Introduction
Although overall scarce and patchy, the foraminiferal fossil record of Antarctica still provides enough information to give us a glimpse of its Cenozoic fauna. For West Antarctica, the reported assemblages represent mainly communities of shallow, inner-shelf settings that are Eocene to Pleistocene in age (e.g., Birkenmajer and Łuczkowska, Reference Birkenmajer and Łuczkowska1987; Gaździcki and Webb, Reference Gaździcki and Webb1996; Gaździcki and Majewski, Reference Gaździcki and Majewski2012; Caramés and Concheyro, Reference Caramés and Concheyro2013; Majewski and Gaździcki, Reference Majewski and Gaździcki2014), whereas those from deeper paleoenvironments are represented by some Miocene assemblages (Birkenmajer and Łuczkowska, Reference Birkenmajer and Łuczkowska1987) and a couple of Paleocene ones (Huber, Reference Huber, Feldmann and Woodburne1988; see paleoenvironmental revisions by Olivero et al., Reference Olivero, Ponce, Marsicano and Martinioni2007 and Marenssi et al., Reference Marenssi, Santillana and Bauer2012). For East Antarctica, it is the opposite, for very few occurrences from shallow, marine settings are known (see Quilty et al., Reference Quilty2010; Majewski et al., Reference Majewski, Olempska, Kaim and Anderson2012, Reference Majewski, Tatur, Witkowski and Gaździcki2017) and some outer-shelf to abyssal assemblages have been recovered from offshore deposits (see Thomas, Reference Thomas and Crame1989; Majewski et al., Reference Majewski, Bart and McGlannan2018). Notwithstanding this uneven but paleoecologically diverse record, occurrences of flysch-type assemblages—the most common and well-studied assemblage type of deep-sea agglutinated foraminifers—has remained unknown for both West and East Antarctica, including nearby offshore areas.
A flysch-type assemblage is mostly composed of large, morphologically simple, and coarsely agglutinated foraminifers, usually of cosmopolitan species, and is associated with ancient deep-sea deposits, especially turbidite flysch sequences (Gradstein and Berggren, Reference Gradstein and Berggren1981). This type of assemblage was present below the carbonate compensation depth (CCD) in bathyal and abyssal zones, in settings with relatively high inputs of sediment and organic matter, and is virtually exclusive for Late Cretaceous and Paleogene deposits (Kaminski and Gradstein, Reference Kaminski and Gradstein2005), with only one known early Miocene exception (Beldean and Filipescu, Reference Beldean, Filipescu, Kaminski and Filipescu2011). The other types of deep-sea agglutinated assemblages are also restricted to the Cretaceous–Paleogene interval, and are differentiated both by their paleodepths and presence or absence of certain taxa: (1) slope marl assemblage, similar to flysch-type assemblage but restricted to the bathyal zone, above the CCD; (2) abyssal assemblage, composed of small and finely-agglutinated foraminifers, in the abyssal zone below the CCD, in settings of extremely low inputs of sediment and organic matter; and (3) Scaglia-type assemblage, similar to abyssal assemblage but in the bathyal zone of seamounts, above the CCD (see Kaminski and Gradstein, Reference Kaminski and Gradstein2005 for thorough characterizations of these assemblages).
Flysch-type and similar deep-sea assemblages are not products of taphonomic bias toward the preservation of agglutinated tests, that is, their concentration is not due to the dissolution of calcareous tests. Instead, they represent exclusively agglutinated communities that thrived in deep-sea settings and the abundance and diversity of which were correlated to complex physical and chemical dynamics, e.g., variation in inputs of sediment, organic matter, and oxygen (e.g., Koutsoukos, Reference Koutsoukos, Hart, Kaminski and Smart2000; Bindiu-Haitonic and Filipescu, Reference Bindiu-Haitonic and Filipescu2016).
Here we describe large agglutinated foraminifers that, although consisting of typical deep-sea and flysch-type taxa, are found in the shallow, glacial-marine strata of the Miocene Hobbs Glacier Formation and the Plio-Pleistocene Weddell Sea Formation on Seymour Island, West Antarctica. They represent re-elaborated remains in these deposits, meaning that they were exhumed and displaced after a previous definitive burial in another deposit and then passed through a second definitive burial in these formations (see Fernández López, Reference Fernández López1991 for details on the concepts of re-elaboration and resedimentation, which differentiate processes that are indiscernible when the concept of reworking is used in a broad sense). In an attempt to uncover their depositional history, we also characterize their taphonomic features and compare them with those from the indigenous, presumably autochthonous foraminiferal assemblages of the same deposits, previously reported in Badaró and Petri (Reference Badaró and Petri2019). Lastly, we make taxonomic comparisons with other assemblages to recognize taxa that are biostratigraphically and biogeographically significant.
Geological setting
Hobbs Glacier Formation (HGF) crops out on James Ross and Seymour islands, with slightly different lithologies on each. On James Ross Island, the formation is characterized by a massive to laminated muddy diamictite in its lower part and a tuffaceous sandstone in its upper part, with crossbedding indicating paleocurrents predominantly to NE and E (Pirrie et al., Reference Pirrie, Crame, Riding, Butcher and Taylor1997a). On Seymour Island, the formation displays a matrix-supported diamictite (Marenssi et al., Reference Marenssi, Casadío and Santillana2010) with a silty matrix replacing the typical clay matrix, except for the Sey-03 section, where the latter is found in its typical form (Badaró and Petri, Reference Badaró and Petri2019).
The marine fauna of the Hobbs Glacier Formation is represented by foraminifers, bryozoans, veneroid bivalves, serpulid polychaetes, and cirripedian crustaceans, but re-elaborated remains from the underlying La Meseta Formation are plentiful (Pirrie et al., Reference Pirrie, Crame, Riding, Butcher and Taylor1997a; Jonkers et al. Reference Jonkers, Lirio, Delvalle and Kelley2002; Concheyro et al., Reference Concheyro, Salani, Adamonis and Lirio2007; Marenssi et al., Reference Marenssi, Casadío and Santillana2010). The Miocene age of the formation is indicated by dinoflagellate and bryozoan index fossils, as well as by strontium isotopes from barnacle shells that indicate a late Miocene age (9.9 ± 0.97 Ma, Tortonian Stage) (Dingle and Lavelle, Reference Dingle and Lavelle1998).
In contrast, the Weddell Sea Formation (WSF) crops out only on Seymour Island and its depositional setting remains uncertain. It has been called ‘Weddell Formation’ since the early 1980s (e.g., Malagnino et al., Reference Malagnino, Olivero, Rinaldi, Spikermann and Irigoyen1981), but was formally defined only more recently (Gaździcki et al., Reference Gaździcki, Tatur, Hara and Del Valle2004). Its diamictites, often with fine-grained matrices and a high density of clasts, were previously considered to be glacial marine (e.g., Zinsmeister and DeVries, Reference Zinsmeister and DeVries1983), but the depositional setting was reinterpreted by Gaździcki et al. (Reference Gaździcki, Tatur, Hara and Del Valle2004) as fully glacial due to the lack of clearly indigenous marine fossils and presence of glacier striae oriented in various directions. Nevertheless, Badaró and Petri (Reference Badaró and Petri2019) argued for at least some events of marine influence, in view of the presence of indigenous foraminifers and trace fossils.
The minimum age of the Weddell Sea Formation is given by the presence of sedimentary clasts coming from the Pliocene Cockburn Island Formation (which crops out in the nearby, homonymous island), and its maximum age remains indeterminate (Gadzicki et al., Reference Gaździcki, Tatur, Hara and Del Valle2004). Cockburn Island Formation was established as late Pliocene by radiometric and biostratigraphic data, which include the presence of the foraminifer Ammoelphidiella antarctica Conato and Segre, Reference Conato and Segre1974 (also identified in re-elaborated clasts on Seymour Island), apparently restricted to the Pliocene (Webb, Reference Webb1988; Gaździcki and Webb, Reference Gaździcki and Webb1996). However, the glacial interpretation of Gaździcki et al. (Reference Gaździcki, Tatur, Hara and Del Valle2004) would denote a late Pliocene to Pleistocene maximum age for the Weddell Sea Formation, because deposition would have occurred after the first marine regression of the latest Pliocene-Pleistocene regressive cycle. On the other hand, the at least occasional marine influence argued by Badaró and Petri (Reference Badaró and Petri2019) would imply a slightly earlier maximum age for its deposition, for it would have occurred under a relatively high sea level.
Materials and methods
Foraminifers occur in the muddy and sandy matrices of diamictites of the Hobbs Glacier and Weddell Sea formations on Seymour Island (Fig. 1), extracted from samples of the following stratigraphic sections: Sey-03 (64°14′05.2″S, 56°37′12.7″W), Sey-07 (64°14′20.1″S, 56°38′16.5″W), Sey-10 (64°15′26.8″S, 56°39′22.2″W), Sey-13 (64°15′31.6″S, 56°38′52.9″W), and Sey-14 (64°14′28.6″S, 56°37′09.3″'W) (Fig. 2). In the Sey-03 section, the re-elaborated specimens are associated with the indigenous, and probably autochthonous, specimens described by Badaró and Petri (Reference Badaró and Petri2019), with levels of occurrence indicated in Figure 2.

