Attachment has a preeminent position as a theory of child mental health and well-being, with implications for life span psychological development (Bowlby, Reference Bowlby1969). One of the strengths of the theory is the interweaving of evolutionary, biological, and psychological constructs to give an integrated model of the development and maintenance of relational bonds between the child and the primary caregiver. Therefore, an awareness of the relevance of underlying biomarkers in relation to attachment is long-standing. Bowlby's formulation of attachment theory suggests that attachment to a primary caregiver provides the infant with a sense of security in the face of novel or stressful situations. Over time, repeated sensitive, congruent attachment interactions lead to the child's development of exploration (Letourneau, Giesbrecht, Bernier, & Joschko, Reference Letourneau, Giesbrecht, Bernier and Joschko2014), resilience (Masten, Reference Masten2001), emotion regulation (Denham et al., Reference Denham, Blair, DeMulder, Levitas, Sawyer, Auerbach-Major and Queenan2003; Thompson, Reference Thompson1994), and the capacity to understand one's own and other's minds (theory of mind/mentalization/mind-mindedness; Meins, Fernyhough, Wainwright, Das Gupta, & Fradley, Reference Meins, Fernyhough, Wainwright, Das Gupta and Fradley2002; Slade, Reference Slade1999), which in turn maximizes positive behavior and further relationships throughout the life course (Mikulincer & Shaver, Reference Mikulincer and Shaver2007).
It has long been argued that sensitive, responsive parenting is vital in developing secure attachment within the child–caregiver dyad (Ainsworth, Reference Ainsworth1979; Chisholm, Reference Chisholm1996). Attachment to sensitive caregivers confers a broad range of developmental benefits to children (Fraley, Roisman, Booth-Laforce, Owen, & Holland, Reference Fraley, Roisman, Booth-Laforce, Owen and Holland2013), including increased likelihood of secure classification on the Strange Situation (Ainsworth, Blehar, Waters, & Wall, Reference Ainsworth, Blehar, Waters and Wall1978), greater likelihood of developing positive peer relationships during early childhood (Kerns, Reference Kerns1994), as well as sustaining strong and trusting friendships into adolescence (Englund, Kuo, Puig, & Collins, Reference Englund, Kuo, Puig and Collins2011). Conversely, if children are exposed to insensitive, inconsistent, or abusive styles of parenting, then they are more likely to develop an insecure or disorganized style of attachment (Cyr, Euser, Bakermans-Kranenberg, & van IJzendoorn, Reference Cyr, Euser, Bakermans-Kranenburg and van IJzendoorn2010; Solomon & George, Reference Solomon and George1999). Children classified with disorganized attachment may often show ambivalence, anxiety, or fear toward their caregivers and others, as well as displaying behaviors that are erratic and contradictory, leading to negative or misdirected externalized behaviors (Zeanah, Keyes, & Settles, Reference Zeanah, Keyes and Settles2003). In longitudinal studies, children classified with disorganized attachment as infants also display developmental problems in middle childhood, adolescence, and adulthood including aggressive behaviors and lower social competence (Solomon & George, Reference Solomon, George, Solomon and George2011; Solomon, George, & De Jong, Reference Solomon, George and De Jong1995).
