Introduction
The Himalayas are spread over an area of 2500 km from west to east. There are 19 rivers flowing from the Himalayas that are very rich in biodiversity; the eastern Himalayas have a greater diversity of coldwater fish species than the western region. There are 218 fish species of the Cyprinidae and Salmonidae family reported and listed from the Himalayan range, a few examples are Labeo dero, Tor putitora, Barilius bendelisis, Schizothorax: S. richardsonii, S. esocinus, S. progastus, S. longipinnis, S. planifrons, Crossocheilus diplochilus, Garra gotyla, G. lamta, G. lamp, G. kalpangi, G. chakpiensis and G. nkhruletisis. The genus Garra consists of about 134 species distributed in the coldwater and fresh water of Asia and Africa (Fishbase, 2018). A few species of genus Garra are G. gotyla (Gray 1830), G. stenorhynchus (Jerdon, Reference Jerdon1849), G. annandalei (Hora, Reference Hora1921), G. lamta (Hamilton, Reference Hamilton1822) G. ruffa (Heckel, Reference Heckel1884), G. barreimiae (Fowler and Steinitz, Reference Fowler and Steinitz1956). Four species, G. tana, G. regressus, G. dembecha and G. dembeenis, are found in Lake Tana in Ethiopia among which two species, G. tana and G. regressus, are endemic to the lake (Stiassny and Getahun, Reference Stiassny and Getahun2007; Getahun, Reference Getahun2010a, Reference Getahun2010b; Darwall et al., Reference Darwall, Smith, Allen, Holland, Harrison and Brooks2011). Geremew et al. (Reference Geremew, Getahun and Dejen2015) studied the reproductive biology of G. tana and G. regressus and found that the mean sizes of mature ova were 1.22 mm and 1.81 mm in diameter and mean fecundity values were 1060 and 1402, respectively. Moreover, they also reported that females matured earlier than males. Masoud et al. (Reference Masoud, Shiva, Mohammadi and Malekpour2011) reported that the maximum population of G. rufa belonged to the 2–3 years age group and had a narrow range of 0–4 years of growth. The absolute and relative fecundity of the G. rufa was in the range 1179 and 109.4 respectively and mean egg size of fish was 0.67 mm in diameter. G. barreinmiae is the only species of the genus known for its troglomorphic character and the only known population lives in the Al-Huda cave of Oman of the southeastern Arabian Peninsula (Kruckenhauser et al., Reference Kruckenhauser, Haring, Seemann and Sattmann2011). G. mullya, a fish in this genus, is widespread and endemic to peninsular India particularly the southwestern Ghats of Tamil Nadu, India (Sabaridasan et al., Reference Sabaridasan, Edwinthangam and Soranam2017). Termvidchakorn et al. (Reference Termvidchakorn, Suksri and Magtoon2016) studied developmental morphology, which included chromophore pigmentation, number of myomeres, dorsal and anal fin rays at four stages of development (yolk sac, larval, post-larval and juvenile) for identification in three species of genus Garra found in Thailand (G. cambodgiensis (Tirant, 1984), G. fulliginosa (Fowler, 1937) and G. notate (Blyth, Reference Blyth1860)). They found that early melanophore pigmentation pattern throughout the body surface and numbers of myomeres are important for the earliest identification of the species. Garra gotyla is widespread in south Asia, which includes Pakistan, Bangladesh, Myanmar (Talwar and Jhingran, Reference Talwar and Jhingran1991), Afghanistan (Coad, Reference Coad1981), Bhutan (Mitrofano and Petr, Reference Mitrofano, Petr and Petr1999) and Nepal (Jha et al., Reference Jha, Waidbacher, Sharma and Straif2005). The fish is commonly known as a Sucker head, is a bottom dweller and feeds on algae, plants and detritus. Its habitat is a variety of freshwater and coldwater sources such as lakes, ponds, rivers and is very adapted to extreme environmental conditions. Jha et al. (Reference Jha, Waidbacher, Sharma and Straif2005) reported that a large percentage of the population of Nepal depends on G. gotyla as a potential source of protein. The body morphometric examination showed that the body is elongated, sub-cylindrical, and the mouth is arched and inferior, has a suctorial disc on the chin, with a snout with a well developed median proboscis and transverse lobes at tips. The aim of the present study was to understand embryonic development from the onset of fertilization to the fry stage to provide a blueprint for mass production of seed for aquaculture and conservation management.
Materials and methods
Fish collection and experimentation
The induced breeding of G. gotyla was carried out for the first time in a flow-through hatchery system maintained by the Division of Fish Genetics and Breeding, ICAR, Directorate of Coldwater Fisheries Research, Uttarakhand, India. Experimental fish were captured from rivers and lakes of Uttrakhand, India, and had been maintained in the germplasm repository of ICAR-DCFR, Bhimtal since 2016 (Fig. 1). The fish is a summer spawner and development of prominent tubercles on the snout of males and bulging of the abdomen in females is an important morpho-indicative feature of maturity. Ten females of mean body weight 35 g and 14 cm in length and three males of mean body weight 52.22 g and 18 cm in length were used for induced breeding performance. The synthetic hormone Ovatide, which is an analogue of GnRH and dopamine antagonist, was administered sub-dermally in the caudal peduncle region above the lateral line to trigger breeding using a 2 ml hypodermis syringe with a 0.1 ml graduation and a no. 22 needle (Jhingran, Reference Jhingran1991). Mating behaviour of the fish was also recorded and these tended to stay in groups preferably in the low light intensity zone in the breeding pool. Spawning of fish was allowed in breeding pools and fertilized eggs were collected using a mesh cloth and were gently poured into hatching trays.