Figure 1. Location of Seymour Island and geologic map of its northeastern portion, showing the localities of the sampled outcrops (see text for coordinates). Modified from Montes et al. (Reference Montes, Nozal, Santillana, Marenssi and Olivero2013), with additional data from Marenssi et al. (Reference Marenssi, Casadío and Santillana2010) and Rocha Campos et al. (Reference Rocha Campos, Kuchenbecker, Duleba, Santos and Canile2017).

Figure 2. Simplified stratigraphic sections of the Hobbs Glacier Formation (HGF) and Weddell Sea Formation (WSF) on Seymour Island, showing the matrix lithologies of the diamictites. Numbers represent sampled levels; stratigraphic depths are indicated by the scale bars coupled with the sections.
Eighty-six samples of ~500 g each were analyzed, corresponding to 86 of 121 sampled levels. The unanalyzed samples are those from the even levels of the Sey-13 section, which was sampled in more detail (every 20 cm) than the other sections. Because we choose to process a large quantity of rock for each sampled level, which resulted in an equally large quantity of sediment to be systematically examined under a stereomicroscope, this was necessary to allow us to complete these procedures in a reasonable amount of time. This decision was based on a pilot study in which we discovered that fossil remains were scarce in the samples and that it was necessary to process large amounts to find only a few foraminiferal tests.
The poorly consolidated samples were primarily disaggregated with water, but moderately consolidated pieces were disintegrated after reprocessing using the kerosene method described by Thomas and Murney (Reference Thomas and Murney1985). A few highly lithified sedimentary clasts, probably originally from much older glacial cycles, could not be disaggregated. The disaggregated material was then passed through sieves of 1 mm, 0.5 mm, and 0.063 mm. All specimens were found in sediments concentrated in the 0.5 mm sieve. Although some tests are slightly over 0.5 mm in maximum diameter, many are considerably larger.
To clean the micrite that we found encrusting the tests, we selected a few poorly preserved, undetermined specimens to test the effectiveness of 10% hydrochloric acid in removing it. Some specimens showed very little improvement after an hour of exposure, whereas others suffered severe damage after a much shorter period, as though the micrite was serving as a structural support for the tests. So, in view of the paucity of specimens, removing this material proved to be unfeasible.
High-resolution images and elemental spectra of selected specimens were acquired through an electron microscope (Quanta 250, FEI). Both scanning electron microscopy (SEM) and energy-dispersive X-ray spectroscopy (EDS) were performed under low vacuum on uncoated specimens. Spectral measurements were taken from areas comprising most of the test surface or the encrusted sediment, and not from isolated dots.
Samples were collected by Antonio C. Rocha Campos (Universidade de São Paulo) and his team during the austral summers of 2011 and 2012, during the 29th and 30th Brazilian Antarctic operations. The stratigraphic and sample notation used herein is that adopted by Rocha Campos's team during their campaigns.
The systematic arrangement in the text follows the classification of Loeblich and Tappan (Reference Loeblich and Tappan1987), also considering modifications proposed by Loeblich and Tappan (Reference Loeblich and Tappan1989, Reference Loeblich, Tappan, Takayanagi and Saito1992), Sen Gupta (Reference Sen Gupta and Sen Gupta1999), Mikhalevich (Reference Mikhalevich, Bubik and Kaminski2004), and Kaminski (Reference Kaminski, Bubík and Kaminski2004). It also incorporates the morphological- and molecular-based supraordinal classification of Pawlowski et al. (Reference Pawlowski, Holzmann and Tyszka2013). Synonymy lists are generally based on Kaminski and Gradstein (Reference Kaminski and Gradstein2005), who revised several cosmopolitan deep-sea taxa. We have included only described species, but cited species are mentioned in remarks when needed for comparative purposes.
Repository and institutional abbreviation
Microfossil slides containing the specimens are deposited in the micropaleontological collection (GP/5E) of the Laboratório de Paleontologia Sistemática of the Instituto de Geociências, Universidade de São Paulo (LPS-IGc-USP), under the numbers 4308, 4310, 4313, 4317, 4342, 4347, 4348, 4353, and 4363–4365.
Systematic paleontology
Phylum Foraminifera d’Orbigny, Reference d'Orbigny1826
Class ‘Monotalamids’ Pawlowski, Holzmann, and Tyszka, Reference Pawlowski, Holzmann and Tyszka2013
Order Astrorhizida Lankester, Reference Lankester1885
Suborder Astrorhizina Lankester, Reference Lankester1885
Superfamily Astrorhizoidea Brady, Reference Brady1881
Family Rhabdamminidae Brady, Reference Brady1884
Subfamily Bathysiphoninae Avnimelech, Reference Avnimelech1952
Genus Bathysiphon Sars, Reference Sars1872
Type species
Bathysiphon filiformis Sars, Reference Sars1872, by original designation.
Bathysiphon sp. indet. 1
Figure 3.1
Occurrence
Levels Sey-03-03 (WSF) and Sey-14-07 (HGF).

Figure 3. Large agglutinated foraminifers from the Hobbs Glacier and Weddell Sea formations on Seymour Island: (1) Bathysiphon sp. indet. 1, Sey-14-07 (HGF), GP/5E-4353; (2) Bathysiphon sp. indet. 2, Sey-03-07 (HGF), GP/5E-4313; (3) Nothia robusta (Grzybowski, Reference Grzybowski1898), Sey-03-07 (HGF), GP/5E-4313; (4) Psammosiphonella cylindrica (Glaessner, Reference Glaessner1937), Sey-03-07 (HGF), GP/5E-4313; (5) Saccammina grzybowskii (Schubert, Reference Schubert1902), Sey-03-01 (WSF), GP/5E-4308; (6) Ammodiscus pennyi Cushman and Jarvis, Reference Cushman and Jarvis1928, Sey-03-07 (HGF), GP/5E-4313; (7–11) Ammodiscus vastus n. sp.: (7, 8) holotype, Sey-03-07 (HGF), GP/5E-4363; (9, 10) paratype, Sey-03-07 (HGF), GP/5E-4364; (11) paratype, Sey-03-03 (WSF), GP/5E-4365; (12) Glomospira charoides (Jones and Parker, Reference Jones and Parker1860), Sey-07-01 (WSF), GP/5E-4317; (13) ?Gobbettia sp. indet., Sey-03-07 (HGF), GP/5E-4313; (14) Haplophragmoides sp. indet., Sey-13-17 (WSF), GP/5E-4342; (15) Sculptobaculites barri Beckmann, Reference Beckmann1991, Sey-03-01 (WSF), GP/5E-4308; (16) Verneuilinoides durus McNeil, Reference McNeil1997, Sey-03-01 (WSF), GP/5E-4308. Scanning electron micrographs.
Description
Test tubular, subcircular to elliptical in cross section. Wall finely agglutinated.
Materials
Seven specimens: five from WSF (GP/5E-4310), two from HGF (GP/5E-4353).
Remarks
The test is composed solely of inorganic grains. There is no sign of poriferan sclerites or other biogenic materials, which are common in some species of the genus (Gooday and Claugher, Reference Gooday and Claugher1989). It is similar in size to rhabdamminids of the López de Bertodano Formation, but these are coarser grained (Huber, Reference Huber, Feldmann and Woodburne1988).
Bathysiphon sp. indet. 2
Figure 3.2
Occurrence
Level Sey-03-07 (HGF).
Description
Test tubular, elliptical in cross section. Wall moderately agglutinated. Discrete, irregular constriction near one end.
Materials
One specimen (GP/5E-4313).
Remarks
The specimen could represent a large morphotype of Bathysiphon sp. indet. 1.
Genus Nothia Pflaumann, Reference Pflaumann1964
Type species
Rhizammina grilli Noth, Reference Noth1951, by original designation.
Nothia robusta (Grzybowski, Reference Grzybowski1898)
Figure 3.3
- Reference Grzybowski1898
Dendrophrya robusta Grybowski, p. 273, pl. 10, fig. 7.
- Reference Friedberg1902
Dendrophrya robusta var. maxima Friedberg, p. 460, pl. 22, fig. 4.
Holotype
Eocene, Krosno, Poland (Grzybowski, Reference Grzybowski1898, pl. 10, fig. 7).
Occurrence
Level Sey-03-07 (HGF).
Description
Test tubular, unbranched, elliptical in cross section. Wall finely to moderately agglutinated. Median longitudinal groove along one side of test.
Materials
Five specimens (GP/5E-4313).
Remarks
It is virtually impossible to distinguish single, unbranched specimens of Nothia from those of Bathysiphon, because the former is characterized by its branching potential (Loeblich and Tappan, Reference Loeblich and Tappan1987), which can only be attested for unbranched individuals by observing their association with virtually identical branched ones. Nevertheless, our specimens present a longitudinal median groove, which is a diagnostic feature of Nothia robusta (see Kaminski and Gradstein, Reference Kaminski and Gradstein2005).
Genus Psammosiphonella Avnimelech, Reference Avnimelech1952
Type species
Bathysiphon arenacea Cushman, Reference Cushman1927, by original designation.
Psammosiphonella cylindrica (Glaessner, Reference Glaessner1937)
Figure 3.4
- Reference Glaessner1937
Rhabdammina cylindrica Glaessner, p. 354, pl. 1, fig. 1.
