Introduction
Etching traces are a characteristic group of borings produced by variable sessile epibiontic organisms colonizing lithic and skeletal hard substrates (see Bromley and Heinberg, Reference Bromley and Heinberg2006, for a review). Often, the etching traces are the only evidence of their producers. Therefore, they carry important information about benthic fossil assemblages, which can be used for paleoenvironmental interpretations. Many of the etching traces are ichnotaxonomically classified and well understood, such as Centrichnus (e.g., Neumann et al., Reference Neumann, Wisshak, Aberhan, Girod, Rösner and Bromley2015) or Podichnus (e.g., Bromley and Surlyk, Reference Bromley and Surlyk1973; Bromley, Reference Bromley2008; Robinson and Lee, Reference Robinson and Lee2008), but others are still awaiting closer characterization.
In this paper, a new ichnogenus and ichnospecies of a large and deep spiral etching trace is presented. It occurs in limestone boulders in one locality on the Miocene rocky shore of the Paratethys sea at the northern margin of the Carpathians Foredeep in southern Poland (Fig. 1), where a similar but partly different etching trace was reported by Radwański (Reference Radwański1969, Reference Radwański1970, Reference Radwański1977) and referred by him to serpulid polychaetes. We are not aware of any further studies on this trace fossil in this locality or elsewhere. New findings of well-preserved, similar specimens in the same locality and their good visualization enabled by rubber casts shed new light on their morphology and origin.
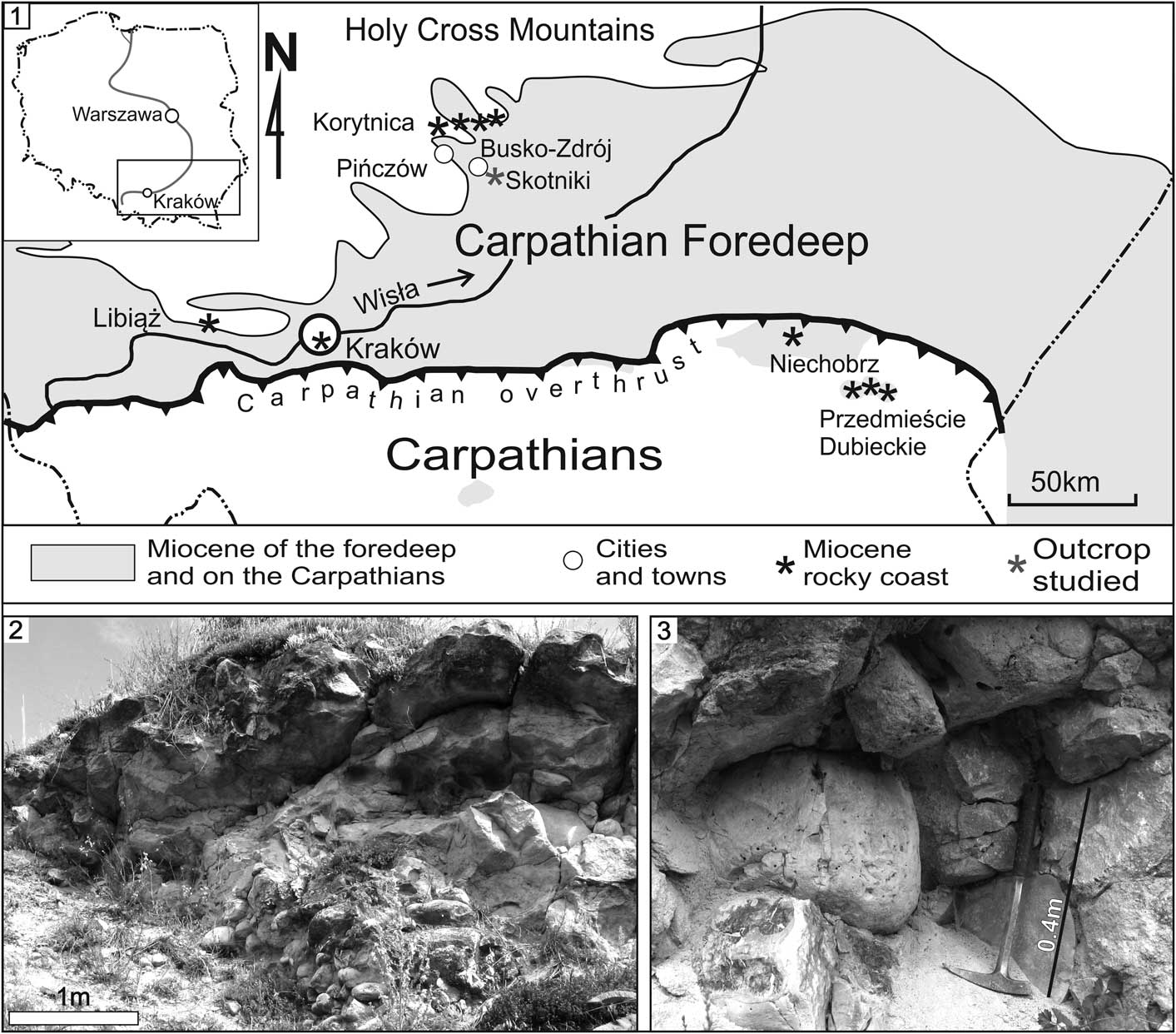
Figure 1 Location and the deposits studied. (1) Location map. Range of the Miocene deposits after Radwański (1968, Reference Radwański1969), Rajchel and Uchman (Reference Rajchel and Uchman1999), and Oszczypko (Reference Oszczypko2001). (2, 3) accumulation of pebbles and boulders (Pińczów Formation) in the wall of the quarry.
The aim of this paper is to: (1) describe a new ichnogenus and ichnospecies of the mentioned trace in the context of its paleoenvironmental conditions and associated borings, and (2) discuss its origin.
Geological setting
The Carpathian Foredeep Basin developed in front of the overthrusting Carpathian orogen during the Miocene (e.g., Oszczypko, Reference Oszczypko2001). It was a part of the Paratethys sea and was connected to other foredeep basins of the Alpine orogenic system (e.g., Rögl, Reference Rögl1998). Its northern range is well marked by the Dalmatian type of rocky shore, on which bays, peninsulas, and islands developed on the Paleozoic and Mesozoic carbonate and clastic rocks, which is the southern cover of the Paleozoic massif of the Holy Cross Mountains; this rocky shore displays well-developed bioerosion (Radwański, Reference Radwański1964, Reference Radwański1965, Reference Radwański1969, Reference Radwański1970, Reference Radwański1977; Radwański and Górka, Reference Radwański and Górka2008). The southern shore of the basin, which developed on the Carpathian orogeny, is eroded or much more poorly exposed, but bioerosion is also present (Rajchel and Uchman, Reference Rajchel and Uchman1999).