The Transmission Gap and Modeling of Biomarkers for Attachment
Following from this, parental sensitivity was identified as a key mediator in the process by which attachment behaviors and representations are transmitted from parent to child, and how this impacts on child development, reflected in the continuity (or perhaps the discontinuity) of patterns of attachment in the parent and those in the child. While parental sensitivity seems to be a critical factor, it has been suggested that its actual predictive power is inconsistent (De Wolff & van IJzendoorn, Reference De Wolff and van IJzendoorn1997), and evidence suggests that the correlation between parental and offspring intergenerational association in attachment has dropped from r = .47 (van IJzendoorn, Reference van IJzendoorn1995) to r = .31 (Verhage et al., Reference Verhage, Schuengel, Madigan, Fearon, Oosterman, Cassibba, Bakermans-Kranenburg and van IJzendoorn2016). As robust meta-analytic data have shown, sensitivity explains less than 50% of the association between parent and infant attachment (summarized as the attachment “transmission gap”; van IJzendoorn, Reference van IJzendoorn1995; Verhage et al., Reference Verhage, Schuengel, Madigan, Fearon, Oosterman, Cassibba, Bakermans-Kranenburg and van IJzendoorn2016). This has generated substantial research into identifying and modeling the effect of potential moderators on the relationship between parent and offspring attachment (van IJzendoorn & Bakermans-Kranenburg, Reference van IJzendoorn and Bakermans-Kranenburg2019). While psychological environmental factors such as parenting styles, parental representations of attachment, and parental sensitivity undoubtedly play a role in developing attachment and in transmission of attachment patterns (Bakermans-Kranenburg & van IJzendoorn, Reference Bakermans-Kranenburg and van IJzendoorn2007; Bernier, Matte-Gagne, Belanger, & Whipple, Reference Bernier, Matte-Gagne, Belanger and Whipple2014), there has been considerable interest in modeling of biological markers for transmission of attachment, although work from a behavioral genetic perspective has failed to produce consistent markers for intergenerational transmission (e.g., Bokhorst et al., Reference Bokhorst, Bakermans-Kranenburg, Fearon, van IJzendoorn, Fonagy and Schuengel2003; Roisman & Fraley, Reference Roisman and Fraley2008). In this respect, the transmission gap has also acted as a driver toward investigating the role of genetic biomarkers in the attachment literature. In the current review, we will focus on the role of genetics in terms of associations with attachment and child outcomes, rather than in their contribution to the transmission gap per se.
The traditional approach to modeling genetic and behavioral influences has generally focused on one of two paths. First, the impact of the individual's own biological makeup on behavior can be considered in relation to the parent–child relationship. In their classic work, Thomas and Chess (Reference Thomas and Chess1977) argued that from a very young age, infants exhibit varying degrees of emotional temperament, which in turn may impact upon the behavior and the developing relationship between mother and child. This constitutes an example of biological makeup influencing the environment. Second, there may be instances where the environment directly impacts and influences an individual's biology. For example, unresponsive caregiving and an insecure attachment could lead to changes within the hypothalamic–pituitary–adrenal (HPA) system, leading to changes in stress response and emotion regulation (Hertzman & Boyce, Reference Hertzman and Boyce2010). However, due to the multiple systems in which a child develops, these individual biological and environmental characteristics cannot be viewed as though they are working in isolation (Esposito, Setoh, Shinohara, & Bornstein, Reference Esposito, Setoh, Shinohara and Bornstein2017) and so in contemporary developmental research, a rapprochement has emerged around nature versus nurture, focusing on how these Genetic × Environmental (G × E) elements work together, both impacting and being impacted upon to create unique phenotypes within each individual child (Letourneau et al., Reference Letourneau, Giesbrecht, Bernier and Joschko2014).
G × E Studies
Early (“first wave”) G × E studies used a dual-risk approach (Sameroff, Reference Sameroff, Kessen and Mussen1983), whereby the gene acts as a filter, with the environment passing through it, and the filter straining out negative factors (Letourneau et al., Reference Letourneau, Giesbrecht, Bernier and Joschko2014). In this way, if the environment is optimal, then the filter has no job to do, but an imperfect filter would be of little use in poor conditions and could cause, in the case of the child, developmental difficulties later on. Much of the early findings on genetic heritability of attachment take this approach, focusing on a twin study methodology (critically reviewed in Barbaro, Boutwell, Barnes, & Shackelford, Reference Barbaro, Boutwell, Barnes and Shackelford2017). Furthermore, the behavioral genetic approach also delineates between shared and nonshared environments, with the majority of attachment-based twin research measuring attachment via the shared environment acting upon the twins (e.g., parental caregiving), without taking into account the longitudinal impact of nonshared environments (e.g., individual life events such as trauma). Furthermore, as the attachment relationship emerges as the result of interactions between the caregiver and child in the first year of life, attachment organization can itself be viewed as a proxy for the environment, introducing additional complexity into the modeling of the transmission of attachment organization.