- Reference Mjatliuk1970
Hyperammina cylindrica cylindrica; Mjatliuk, p. 57, pl. 1, fig. 12, pl. 2, figs. 7, 8, pl. 3, fig. 1.
Holotype
Paleocene or Eocene, Psekups River, Russia (Glaessner, Reference Glaessner1937, pl. 1, fig. 1).
Occurrence
Level Sey-03-07 (HGF).
Description
Test tubular, circular in cross section. Wall coarsely agglutinated.
Materials
One specimen (GP/5E-4313).
Remarks
The circular cross section of this specimen makes it different from the other rhabdamminids described herein, the elliptical cross sections of which could be interpreted as evidence of compaction.
Suborder Saccamminina Lankester, Reference Lankester1885
Superfamily Saccamminoidea Brady, Reference Brady1884
Family Saccamminidae Brady, Reference Brady1884
Subfamily Saccammininae Brady, Reference Brady1884
Genus Saccammina Carpenter, Reference Carpenter1869
Type species
Saccammina sphaerica Brady, Reference Brady1871, by subsequent designation (Cushman, Reference Cushman1928).
Saccammina grzybowskii (Schubert, Reference Schubert1902)
Figure 3.5
- Reference Grzybowski1898
Reophax difflugiformis Brady in Grzybowski, p. 277, pl. 10, figs. 11, 12.
- Reference Schubert1902
Reophax grzybowskii Schubert, p. 20, pl. 1, fig. 13a, b.
- Reference Franke1912
Pelosina complanata Franke, p. 107, pl. 3, fig. 1a, b.
- Reference Majzon1943
Placentammina gutta Majzon, p. 152, pl. 2, fig. 5a–c.
- Reference Cushman and Renz1948
Pelosina dubia Cushman and Renz, p. 5, pl. 1, fig. 7.
Holotype
Oligocene, Lake Garda, Italy (Schubert, Reference Schubert1902, pl. 1, fig. 13a, b).
Occurrence
Level Sey-03-01 (WSF).
Description
Test highly compressed. Body discoid. Neck well-defined, long, broad. Body surface slightly corrugated. Wall finely agglutinated.
Materials
One specimen (GP/5E-4308).
Remarks
We parsimoniously assign this specimen to Saccammina grzybowskii recognizing that it does not have the narrower neck typical of the species, rather, as the revised diagnosis of Kaminski and Gradstein (Reference Kaminski and Gradstein2005, p. 132) stated, only a “produced neck.” Therefore, there is no need to emend its diagnosis or to designate a new species for this morphotype, which could merely represent an ecophenotype or subspecies.
The specimen is similar to Saccammina sp. indet. from the Late Cretaceous of the eastern Alps (Butt, Reference Butt1981, pl. 16, fig. F) and to ‘Lagenammina grzybowski’ from the Late Cretaceous of Trinidad (Bolli et al., Reference Bolli, Beckmann and Saunders1994, fig. 18.10, but not the conical specimen in fig. 18.9), which are nearly identical in shape (discoid body, long and relatively broad neck) and size (~740 and 700 μm in length, respectively). It is also similar to the specimen of ‘L. grzybowski’ illustrated by Kaminski et al. (Reference Kaminski, Gradstein and Berggren1988, pl. 2, fig. 7), also from the Late Cretaceous of Trinidad, the neck of which is considerably broader at its base and tapers toward its distal end. The discoid morphology of all of these specimens indicates that they belong to the genus Saccammina, and not to the conical Lagenammina Rhumbler, Reference Rhumbler1911 (Loeblich and Tappan, Reference Loeblich and Tappan1987); the produced neck and test coarseness are diagnostic of Saccammina grzybowski (see Kaminski and Gradstein, Reference Kaminski and Gradstein2005).
Our specimen closely resembles Saccammina sphaerica, as earlier reported by Webb (Reference Webb1975, p. 834, pl. 1, fig. 7) for the Paleocene of the southern Tasman Sea, which is also represented by a single specimen. With a discoid body and a distinct neck, it can be attributed to Saccammina grzybowskii, and its corrugated surface and considerably broader neck makes it rather similar to the Seymour Island specimen.
At 1,220 μm in length, our specimen is considerably larger than the topotypes from the Oligocene of Italy (to 620 μm length) and the specimens from the Paleocene of Trinidad (to 550 μm length) (Kaminski and Gradstein, Reference Kaminski and Gradstein2005) and Miocene of Romania (to 400 μm length) (Beldean and Filipescu, Reference Beldean, Filipescu, Kaminski and Filipescu2011). The Tasman Sea specimen is larger than these other occurrences, at ~750 μm in length.
Class Tubothalamea Pawlowski, Holzmann, and Tyszka, Reference Pawlowski, Holzmann and Tyszka2013
Order Spirillinida Hohenegger and Piller, Reference Hohenegger and Piller1975 emend. Pawlowski, Holzmann, and Tyszka, Reference Pawlowski, Holzmann and Tyszka2013
Superfamily Ammodiscacea Reuss, Reference Reuss1862
Family Ammodiscidae Reuss, Reference Reuss1862
Subfamily Ammodiscinae Reuss, Reference Reuss1862
Genus Ammodiscus Reuss, Reference Reuss1862
Type species
Ammodiscus infimus Bornemann, Reference Bornemann1874, by subsequent designation (Loeblich and Tappan, Reference Loeblich and Tappan1954).
Ammodiscus pennyi Cushman and Jarvis, Reference Cushman and Jarvis1928
Figure 3.6
- Reference Cushman and Jarvis1928
Ammodiscus pennyi Cushman and Jarvis, p. 87, pl. 12, figs. 4, 5.
Holotype
Paleocene, Lizard Springs, Trinidad (Cushman and Jarvis, Reference Cushman and Jarvis1928, pl. 12, figs. 4, 5).
Occurrence
Level Sey-03-07 (HGF).
Description
Test circular in outline. Three whorls. Last whorl irregular, but still of planispiral plan. Wall finely agglutinated. Broad secondary chamber, with overall homogeneous diameter in last whorl.
Materials
Two specimens (GP/5E-4313).
Remarks
The specimens are larger, more irregular, and has one to two whorls less than those of Ammodiscus pennyi from the underlying López de Bertodano Formation (Huber, Reference Huber, Feldmann and Woodburne1988, fig. 16.7).
Ammodiscus vastus new species
Figure 3.7–3.11
Type specimens
Holotype (Fig. 3.7, 3.8), Miocene, level Sey-03-07 (HGF), Seymour Island, Antarctica, LPS-IGc-USP, GP/5E-4363. Paratypes: six from level Sey-03-07 (HGF), GP/5E-4364; three from level Sey-03-03 (WSF), GP/5E-4365.
Diagnosis
Larger than other species of genus, except for modern Ammodiscus mestayeri Cushman, Reference Cushman1919. Test distinctly elliptical in outline, reaching or exceeding 3 mm in major axis diameter. Secondary chamber diameter increasing by approximately one tenth with each whorl. Up to six whorls, potentially more in larger, older specimens.
Occurrence
Miocene to Plio-Pleistocene, Hobbs Glacier Formation (level Sey-03-07) and Weddell Sea Formation (level Sey-03-03), northeast of Seymour Island, West Antarctica.
Description
Test large, elliptical in outline, biconcave. Prolocolus large. Secondary chamber increasing gradually in diameter. To six whorls in adult, probably surpassing this in larger, older specimens. Wall finely agglutinated, dark gray-blue in color. Aperture semicircular. No true constrictions nor striations, but could display few sparse annular irregularities.
Etymology
From the Latin vastus, meaning enormous or immense, recognizing it as one of the largest species of Ammodiscus.
Dimensions
Larger complete specimen (holotype) of 3,328 μm in major axis diameter and 430 μm width. Largest incomplete specimen (paratype, Fig. 3.11) of ~1,863 μm in minor axis diameter, but major axis diameter estimated at ~3,700 μm.
Remarks
Among the fossil species of the genus, Ammodiscus vastus n. sp. is comparable in size only to the Eocene-Miocene Ammodiscus latus Grzybowski, Reference Grzybowski1898 and the Campanian-Paleocene Ammodiscus pennyi, the largest specimens of which rarely reach 2 mm and never surpass 2.5 mm diameter (Webb, Reference Webb1973; Kaminski and Gradstein, Reference Kaminski and Gradstein2005; Holbourn et al., Reference Holbourn, Henderson and MacLeod2013; Waśkowska and Kaminski, Reference Waśkowska, Kaminski, Kaminski and Alegret2017). Among modern species, Ammodiscus vastus n. sp. is surpassed in size only by Ammodiscus mestayeri Cushman, Reference Cushman1919, which reaches 4.5 mm diameter (Cushman, Reference Cushman1919), although larger specimens of Ammodiscus incertus (d’Orbigny, Reference d'Orbigny, Barker-Webb and Berthelot1839) and Ammodiscus tenuis (Brady, Reference Brady1881) reach slightly smaller sizes, to 3 mm diameter (Brady, Reference Brady1884).