The new ichnotaxon was found in an inactive quarry located on Zajęcza Góra hill at Skotniki, close to the line of the fossil rocky shore (Fig. 1; 50˚25'49.8"N, 20˚48'15.3"E). In the quarry, middle Miocene deposits of the Pińczów Formation lie discordantly on Mesozoic rocks. The lower part of the formation contains indistinctly bedded clast accumulations, which are almost 10 m thick and fill a large furrow eroded in soft Cenomanian sandstones. These deposits are considered a classic example of the transgressive succession of the Miocene (early Badenian) sea encroaching from the south on the southern margin of the Holy Cross Mountains (Radwański, Reference Radwański1969, Reference Radwański1973; Radwański and Górka, Reference Radwański and Górka2015).
The clasts include gravels, cobbles, and half-meter boulders and blocks (Figs. 1, 2). They are composed of hard Jurassic and Cretaceous limestones, marls, and cherts. Numerous clasts are bored by macro-organisms. Smaller clasts usually occur as matrix between larger clasts. Cementation of these deposits varies from loose rock to a hard-cemented conglomerate. Laterally, these deposits transition to sandy limestones and they are replaced at the top by red-algal limestones of the Pińczów Formation, which contains abundant fossils of benthic fauna, mostly pectenid bivalves, bryozoans, and large foraminifers.
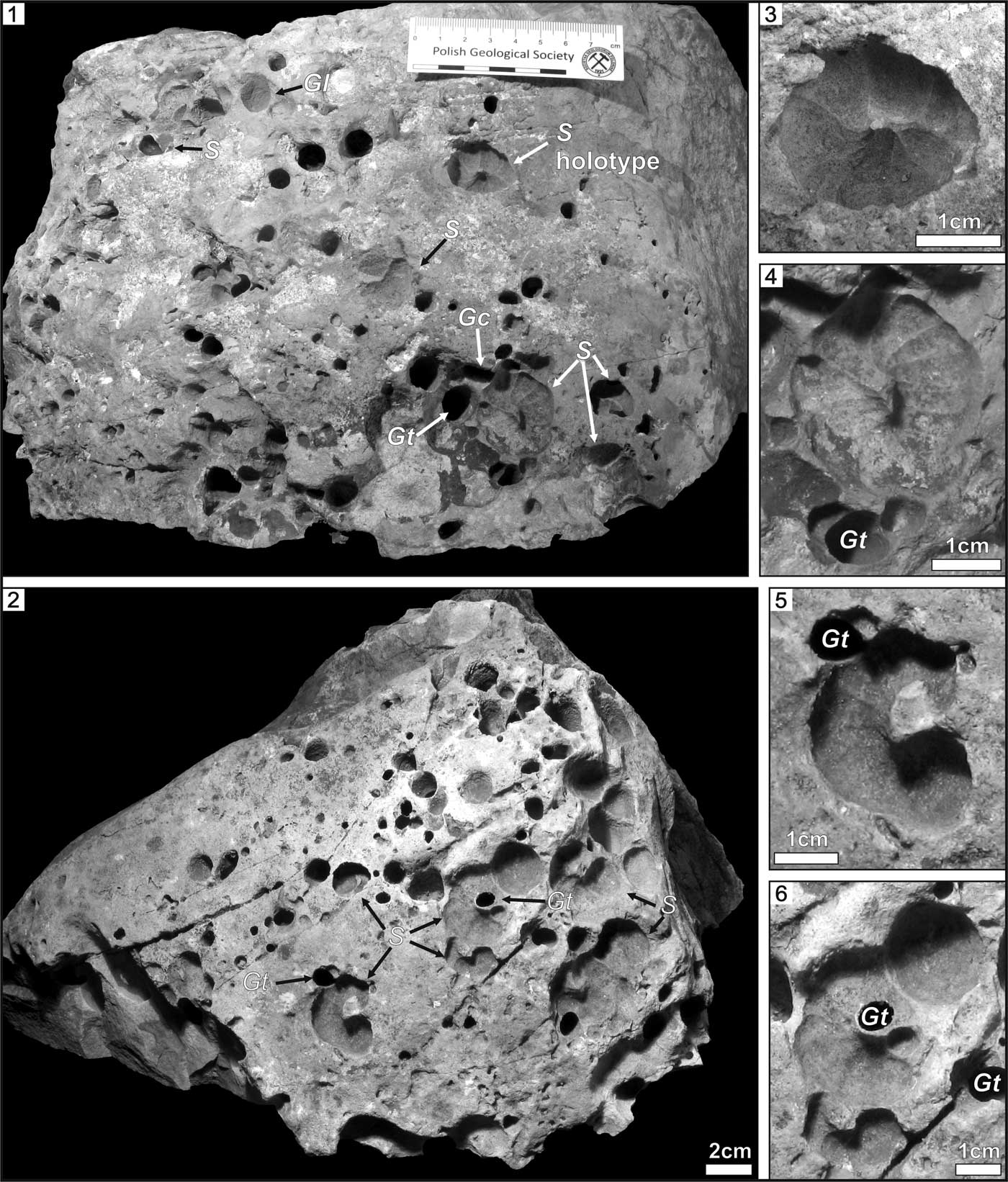
Figure 2 Bored boulders with Spirolites radwanskii n. igen. n. isp. (1, 3, 4) INGUJ214P/SZG1; (2, 5, 6) INGUJ214P/SZG2. (1, 2) Views of the upper surfaces of the boulders; (3) holotype of S. radwanskii n. isp.; (4–6) paratypes. S=Spirolites radwanskii n. igen. n. isp., Gc=Gastrochaenolites cf. G. cluniformis, Go=Gastrochaenolites ?orbicularis, Gt=Gastrochaenolites torpedo.
The lower Badenian deposits with abundant Mesozoic clasts represent the lower part of the Pińczów Formation (= Baranów Beds; see Alexandrowicz et al., Reference Alexandrowicz, Garlicki and Rutkowski1982; Jasionowski, Reference Jasionowski1997) of the southern margin of the Holy Cross Mountains. This member has not been lithostratigraphically classified so far, but it is distinctly differ from the neighboring rocks.
Materials and methods
Repository and institutional abbreviation
The illustrated specimens are housed in the Nature Education Centre (CEP) of the Jagiellonian University – Museum of Geology in Kraków (institutional abbreviation INGUJ214P/SZG).
Systematic paleontology
Ichnogenus Spirolites new ichnogenus
Type ichnospecies
Spirolites radwanskii n. igen. n. isp., by monotypy.
Diagnosis
Depression in form of an involute spiral, smooth or with perpendicular annulation. Width of the spiral increases outward.
Etymology
From the Latin spira, spiral, and -lites, a common latinized ending in names of popular borings (e.g., Gastrochaenolites), in reference to lithic substrate.
Remarks
No other boring of this shape, except for Kardopomorphos Beuck et al., Reference Beuck, López Correa and Freiwald2008, which is typified Kardopomorphos polydioryx Beuck, López Correa, and Freiwald, Reference Beuck, López Correa and Freiwald2008, and produced by parasitic foraminifers. However, these borings are much smaller than Spirolites n. igen. and occur on skeletal substrates (Spirolites n. igen. occurs on boulders), may display branched canals, which can be extended deeply into the substrate, and have more or less the same width (Beuck et al., Reference Beuck, López Correa and Freiwald2008). Renichnus Mayoral, Reference Mayoral1987 can display some similarities referred to trochospiral shape of the producing vermetid gastropods, but it is composed basically of crescent depressions arranged in rows (Mayoral, Reference Mayoral1987; Taddei Ruggiero, Reference Taddei Ruggiero1999; Jagt, Reference Jagt2003; Hoşgör and Okan, Reference Hoşgör and Okan2010).