Light microscopy-based photomicrography
Fertilized eggs were placed in Petri dishes to study embryo developmental stages and these were observed, monitored, photographed and documented at each stage of development. A light microscope (Olympus CKX53, Tokyo, Japan) with a mounted camera and assisted by a computer (Magvision software) was used to capture and record chronologically developmental stages such as fertilization of eggs, blastulation, gastrulation, neurulation, sac fry, swim fry and adult fry. Developmental progress was also verified in eggs placed in hatching trays at each ongoing stage. After yolk absorption, the swim-up larvae were fed on egg albumin and phytoplankton. The chronological study of embryonic development in the present study within the time frame is a very important aspect of reproductive biology for oocyte-based manipulation in future studies. The complete overview of major changes observed at each stage of development is given in Table 1.
Table 1. Embryonic and larval development with major changes observed during the time frame in G. gotyla

Results and discussion
Response of fish to Ovatide
Time taken for the release of mature oocytes from females and sperm (Milt) from the males after the injection of synthetic hormone (Ovatide) was recorded as 8 h. The morpho-indicative features of unfertilized oocytes are whitish in colour, non-sticky and opaque (Fig. 2A). Fertilization rate was 70% and the fertilized egg was measured as 0.8–1.0 mm in diameter, whereas Abedi et al. (Reference Abedi, Shiva, Mohammad and Malekpour2011) reported oocyte size as 0.7 mm in G. ruffa; Geremew et al. (Reference Geremew, Getahun and Dejen2015) reported it as 1.22 mm in G. tana and 1.81 mm in G. regresscus, and others as 0.89 mm in G. macrocephala (Ko et al., Reference Ko, Kim, Park and Bang2011a). The fertilized oocyte size was 0.7 mm in Danio rerio (Kimmel et al., Reference Kimmel, Ballard, Kimmel, Ullmann and Schilling1995); C. gariepinus oocyte size was 1–1.2 mm (De Graaf and Janssen, Reference De Graaf and Janssen1996), and 1.80 mm in M. koreensis and R. oxycephalus (Han et al., Reference Han, Noh, Oh, Park, Cho and Seong1999; Kim et al., Reference Kim, Yoon, Kim, Kim, Park and Park2012). Oncorhynchus mykiss oocytes are deep orange in colour, non-sticky and 3.36–5.56 mm in diameter (Springate and Bromage, Reference Springate and Bromage1985). The difference in size is highly species specific and also depends on maturity, age of brooder and fecundity.
Embryonic stages
Embryonic staging and developmental changes in the present study were evaluated based on published reports of different fish species (Kimmel and Law, Reference Kimmel and Law1985; Olaniyi and Omitogun, Reference Olaniyi and Omitogun2013). The oocytes soon after fertilization underwent mitotic division and the yolk content of the oocyte started to migrate towards the centre; the perivitelline space became apparent. The perivitelline membrane became thick progressively, an animal pole that is also known as a germ pole consisted of pigmented granules (Kimmel et al., Reference Kimmel, Ballard, Kimmel, Ullmann and Schilling1995). These pigmented granules were the nucleus and cytoplasm of the dividing cells of the embryo, while the vegetal pole which is the vitelline part was comprised of yolk and nourished dividing cells of the embryo (Fig. 2B). The animal pole was in a continuous state of division and became intensely pigmented, devoid of yolky cytoplasm and bulged outwards to enter the next stage of development, whereas the vegetal pole became transparent and fully translucent. Mitotic division was discoidal and meroblastic, a characteristic feature of the telolecithal egg (Kimmel and Law, Reference Kimmel and Law1985; Hall et al., Reference Hall, Smith and Johnston2004; Buzollo et al., Reference Buzollo, Veríssimo-Silveira, Oliveira-Almeida, Alexandre, Okuda and Ninhaus-Silveira2011).
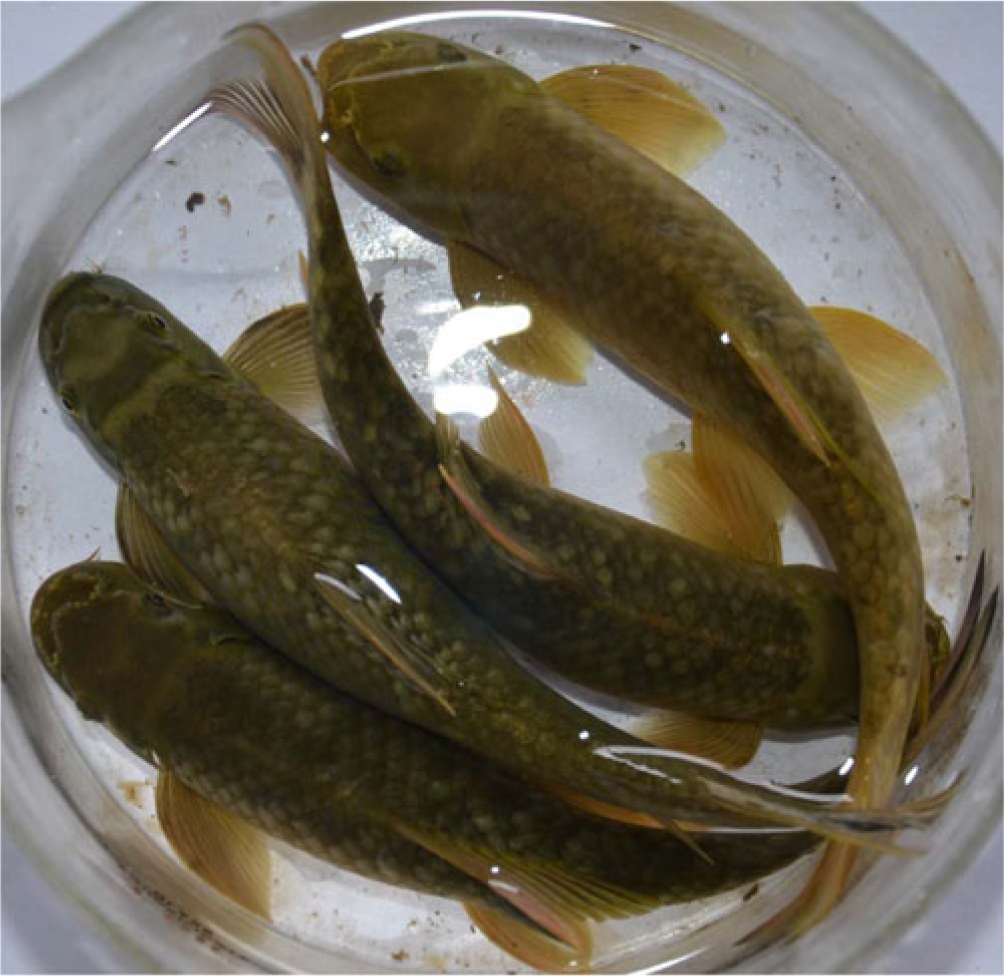
Figure 1. Brooders of Garra gotyla.