Ammodiscus vastus n. sp. shares one or more morphological traits with the Cretaceous-Eocene Ammodiscus cretaceous (Reuss, Reference Reuss1845), with the Maastrichtian-Eocene Ammodiscus glabratus Cushman and Jarvis, Reference Cushman and Jarvis1928, and, especially, with the Late Cretaceous–Eocene Ammodiscus peruvianus Berry, Reference Berry1928, which is also distinctly elliptical in outline. However, Ammodiscus vastus n. sp. shows distinctive features, differing from: (1) Ammodiscus cretaceous by having fewer whorls, a secondary chamber increasing more gradually in diameter, and being distinctly elliptic instead of slightly elliptic in outline, as is the case of some Ammodiscus cretaceous specimens (Muftah, Reference Muftah, Kaminski and Geroch1995, p. 176, pl. 1, fig. 6; Kaminski and Gradstein, Reference Kaminski and Gradstein2005, p. 145, 146, pl. 14); (2) Ammodiscus glabratus by having fewer whorls, a more defined coil suture, and a secondary chamber increasing more gradually in diameter (Kaminski and Gradstein, Reference Kaminski and Gradstein2005, p. 148–150, pl. 15); and (3) Ammodiscus peruvianus by having fewer whorls and a secondary chamber increasing slightly more gradually in size (Kaminski and Gradstein, Reference Kaminski and Gradstein2005, p. 157, 158, pl. 18). Furthermore, Ammodiscus vastus n. sp. is considerably larger than these species, ~250% greater in size than the largest specimens of Ammodiscus peruvianus (see Kaminsk and Gradstein, Reference Kaminski and Gradstein2005).
Ammodiscus vastus n. sp. differs from most of the modern Ammodiscus spp. especially in its elliptical outline and larger maximum size. Further differences can be illustrated by the following examples: (1) its complete lack of striations makes it readily distinct from Ammodiscus anulatus Fiorini, Reference Fiorini2009 (p. 95, pl. 1) and Ammodiscus reesi LeRoy in LeRoy and Hodgkinson, Reference LeRoy and Hodgkinson1975 (p. 425, 426, pl. 2, fig. 7); (2) its fewer whorls distinguish it from Ammodiscus anguillae Höglund, Reference Höglund1947 (Jones, Reference Jones1994, p. 43, pl. 38, figs. 1, 3) as well as from the minute Ammodiscus antarcticus Saidova, Reference Saidova1975 (p. 69, 70, pl. 95, fig. 9). The larger Ammodiscus mestayeri displays even fewer whorls than Ammodiscus vastus n. sp., and its test is biconvex due to its width being greater in the proloculus and in the initial portion of the secondary chamber (Cushman, Reference Cushman1919, p. 597, 598, pl. 74, figs. 1, 2). In relation to the slightly smaller Ammodiscus incertus and Ammodiscus tenuis, the former has several more whorls, and the latter displays an extra whorl and the relative width of its secondary chamber is much smaller, giving it a less robust appearance (Brady, Reference Brady1884, p. 332, 333, pl. 38, figs. 1–6).
Our specimens can be readily distinguished from those of Ammodiscus cretaceous and Ammodiscus peruvianus from the Late Cretaceous of the nearby Bellinghausen Abyssal Plain (Rögl, Reference Rögl1976, pl. 2, figs. 22, 23), which, in addition to basic morphological differences, are > 10 times smaller.
The relatively large procoluli of the specimens suggest that they are macrospheric forms. This would imply that microspheric forms could have been even larger than the collected specimens.
Subfamily Usbekistaniinae Vyalov, Reference Vyalov1968
Genus Glomospira Rzehak, Reference Rzehak1885
Type species
Trochammina squamata var. gordialis Jones and Parker, Reference Jones and Parker1860, by monotypy.
Glomospira charoides (Jones and Parker, Reference Jones and Parker1860)
Figure 3.12
- Reference Jones and Parker1860
Trochammina squamata var. charoides Jones and Parker, p. 304 (unillustrated).
- Reference Majzon1943
Glomospira saturniformis Majzon, p. 31, pl. 5, fig. 9.
- Reference Emiliani1954
Glomospira favilla Emiliani, p. 133, pl. 13, fig. 15a–c.
- Reference Khalilov1959
Glomospira subcharoides Khalilov, p. 25, pl. 1, fig. 1a–c.
- Reference Subbotina1960
Glomospira pileolus Subbotina, p. 179, pl. 1, figs. 13a–14c.
- Reference Kavary and Frizzell1964
Glomospira iranensis Kavary in Kavary and Frizzel, p. 10, pl. 1, figs. 2–7.
- Reference Chamney1969
Glomospira subarctica Chamney, p. 16, pl. 2, figs. 7–9.
- Reference Soliman1972
Glomospira praecharoides Soliman, p. 36, pl. 1, figs. 1, 2, pl. 2, fig. 1.
- Reference Maslun1987
Glomospira fulgida Maslun, p. 69, pl. 2, fig. 3.
Holotype
Holocene, Mediterranean Sea (Jones and Parker, Reference Jones and Parker1860); lectotype figured by Berggren and Kaminski (Reference Berggren, Kaminski, Hemleben, Kaminski, Kuhnt and Scott1990, pl. 1, fig. 2).
Occurrence
Level Sey-07-01 (WSF).
Description
Chamber tubular and broad, twisted in vertical axis but streptospiral in final morphology. Wall coarsely agglutinated. Aperture broad.
Materials
Two specimens (GP/5E-4317).
Remarks
The specimens from WSF have no significant differences from typical Glomospira charoides, except for being considerably larger (to 1,352 μm diameter) than the largest specimens known (to 400 μm diameter; Kaminski and Gradstein, Reference Kaminski and Gradstein2005). Very small specimens were reported for the Southern Ocean by Saidova (Reference Saidova1975).
Class Globothalamea Pawlowski, Holzmann, and Tyszka, Reference Pawlowski, Holzmann and Tyszka2013
Subclass Textulariia Mikhalevich, Reference Mikhalevich1980
Order Lituolida Lankester, Reference Lankester1885
Suborder Lituolina Lankester, Reference Lankester1885
Superfamily Lituoloidea de Blainville, Reference de Blainville1827
Family Haplophragmoididae Maync, Reference Maync1952
Genus Gobbettia Dhillon, Reference Dhillon1968
Type species
Gobbettia wilfordi Dhillon, Reference Dhillon1968, by original designation.
?Gobbettia sp. indet.
Figure 3.13
Occurrence
Level Sey-03-07 (HGF).
Description
Test elliptical in outline, umbilicated. Planispiral, but somewhat irregularly coiled. Wall coarsely agglutinated. Chambers increasing rapidly in size. Last chamber inflated. Sutures radial, slightly depressed, slightly distinct. Aperture indistinct.
Materials
One specimen (GP/5E-4313).
Remarks
The minor irregularity in the planispiral coiling seems to be a common feature of Gobbettia (Loeblich and Tappan, Reference Loeblich and Tappan1987).
Genus Haplophragmoides Cushman, Reference Cushman1910
Type species
Nonionina canariensis d’Orbigny, Reference d'Orbigny, Barker-Webb and Berthelot1839, by original designation.
Haplophragmoides sp. indet.
Figure 3.14
Occurrence
Level Sey-13-17 (WSF).
Description
Test planispiral, involute, roughly elliptical in outline. Nine chambers in last whorl, rapidly increasing in size. Sutures barely visible. Periphery rounded. Aperture as equatorial slit at base of apertural face.
Materials
One specimen (GP/5E-4342).
Remarks
Despite its relatively large size, the simple morphology and elliptical outline suggest the specimen is a juvenile, as is the case for at least one species of the genus (Kaminski et al., Reference Kaminski, Waśkowska and Chan2016)
Genus Sculptobaculites Loeblich and Tappan, Reference Loeblich and Tappan1984
Type species
Ammobaculites goodlandensis Cushman and Alexander, Reference Cushman and Alexander1930, by original designation.
Sculptobaculites barri Beckmann, Reference Beckmann1991
Figure 3.15
- Reference Beckmann1991
Sculptobaculites barri Beckmann, p. 823, pl. 1, figs. 6–9.
Holotype
Paleocene, Moruga, Trinidad (Beckmann, Reference Beckmann1991, pl. 1, figs. 6–9).
Occurrence
Level Sey-03-01 (WSF).
Description
Early stage planispiral; later state uncoiled, relatively short, extending tangentially from planispiral portion. Wall coarsely agglutinated. Sutures nearly indistinct in both planispiral and uncoiled stages. Aperture terminal, subcircular.
Materials
One specimen (GP/5E-4308).
Remarks
The specimen is similar to those from the Paleocene of Trinidad (Kaminski and Gradstein, Reference Kaminski and Gradstein2005, pl. 86, fig. 5), but less laterally compressed.
Suborder Verneuilinina Mikhalevich and Kaminski in Kaminski, Reference Kaminski, Bubík and Kaminski2004
Superfamily Verneuilinoidea Cushman, Reference Cushman1911
Family Verneuilinidae Cushman, Reference Cushman1911
Subfamily Verneuilinoidinae Suleymanov, Reference Suleymanov1973
Genus Verneuilinoides Loeblich and Tappan, Reference Loeblich and Tappan1949
Type species
Verneuilina schizea Cushman and Alexander, Reference Cushman and Alexander1930, by original designation.