Spirolites radwanskii new ichnospecies
Figures 2–4, 5.2–5.5, 6.1, 6.3, 6.4
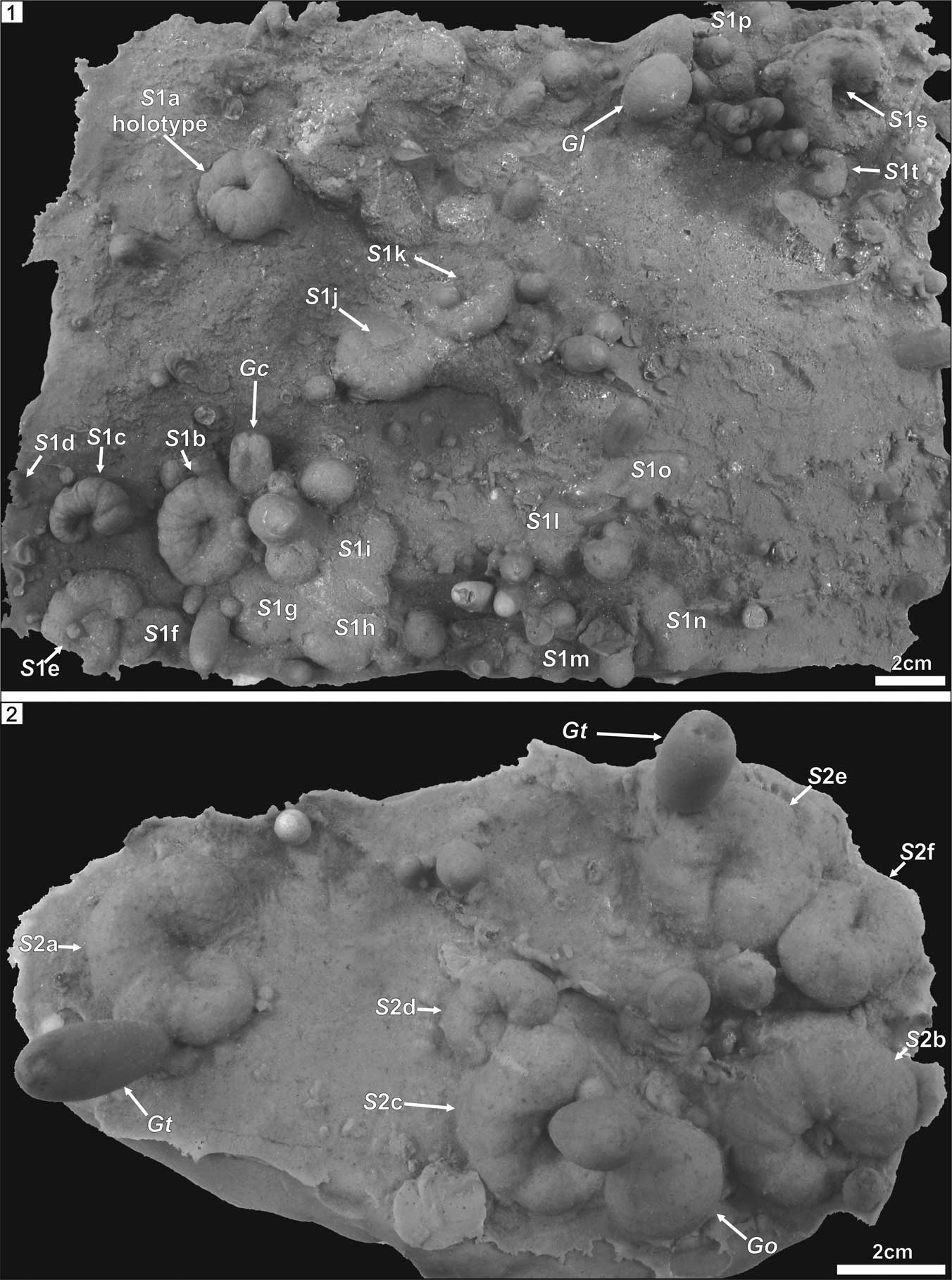
Figure 3 Rubber casts of the bored surfaces of the boulders. (1) INGUJ214P/SZG1; (2) INGUJ214P/SZG2. S1a–t and S2a–f=the type material of Spirolites radwanskii n. igen. n. isp. Gc=Gastrochaenolites cf. G. cluniformis, Go = Gastrochaenolites ?orbicularis, Gt=Gastrochaenolites torpedo.
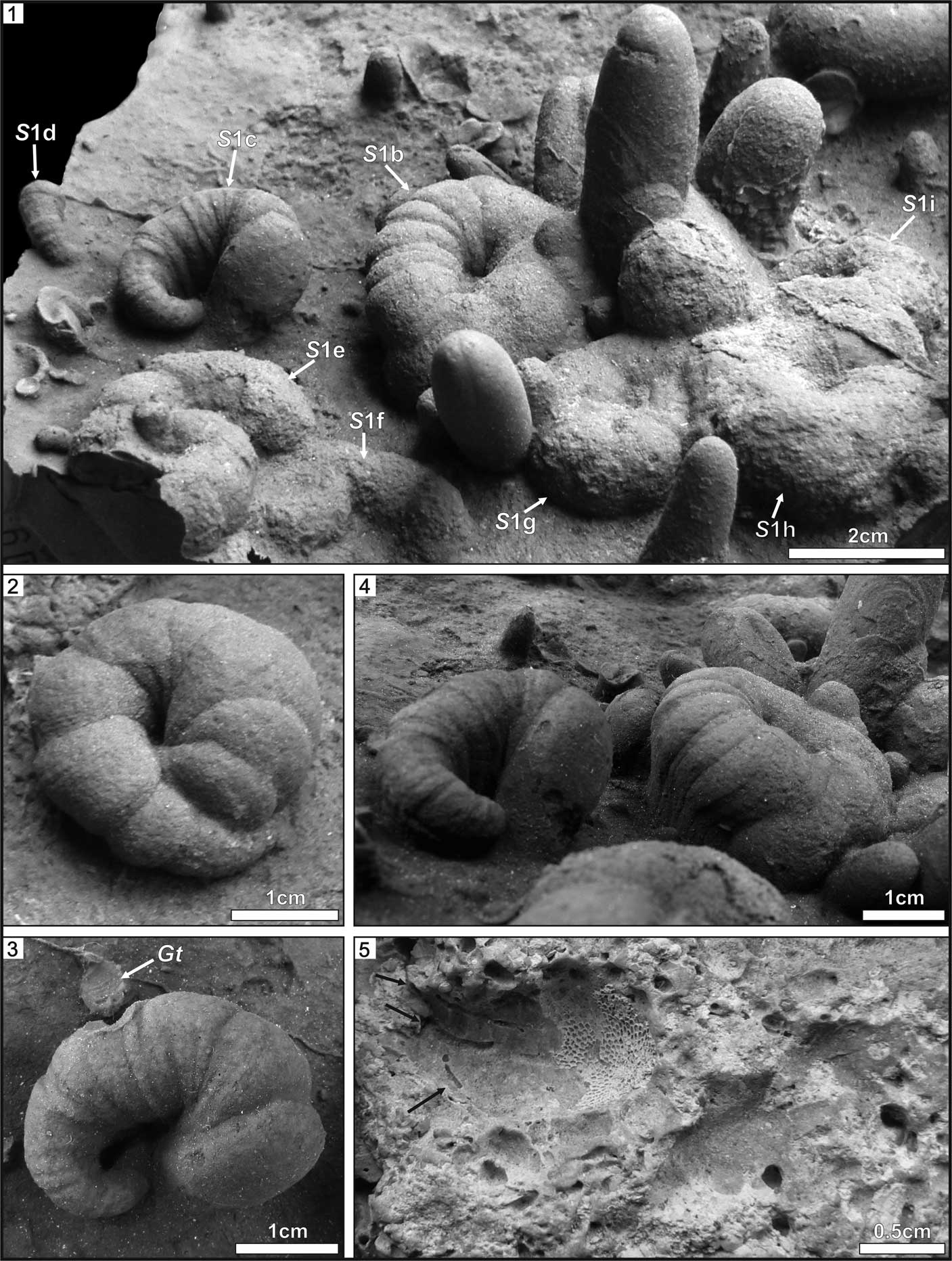
Figure 4 Details of the rubber casts (1–4) of the boulder INGUJ214P/SZG1 and a specimen (5) of Spirolites radwanskii n. igen. n. isp. INGUJ214P/SZG4. (1) Four stages of development of the borings: S1e–i=partly abraded specimens, S1b=fully coiled, well-preserved specimen, S1c=partly coiled specimen, S1d=small boring in the initial phase of development; (2) fully coiled specimen, S1a=the