Contemporary research on G × E as related to attachment adopts a more nuanced position that some genes may act with greater or lesser plasticity, and correspondingly the gene may cause atypical development in poor conditions, but may enhance positive development in an encouraging environment or vice versa (Ellis, Boyce, Belsky, Bakermans-Kranenburg, & van IJzendoorn, Reference Bakermans-Kranenburg and van IJzendoorn2011). In these “differential susceptibility” models, the gene may be responding more uniquely to the environment in which it is found. As Bakermans-Kranenburg and van IJzendoorn (Reference Bakermans-Kranenburg and van IJzendoorn2006) suggest, the most important effects of biology on attachment may be moderated by the environment in which the child finds himself or herself. For example, children living in institutionalized care, who were identified as carrying a specific gene variation, showed a higher likelihood of attachment disorganization than children with the same gene variation who were raised in foster homes (Bakermans-Kranenburg, Dobrova-Krol, & van IJzendoorn, Reference Bakermans-Kranenburg and van IJzendoorn2011).
Over the last two decades, a number of longitudinal cohorts have reported on the interactions between candidate genes and environmental factors (e.g., caregiving setting, parental sensitivity, and so on). Within these research cohorts, evidence has accumulated to support the view that specific genes do interact with the environment to influence attachment, and therefore secure attachment and attachment disorganization may be predicted by the presence or absence of specific candidate genes. However, there is little consensus as to which genes have a significant impact, and as with many approaches to G × E in developmental psychopathology, there are difficulties in replicating significant findings (Papageorgiou & Ronald, Reference Papageorgiou, Ronald, Centifani and Williams2017). In addition, many studies are hampered by small sample sizes, making it difficult to state a definitive association between G × E interactions and their impact on attachment (Hygen, Guzey, Belsky, Berg-Neilson, & Wichstrøm, Reference Hygen, Guzey, Belsky, Berg-Nielson and Wichstrøm2014; Luijk, Roisman, et al., Reference Luijk, Roisman, Haltigan, Tiemeier, Booth-LaForce, van IJzendoorn and Bakermans-Kranenburg2011). Furthermore, in these approaches, the effects of G × E interactions on attachment concern patterns of association between genes, specific environmental factors, and their impact on attachment organization as an outcome. Alternatively, it is also possible to delineate G × E interactions involving attachment whereby the gene marker interacts with attachment (as the “E” marker) to impact on a given developmental outcome (e.g., problem behavior; Li et al., Reference Li, Hygen, Widaman, Berg-Nielson, Wichstrøm and Belsky2016).
Potential Candidate Genes Identified Within Attachment Studies
Most of the research surrounding this area of study has concentrated on a small number of candidate genes that have been proposed as influential upon attachment organization. These studies can be considered to represent genes as main effects on attachment as an outcome. The candidate gene association approach assumes an association between measured characteristic and gene, enabling identification of variance in the association. From a developmental psychopathology perspective, likely candidate genes can be identified among the dopamine, serotonin, and oxytocin systems. These neurotransmitter systems are intimately connected to the development and operation of affect processing and emotion as experienced by the child, as well as being implicated in the formation of social bonds between humans (Luijk, Roisman, et al., Reference Luijk, Roisman, Haltigan, Tiemeier, Booth-LaForce, van IJzendoorn and Bakermans-Kranenburg2011).
Notable candidate genes within the dopamine system include DRD4, DRD2, and COMT variants. First, with regard to dopaminergic systems, the dopamine D4 receptor (DRD4) is a significant genetic marker for cognitive and emotional processes in the prefrontal cortex (PFC; Wang et al., Reference Wang, Ding, Flodman, Kidd, Kidd, Grady and Moyzis2004). As part of the dopaminergic system, it is also argued that DRD4 is related to concentration and attention levels and that this too may affect the attachment bond that develops between a child and primary caregiver (Graffi et al., Reference Graffi, Moss, Jolicoeur-Martineau, Moss, Lecompte, Pascuzzo and Wazana2015). Research suggests that carriers of the DRD4 7-repeat allele show lower levels of dopamine reception (Bakermans-Kranenburg & van IJzendoorn, Reference Bakermans-Kranenburg and van IJzendoorn2011). With this in mind, a link between DRD4 genotyping and attachment disorganization could be reflective of alterations in the function of these attachment-related cognitive systems. Similarly, the A1 allele of the DRD2 gene has been linked to a reduced binding effect of dopamine, leading to lower levels of dopamine in the system (Jönsson et al., Reference Jönsson, Nöthen, Grünhage, Farde, Nakashima, Propping and Sedvall1999).