Verneuilinoides durus McNeil, Reference McNeil1997
Figure 3.16
- Reference McNeil1997
Verneuilinoides durus McNeil, p. 33, pl. 10, figs. 5–8.
Holotype
Paleocene, Beaufort-Mackenzie Basin, Canadian Arctic (McNeil, Reference McNeil1997, pl. 10, figs. 5–8).
Occurrence
Level Sey-03-01 (WSF).
Description
Test triserial, elongated, lobulated in section. Wall coarsely agglutinated. Chambers moderately inflated; peripheral outline slightly subacute. Four chambers in each one of the series. Sutures depressed, slightly distinct to indistinct. Aperture an interiomarginal arch.
Materials
Four specimens (GP/5E-4308).
Remarks
The specimens resemble the holotype of Verneuilinoides durus (see McNeil, Reference McNeil1997, pl. 10, figs. 5–8), and they are remarkably similar to Verneuilinoides aff. V. durus from the Late Cretaceous of the Barents Sea, Norway (Setoyama et al., Reference Setoyama, Kaminski, Tyszka, Kaminski and Filipescu2011, pl. 10, fig. 11a–c). This wider, shorter morphotype makes them distinct from the otherwise similar Verneuilinoides paleogenicus (Lipman, Burtman, and Khokhlova, Reference Lipman, Burtman and Khokhlova1960) from the Paleocene of western Siberia (Podobina, Reference Podobina, Hart, Kaminski and Smart2000, pl. 1, fig. 8a–c), and from the cosmopolitan Verneuilinoides neocomiensis (Myatlyuk, Reference Myatlyuk1939), a common species in the Early Cretaceous of the Atlantic and Pacific oceans (Maync, Reference Maync1973, pl. 1, figs. 29, 30; Kaminski et al., Reference Kaminski, Gradstein and Geroch1992, pl. 7, fig. 13; Holbourn et al., Reference Holbourn, Henderson and MacLeod2013, p. 610, fig. 1).
Suborder Loftusiina Kaminski and Mikhalevich in Kaminski, Reference Kaminski, Bubík and Kaminski2004
Superfamily Loftusioidea Brady, Reference Brady1884
Family Cyclamminidae Marie, Reference Marie1941
Subfamily Alveolophragmiinae Saidova, Reference Saidova1981
Genus Alveolophragmium Shchedrina, Reference Shchedrina1936
Type species
Alveolophragmium orbiculatum Shchedrina, Reference Shchedrina1936, by original designation.
Alveolophragmium orbiculatum Shchedrina, Reference Shchedrina1936
Figure 4.1, 4.2
- Reference Shchedrina1936
Alveolophragmium orbiculatum Shchedrina, p. 312, figs. 1–3.

Figure 4. Large agglutinated foraminifers from the Hobbs Glacier and Weddell Sea formations on Seymour Island: (1, 2) Alveolophragmium orbiculatum Shchedrina, Reference Shchedrina1936, Sey-13-69 (HGF), GP/5E-4348; (3, 4) Reticulophragmium cf. R. acutidorsatum (Hantken, Reference Hantken1868), Sey-13-17 (WSF), GP/5E-4342; (5, 6) Reticulophragmium garcilassoi (Frizzell, Reference Frizzell1943), Sey-13-67 (HGF), GP/5E-4347; (7, 8) Reticulophragmium sp. indet., Sey-13-69 (HGF), GP/5E-4348; (9, 10) Cyclammina cancellata Brady, Reference Brady1879, Sey-07-01 (WSF), GP/5E-4317; (11, 12) Cyclammina placenta (Reuss, Reference Reuss1851), Sey-03-07 (HGF), GP/5E-4313; (13–15) Budashevaella cf. B. laevigata (Voloshinova and Budasheva, Reference Voloshinova and Budasheva1961), Sey-07-01 (WSF), GP/5E-4317; (16) Recurvoides cf. R. torquis Schröder-Adams and McNeil, Reference Schröder-Adams and McNeil1994, Sey-07-01 (WSF), GP/5E-4317; (17) Recurvoides sp. indet., Sey-03-07 (HGF), GP/5E-4313. Scanning electron micrographs (1–14, 16, 17); photomicrograph (15).
Holotype
Holocene, Kara Sea, Siberian Arctic (Shchedrina, Reference Shchedrina1936, figs. 1–3).
Occurrence
Levels Sey-03-07 (HGF) and Sey-13-69 (HGF).
Description
Test almost circular in outline, biumbilicated. Wall finely to moderately agglutinated. Twelve chambers in last whorl. Sutures radial to sigmoid, slightly depressed, slightly distinct.
Materials
Five specimens: three from Sey-03-07 (GP/5E-4313), two from Sey-13-69 (GP/5E-4348).
Remarks
Our specimens are very similar to those from the early Miocene of Chile (Finger, Reference Finger2013, pl. 1, fig. 12a, b), despite being almost twice as large.
Genus Reticulophragmium Maync, Reference Maync1955
Type species
Alveolophragmium venezuelanum Maync, Reference Maync1952, by original designation.
Reticulophragmium cf. R. acutidorsatum (Hantken, Reference Hantken1868)
Figure 4.3, 4.4
Holotype
Oligocene, Budapest, Hungary (Hantken, Reference Hantken1868, pl. 1, fig. 1a, b).
Occurrence
Level Sey-13-17 (WSF).
Description
Test lenticular in outline, laterally compressed. Periphery acute. Wall moderately agglutinated. Eleven chambers in last whorl, increasing slowly in size. Sutures oblique, depressed, slightly distinct. Aperture indistinct.
Materials
Two specimens (GP/5E-4342).
Remarks
The specimens are similar to those of Reticulophragmium acutidorsatum from the Oligocene of Austria (Kaminski and Gradstein, Reference Kaminski and Gradstein2005, pl. 122, figs. 2, 3), but they have a more acute periphery and three chambers fewer in the last whorl (although still falling within the range of Reticulophragmium acutidorsatum).
Reticulophragmium garcilassoi (Frizzell, Reference Frizzell1943)
Figure 4.5, 4.6
- Reference Frizzell1943
Cyclammina garcilassoi Frizzell, p. 338, pl. 55, fig. 11.
- Reference Serova1987
Cyclammina radiata Serova, p. 79, pl. 4, fig. 5a, b.
Holotype
Paleocene, Piura, Peru (Frizzell, Reference Frizzell1943, pl. 55, fig. 11a, b).
Occurrence
Levels Sey-13-67 (HGF) and Sey-13-69 (HGF).
Description
Test lenticular, circular to lobate in outline. Peripheral margin subacute. Chambers 12–14 in last whorl. Aperture indistinct.
Materials
Three specimens: two from Sey-13-67 (GP/5E-4347), one from Sey-13-69 (GP/5E-4348).
Remarks
The characteristic internal alveolar structure is faintly visible, probably due to the sedimentary filling.
Reticulophragmium sp. indet.
Figure 4.7, 4.8
Occurrence
Levels Sey-13-67 (HGF) and Sey-13-69 (HGF).
Description
Test lenticular, circular in outline. Peripheral margin subacute. Chambers ~13 in last whorl. Aperture as narrow slit in base of last chamber.
Materials
Two specimens: one from Sey-13-67 (GP/5E-4347), one from Sey-13-69 (GP/5E-4348).
Remarks
The micrite cover prevents observation of other features, e.g., the umbilical area, suture details, and the distribution pattern of alveoles, but the overall shape of the test is very similar to some morphotypes of Reticulophragmium pauperum (Chapman, Reference Chapman1904) emend. Ludbrook, Reference Ludbrook1977 from the Paleocene of Australia (Kaminski and Gradstein, Reference Kaminski and Gradstein2005, pl. 126, fig. 1a, b). This taxon could represent poorly preserved specimens of Reticulophragmium cf. R. acutidorsatum.
Subfamily Cyclammininae Marie, Reference Marie1941
Genus Cyclammina Brady, Reference Brady1879
Type species
Cyclammina cancellata Brady, Reference Brady1879, by original designation.
Cyclammina cancellata Brady, Reference Brady1879
Figure 4.9, 4.10
- Reference Brady1879
Cyclammina cancellata Brady, p. 62.
- Reference Brady1884
Cyclammina cancellata Brady, p. 351, pl. 37, figs. 8–16.
- Reference Cushman1917
Cyclammina compressa Cushman, p. 653 (unillustrated).
- Reference Cushman1917
Cyclammina pauciloculata Cushman, p. 653 (unillustrated).
Holotype
Holocene, undefined locality (Brady, Reference Brady1884, pl. 37, figs. 8–16).
Occurrence
Level Sey-07-01 (WSF).
Description
Test almost circular in outline, laterally compressed. Peripheral margin rounded. Wall coarsely agglutinated. Sutures sigmoid, slightly depressed, distinct. Umbilicus depressed. Chambers 12–15 in last whorl, increasing slowly in size. Aperture as interiomarginal slit.
Materials
Four specimens (GP/5E-4317).
Remarks
Our specimens are not directly comparable with those from the early Miocene of Chile (Finger, Reference Finger2013, p. 109, pl. 1, figs. 15–18) and King George Island (Birkenmajer and Łuczkowska, Reference Birkenmajer and Łuczkowska1987, pl. 2, fig. 2a, b). They show lateral compression, as do those from Chile, but their shell width and suture morphology resemble those from King George Island.