holotype; (3) different, partly coiled specimen, S1c; (4) side view of a trench, which shows the inverted Ω-like outline in cross section and two orders of annulation along the course; (5) Spirolites cuts Entobia (arrowed) and shows an encrustation by a Cheilostomata bryozoan.
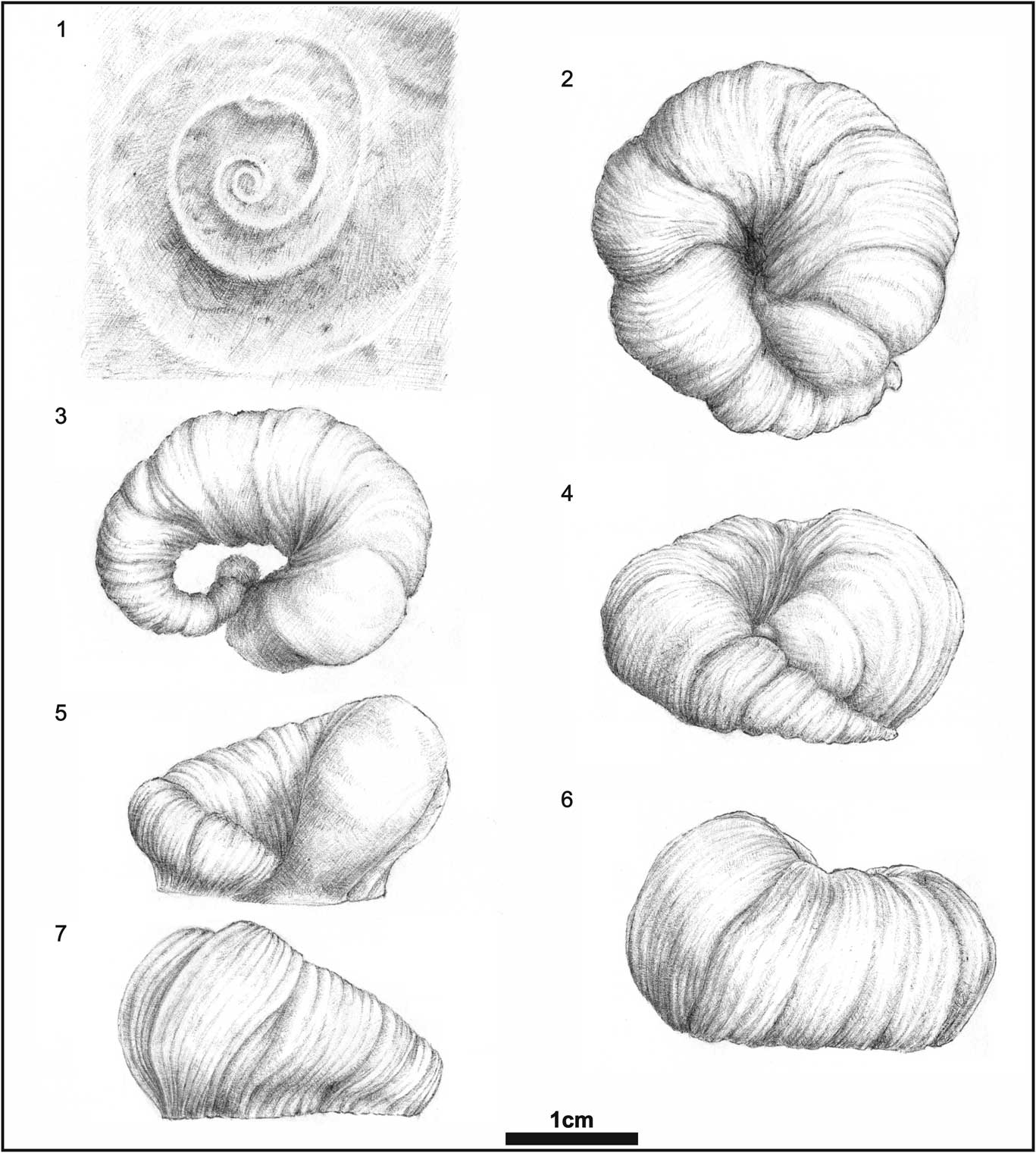
Figure 5 Sketch drawings of the spiral etching traces from the Polish Miocene. (1) Re-drawing of a trace from photograph by Radwański (Reference Radwański1977, pl. 10d), interpreted by him as a serpulid etching; (2, 3) Spirolites radwanskii n. igen. n. isp., top views of the negatives (rubber casts); (4–7) Spirolites radwanskii n. igen. n. isp., side views of the negatives (rubber casts); (2, 4, 6) specimen INGUJ214P/SZG1a, the holotype; (3, 5, 7) specimen INGUJ214P/SZG1c. Drawings by A. Wierzbicki.
