philippot, Raaijmakers, Lemanceau and van der Putten2013). Thus, soil fungal community was more sensitive than bacterial community to the effect of intercropping system, which was also proved by the less proportion of bacterial OTUs shared between two cropping systems than fungi. While, it was reported that intercropping with green garlic significantly decreased fungal populations, but stimulated the bacterial and actinomycetes population in the cucumber/garlic intercropping (Xiao et al., Reference Xiao, Cheng, Meng, Liu, Li and Dong2013). Moreover, the intercropped onion or garlic with cucumber promoted both bacterial and fungal communities, and the effects persisted into later growing seasons (Zhou et al., Reference Zhou, Yu and Wu2011). These differences might be attributed to different allelopathy of the released root exudates and antifungal effects between Allium species, as well as different intercropping systems studied (agriculture v. agroforestry). Playing an important role in C and N cycling in terrestrial ecosystems, fungi was found to be decreased by management practices (e.g. vegetation-type shifts, control of weeds and fertilization) (Philippot et al., Reference Philippot, Raaijmakers, Lemanceau and van der Putten2013; Cai et al., Reference Cai, Zhang, Yang and Wang2018). Reduction of total fungi in the intercropping system might also be associated with the inhibitory effect of root exudates of Chinese leek on soil-borne diseases (Yu, Reference Yu1999). Based upon weighted (based on abundances of taxa) and unweighted (sensitive to rare taxa) UniFrac distances, microbial structure within the intercropping was distinctly different from that in monoculture for both bacterial and fungal communities, indicating that changes of soil bacterial and fungal compositions as a response to different cropping system existed. This was consistent with the numbers of other intercropping systems that intercropping often resulted in distinct soil microbial community structures (Zhou et al., Reference Zhou, Yu and Wu2011). The interaction of soil microorganisms and different amount and kinds of plant exudates (Chinese leek v. Sacha Inchi) may affect soil microbial communities, and these differences can stimulate species-specific shifts in soil microbial communities (Zhou et al., Reference Zhou, Yu and Wu2011; Zhang et al., Reference Zhang, Mallik and Zeng2013). Whereas, Bacillus spp., generally stimulating plant growth through increasing the uptake of nutrition (e.g. P, K and Fe) (Sansinenea, Reference Sansinenea, Singh, Keswani, Reddy, Sansinenea and García-Estrada2019), was enhanced in the intercropping system. Higher Bacillus abundance could also contribute to the higher soil available P and K contents in the intercropping system than monoculture.
Additionally, in a nearby Sacha Inchi plantation (<800 m), Chai et al. (Reference Chai, Yang, Fu, Pan, Tang, Li and Xu2018) found that the fungus, F. oxysporum isolate FoPvo1, can cause root rot, growth retardation, wilting and plant death. In our studied plantation, population size of Fusarium in the intercropping system was much lower than that in monoculture, although the species level was not identified due to the current limited technology. Therefore, it is possible that intercropping with Chinese leek can suppress the root wilt of Sacha Inchi plants. It was also reported that Chinese leek significantly inhibited the growth of F. oxysporum f. sp. cubense W. C. Snyder and H. N. Hansen 1940, a causal agent of Fusarium wilt in banana in the same studied geographical area (Zhang et al., Reference Zhang, Mallik and Zeng2013). The enhanced antifungal activity, such as Lysobacter (Zhou et al., Reference Zhou, Yuen, Wang, Wei and Ji2016) and Bacillus (Sansinenea, Reference Sansinenea, Singh, Keswani, Reddy, Sansinenea and García-Estrada2019; Topalovic et al., Reference Topalovic, Hussain and Heuer2020) in our studied intercropping system, could also play a keystone role in controlling this pathogen, although we have not demonstrated whether the relationships are causal.
Conclusion
The spatio-temporal divergence due to different plant adult stature and phenology between the two crops play important roles in our studied intercropping system. The phenology, fine-root traits and growth of healthy Sacha Inchi plants were not greatly affected by intercropping. Higher total seed yield per unit area in the intercropping system was ‘protected’ (i.e. higher plant survival rate of Sacha Inchi plants), rather than being increased directly. Relative to monoculture, the enhanced specific antagonistic microbial consortia in the rhizosphere, e.g. Bacillus, Lysobacter, Humicola and Mortierella, may attribute to the suppressiveness of M. javanica populations. The decreased size of pathogen-containing Fusarium, which potentially causes wilt and root rot disease, is also helpful for the survival of Sacha Inchi plants in the intercropping system. The diversified production per unit area and much higher net economic returns in the intercropping system provide higher insurance for farmers. Further attempts are required to expand the comprehensive understanding of the plant-RNKs-microbes (e.g. plant-beneficial microbe and pathogen) interactions in the suppressive soils over time, which may help to develop efficient and sustainable practices for the control of the populations of M. javanica and soil-borne diseases in the Sacha Inchi plantations.
Supplementary material
The supplementary material for this article can be found at https://doi.org/10.1017/S0021859621000708
Acknowledgements
We thank Yang C., Dong X., Liu Y and Qiao M. for their help in the field work and measurements. The first two authors contributed equally.
Financial support
This work was funded by grants from the National Natural Science Foundation of China (31971697) and the Forestry Innovation Project in Guangdong (2021KJCX002).
Conflict of interest
None.
Ethical standards
Not applicable.