Figure 2. Embryo during the cleavage stage. (A) Unfertilized egg, (B) fertilized egg, (C) 4-cell stage lateral view, (D) 8-cell stage after 67 min pf, (E) 16-cell stage after 80 min, (F) 32-cell stage after 83 min, (G) 64-cell stage (morula) after 111 min pf. Abbreviations: pf: post fertilization, EVL: enveloping layer.
Two-cell stage (32 min pf): The cleavage furrow was vertical and a similar pattern was observed up to the 32-cell stage. The furrow was directed from the animal pole towards the vegetal pole through the blastodisc but not through the yolk cell. The dividing cells were equal in size and further cleavage was oriented by the first one. The time taken for completion of two-cell stage was 38 min recorded after the fertilization.
Four-cell stage (55 min pf): The two cells formed in the first cleavage underwent further mitotic division at a right angle to the plane of the first division. Hence, four cells in 2 × 2 arrays were formed. The four cells formed at the animal pole were similar to fingertips (Fig. 2C).
Eight-cell stage (67 min pf): Cell division was mitotic resulting in the formation of eight cells in a 4 × 2 array; the pattern of cleavage was similar to that observed in the first cell stage. The four blastomeres facing towards the eyepiece mask the blastomeres positioned behind (Fig. 2D).
Sixteen-cell stage (80 min pf): The fertilized ova progressively proceeded for the completion of the fifth stage. Mitotic cell division was vertical to the first one and established two new cleavage furrows with 16 blastomeres in a 4 × 4 array (Fig. 2E). The cleavage furrow cut the blastodisc centrally and did not reach the margins. The four centrally located blastomeres were partially separated and established cytoplasmic connections in a similar pattern as reported by Buzollo et al. (Reference Buzollo, Veríssimo-Silveira, Oliveira-Almeida, Alexandre, Okuda and Ninhaus-Silveira2011) and Olaniyi and Omitogun (Reference Olaniyi and Omitogun2013).
Thirty-two-cell stage (83 min pf): The blastomeres formed after this stage of mitotic division were present in 4 × 8 arrays. Microscopic observation of the cell at the animal pole resembled masking of the moon by clouds and therefore it was very difficult to count cells individually at this stage. Cleavage occurred in four parallel planes rather than two, and two tiers of cells could be easily seen at the animal pole; this stage is known as the early morula stage (Fig. 2F).
Sixty-four-cell stage (111 ‘min’ pf): This is the seventh stage of cleavage and blastomeres and similar to the 32-cell stage; the difference was in reduced cell size compared with the previous cell stage. The few blastomeres completely mask others seen at the 32-cell stage in the topmost tiers of cells at the animal pole, now known as the enveloping layer (EVL) of the blastodisc. In the former stage two layers of EVL could be seen, whereas at the 64-cell stage three tiers of EVL were visible (Fig. 2G).
Blastula stage
Formation of morula and completion of the cleavage stage resulted in the beginning of the blastula stage. The blastoderm was a highly compacted structure on top of the yolk sac and formed by dividing blastomeres. Significant changes observed at this stage were mid blastula transition (MBT), formation of the yolk syncytial layer (YSL) and onset of gastrulation period (30% epiboly). The blastocoel is a small irregular extracellular space between deeply coherent cells of the blastodisc (Fig. 3). Mitotic cell divisions are indeterminate and irregular compared with the earlier stage of the embryo. Cleavage was meta-synchronous because all cells do not enter mitosis at the same time, Similar observations were reported by Kimmel et al. (Reference Kimmel, Ballard, Kimmel, Ullmann and Schilling1995) in zebrafish and in cichlid fish species (Kratochwil et al., Reference Kratochwil, Maggie and Meyer2015). Cells near the animal pole entered cell cycle first and marginal cells entered last. Marginal blastomeres have a predetermined different fate as they remain connected to the yolk cells by cytoplasm throughout the blastula stage. The marginal blastomeres underwent further structural changes like collapsing of blastomeres, and fusion of cytoplasm and nuclei with adjacent yolk cells resulting in the formation of the YSL (Kimmel et al., Reference Kimmel, Ballard, Kimmel, Ullmann and Schilling1995; Honji et al., Reference Honji, Tolussi, Mello, Caneppele and Moreira2012; Olaniyi and Omitogun, Reference Olaniyi and Omitogun2013). Those blastomeres present in the penultimate layers are marginal in position and do not establish cytoplasmic connections, therefore are referred to as non-syncytial. The YSL divides mitotically in the mid blastula stage in such a way that the cytoplasmic stage does not follow nuclear division and the uncleaved yolk cell remains syncytial. This cell division pattern resulted in the onset of epiboly and will continue into the gastrulation stage, a similar observation was reported in zebrafish and African catfish (Kimmel et al., Reference Kimmel, Ballard, Kimmel, Ullmann and Schilling1995; Olaniyi and Omitogun, Reference Olaniyi and Omitogun2014). The YSL forms a thin ring around the edge of the blastodisc and after few divisions spread underneath (Fig. 3G), which resulted in the formation of the complete internal syncytium. The epiboly started at the end of the blastula and before the onset of gastrulation, therefore ‘stereoblastula’ is the appropriate term to describe this stage (Kimmel and Law, Reference Kimmel and Law1985; Kimmel et al., Reference Kimmel, Ballard, Kimmel, Ullmann and Schilling1995; Olaniyi and Omitogun, Reference Olaniyi and Omitogun2014). Thinning and spreading of the YSL and blastodisc during the early onset of the stage is a characteristic morphogenetic feature of epiboly (30% epiboly). The high blastula (Mulberry) in which dividing blastomeres are highly structured as seen from the early blastula towards the end of mid blastula (Fig. 2A–C) and these blastomeres are collectively termed as the ‘enveloping layer’ (EVL) (Kimmel and Law, Reference Kimmel and Law1985; Olaniyi and Omitogun, Reference Olaniyi and Omitogun2014). Transition from high blastula to low is characterized by compression and flattening of blastodermal cells in the EVL (Fig. 2E–G). The yolk cell present underneath becomes more spherical than in the previous stages and a similar event has been reported in zebrafish (Kimmel et al., Reference Kimmel, Ballard, Kimmel, Ullmann and Schilling1995).