Cyclammina placenta (Reuss, Reference Reuss1851)
Figure 4.11, 4.12
- Reference Reuss1851
Nonionina placenta Reuss, p. 72, pl. 5, fig. 33a, b.
- Reference Beck1943
Cyclammina pacifica Beck, p. 591, pl. 98, fig. 23.
Holotype
Oligocene, Berlin, Germany (Reuss, Reference Reuss1851, pl. 5, fig. 33a, b).
Occurrence
Level Sey-03-07 (HGF).
Description
Test elliptical in outline, laterally compressed. Chambers nine in last whorl, increasing rapidly in size. Periphery slightly lobulated. Umbilicus depressed on both sides. Planispiral but showing convolution that discretely tends to left side at ~30°. Sutures straight to slightly waved. Aperture as interiomarginal slit.
Materials
One specimen (GP/5E-4313).
Remarks
Regarding the overall morphology and especially the asymmetry in the coiling plan, the specimens are similar to those from the Eocene of the United States (Kaminski and Gradstein, Reference Kaminski and Gradstein2005, pl. 119, fig. 6a, b).
Superfamily Recurvoidoidea Alekseychik-Mitskevich, Reference Alekseychik-Mitskevich1973
Family Ammosphaeroidinidae Cushman, Reference Cushman1927
Subfamily Recurvoidinae Alekseychik-Mitskevich, Reference Alekseychik-Mitskevich1973
Genus Budashevaella Loeblich and Tappan, Reference Loeblich, Tappan and Moore1964
Type species
Circus multicameratus Voloshinova and Budasheva, Reference Voloshinova and Budasheva1961, by original designation.
Budashevaella cf. B. laevigata (Voloshinova and Budasheva, Reference Voloshinova and Budasheva1961)
Figure 4.13–4.15
Holotype
Miocene, Sakhalin Island, Russia (Voloshinova and Budasheva, Reference Voloshinova and Budasheva1961, pl. 9, fig. 2a–c).
Occurrence
Level Sey-07-01 (WSF).
Description
Test discoid. Chambers 13 in last whorl. Finely agglutinated. Sutures depressed, straight to slightly sigmoid. Chamber periphery rounded to slightly subacute. Apertural face somewhat triangular, with indistinct aperture.
Materials
Two specimens (GP/5E-4317).
Remarks
Despite being considerably larger, our specimens are similar to those of Budashevaella laevigata from the Triassic of Romania (Beldean and Filipescu, Reference Beldean, Filipescu, Kaminski and Filipescu2011, p. 8, pl. 4, fig. 1) by their outline, slight asymmetry in the planispiral coiling, and the number and morphology of the chambers. The internal alveolar structure is visible under transmitted light (Fig. 4.15).
Genus Recurvoides Earland, Reference Earland1934
Type species
Recurvoides contortus Earland, Reference Earland1934, by original designation.
Recurvoides cf. R. torquis Schröder-Adams and McNeil, Reference Schröder-Adams and McNeil1994
Figure 4.16
Holotype
Oligocene–Miocene, Beaufort-Mackenzie Basin, Canadian Arctic (Schröder-Adams and McNeil, Reference Schröder-Adams and McNeil1994, pl. 4, figs. 1–4).
Occurrence
Level Sey-07-01 (WSF).
Description
Test elliptical in outline. Initial stage streptospiral; later stage planispiral. Wall finely agglutinated. Chambers 12 in last whorl, subtriangular, increasing slowly in size. Sutures slightly distinct. Aperture indistinct.
Materials
Ten specimens (GP/5E-4317).
Remarks
Recurvoides torquis differs from Recurvoides contortus—a cosmopolitan species, common in Recent bathyal to abyssal deposits (Kaminski and Gradstein, Reference Kaminski and Gradstein2005), including those of Antarctica (Pflum, Reference Pflum1966; Jones and Pudsey, Reference Jones and Pudsey1994)—by its elliptical outline, instead of oval; its early streptospiral coiling, instead of inclined by ~90° to the last spiral part; and a final planispiral coiling, instead of nonplanispiral to slightly trochospiral. Additionally, our specimens differ from Recurvoides contortus by their smooth surface and planispiral or almost planispiral last whorl.
Recurvoides sp. indet.
Figure 4.17
Occurrence
Level Sey-03-07 (HGF).
Description
Test ellipsoidal in outline, elongated. Initial stage streptospiral; later stage nearly planispiral. Wall finely agglutinated. Chambers 10 in planispiral part, subtriangular, increasing slowly in size. Sutures slightly distinct.
Materials
Three specimens (GP/5E-4313).
Remarks
This taxon could represent poorly preserved specimens of Recurvoides cf. R. torquis.
Results
Most of the large agglutinated foraminifers come from the Sey-03 section, from strata of both the Hobbs Glacier and Weddell Sea formations, but they also occur in levels of the WSF in section Sey-07 and of the HGF in sections Sey-10, Sey-13, and Sey-14 (Figs. 3, 4); for precise stratigraphic positions, confront Figure 2 with the data of occurrence for each taxon as specified in the Systematic paleontology section. No taxa are present at > 10 individuals and some are represented by single specimens. Cases in which a given species is also found in another Antarctic deposit, ancient or Recent, are indicated in the remarks in the form of a morphological comparison.
All foraminifers described herein are internally filled and partially covered by lithified micrite, and a few exhibit postfill fragmentation, indicating that they were previously entombed in consolidated deposits and were then re-elaborated into Miocene and Plio-Pleistocene sediments. Nevertheless, micrite-free surficial areas show that the agglutinated walls are well preserved (i.e., with no signs of abrasion or bioerosion), which is a sign of a rapid, definitive burial preceding their re-elaboration, as well as of a quick reburial after any previous exhumation.
Due to sedimentary filling, the internal alveolar structure of cyclamminids is faintly visible even when the specimens are wet in oil and being observed under intense light. Similarly, the external features of some specimens are obscured by a cover of micrite.
A few undetermined specimens, which are also considerably large, were found in the 7th level of the Sey-03 section and in the 5th, 6th, and 7th levels of the Sey-14 section. These tests are clearly agglutinated, coiled, and multichambered, but they are fragmented and/or covered by micrite in the apertural or umbilical areas, preventing further taxonomic assignments.
Energy-dispersive X-ray spectroscopy, conducted on re-elaborated specimens as well as in previously described indigenous specimens, show that the element titanium is present only in former (Fig. 5), in small amounts both in the test surface and in the encrusting sediment. The atomic percentage varies from 0.02–0.03%. Because titanium was found associated with elements such as calcium, iron, silicon, and oxygen, it is not possible to infer which are the titanium-bearing minerals, ilmenite (FeTiO3), perovskite (CaTiO3), titanite (CaTiSiO5), or the polymorphs anatase, brookite, and rutile (TiO2). Other differences between the spectra are not significant, since they are related to the specific composition of each particular test (i.e., secreted calcareous or agglutinated siliciclastic grains).
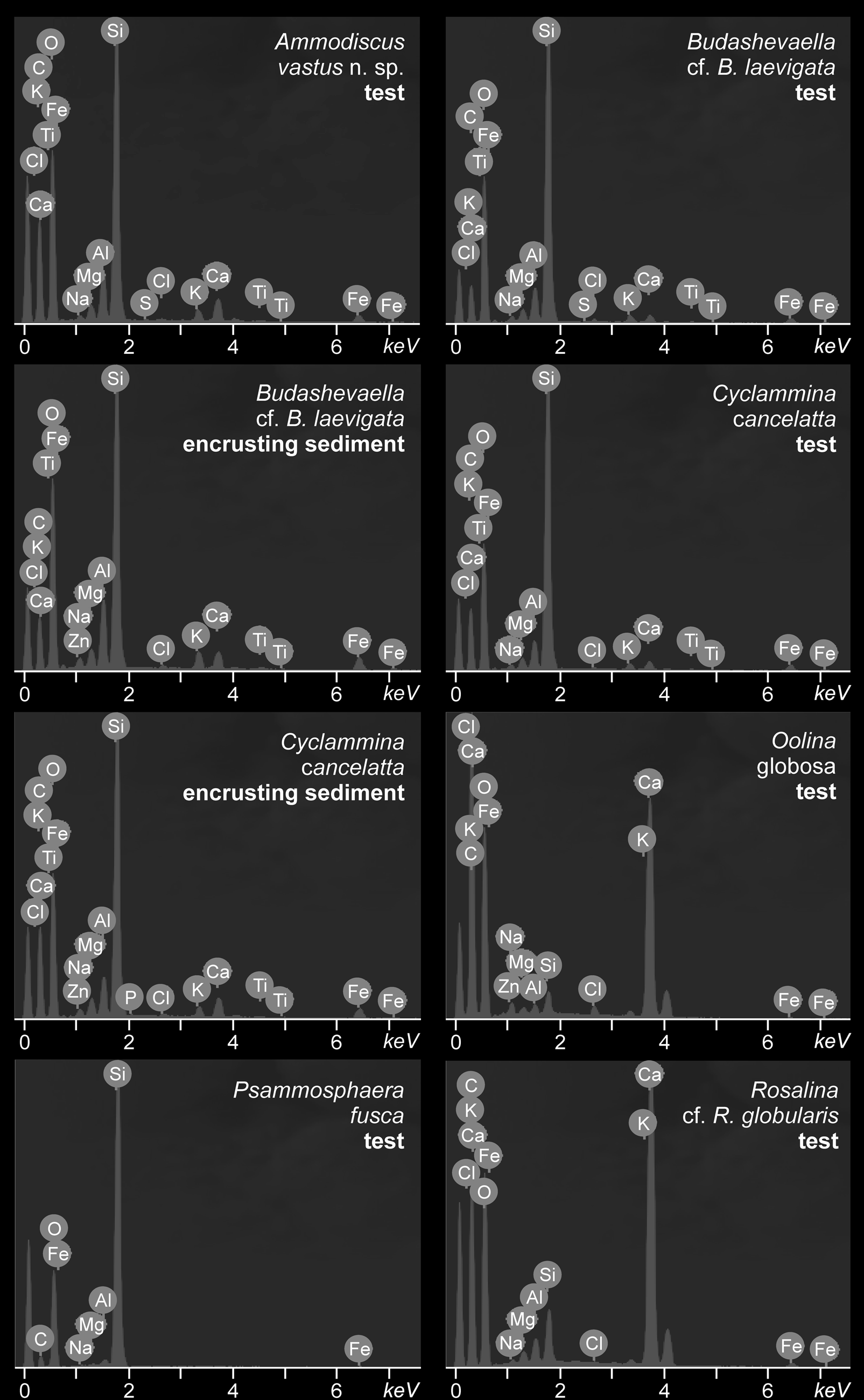
Figure 5. Energy-dispersive X-ray spectroscopy (EDS) of selected re-elaborated and indigenous foraminifers from the Hobbs Glacier and Weddell Sea formations on Seymour Island.