Second, the gene coding for COMT, an enzyme that works to break down the dopamine, epinephrine, and norepinephrine in the PFC, is dependent upon the homozygous or heterozygous Val/Met allele. COMT is responsible for more than 60% of the dopamine breakdown in the PFC (Li et al., Reference Li, Hygen, Widaman, Berg-Nielson, Wichstrøm and Belsky2016), and individuals carrying the Val/Val genotype show COMT activity increased by fourfold compared to those carrying a Met/Met genotype (Hygen et al., Reference Hygen, Guzey, Belsky, Berg-Nielson and Wichstrøm2014). This suggests that children carrying the Val/Val genotype will have lower levels of dopamine within their system, which may ultimately impact on the ways in which they interact with primary caregivers.
Third, within the serotonin system, 5HTTLPR acts to impact upon stress levels and anxiety (Leerkes et al., Reference Leerkes, Gedaly, Zhou, Calkins, Henrich and Smolen2017; Zimmerman, Mohr, & Spangler, Reference Zimmerman, Mohr and Spangler2009). The short (s) allele of 5HTTLPR has been connected to lower efficiency compared to the long (l) allele (Lesch et al., Reference Lesch, Bengel, Heils, Sabol, Greenberg, Petri and Murphy1996), which in turn suggests that individuals who are carriers of the (s) allele (s/s or s/l) could be more susceptible to anxiety and stress than homozygous carriers of the (l) allele (l/l; Sen, Burmeister, & Ghosh, Reference Sen, Burmeister and Ghosh2004). This has potential implications for attachment systems, as infants exhibiting higher levels of stress and anxiety may experience greater difficulty in forming trusting bonds with primary caregivers.
Fourth and finally, OXTR has been highlighted as a candidate gene within the oxytocin system. As the oxytocin system is related to human empathy and bonding (Carter, Reference Carter1998), there are clear parallels to the social–affective interaction behaviors seen in attachment caregiving and receipt. It has been suggested that carriers of the GG allele of OXTR have higher levels of social cognition leading to increased prosocial behavior (Bartz, Zaki, Bolger, & Ochsner, Reference Bartz, Zaki, Bolger and Ochsner2011). This could impact on attachment security, as children with this gene may be more prone to exhibit behaviors that appeal to parents, from an early age.
In addition, as further research is undertaken within the field, other novel genes are being identified as possible candidate biomarkers. These genes go beyond what have been called the “usual suspects” (Ebstein, Israel, Chew, Zhong, & Knafo, Reference Ebstein, Israel, Chew, Zhong and Knafo2010; Pappa et al., Reference Pappa, Szekely, Mileva-Seitz, Luijk, Bakermans-Kranenburg, van IJzendoorn and Tiemeier2015), identifying additional biological systems that are influencing and influenced by the environment in which the child develops. Specific genes, and their pathways, such as HDAC1, ZNF675, and BSCD1 have been linked to disorganized attachment (Pappa et al., Reference Pappa, Szekely, Mileva-Seitz, Luijk, Bakermans-Kranenburg, van IJzendoorn and Tiemeier2015), and FKBP5 and related single nucleotide polymorphisms (SNPs) are recognized as focal due to their connections to the glucocorticoid receptor (GR) and mineralocorticoid (MR) systems that interact with the HPA axis during stressful experiences (Ising et al., Reference Ising, Depping, Siebertz, Lucae, Unschuld, Kloiber and Holsboer2008). More recently, within the GR system, NR3C1 methylation has also been identified as a possible mediator of attachment between parent and child, when external environmental factors are taken into consideration (Bosmans, Young, & Hankin, Reference Bosmans, Young and Hankin2018). This extension of research into molecular genetics, and the tentative links that are made to environmental interaction, may represent future avenues for exploration of G × E interactions. There is also the additional question of interactions between gene markers on attachment outcomes (Gene × Gene “G × G” effects; see Cicchetti, Rogosch, & Toth, Reference Cicchetti, Rogosch and Toth2011; Propper, Reference Propper2006).