Figure 3. Embryo during blastulation stage. (A) 256-cell stage, (B) 512-cell stage, (C) 1 K-cell stage, (D) oblong cell stage, (E) sphere stage 240 min, (F) dome-shaped initial epiboly after 255 min pf, (G) 30% epiboly after 280 min after pf, (H) cell at ×40 magnification of 30% epiboly, (H1) yolky cells, (I) high compacted cell shapes at ×40 magnification. Abbreviations: bd: blastoderm, gr: germ ring.
256-cell stage (150 min pf): Seven irregular tiers of blastomeres are formed up to completion of the eighth stage. Thinning of EVL cells is maximum in cells buried deeper than presented outward and cleavage is highly synchronous (face view: Fig. 3A).
512-cell stage (165 min pf): This stage marked the onset of mid blastula and the period for completion of each cell cycle progressively increased. In the face view of the embryo, eight to nine tiers of blastomeres of the EVL are visualized between animal pole and margins. During this stage, blastomeres are compressed and marginal ones will establish a cytoplasmic bridge with the yolk cell resulting in the formation of the YSL (Fig. 3B).
1 K-cell stage (180 min pf): This stage consists of approximately 1024 blastomeres; those blastomeres present in the second tier in the previous stage are taken as the first tier. The EVL is comprised of 11 layers of blastomeres after this stage (Fig. 3C).
High blastula (195 min pf): This stage was the last stage in which stacking of blastomeres occurred on the yolk cell and is a structural feature of EVL (Fig. 3D). Construction in the form of the pinched ring was seen in which the marginal cell layer of the EVL meets the YSL. The distinguishing feature of this stage is the appearance of blastodisc cells and a number of nuclei (Kimmel et al., Reference Kimmel, Ballard, Kimmel, Ullmann and Schilling1995). Most blastodisc cells are found at the interface of the 12th cell cycle and only a few have completed the 13th cell stage.
Oblong stage (210 min pf): Shortening of the animal–vegetal axis of the embryo and the ellipsoidal shape of the yolk cell is formed due to exertion of uniform compression of overlying blastodisc and the ellipsoidal shape of blastula in due course, hence named the oblong stage (Fig. 3E).
Sphere stage (240 min pf): Further compression of blastodisc and shortening of the animal–vegetal axis resulted in the spherical shape of the embryo (Fig. 3F). The spherical shape remains constant until the late gastrula stage and cell rearrangement is very rapid at this stage. Towards completion of this stage, the appearance of the slight notch between blastodisc and yolk cell is the characteristic feature of this stage (Kimmel et al. Reference Kimmel, Ballard, Kimmel, Ullmann and Schilling1995).
Dome stage (255 min pf): Overall shape remained the same only at the interface between the blastodisc and the yolk cell protruded towards the overlying blastodisc. The EVL cells spread more at the margins to cover the yolk cells and resembled a dome (Fig. 3G). Most cells were in the late 13th cell cycle.
30% epiboly (280 min pf): Formation of the blastoderm occurs in this stage and overcompression of blastomeres resulted in the dome-like or slight spherical structure of yolk cells (Fig. 3H). EVL cells are highly flattened due to the spread of the blastoderm and the extant of spread over and across the yolk cell are important for demarcating different stages of epiboly. Spread of blastoderm over the yolk cell is commonly referred to in percentiles (Fig. 3H1). The 30% epiboly occurs when the blastoderm covers the yolk cell from animal to vegetal axis by a 30th fraction (Fig. 3I).