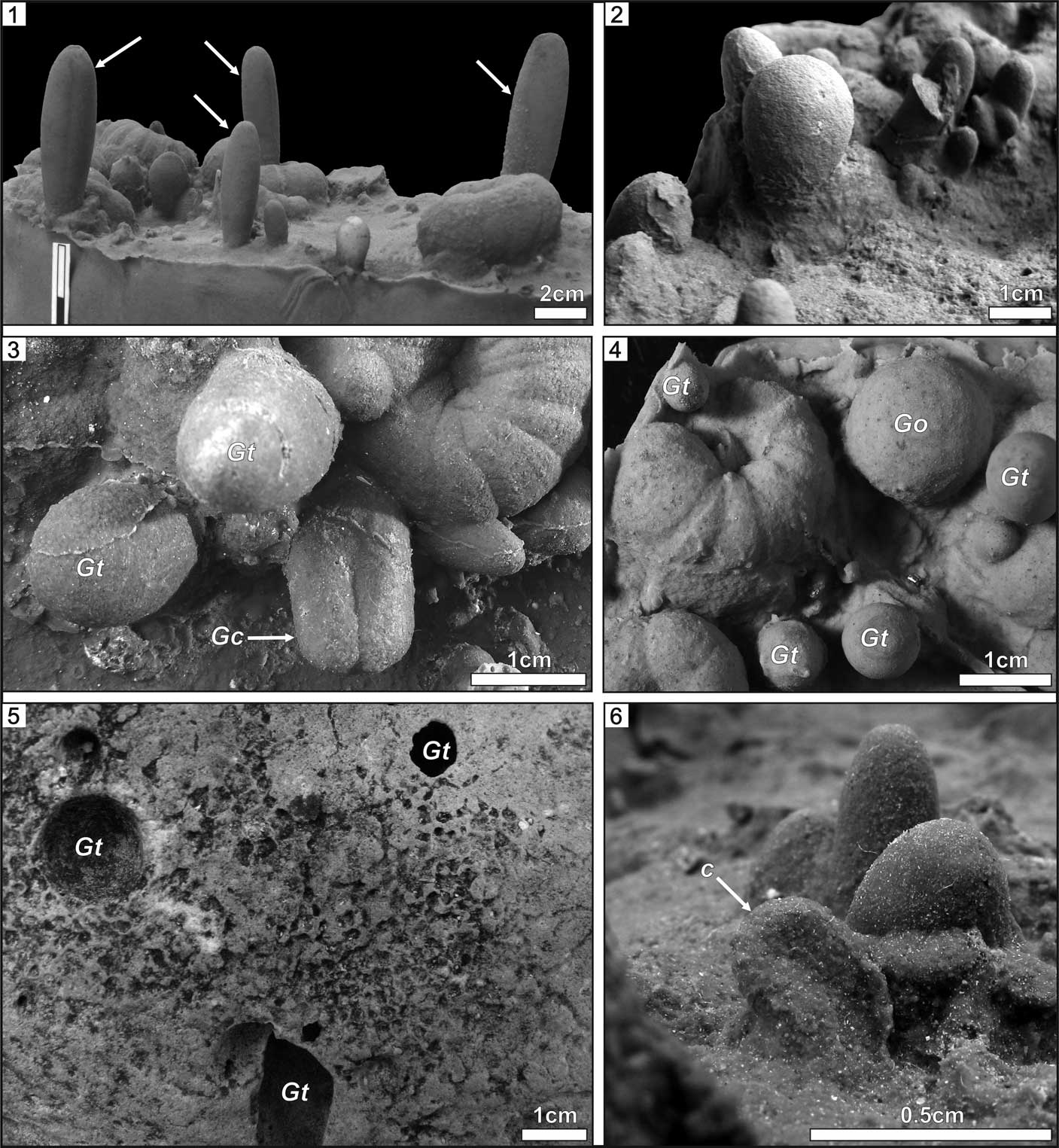
Figure 6 Associated borings. (1) Gastrochaenolites torpedo, arrowed; (2) Gastrochaenolites lapidicus; (3) Gastrochaenolites cf. G. cluniformis (Gc) and G. torpedo (Gt); (4) Gastrochaenolites ?orbicularis (Go) and G. torpedo (Gt); (5) Entobia isp. and G. torpedo (Gt); (6) Caulostrepsis isp., (C) arrowed.
Holotype
The specimen INGUJ214P/SZG1a is the holotype.
Paratypes and other material
The specimens in boulders INGUJ214P/SZG1 and INGUJ214P/SZG2 and pebbles INGUJ214P/SZG3 and INGUJ214P/SZG4 are paratypes. Two bored boulders of Jurassic limestones with chert nodules (INGUJ214P/SZG1 contain at least 17 specimens, INGUJ214P/SZG2 contains at least 10 specimens), two small pebbles (INGUJ214P/SZG3 and INGUJ214P/SZG4) with three specimens and two silicone casts of the borings in parts of INGUJ214P/SZG1 and INGUJ214P/SZG2. The particular specimens of Spirolites radwanskii n. igen. n. isp. are indicated by small letters as INGUJ214P/SZG1a–t, INGUJ214P/SZG2a–j, INGUJ214P/SZG3, and INGUJ214P/SZG4a, b.
Diagnosis
Depression in form of an involute spiral, with perpendicular annulation of the first and second order. It shows an increase in width outward with the spiral and steep margin from the wider side.
Type locality
Inactive quarry at Zajęcza Góra near Skotniki, central Poland (50°25'49.8"N, 20°48'15.3"E).
Type stratum
Pińczów Formation (Langhian—equivalent of the lower Badenian in the Paratethys divisions).
Description
Deep, trochospiral depression (trench), more or less tightly coiled. Its maximum width ranges in different specimens from 2–16 mm; usually its depth is slightly larger than wide. The coil is 12–43 mm wide. The completeness of coils differs among specimens, from 0.3 whorl in the smallest specimens (Figs. 2, 3.1) to 1.3 whorls in larger specimens (Figs. 3.1, 4.2). The direction of coiling in actual specimens is sinistral. The complete coil is circular to elliptical in outline. Width of the depression (trench) changes gradually along course of the coil. The narrowest (initial or proximal) and the widest (apertural or distal) parts are directed upward and located close to the surface of the substrate. Commonly, the apertural part is more steeply inclined than the initial, narrower part. The middle part of the coil is the deepest part of the boring. Locally, the trench is partly roofed by the substrate (Fig. 2.1, 2.5). The widest part (apertural) may surround or cut the initial part in some specimens (Fig. 4.2, 5.2–5.7). Cross section of the depression is in form of a half or incomplete circle (the latter resembles inverted Ω). Margins of the trench are annulated, with distinct, wider, and deeper annuli of the first order, 8–12 in number in most of specimens, within which distinct, narrower, and shallower, 5–10 second-order annuli can be present in some specimens. The first-order annulation is more distinct in larger specimens. The first-order annuli are straight or slightly curved, narrow crests (furrows on the casts) that are perpendicular to the trench. Individual annuli of the first order are 1–16 mm wide. The segments between annuli are convex outward, and become inwardly narrower. They form a series of wedges dividing the cast perpendicularly. The trench around the bounding furrows is slightly constricted. The last annuli (i.e., those in the distal [apertural] part) are usually distinct and show hemispherical surface. The second-order annuli are straight, indistinct ridges and furrows, <1 mm wide. Beside well-preserved specimens, shallower and less crisply preserved specimens of Spirolites are present (e.g., S1g–i in Fig. 4.1). The first-order annuli are poorly visible in them and their surface is slightly corrugated.