Discussion
Taphonomy and comparisons with other assemblages
It is very implausible that the specimens were re-elaborated from the known underlying deposits, in which large agglutinated foraminifers do occur. Except for Ammodiscus pennyi, none of our taxa is found in the Cretaceous portion of the López de Bertodano Formation, where the most common species are Cyclammina cf. C. complanata Chapman, Reference Chapman1904, Alveolophragmium macellari Huber, Reference Huber, Feldmann and Woodburne1988, and Hyperammina elongata Brady, Reference Brady1878; and there is no taxonomic correlation with the Paleocene portion of the same formation, where agglutinated taxa, e.g., Hyperammina elongata and Reophax sp. indet., are uncommon or rare (Huber, Reference Huber, Feldmann and Woodburne1988). Even in the case of Ammodiscus pennyi, our specimens display significant morphological and size differences, strongly suggesting that, despite belonging to the same species, they represent different populations and environments from those of the underlying deposits.
It is also extremely unlikely that they originated from the underlying La Meseta Formation, because the only indigenous agglutinated foraminifers known for this Eocene deposit represent small and fragile textulariids (Badaró, Reference Badaró2019). Besides these, only a single, poorly preserved trochamminid was reported (Gaździcki and Majewski, Reference Gaździcki and Majewski2012), which could have been re-elaborated from the López de Bertodano Formation because similar Trochammina species are found in this unit (Huber, Reference Huber, Feldmann and Woodburne1988).
The re-elaborated taxa rarely occur in other Antarctic deposits, whereas the indigenous species tend to be found in deposits of various ages and in different areas of the continent. In summary: (1) Psammosphaera fusca Schulze, Reference Schulze and Meyer1875 is common in the Recent deposits of the Weddell Sea and King George Island, and is also found in Cretaceous and Miocene deposits of West Antarctica; (2) Favulina hexagona (Williamson, Reference Williamson1848) occurs in Miocene deposits of the King George Island; (3) Oolina globosa (Montagu, Reference Montagu1803) is found in the Pliocene–Recent interval of the Antarctic Peninsula; (4) Globocassidulina subglobosa (Brady, Reference Brady1881) is found in the Eocene–Pliocene interval of West Antarctica; and (5) Rosalina globularis d’Orbigny, Reference d'Orbigny1826 occurs only in Recent deposits of the King George Island, but is found throughout the Oligocene–Recent interval in East Antarctica (see Badaró and Petri, Reference Badaró and Petri2019 for further geographic and stratigraphic details). In contrast, the only re-elaborated species found in another Antarctic deposit are Ammodiscus pennyi, Cyclammina cancellata, and Glomospira charoides (see Systematic paleontology section), not including the rhabdamminids, for which roughly similar but coarser morphotypes can be found in the López de Bertodano Formation (Huber, Reference Huber, Feldmann and Woodburne1988). Thus, the peculiar distribution of the large agglutinated taxa, both geographically and stratigraphically restricted, also suggest that they did not originate from the deposits that harbor the most typical taxa of the Antarctic Cenozoic.
The large agglutinated specimens differ from the indigenous specimens of the Hobbs Glacier and Weddell Sea formations, as described in Badaró and Petri (Reference Badaró and Petri2019), in paleoecologic and taphonomic ways. The benthic forms of the latter represent small and shallow infaunal taxa (Favulina hexagona, Globocassidulina cf. G. subglobosa, Oolina globosa, Psammosphaera fusca, and Rosalina cf. R. globularis), and they are in pristine condition (i.e., tests are hollow, not fragmented, and with no encrusting sediment), except for Rosalina cf. R. globularis, the signs of abrasion and dissolution of which suggest it was reworked in previous glacial cycles (for further details, see Badaró and Petri, Reference Badaró and Petri2019 and references therein). On the other hand, the large agglutinated forms comprise both erect epifaunal taxa (rhabdamminids) and surficial epifaunal to shallow infaunal taxa (ammodiscids, cyclamminids, haplophragmoidids, and recurvoidoids), and their taphonomic features (i.e., sedimentary fill and post-fill fragmentation), clearly indicate that they were entombed in, and then exhumed from, older deposits. Furthermore, there is a substantial diachrony between the taxa of the Hobbs Glacier Formation, in which the Neogene–Quaternary O. globosa co-occurred with the Paleocene index fossil Reticulophragmiun garcilassoi and the Late Cretaceous–Paleocene Ammodiscus pennyi.
Even though we can consider features such as sedimentary fill and postfill fragmentation as consistent signs of re-elaboration in foraminiferal assemblages (Reolid, Reference Reolid2008), the only unambiguous evidence for such a process is the diachronism between biostratigraphically well-defined taxa (Herrero and Canales, Reference Herrero and Canales2002). In this case, not only the paleoecologic constrast but the taphonomic and diachronic disparities alike indicate the re-elaborated origin of the large agglutinated specimens.
Nevertheless, additional evidence of the different origins of the large agglutinated and small calcareous specimens is that the element titanium is found exclusively in the former. The presence of this element could be interpreted in two ways. First, it could be seen as the result of different taphonomic histories, with titanium reacting with the re-elaborated tests sometime during their fossil diagenesis, certainly before they were mixed with the indigenous tests. In this case, titanium ions might have originated from the alteration of igneous rocks, which are common in the Antarctic Peninsula due to major magmatic events occurring in the late Paleozoic and Mesozoic (Jordan et al., Reference Jordan, Riley and Siddoway2020). The second interpretation is that titanium might have reacted with the tests while they were lying on the seafloor, when they were still alive or during their biostratinomic phase. This seems an equally probable possibility, because dissolved titanium is depleted in shallow waters but is enriched in deep waters (Orians et al., Reference Orians, Boyle and Bruland1990). This would explain the presence of titanium both in the test surface and in the encrusted micrite. It is worth mentioning that even though some agglutinated species actively select titanium for constructing their tests (Cole and Valentine, Reference Cole and Valentine2005; Makled and Langer, Reference Makled and Langer2010), the fact that this element was also found in the encrusted sediment allows us to exclude this inference for these particular specimens.
Notwithstanding these interpretations, the presence of titanium exclusively in the large agglutinated tests and in the encrusting sediment indicates that these have a different origin than that of the small calcareous specimens. The second interpretation corroborates the hypothesis that these tests originated from a deep-sea setting.
This hypothetical originally deep-sea assemblage can be readily distinguished from Scaglia-type and abyssal assemblages due to the large size and coarseness of its components. We can also infer that this assemblage was probably of the flysch-type, because it would have contained large tubular morphotypes (the rhabdamminids and ammodiscids as a whole) as well as species that, although not exclusive, are common in several flysch-type assemblages (Nothia robusta, Psammosiphonella cylindrica, Saccammina grzybowski). Because the difference between flysch-type and slope assemblages can lie in the presence or absence of key taxa or in the ratio of organic-cemented and calcareous-cemented species (see Kaminski and Gradstein, Reference Kaminski and Gradstein2005; Beldean and Filipescu, Reference Beldean, Filipescu, Kaminski and Filipescu2011), one cannot fully reject the possibility that the original assemblage was of the slope type.
Possible source deposits
Although it is clear that the foraminifers were re-elaborated from older, consolidated deposits, it is not possible to postulate a specific unit as the most probable source. There are, however, two basic possibilities.