Gastrulation
Slow morphogenetic peristaltic movement of perivitelline fluid and expansion of the blastoderm was seen in the developing embryo. When epiboly was at its halfway mark (Fig. 4A) a definite germ ring-like structure was noticed around the blastoderm rim. This process is known as involution and marked the onset of gastrulation (Kimmel and Law, Reference Kimmel and Law1985; Kimmel et al., Reference Kimmel, Ballard, Kimmel, Ullmann and Schilling1995; Trinkaus, Reference Trinkaus1996; Rhode and Heisenberg, Reference Rhode and Heisenberg2007; Olaniyi and Omitogun, Reference Olaniyi and Omitogun2014). The movement of the blastoderm is a morpho-indicative feature of the germ ring and embryonic axis formation (Fig. 4). The germ ring consists of two types of cell layer, one is the epiblast and the other is the hypoblast. The mechanism of hypoblast formation is not fully understood, but it is thought that involution of superficial cells at the margins followed by movement towards the animal pole might be the reason (Trinkaus, Reference Trinkaus1996; Montero et al., Reference Montero, Carvalho, Brauninger, Kilian, Mustafa and Heisenberg2004). Brachet’s cleft is one of the critical structures formed when the embryo is in 70% epiboly and this structure does not allow mixing of the epiblast with the hypoblast, as movement of cells are redirected in the opposite direction. The event of convergence begins just after completion of involution (Kimmel et al. Reference Kimmel, Ballard, Kimmel, Ullmann and Schilling1995). The accumulation of cells at one point of the germ ring is known as convergence and the structure so formed is called the embryonic shield (Kimmel et al., Reference Kimmel, Ballard, Kimmel, Ullmann and Schilling1995; Trinkaus, Reference Trinkaus1996; Montero et al., Reference Montero, Carvalho, Brauninger, Kilian, Mustafa and Heisenberg2004). A brief arrest of epiboly occurred at this stage until completion of the shield after movement of the blastoderm continues to wrap the yolk cell completely. Epiblast cell movement is towards margins and once reached at the margins started streaming inwards into the hypoblast. At the end of the gastrulation cell present in the epiblast is ectoderm nominated and differentiates for the formation of the epidermis, central nervous system, neural crest and sensory placode (Kimmel et al., Reference Kimmel, Ballard, Kimmel, Ullmann and Schilling1995; Rhode and Heisenberg, Reference Rhode and Heisenberg2007). The organs with the fate map from the endodermis are derived from the hypoblast. The embryonic shield was discernible by the thickness of the chorionic perivitelline capsule (Fig. 4C); the normal cellular inward movement continued until the closure of blastopore and marked the completion of the gastrulation stage (Kimmel et al., Reference Kimmel, Ballard, Kimmel, Ullmann and Schilling1995). The hypoblast around the embryo is either one cell or two cells thick when the face was viewed under the microscope. As in higher vertebrates, three completely demarcated germ rings were not seen in this study. When the shield extended towards the animal pole (Fig. 4E), the blastoderm was thicker on the dorsal side than ventrally due to the presence of the axial hypoblast dorsally. Muscles that support movement of the eyes, gills and jaws are derivatives of the anterior para-axial hypoblast. Those para-axial hypoblasts positioned posterior were designated for the formation of muscle precursors (somites). Protrusion of yolk cells beyond the blastoderm is known as the yolk plug (Fig. 4E, F) and rudimentary tail buds were seen prior to completion of the gastrula stage (Fig. 4F).

Figure 4. Embryo in gastrulation stages. (A) 50 % epiboly after 315 min after pf, (B) germ ring after 340 min after pf, (C) shield 360 mint pf, (D) 75% epiboly 480 min after pf, (E) 90% epiboly after 540 min after pf, (F) initial bud stage after 590 min after pf, evacuation zone on ventral side. Es: embryonic shield (blue arrows), pf: post fertilization, pcp: prechordal plate or anterior axial hypoblast, ez: evacuation zone on ventral side (short blue arrow). Amd: axial mesoderm, pamd: para-axial mesoderm.
50% epiboly (315 min pf): The end of 30% epiboly marked the onset of 50% epiboly in which the blastoderm covered half of the yolk cell. The presence of a thick blastoderm at and along the margins is the characteristic feature of this stage. Radial formation of the primitive streak (an equivalent to the blastopore) is crucial for uniform thickness of the blastoderm and for germ ring formation (Fig. 4A).
Germ ring stage (340 min pf): Germ ring formation was seen as a uniform thickened annulus-like structure at margins of the blastoderm when the face was viewed through the animal pole (Fig. 4B). The germ ring consisted of two layers of the blastoderm in addition to the EVL. The uppermost of the germ ring is known as epiblast and is a three cell thick structure. The hypoblast lies beneath the epiblast, very close to the YSL.
Shield stage (360 min pf): The germ ring and embryonic shield were viewed through the animal pole under a dissecting microscope (Fig. 4C, D). Although the developing embryo was still in 50% epiboly and remained until the blastoderm covered the yolk cell up to more than the halfway mark. When the epiblast and hypoblast thickened at the shield it was then considered as the dorsal side of the embryo and organs designated from the dorsal part will develop.
75% epiboly stage (480 min): Based on the area of the yolk cell covered by the blastoderm differentiates epiboly on a percentile basis (Fig. 4D). The shield observed in the previous stage is no longer distinct due to repacking of cells for elongation of the blastoderm along the animal pole. The blastoderm is thinner on the ventral side than anywhere else and this region is termed as an evacuation zone, further elongation along the anterior–posterior axis resulted in the formation of the prechordal plate (Fig. 4D).
90% epiboly and initial bud stage (540 and 580 min pf): At the vegetal pole, only a small part of the protruding yolk cell was seen and known as the yolk plug (Fig. 4E); the complete yolk plug was seen when the cells left at the ventral region were very thin (Fig. 4F). Thickening of the dorsal epiblast differentiated into the neural plate, a site of the future brain. The prechordal plate extended further to the animal pole towards the closing stage of 90% epiboly. The spread of the blastoderm to cover the yolk cell due to the convergence and extension processes was faster at the dorsal side than on the ventral side of the developing oocyte. The disappearance of the yolk plug, although considered to be at the vegetal pole, was actually slightly ventral to the yolk cell (Kimmel et al., Reference Kimmel, Ballard, Kimmel, Ullmann and Schilling1995). The distinct swelling that appeared at the posterior or caudal end of the embryonic axis is known as the tail bud. The neural plate formation anterior to the tail bud, increased in cell mass all along the embryonic axis. The shallow midsagittal groove underlies the forebrain. The pollster, a hatching rudiment, will develop deep to the anteriormost part of the neural plate (Fig. 4F).