Morphometric parameters of separate specimens are shown in Table 1.
Table 1 Morphometric parameters of Spirolites radwanskii n. igen. n. isp. (in mm); 33 specimens. *=minimum value is taken in the case of values ‘at least’ (>).
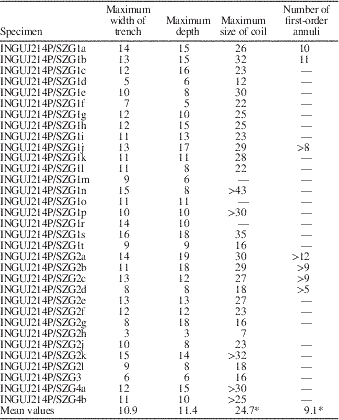
Spirolites radwanskii n. igen. n. isp. occurs foremost in patches on the upper surface of large boulders, which are at least 32 cm wide and ~30 cm high. Isolated specimens of Spirolites are more rare. Some specimens touch each other, or the less-distinct, shallower specimens are slightly truncated by deeper, sharply defined specimens (Fig. 2.5). The associated Gastrochaenolites cross cuts some specimens of Spirolites n. igen. on the edge of the coil or in the middle (Figs. 3.2, 4.1).
Etymology
To honor Professor Andrzej Radwański (1935–2016), who studied borings of the Polish Miocene for several years and brought them to international attention through his seminal publications.
Remarks
Spirolites n. igen. falls into the category “Spiral Borings” of Buatois et al. (Reference Buatois, Wisshak, Wilson and Mángano2017). The smallest specimen of Spirolites n. igen., intermediate, and large ones (Fig. 4.1) are considered as a series related to ontogenetic development of the trace maker. Apart from size differences, the development is expressed by increasing completeness of coils. The less-distinct, shallower specimens represent earlier stages of colonization of the substrate.
Ichnogenus Gastrochaenolites Leymerie, Reference Leymerie1842
Gastrochaenolites torpedo Kelly and Bromley, Reference Kelly and Bromley1984
Description
Date-shaped boring, 15–30 mm long, with smooth surface. The main chamber is 8–12 mm wide. The neck is absent, aperture is 6–10 mm wide. Both the basal and apertural parts are rounded to oval in transverse section. In some specimens, a cast commissure is visible as a straight, longitudinal shallow furrow.
Remarks
According to Radwański (Reference Radwański1964, Reference Radwański1969), this boring was formed by Lithophaga, but Gastrochaena also can be a producer of this ichnospecies (Kelly and Bromley, Reference Kelly and Bromley1984).
Gastrochaenolites lapidicus Kelly and Bromley, Reference Kelly and Bromley1984
Description
Flask-shaped structure, circular in transverse section, with a distinct chamber and a neck. The chamber is up to 8 mm wide. The whole trace is 27 mm long.
Remarks
Gastrochaenolites lapidicus is produced by the bivalves Lithophaga, Hiatella (Kelly and Bromley, Reference Kelly and Bromley1984), Rocellaria (Gastrochaena) dubia Pennant, Reference Pennant1777 (Bromley and Asgaard, Reference Bromley and Asgaard1993a), and possibly by Parapholas (Uchman, Kleemann, and Rattazzi, Reference Uchman, Kleemann and Rattazzi2017). Radwański (Reference Radwański1964, Reference Radwański1965, Reference Radwański1969) referred such borings to Aspidopholas.
Gastrochaenolites ?orbicularis Kelly and Bromley, Reference Kelly and Bromley1984
Figures 3.2, 6.4
Description
Boring composed of a smooth, spherical chamber, ~18 mm in diameter. The chamber contacts the surface, so the neck is not preserved.
Remarks
Because the neck is not preserved, the determination is unsure. Nevertheless, this ichnospecies is present in the quarry. Gastrochaenolites orbicularis is produced by the bivalve Jouannetia (Radwański, Reference Radwański1969; Kelly and Bromley, Reference Kelly and Bromley1984; Uchman et al., Reference Uchman, Kleemann and Rattazzi2017).
Gastrochaenolites cf. G. cluniformis Kelly and Bromley, Reference Kelly and Bromley1984
Description
Clavate boring, 15 mm long, with a smooth surface and a more or less distinct principal, vertical ridge (visible as a furrow in the cast) on one side of the chamber. The ridge on the opposite side of the chamber is absent. Both the basal and apertural parts are oval in horizontal section, with an indentation to the ridge. The chamber is 12 mm wide.
Remarks
According to Radwański (Reference Radwański1969), this boring was formed by the bivalve Gastrochaena, but the bivalve Botula also can produce Gastrochaenolites cluniformis in corals (Kelly and Bromley, Reference Kelly and Bromley1984).
Ichnogenus Entobia Bronn, Reference Bronn1838
Entobia ispp.
Description
A system of diverse, straight, curved, branched, rarely simple, usually discontinuous, partly unroofed channels, tunnels, and chambers, which in the outer side are arranged in linear tracts. The tunnels are discontinuous, usually 0.5–1 mm wide, up to 8 mm long. On the boulder surface, the chambers in outline form meshes that are 2–3 mm wide. They show different sizes, usually circular, ellipsoid, or polygonal in cross section.
Remarks
This trace fossil is rare in the clasts with Spirolites n. isp., but is more frequent in other cobbles and boulders. It is partly abraded. Entobia is produced by several species of sponges belonging to the family Clionaidae (Radwański, Reference Radwański1969; Bromley and D’Alessandro, Reference Bromley and D’Alessandro1984).
Ichnogenus Caulostrepsis Clarke, Reference Clarke1908
Caulostrepsis isp.
Description
U-shaped, slightly inclined structure, which appears as a flat pouch with an arcuate distal margin, encircled by a marginal tunnel, which slightly diverges to the surface. The pouch is 7.5 mm deep and up to 4 mm wide.
Remarks
This trace fossil was described by Radwański (Reference Radwański1964, Reference Radwański1969, Reference Radwański1970, Reference Radwański1977) as the boring of Polydora ciliata (Johnston, Reference Johnston1838). Caulostrepsis Clarke, Reference Clarke1908 is produced by spionid polychaetes, mostly Polydora Bosc, particularly P. ciliata (Johnston), mostly in up to 25 m of water depth (Boekschoten, Reference Boekschoten1966). The tracemakers are adapted to a wide range of environmental conditions, including low temperature and lowered salinity (see Hanken et al., Reference Hanken, Uchman and Jakobsen2012). The ichnotaxonomy of Caulostrepsis was discussed Bromley and D’Alessandro (Reference Bromley and D’Alessandro1983).