However, given the aforementioned inconsistencies in the literature, there are limits to the confidence with which we can state that there are meaningful G × E associations in attachment. In addition, there is a need to more clearly delineate distinctions between attachment as an environmental factor in a G × E interaction on a child outcome, and attachment as a child outcome variable influenced by a G × E interaction. Although there have been a number of narrative overviews of the genetics of attachment (Bakermans-Kranenburg & van IJzendoorn, Reference Bakermans-Kranenburg and van IJzendoorn2007), to date it seems that there has been no systematic review and synthesis of the existing literature on G × E interactions impacting on attachment. Furthermore, many of the authors of previous studies have reported contradictory findings to that of their peers, and have themselves argued that inconsistent findings offer little to predict the conditions in which candidate genes affect attachment directly, or interact with the environment to impact attachment (Leerkes et al., Reference Leerkes, Gedaly, Zhou, Calkins, Henrich and Smolen2017; Roisman, Booth-Laforce, Belsky, Burt, & Groh, Reference Roisman, Booth-Laforce, Belsky, Burt and Groh2013).
Given the rapidly accumulating evidence around the genetics of attachment, we therefore aimed to systematically collate, synthesize, and critically evaluate the data that has thus far been presented within the area of G × E interactions and attachment. This literature incorporates multiple cohort studies and intervention trials.
The primary aims of the review were to examine the strength of association between candidate genes and (a) child attachment security/insecurity and (b) child attachment disorganization. The review sought to ascertain whether reported associations were significant, and if any consistent patterns of association could be established between attachment organization and specific gene markers. A second aim was to identify the extent to which external environmental influences may impact on attachment outcomes via G × E associations, and third, to assess whether the existing literature identifies associations between candidate genes and attachment classification (where attachment forms the “E” in a G × E interaction) upon child developmental outcomes. Fourth and finally, the review appraised methodological sources of bias in the current literature.
Method
Inclusion and exclusion criteria
A systematic search was conducted using PRISMA guidelines (Moher, Liberati, Tetzlaff, Altman, & PRISMA Group, Reference Moher, Liberati, Tetzlaff and Altman2009). The inclusion criteria identified articles that (a) reported on original primary data; (b) measured attachment of the child to a primary caregiver; (c) included a population sample of children aged 18 years old or younger; (d) identified specific genotyping; (e) presented statistical data on any association, or lack thereof, between specific genetic markers and attachment of the child–carer dyad, or external environmental factors; (f) were published between 1990 and 2018; and (g) were written in the English language. During extraction, it was also noted whether the studies had identified any significant environmental factors that may have impacted upon the associations that they reported. This allowed for identification of studies that concentrated purely on genetic influence or for G × E impact on attachment. In order to eliminate overlap of data when using cohort studies with the same population sample, separate papers were only included if it was found that they reported on different genetic markers, different haplotypes or SNPs of genetic markers, or different external environmental influences
Exclusion criteria comprised (a) articles that discussed associations between genetic markers and attachment but presented no statistical data; (b) book chapters summarizing findings of previous studies; (c) previous systematic reviews, which again only summarized previous findings; and (d) reports using nonhuman samples. Twin studies were excluded from the review, as these concerned behavioral genetics, rather than specific genetic biomarkers.