The first is their origin from a different facies, or perhaps a different formation, correlated with the Paleocene deposits of the James Ross Island archipelago. This hypothesis is nevertheless weakened by the fact that the regional stratigraphy is relatively well-defined and none of the early Paleogene units seems likely to contain any still unknown deep-water facies (see Macellari, Reference Macellari1988; Sadler, Reference Sadler1988; Pirrie et al., Reference Pirrie, Crame, Lomas and Riding1997b; Montes et al., Reference Montes, Nozal, Santillana, Marenssi and Olivero2013), since the three Paleocene deposits—i.e., the upper López de Bertodano, Sobral, and Cross Valley formations—are interpreted as inner-shelf, possibly estuarine paleoenvironments (Olivero et al., Reference Olivero, Ponce, Marsicano and Martinioni2007; Marenssi et al., Reference Marenssi, Santillana and Bauer2012). Yet, it is worth mentioning that Sadler (Reference Sadler1988) interpreted the Cross Valley Formation as a paleocanyon, a view that would provide a suitable environment for the development of deep-sea agglutinated communities, at least from the uniformitarian perspective (Kuhnt et al., Reference Kuhnt, Collins, Scott, Hart, Kaminski and Smart2000; Gooday and Jorissen, Reference Gooday and Jorissen2012).
The second possibility is their origin from the most inner parts of West Antarctica. In this case, the Transantarctic Mountains provide a concrete example of glacial remobilization and transportation of microfossils from the mountain range to the very edge of the Antarctic Peninsula: an assemblage of the Cambrian Tommotian fauna was initially recovered from King George Island (Wrona, Reference Wrona1989, Reference Wrona2004), found in reworked clasts at a time putatively correlated to the Cambrian limestones of the mountains, and very similar assemblages were recently described from its central area (Claybourn et al., Reference Claybourn, Jacquet, Skovsted, Topper, Holmer and Brock2019). It is evident that these microfossils were able to endure such long travel only because they were still encased in sedimentary clasts, otherwise they will have been obliterated beyond the point of recognition. The large foraminifers described herein would have endured the same process, but perhaps in a more friable matrix, which would account for preservation of relatively few tests. Nevertheless, Cretaceous–Paleocene deposits have not been identified in the Transantarctic Mountains, and there are conflicting interpretations on its tectonic history—including the formation of Paleogene seaways and protomountains, crucial aspects to explain ocean-floor uplifting and subsequent re-elaboration of an agglutinated assemblage from it (see Bjil et al., Reference Bjil, Pross, Warnaar, Stickley, Huber, Guerstein, Houben, Sluijs, Visscher and Brinkhuis2011; Burton-Johnson and Riley, Reference Burton-Johnson and Riley2015; Bowman et al., Reference Bowman, Ineson, Riding, Crame, Francis, Condon, Whittle and Ferraccioli2016 and references therein). Within this same line of reasoning, an alternative source would be an accretionary terrain such as the one found in Alexander Island, which contains Cretaceous turbidites, albeit metamorphosed (Tranter, Reference Tranter1992; Burton-Johnson and Riley, Reference Burton-Johnson and Riley2015). But as in the case of the Transantartic Mountains, if any Paleocene sedimentary succession exists in the region, it is yet to be discovered.
Biostratigraphic and biogeographic implications
If one assumes the unifying taphonomic and taxonomic features of our specimens—which indicate the same depositional history and an association of deep-sea taxa—as evidence of their single source, the Paleocene age of Reticulophragmium garcilassoi must be extended to the other taxa, and the co-occurrence of the Cretaceous-Paleocene Ammodiscus pennyi further corroborates this interpretation. In fact, there is no substantial diachrony between them to invalidate such a hypothesis, except for the occurrence of Cyclammina cancellata and Cyclammina placenta, the first occurrences of which are considered as Eocene (Kaminski and Gradstein, Reference Kaminski and Gradstein2005; Holbourn et al., Reference Holbourn, Henderson and MacLeod2013). Furthermore, Ammodiscus pennyi, Cyclammina placenta, Reticulophragmium garcilassoi, and Sculptobaculites barri co-occur in the Paleocene of New Guinea (Milner, Reference Milner1997), although Kaminski and Gradstein (Reference Kaminski and Gradstein2005) stated that this occurrence of Cyclammina placenta needs further scrutiny. In the same fashion, the distinct morphotype of Saccammina grzybowskii from Seymour Island seems remarkably similar to the one found in the Paleocene of the southern Tasman Sea (which is also associated with Ammodiscus pennyi), although comparable specimens are also found in the Late Cretaceous of Trinidad and the eastern Alps (see remarks in the Systematic paleontology section).
It is difficult to interpret the possibly diachronic presence of these cyclamminids. Both Cyclammina cancellata and Cyclammina placenta are absent in the underlying deposits, and only Cyclammina cancellata occurs in another pre-Quaternary deposit of West Antarctica, this being the early Miocene Cape Melville Formation on King George Island (Birkenmajer and Łuczkowska, Reference Birkenmajer and Łuczkowska1987). Because these cyclamminids are also filled with sediment and display postfill fragmentation, like the other large agglutinated taxa, they certainly experienced similar, if not the same, diagenetic process; because this process spared the associated pristine, small foraminifers that are considered to be contemporaneous with Miocene and Plio-Pleistocene deposition (Badaró and Petri, Reference Badaró and Petri2019), the cyclamminids must be considered as older elements, possibly pre-Miocene. Consequently, the best explanation is that they were in fact Paleocene in age, which, for the case of Cyclammina placenta, would be in accordance with the aforementioned occurrence of this taxon in the Paleocene of New Guinea. The alternative explanation seems convoluted and unlikely, for it would require the existence of a second unknown deposit, post-Paleocene in age.
Regarding foraminiferal faunas, the Austral Biogeographic Province was already established by the Campanian-Paleocene, and it is well-characterized in deposits from Antarctica, southern Australia, New Zealand, and southern South America (Huber, Reference Huber, Feldmann and Woodburne1988, Reference Huber, Thomson, Crame and Thomson1991). By the Eocene, the communities of the West Antarctic continental shelf still showed strong affinities with those from southern South America and southern Oceania (Gaździcki and Majewski, Reference Gaździcki and Majewski2012), but by the Oligocene and early Miocene, very few benthic and planktonic taxa were shared with New Zealand, whereas still preserving similarities with those from southern South America (Gaździcki, Reference Gaździcki1989; Majewski and Gaździcki, Reference Majewski and Gaździcki2014).
This makes it rather interesting that the putative Paleocene assemblage from Seymour Island shares more similarities with those from the South Pacific (i.e., New Guinea and the Tasman Sea) than with those from the South Atlantic (Tjalsma, Reference Tjalsma1977; Decima and Bolli, Reference Decima and Bolli1978; Dailey, Reference Dailey1983; Widmark, Reference Widmark1997). It seems to suggest that the dispersion of deep-sea foraminifers was driven by different factors than those influencing the communities of shallower environments in the Paleogene Southern Ocean. However, it is necessary to find an in situ assemblage to better substantiate this hypothesis by further investigation.
Table 1. Main benthic foraminiferal assemblages of the Hobbs Glacier (HGF) and Weddell Sea (WSF) formations on Seymour Island. I = indigenous, reported by Badaró and Petri (Reference Badaró and Petri2019); R = re-elaborated, described herein.

Conclusion
The complex taphonomy of the deposits makes it difficult to recognize details of the depositional history of their large agglutinated foraminifers. Even though they are unified by virtually identical taphonomic features and by consisting of typical deep-sea forms and some flysch-type taxa, they occur scattered through several strata and it is impossible to affirm, with certainty, their origin from a single deep-sea assemblage. On the other hand, although it is not possible to exclude the hypothesis that at least some particular taxa were re-elaborated from the Cretaceous portion of the López de Bertodano Formation, this seems highly unlikely, because the more abundant and robust taxa of that unit are absent in the Hobbs Glacier and Weddell Sea formations. Moreover, there are important morphological and size differences between specimens of the same taxa occurring in both these Cretaceous and Neogene-Quaternary deposits. The fact that the re-elaborated taxa rarely occur in other Antarctic deposits, whereas the indigenous taxa are also found in deposits of different ages and areas of the continent, also strongly suggests the foreign origin of the former.
Therefore, the most parsimonious interpretation is that a flysch-type assemblage, representing a foraminiferal community of the Southern Ocean deep-sea floor, was slowly and gradually re-elaborated from an unknown—and by now probably completely vanished—Paleocene deposit. This represents one of the few deep-sea foraminiferal communities known for West Antarctica and, more significantly, the first flysch-type assemblage reported for the Antarctic Cenozoic. It also suggests that, in spite of the establishment of the Austral Biogeographic Province in the Late Cretaceous-Paleocene interval, the deep-sea foraminiferal communities of Antarctica still share more similarities with their Pacific counterparts.
Acknowledgments
Samples were made available by the late A. C. Rocha Campos, who supervised their collecting on Seymour Island, and by W. Duleba, who was responsible for them during the time that they were stored in her laboratory. Two anonymous reviewers provided valuable suggestions and important corrections for an earlier version of our manuscript. Funding was provided by CNPq (grant no. 5570362009-7) and CAPES (grant no. 1267397).