Neurulation and hatching
Formation of yolk plug is completion of the gastrulation stage (Buzollo et al., Reference Buzollo, Veríssimo-Silveira, Oliveira-Almeida, Alexandre, Okuda and Ninhaus-Silveira2011), however studies have reported movement in the interior cells of the hypoblast and the epiblast in gastrulation up to the bud stage (Kimmel et al. Reference Kimmel, Ballard, Kimmel, Ullmann and Schilling1995). The most significant changes such as tissue level differentiation or organ level differentiation is of a similar pattern as reported in other bony fishes. Prominent changes observed were complete differentiation of tail bud and polster (Fig. 5A–C, arrowhead), formation of somites in number and pattern (Fig. 5H–K), elongation of embryo along the anterior and posterior axes (Fig. 5K), dorsal and ventral axes and first movement in the ova. Olaniyi and Omitogun (Reference Olaniyi and Omitogun2014) reported similar observations in the embryonic development of Heterobranchus bidorsalis. Morphometric changes in the tail and head became more prominent after each passing stage. Thickness developed at the posterior and caudal ends of the embryonic axes were the tail bud and polster. The characteristic feature of the neurula stage is partition of the ectoderm into four distinct domains: neural plate, neural crest, pre-placodal region (PPR) and future epidermis; Kratochwil et al. (Reference Kratochwil, Maggie and Meyer2015) reported similar development events in the head part of the Medas cichlid fish species. The thickness of the neural plate along the embryonic axis although developing dorsally was anterior to the tail bud, whereas the spinal cord trunk developed from posterior cells (Fig. 5D). Most interesting observations in the development of the embryo started after the formation of the bud and polster. The PPR underwent further cellular differentiation into both sensory and ganglionic cranial placodes (Kimmel et al. Reference Kimmel, Ballard, Kimmel, Ullmann and Schilling1995). As the optic primordia (Fig. 5H) evaginated from the diencephalon after the five-somite stage (Fig. 5F) and approached the surface ectoderm at the 11 somite stage, the prospective lens ectoderm developed from the overlying cells of the optic vesicle and further to lens placode through the columnar shape of cells (Fig. 5M; arrow in Fig. 5M1) at the 21 somite stage (Fig. 5K–M). The neural plate formation was seen as a thickened structure due to assembly of the dividing cell along the embryonic axis. The rod-like structure so formed is a notochord at the dorsal part of the developing embryo (Fig. 5J). The dorsally developed embryo supported the vertebral column and a distinct head region and tail bud were seen. The first appearance of the otic vesicle (inner ear) in the head part and Kupffer’s vesicle in the tail bud was seen at the 17 somite stage (Fig. 5J). At this stage, it was possible to differentiate the cephalic and caudal regions. The otic vesicle is an invagination of the otic placode and originates from the head ectoderm during somatogenesis (Kimmel et al., Reference Kimmel, Ballard, Kimmel, Ullmann and Schilling1995). The otic vesicle is a primordium of the cochleovestibular ganglion and the inner ear; morphogenesis and neurogenesis of the otic vesicle results in hindbrains were directed via non-autonomous cell signalling (Freyer et al., Reference Freyer, Aggarwal and Morrow2011). The otolith present in the otic vesicle is formed due to biomineralization of calcium carbonate and is highly conserved in mammals and fishes (Hughes et al., Reference Hughes, Blasiole, Huss, Warchol, Rath, Hurle, Ignatova, Dickman, Thalmann and Levenson2004; Lundberg et al., Reference Lundberg, Zhao and Yamoah2014). Kupffer’s vesicle formation is transitory and disappears after the true intestines are formed in all teleost embryos (Kimmel et al., Reference Kimmel, Ballard, Kimmel, Ullmann and Schilling1995; Buzollo et al., Reference Buzollo, Veríssimo-Silveira, Oliveira-Almeida, Alexandre, Okuda and Ninhaus-Silveira2011; Olaniyi and Omitogun, Reference Olaniyi and Omitogun2014). Gao et al. (Reference Gao, Xu, Guan, Liu and Han2011) reported Kupffer’s vesicle formation under the control of the Wdr18 gene in zebrafish. First embryo movement within the chorion was seen after the 11 somite stage (Fig. 5I). The head part further underwent distinct differentiation into the hindbrain, midbrain and cephalon; the heart was visualized just underneath the cephalon (Fig. 5L) at the 26th somite stage. The completely developed muscle myofibrils were seen in an array (>) and the the arrow pointed towards the cephalic region (Fig. 5L1). The end of this stage occurred when the tail fully detached from the yolk mass, growth of somites and well developed optic vesicles. The enclosed embryo within the chorion made vigorous movements to break the chorion, now soft and thinned, that helps the hatchling. The sac fry were 3 mm in length and the sac pouch was 1.8 mm in length.

Figure 5. Development during neurulation and segmentation. (A) 90% completion of bud stage, (B) polster buds, (C) fully visualization of polester head and tail, (D) two somite stage anterior view, (E) two somite stage ventral view, (F) five-somite stage, (G) 11 somite stage, (H) optic primordia and Kupffer’s vesicle, (I) visualization of first time embryo movement within the chorion, (J) visualization of otic vesicle, lens placode and Kupffer’s vesicle, (K) 21 somite stage, (K1) size of yolksac 21 somite stage, (L) vizualization of different parts of head at the embryonic stage, (L1) muscle myofibrils, (L2) otolith of otic vesicle, (L3) optic primordial, (M) complete differentiation of head part and appearance of lens in optic primordia, (M1) appearance of lens, (M2) appearance of otolith. yp: yolk plug, tez: thin evacuation zone, tb: tailbud, p: polster, dp: distinct polester. s2: two somites, s5: five somites s11: eleven somites, op: optic primordia, KV: Kupffer’s vesicle, OV: otic vesicle, OT: otolith, fb: forebrain, mb: midbrain, hb: hindbrain, c: cephalon, dtb: distinct tail bud, L: lens, mm: muscle myofibrils.