Literature search
Relevant studies were initially identified via an electronic database search of OVID (comprising PsycINFO 1806 to November Week 1 2018, Embase Classic+Embase 1947 to 2018 November 1, MEDLINE®, Ovid MEDLINE(R) Epub Ahead of Print, In-Process & Other Non-Indexed Citations, Ovid MEDLINE(R) Daily, and Ovid), Applied Social Sciences Index and Abstracts, and Google Scholar. The search terms were developed in consultation with a specialist librarian and were as follows:
attachment AND (behavio* OR organi* OR disorgani*) AND “DRD-4” OR drd4 OR COMT OR “Val/Met” OR “Val/Val” OR “5-HTTLPR” OR 5httlpr OR “g x e” OR “gene x environment”.
The year of publication was limited from 1990 to 2018, as this was deemed to be a period long enough for capturing the advanced molecular genetic results necessary, and language was limited to English. Truncation [*] was employed to increase the sensitivity of the search to include both American English and British English spelling, as well as to allow a number of word-ending inflections that would broaden the literature search (e.g., disorganized, disorganization, etc.). Duplicates across the various databases were then removed, and inclusion and exclusion criteria were applied to titles, abstracts, and full texts. The search strategy and identification of eligible studies was independently conducted by two researchers.
After confirming studies that met inclusion and exclusion criteria at the full-text level, reference lists of all included papers were checked to ascertain that no additional studies of relevance had been overlooked during the primary search. These additional studies were then also subject to a thorough scrutiny using the inclusion and exclusion criteria at the abstract and full-text levels. Disagreements over inclusion between the two researchers was resolved through consensus discussion with a third researcher not involved in the initial search process. For details of the search process see Figure 1.

Figure 1. PRISMA flowchart of identification and elimination of studies for review.
Outcomes
Outcomes were characterized as reporting a significant association between genetic markers and attachment classification, or a significant two-way association between genetic markers, environmental impact, and attachment classification. For the purposes of clarity, environmental impact was defined as any influence upon the child that was not caused by any genetic effect (Beaver, Eagle Shutt, Vaughn, DeLisi, & Wright, Reference Beaver, Eagle Shutt, Vaughn, DeLisi and Wright2012). A significant association was defined as having a p value of .05 or less. Any significant associations were then reported to compare and contrast the published results.
Quality assessment
The risk of quality assessment bias for all included studies was carried out using an adapted version of the Agency for Healthcare Research and Quality checklist (Williams, Plassman, Burke, Holsinger, & Benjamin, Reference Williams, Plassman, Burke, Holsinger and Benjamin2010). For this review, the Agency for Healthcare Research and Quality checklist was specifically adapted to test each paper against 11 criteria to ensure that author bias had been minimalized, and that limitations for each study had been addressed openly. The 11 criteria comprised (a) unbiased selection of cohort; (b) selection minimizes baseline differences; (c) sample size calculated; (d) adequate description of cohort; (e) validated method for ascertaining attachment status; (f) validated method for ascertaining participant genotype; (g) outcome assessment blind to exposure; (h) adequate follow-up period (longitudinal studies); (i) missing data/drop out addressed; (j) analysis controls for confounding variables; and (k) analytic methods appropriate. The outcome of each criteria was then entered into a scoring system and could be assigned a number of ratings including: yes = 2, partially = 1, no = 0, and N/A = 0, allowing scores to range from 0 to 22. The AHQR was conducted by two researchers, and scores were compared. On an individual item level, interrater agreement ranged from 84% to 100%, with κ values ranging from 0.6 to 1. For the final total scores for each study, the interrater agreement score was 68% with a κ value of 0.6, indicating substantial agreement. After full analysis, any studies that showed discrepancies between scores were reassessed.