Discussion
Tracemaker of Spirolites radwanskii
Spirolites radwanskii n. igen. n. isp. does not contain any remains of calcareous tubes or shells, which would directly point to the trace maker. Morphology of the borings suggests that it was propagated deep into the rock gradually along a spiral path in the proximal part, increased its size, and steeply directed up in the distal part. In some cases, the distal part of the boring truncates the initial (proximal) part. The shape of the trace fossil resembles spiral tubes of some serpulid polychaetes and shells of some vermetid gastropods, which are considered here as potential trace makers.
The serpulid polychaete hypothesis is cited often because similar spiral trace fossils from the Miocene of Skotniki have been described by Radwański (Reference Radwański1969, pl. 8, figs. 3, 4) as etchings of the serpulid polychaete Pomatoceros sp. However, no direct evidence of that interpretation was provided. In a later paper, Radwański (Reference Radwański1977, pl. 10c–e) illustrated the same trace and referred it to etchings of serpulids, similar to the modern Serpula vermicularis Linnaeus, Reference Linnaeus1767 from the Adriatic Sea coast, but also without any direct evidence. However, this trace fossil is much shallow than Spirolites radwanskii n. igen. n. isp., displays three whorls, and no distinct annulation. Therefore, it represents a different ichnotaxon.
Santos et al. (Reference Santos, Mayoral, Muñiz, Vera-Peláez and Lozano Francisco2003) characterized bioerosive structures produced by Serpulidae (Serpula and Spirorbis) on Pliocene mollusk shells and echinoid tests from southern Spain. They are shallow, sinuous or spiral grooves, bounded a narrow zone, and show perpendicular ribbing. Their depth and width are much smaller than in Spirolites n. igen., which does not show any lateral zone. Because they are not photographically documented, further comparisons are difficult. Taylor and Vinn (Reference Taylor and Vinn2006, fig. 1) illustrated the spiral polychaete tube ‘Spirorbis’ incised in the substrate. Tubes determined as the serpulid Pomatoceros invoked by Radwański (Reference Radwański1969) can be deeply incised in the rock, as exemplified by specimens from the Oligocene of northern Germany illustrated by Polkowsky (Reference Polkowsky2010), if the determination is correct. These papers show that serpulid polychaetes are able to produce attachment scars and bioerode the substrate, but their traces are different than Spirolites n. igen. Some spiral tubes of the polychaete families Spionidae and Sabellidae (e.g., Protolaeospira strophostoma (Boettger, Reference Boettger1907) from the Miocene) that could produce etchings (Radwańska, Reference Radwańska1994) are similar in shape to Spirolites n. igen., but they are a magnitude smaller.
The morphology of Spirolites n. igen. also resembles that of some vermetid gastropods (see Bromley and Heinberg, Reference Bromley and Heinberg2006, fig. 13). Hadfield et al. (Reference Hadfield, Kay, Gillette and Lloyd1972) reported a few species of the recent Dendropoma from the Hawaiian Islands, the spiral shells of which were embedded in the substrate. Bromley (Reference Bromley1978) reported Dendropoma sp. from Bermuda, which produced grooves with a roof. Golding et al. (Reference Golding, Bieler, Rawlings and Collins2014) counted several species of Dendropoma that are coiled and deeply ‘entrenched’ in the substrate, similar to species of the related genera Novastoa, Ceraesignum, and Cupolaconcha. Dendropoma cristatum (Biondi Giunti, Reference Biondi Giunti1859) from the Mediterranean Sea produces shallow attachment scars and deeper traces, and its shell can be easily detached from the substrate (Rosso et al., Reference Rosso, Sanfilippo, Di Geronimo and Bonfiglio2016). Savazzi (1996, Reference Savazzi2001) described deeply endobenthic Dendropoma sp. from the Philippines, including those with spiral shells. The coiling direction of the shell looks to be sinistral (as in actual specimens of Spirolites n. igen.), but taking in account that evolutionally the shell developed down into the substrate, not above the substrate, it must be considered as dextral, as in the majority of vermetids (Savazzi, Reference Savazzi2001) and gastropods in general (Schilthuizen and Davison, Reference Schilthuizen and Davison2005). Some of the aforementioned Dendropoma sp. from the Philippines bore below the level of the substrate. Presumably, they bore chemically by marginal accretion of the shell. During initial development, the boring increases its depth and emerges from the substrate in the final phase. Vermetid gastropods in general bore in order to be better attached to the surface, which is weakened by physical and biological degradation, or the bioerosion is a way to obtain calcium carbonate for building of the shell (Savazzi, Reference Savazzi2001).
The last whorls of extant Dendropoma and related bioeroding species are distinctly smaller (6–28 mm across, see Golding et al. Reference Golding, Bieler, Rawlings and Collins2014, for taxonomy and characteristics of Dendropoma) than in the majority Spirolites n. igen. Nevertheless, vermetid gastropods similar to Dendropoma are more reliable candidates for the trace maker, because: (1) they can bore deeply into the substrate; (2) their shells, as for most of gastropods, show consistently dextral coiling (even if they appear as sinistral because of their development down into the substrate, similarly to Spirolites n. igen.); (3) shells of vermetids show second-order annuli, as in Spirolites n. igen., as opposed to tubes of serpulids that display first-order annulation; and (4) the gradual deepening into the rock, increase in size, and steep emerging between the whorls, with possible erosion of the preceding part of the shell, which is typical of vermetids (see Savazzi, Reference Savazzi2001). All these features can be observed in Spirolites n. igen.
Vermetid gastropods have been noted in Skotniki by Małecki (Reference Małecki1966) and Radwański (Reference Radwański1967, Reference Radwański1969, Reference Radwański1977), but they are small taxa, which either did not leave attachment scars, or, as Vermetus intortus (Lamarck, Reference Lamarck1818), produce the etching trace Renichnus arcuatus Mayoral, Reference Mayoral1987. None of them fits morphologically to Spirolites n. igen.
Living environment
The large boulders contain Spirolites n. igen. and the associated borings mainly on one side, presumably the upper surface. This suggests that they were not rotated. The co-occurrence of degraded and well-preserved Spirolites n. igen. suggests multiphase and long colonization by the vermetid trace makers. This style of colonization at Skotniki was proposed by Radwański (Reference Radwański1969). Smaller clasts, mostly cobbles and pebbles, are bored on all sides. The bored clasts were deposited in a cliff-foot ramp. According to Radwański (Reference Radwański1967, Reference Radwański1969) and Radwański and Górka (Reference Radwański and Górka2008, Reference Radwański and Górka2015), the clasts filling the depression were supplied from a cliff shore of a nearby island, but from its different parts, as evidenced by diverse composition and mechanical abrasion of the clasts. The clasts rolled by waves were bored from all sides, and those that were stabilized on the sea floor were bored only from the exposed sides. Those clasts that were quickly buried were not bored at all. Large numbers of bored clasts suggest good life conditions for the bioeroding organisms. Their borings were readily colonized by encrusters and cavity dwellers sheltering themselves from high wave energy (Radwański, Reference Radwański1977).