Post embryonic development
This period of development commenced instantly when the embryo (sac fry) hatched out from the chorion and was broadly classified into three stages namely sac fry, spawn and fry. The post-hatching period started once the embryo had broken out of the chorion due to vigorous movement. The length of the 1-day-old sac fry here was 1.8 mm, with reported lengths of 4.1 mm to 5.0 mm in different species of the Garra genus after 6 h of hatching. The length of larvae of G. macrocepha and G. brevibarba were 4.60 mm and 5.50 mm respectively (Ko et al., Reference Ko, Kim, Park and Bang2011a, Reference Ko, Park, Lee and Bang2011b). Lengths of 1-day-old larvae of Cyprinus carpio were reported to be 5.0 mm and 5.38 mm (Uchida, Reference Uchida1939; Jae Min Park et al., Reference Park, Mun, Yim and Han2017), whereas Lee et al. (Reference Lee, Han and Hwang2002, Reference Lee, Han, Yoon, Hwang, Yoo, Lee, Kim and Son2004) reported 6.0 mm and 5.80 mm in P. herzi and P. nigra respectively. The hatchling (sac fry) underwent various structurally differentiation changes in the head, development of fins, alimentary canal, rudiments of almost every organ and melanophore pigmentation. The distance of two otoliths in the otic vesicle, which are present in a semi-circular structure, marked the onset of a pharyngeal period (Kimmel et al., Reference Kimmel, Ballard, Kimmel, Ullmann and Schilling1995). Gill formation occurred from four branchial arches and blood circulation in the earliest phase of post hatching was performed by aortic arches (Kimmel et al., Reference Kimmel, Ballard, Kimmel, Ullmann and Schilling1995). The hindbrain and midbrain are connected by an isthmus, which became prominent in 1-day-old sac fry. Development of pectoral and caudal fins is very important for staging during the earliest part of the hatchling stage (Kimmel et al., Reference Kimmel, Ballard, Kimmel, Ullmann and Schilling1995).
Day 1 post hatching: The length of the hatchling was 3 mm and extended yolk pouch is 1.8 mm (Fig. 6D1). The mouth and anus are closed; membranous fin was continuous dorsally in position (Fig. 6D1a, D1b). The heart was seen just ahead of the yolk pouch. The otic vesicle was seen as a semi-circular rudiment in which two tiny dot-like structures termed as otolith were also seen. Posterior to the otic vesicle a dome-like structure was seen and thought to be the rudimentary pectoral fin. Accumulation of the melanophore in the lens of the eye, presence of the adhesive gland, and differential structures of the brain-like forebrain, mid hindbrain, hindbrain, myomeres and notochord were also seen (Fig. 6D1, D1a, D1b).
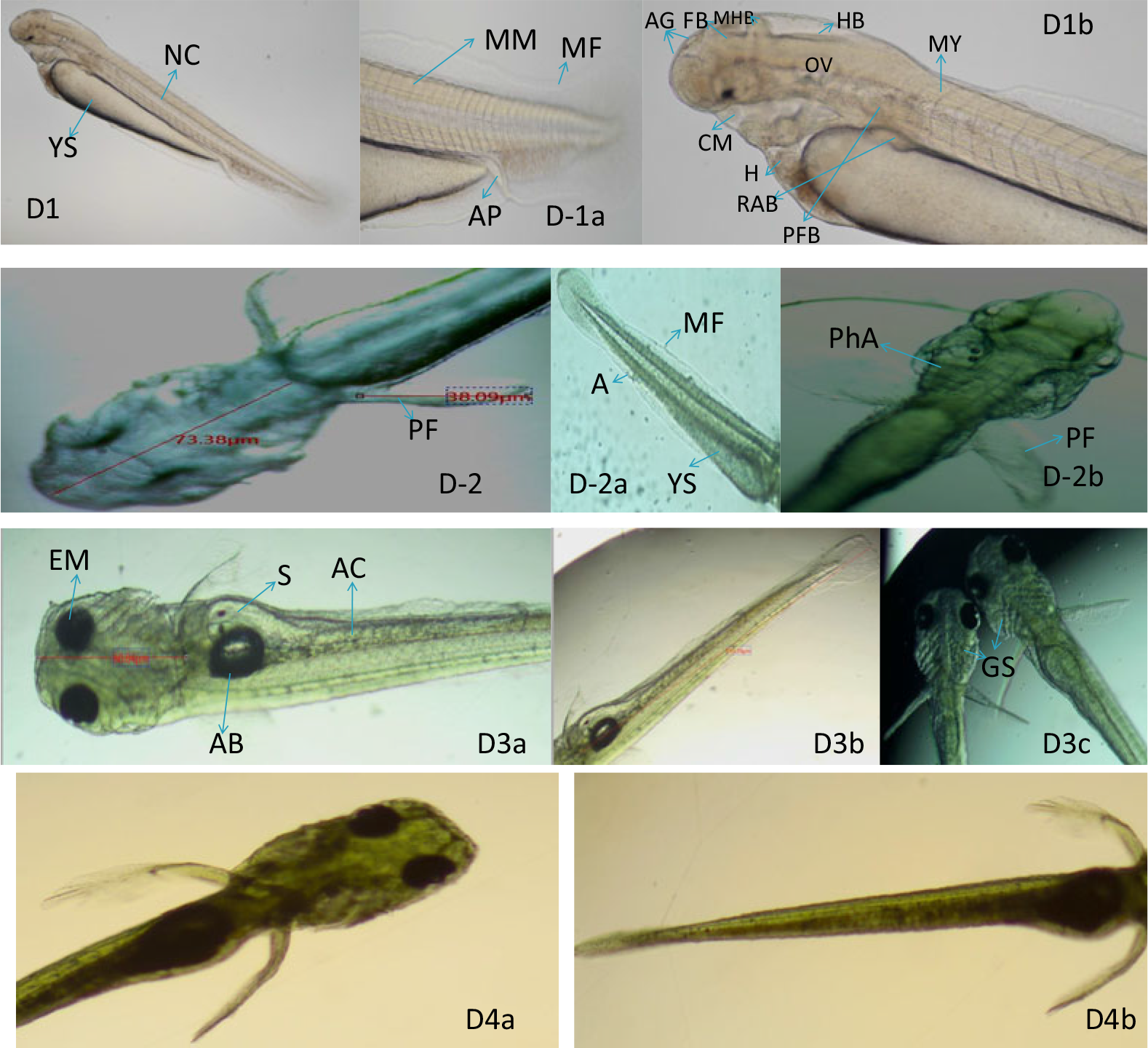
Figure 6. Development of the embryo after post hatching from Day 1 to Day 4. (D1, D1a, D1b) Left view of the newly hatched larvae. Brain is distinctly sculptured, melanophore formation though begin but not much deeper, each arrow provide structural information with respect to position of organs. (D2, D-2a, D-2b) Melanophore distribution and concentration throughout the body and visualization of pectoral fins. (D3a–c, D4a, b) Melanophore much denser in head and airbladder, distinct alimentary canal, brachiostegal membrane of operculum. YS: yolk sac, NC: notochord, MM: muscle myofibrils, MF: membranous fins, AP: anal pore, AG: adhesive gland, FB: forebrain, MB: midbrain, HB: hindbrain, CM: closed mouth, H: heart, RAB: rudiments of air bladder, PFB: pectoral fin bud, PF: pectoral fin, PhA: pharyngula of gill, EM: eye melanophore, AB: air bladder, AC: alimentary canal, GS: gill shaft, MY: myomere, S: somite.