Results
Characteristics of the studies
In total, 27 studies were included in the review. All of the studies used primary data, and 24 used a prospective cohort design (Bakermans-Kranenburg et al., Reference Bakermans-Kranenburg, Dobrova-Krol and van IJzendoorn2011; Barry, Kochanska, & Philibert, Reference Barry, Kochanska and Philibert2008; Borelli et al., Reference Borelli, Smiley, Rasmussen, Gómez, Seaman and Nurmi2017; Bosmans et al., Reference Bosmans, Young and Hankin2018; Cicchetti et al., Reference Cicchetti, Rogosch and Toth2011; Gervai et al., Reference Gervai, Nemoda, Lakatos, Ronai, Toth, Ney and Sasvari-Szekely2005; Graffi et al., Reference Graffi, Moss, Jolicoeur-Martineau, Moss, Lecompte, Pascuzzo and Wazana2015 and Graffi et al., Reference Graffi, Moss, Jolicoeur-Martineau, Moss, Lecompte, Pascuzzo and Wazana2018; Humphreys, Zeanah, Nelson, Fox, & Drury, Reference Humphreys, Zeanah, Nelson, Fox and Drury2015; Hygen et al., Reference Hygen, Guzey, Belsky, Berg-Nielson and Wichstrøm2014; Kochanska, Philibert, & Barry, Reference Kochanska, Philibert and Barry2009; Lakatos et al., Reference Lakatos, Toth, Nemoda, Ney, Sasvari-Szekely and Gervai2000, Reference Lakatos, Nemoda, Toth., Ronai, Ney, Sasvari-Szekely and Gervai2002; Leerkes et al., Reference Leerkes, Gedaly, Zhou, Calkins, Henrich and Smolen2017; Li et al., Reference Li, Hygen, Widaman, Berg-Nielson, Wichstrøm and Belsky2016; Luijk et al., Reference Luijk, Velders, Tharner, van IJzendoorn, Bakermans-Kranenburg, Jaddoe and Tiemeier2010; Luijk, Tharner, et al., Reference Luijk, Tharner, Bakermans-Kranenburg, van IJzendoorn, Jaddoe, Hofman and Tiemeier2011; Pappa et al., Reference Pappa, Szekely, Mileva-Seitz, Luijk, Bakermans-Kranenburg, van IJzendoorn and Tiemeier2015; Propper, Reference Propper2006; Raby et al., Reference Raby, Chicchetti, Carlson, Cutuli, Englund and Egeland2012; Spangler, Johann, Ronai, & Zimmerman, Reference Spangler, Johann, Ronai and Zimmerman2009; van IJzendoorn & Bakermans-Kranenburg, Reference van IJzendoorn and Bakermans-Kranenburg2006; Viddal, Berg-Neilson, Belsky, & Wichstrøm, Reference Viddal, Berg-Neilsen, Belsky and Wichstrøm2017; Zimmerman et al., Reference Zimmerman, Mohr and Spangler2009).
Within the 24 studies that employed a prospective cohort design, 18 cohort samples were identified. A number of cohorts were used across multiple studies, where the sample population was used to understand a variety of genetic or environmental influences. The Generation R cohort (the Netherlands) was used across 3 studies: Luijk et al. (Reference Luijk, Velders, Tharner, van IJzendoorn, Bakermans-Kranenburg, Jaddoe and Tiemeier2010), Luijk, Tharner, et al. (Reference Luijk, Tharner, Bakermans-Kranenburg, van IJzendoorn, Jaddoe, Hofman and Tiemeier2011), and Pappa et al. (Reference Pappa, Szekely, Mileva-Seitz, Luijk, Bakermans-Kranenburg, van IJzendoorn and Tiemeier2015), using data collected between 2003 and 2005. The Maternal Adversity, Vulnerability and Neurodevelopment Project (Canada) was sampled for 2 studies; Graffi et al., Reference Graffi, Moss, Jolicoeur-Martineau, Moss, Lecompte, Pascuzzo and Wazana2015; Graffi et al., Reference Graffi, Moss, Jolicoeur-Martineau, Moss, Lecompte, Pascuzzo and Wazana2018 with data collected between 2003 and 2009. Spangler et al. (Reference Spangler, Johann, Ronai and Zimmerman2009) and Zimmerman et al. (Reference Zimmerman, Mohr and Spangler2009) both used data collected from the Regensburg Longitudinal Study (Germany), with longitudinal data collected between 1974 and 2005. Two studies used a sample population from the Trondheim