The limestones, which laterally replace and cover the clast-bearing facies, contain benthic macrofossils, mainly red algae and pectenids. These fossils and lithofacies features prove a lowering energy in a greater distance from the cliff.
The dominant boring associated with Spirolites radwanskii n. igen. n. isp. is Gastrochaenolites torpedo. This boring was referred to Lithophaga in papers by Radwański (Reference Radwański1969, Reference Radwański1977). The extant Mediterranean L. lithophaga (Linnaeus, Reference Linnaeus1758) is a chemical borer, which lives in very shallow (mostly from 0–6 m deep) and clean waters (Kleemann, 1973, 1974, Reference Kleemann1990; Galinou-Mitsoudi and Sinis, 1995, Reference Galinou-Mitsoudi and Sinis1997), commonly on steep or overhanging surfaces (Bromley and Asgaard, Reference Bromley and Asgaard1993a). This suggests that Spirolites n. igen. also originated in very shallow, clean waters, in accordance with previous interpretations (Radwański, Reference Radwański1969). The boring assemblage (Gastrochaenolites, Caulostrepsis, and Entobia) represents a mature stage of bioerosion that developed over the years and can be referred to the Entobia ichnofacies (Bromley and Asgaard, Reference Bromley and Asgaard1993b), which is typical of Mesozoic–Cenozoic rocky shores (Gibert et al., Reference Gibert, de, Martinell and Domènech1998).
Generally, vermetid gastropods live in very shallow and warm waters. The bioeroding Dendropoma sp. from Philippines lives at a depth of 0–1 m (Savazzi, Reference Savazzi2001), and one from Bermuda does not inhabit waters deeper than 4 m (Bromley, Reference Bromley1978). Most species of this genus and related genera live in intertidal to shallow subtidal waters (Schiaparelli et al., Reference Schiaparelli, Albertelli and Cattaneo-Vietti2006). Nevertheless, the vermetid trace Renichnus arcuatus, at a depth of >60 m, also has been noted in the Azores (Wisshak et al., 2011, Reference Wisshak, Berning, Jakobsen and Freiwald2015). In addition, Dendropoma mejillonensis Pacheco and Laudien, Reference Pacheco and Laudien2008 from Chile, which leaves attachment scars, was reported from a submarine wall at a depth of 17 m (Pacheco and Laudien, Reference Pacheco and Laudien2008). Irrespective of the depth, vermetid gastropods require water turbulence for production of the mucus nets for feeding or filter feeding (Schiaparelli and Cattaneo-Vietti, Reference Schiaparelli and Cattaneo-Vietti1999; Schiaparelli et al., Reference Schiaparelli, Albertelli and Cattaneo-Vietti2006). The data on associated borings and bathymetric range of vermetid gastropods suggest that Spirolites n. igen. was produced in very shallow, turbulent waters.
Recent vermetids live in tropical and temperate marine waters between 44°N and 44°S, where they can form small reefs (Safriel, Reference Safriel1975; Schiaparelli and Cattaneo-Vietti, Reference Schiaparelli and Cattaneo-Vietti1999; Silenzi et al., Reference Silenzi, Antonioli and Chemelloc2004; Schiaparelli et al., Reference Schiaparelli, Albertelli and Cattaneo-Vietti2006). Generally, the period between 17 Ma and 15 Ma (Pińczów Formation, which can be related to the late phase of this because the overlying Miocene series is dated to NN5–NN6; see Radwański and Górka, Reference Radwański and Górka2008) is referred to the Middle Miocene Climatic Optimum, which is characterized by global warmth, high content of CO2 in the atmosphere, and a significant decrease in the Antarctic icecap (Foster et al., Reference Foster, Lear and Rae2012). As evidenced by data on echinoids, the temperature rose up to tropical levels (sea surface temperatures 16–17°C during winter and up 28°C during summer) from the Karpatian (late Burdigalian) stage to the early Badenian optimum for the Central Paratethys, but the Carpathian Foredeep remained in the temperate climate zone (Kroh, Reference Kroh2007). However, data on facies from the eastern part of the Carpathian Foredeep (Stebnyk Formation, Ukraine) suggest that the increase in temperature also touched this region (Oszczypko et al., Reference Oszczypko, Uchman and Bubniak2016). Only local, colder waters related to an upwelling are interpreted for the Paratethys sea at that time (Key et al., Reference Key, Zágoršek and Patterson2013). According to Bałuk and Radwański (Reference Bałuk and Radwański1977), the slightly younger biota of the nearby Korytnica Clay (NN6) suggests tropical or subtropical environment. However, common occurrences of echinoids and large foraminifera in the lower Badenian deposits that purportedly might suggest thermal conditions typical of subtropical or tropical climate are questioned by some authors. Studencki (Reference Studencki1999) and Randazzo et al. (Reference Randazzo, Müller, Lelkes, Juhasz and Hamor1999) suggested that paleo-temperature in the Paratethys basin during that time was rather typical of moderate climatic zones, on the border with the subtropical zone. This is suggested by poorly developed coral reefs, scarcity of green algae, and inorganic calcareous grains, especially ooids. According to Studencki (Reference Studencki1999) and Randazzo et al. (Reference Randazzo, Müller, Lelkes, Juhasz and Hamor1999), red algae, bryozoans, and mollusks that are very common in the lower Badenian deposits are typical of the rhodalgal biolithofacies, which occurs in Recent moderate climatic zones (e.g., near the coast of Scotland). Studencki (Reference Studencki1999) postulated that the strong expansion of fauna known from the Korytnica Clay and the Heterostegina Sand, including large mollusks and large foraminifera, was a local effect of peculiar topographic conditions during the climatic optimum. The latitude of Skotniki (recently N50°25') during sedimentation of the gravelstone might be lower by 10° or more (e.g., Vrielynck et al., Reference Vrielynck, Odin and Dercourt1997). This, in coincidence with the Middle Miocene Climatic Optimum, suggests that the temperature of waters in the Paratethys Sea on the northern margin of the Carpathian Foredeep was higher at that time than the Atlantic waters in the same latitudes at present. Such waters were inhabited by vermetid gastropods, the shells of which are preserved and have small traces of Renichnus and the larger trace Spirolites n. igen.
Conclusions
Spirolites radwanskii is a new ichnogenus and ichnospecies of a spiral bioerosion trace in a Miocene rocky (limestone) substrate. Its morphological features (initial dipping into the rock, increasing in size, steep emerging from the substrate, second-order annuli, direction of coiling, erosion of the former part) point to vermetid gastropods as the trace makers, resembling the recent Dendropoma. The trace fossil occurs within the Entobia ichnofacies and was produced in very shallow, warm, and clean waters in the Miocene rocky shore of the Paratethys Sea of the Carpathian Foredeep.
Acknowledgments
Jagiellonian University (DSC funds, no DS/MND/WbiNoZ/ING/2/2013) supported the studies by M.S. on the Miocene rocks in the Carpathian Foredeep; (DS funds K/ZDS/005424 for A.U.). A previous version of the paper benefitted from critical reviews of M. Wisshak and M. Wilson.