Day 2 post hatching: The length of the head was 73.38 μm, the pectoral fin was 38.09 μm in length (Fig. 6D2) and membranous ventral fin and anal fin started differentiating by the developing anus (Fig. 6D2a). The pharyngeal differentiation was clearly viewed as seven arches out of which four were easily viewed and known as branchial arches that will bear gills (Fig. 6D2b). The head increased in size, cranial crust covered the brain, eye melanophores became much darker (Fig. 6D2b) and only three-quarters of the yolk content remained unconsumed towards the end of the day.
Day 3 of post hatching: The total length of the fry was 3.5 mm and only a small amount of yolk content was seen in the yolk pouch. A dark oval-like thick structure in the midline over the yolk sac was seen developing as densely packed with melanophores termed as the swim bladder; a developing alimentary canal with little food content was also seen (Fig. 6D3a). The ceratobranchial cartilage began to develop initially as single simple linear elements from each branchial arch asynchronously (Fig. 6D3c).
Day 4 post hatching: The pectoral fin, caudal fin and anal fin developed fin rays and the pattern of melanophore pigmentation was differential (Fig. 6D4a, D4b). Patches of xanthophores and melanophores were seen outnumbered in the head–trunk and caudal region; a deep yellow colour in the head and trunk regions was due to xanthophores. The operculum was in a much-advanced stage of development with the branchiostegal membrane. The melanophores and xanthophores occurred on the dorsal side of the craniofacial structure in the similar pattern as reported in zebrafish (Kimmel et al., Reference Kimmel, Ballard, Kimmel, Ullmann and Schilling1995), whereas in a few fish species the appearance is ventral (Swarup, Reference Swarup1958; Haffter et al., Reference Haffter, Odenthal, Mullins, Lin, Farrell and Vogelsang1996; Furutani-Seiki and Wittbrodt, Reference Furutani-Seiki and Wittbrodt2004; Fujiumura and Okada, Reference Fujimura and Okada2007).
Day 5 post hatching: The whole body length was 6 mm, deep yellow colour pigmentation in the head, trunk and the caudal region were very advanced. The cranial cover clearly differentiated into five distinct structural identities such as nasal bones, lacrimal, suborbital, postorbital, operculum, sub-operculum and cleithrum. The muscular structures of the mouth such as maxilla and premaxilla were very much in an advanced stage of development. The positioning of the pectoral fins deep from the basal position was also visible at this stage. The vertebral column was distinct and both sides of the myomeres were seen. The air bladder occupied space well in the extended formed in-between the vertebral column and gut (Fig. 7).

Figure 7. Five-day-old larvae post hatching. (D5, D5A, D5B) Cranial roof fully differentiated and sculptured in to different distinct parts along with formation and occurrence of xanthophylls all over the body. CT: cleithrum, GS: gill shaft, IE: inner ear, FR: fin rays, H: heart, L: lacrimal bone, N: nasal bone, NC: notochord, OP: operculum, PG: pituitary gland, PO: post orbital, SO: suborbital, SOP: sub-operculum, XP: xanthophores.
Conclusion
The study of embryonic and larval developmental stages in G. gotyla was carried out for the first time, as reported in the literature. This study will help to compare ontogenetic variations in the Garra genus in particular and in teleosts in general. The habitat and breeding ground destruction of most of the indigenous fish puts them at risk of vulnerability and extinction. This study will help in mass scale production of fingerlings for recreation, conservation and aquaculture purposes.
Financial support
This research received no specific grant from any funding agency, or commercial or not-for-profit sectors.
Conflicts of interest
None.
Ethical standards
Not applicable.