Early Secure Study (Norway): Hygen et al. (Reference Hygen, Guzey, Belsky, Berg-Nielson and Wichstrøm2014) and Viddal et al. (Reference Viddal, Berg-Neilsen, Belsky and Wichstrøm2017), using data collected between 2007 and 2011. The Budapest Infant Parent Study (Hungary) was used across 3 studies: Gervai et al. (Reference Gervai, Nemoda, Lakatos, Ronai, Toth, Ney and Sasvari-Szekely2005), Lakatos et al. (Reference Lakatos, Toth, Nemoda, Ney, Sasvari-Szekely and Gervai2000), and Lakatos et al. (Reference Lakatos, Nemoda, Toth., Ronai, Ney, Sasvari-Szekely and Gervai2002). Two studies were also published by the University of Iowa: Barry et al. (Reference Barry, Kochanska and Philibert2008) and Kochanska et al. (Reference Kochanska, Philibert and Barry2009). The remaining studies were published by individual cohorts, most originating from universities or health centers. The samples were researched in a number of countries, including the Netherlands (van IJzendoorn & Bakermans-Kranenburg, Reference van IJzendoorn and Bakermans-Kranenburg2006), the United States (Borelli et al., Reference Borelli, Smiley, Rasmussen, Gómez, Seaman and Nurmi2017; Bosmans et al., Reference Bosmans, Young and Hankin2018; Cicchetti et al., Reference Cicchetti, Rogosch and Toth2011; Leerkes et al., Reference Leerkes, Gedaly, Zhou, Calkins, Henrich and Smolen2017; Li et al., Reference Li, Hygen, Widaman, Berg-Nielson, Wichstrøm and Belsky2016; Propper, Reference Propper2006; Raby et al., Reference Raby, Chicchetti, Carlson, Cutuli, Englund and Egeland2012), Ukraine (Bakermans-Kranenburg et al., Reference Bakermans-Kranenburg, Dobrova-Krol and van IJzendoorn2011), and Romania (Humphreys et al., Reference Humphreys, Zeanah, Nelson, Fox and Drury2015).
The 3 remaining studies: Brumariu, Bureau, Nemoda, Sasvari-Szekely, and Lyons-Ruth (Reference Brumariu, Bureau, Nemoda, Sasvari-Szekely and Lyons-Ruth2016), Gervai et al. (Reference Gervai, Novak, Lakatos, Toth, Danis, Ronai and Lyons-Ruth2007), and Luijk, Roisman, et al. (Reference Luijk, Roisman, Haltigan, Tiemeier, Booth-LaForce, van IJzendoorn and Bakermans-Kranenburg2011), employed cross-cohort designs. Luijk, Roisman, et al. (Reference Luijk, Roisman, Haltigan, Tiemeier, Booth-LaForce, van IJzendoorn and Bakermans-Kranenburg2011) compared findings between the Generation R Study (the Netherlands) and the Study of Early Child Care and Youth Development (SECCYD; USA). The papers by Brumariu et al. (Reference Brumariu, Bureau, Nemoda, Sasvari-Szekely and Lyons-Ruth2016) and Gervai et al. (Reference Gervai, Novak, Lakatos, Toth, Danis, Ronai and Lyons-Ruth2007) reported findings from combined cohorts drawn from the Budapest Infant Parent Study (Hungary) and Harvard Medical (United States). Characteristics of all studies are described in Table 1.
Table 1. Characteristics of included studies

Note: BEIP, Bucharest Early Intervention Project. BIPS, Budapest Infant–Parent Study. ECR-RS, Experiences of Close Relationships—Relationship Structures Questionnaire. GEM, Gene Environment Mood Study. LCAI, Late Childhood Attachment Interview. MCAST, Manchester Child Attachment Story Task. MAVAN, Maternal Adversity, Vulnerability and Neurodevelopment Project. SSP, Strange Situation Procedure. SECCYD, Study of Early Child Care and Youth Development. TESS, Trondheim Early Secure Study.
Sample population
The total population for the included studies was n = 6,347 infants and children, representing 18 cohorts, (27 studies including multicohort samples), with individual samples ranging from n = 37 (Bakermans-Kranenburg et al., Reference Bakermans-Kranenburg, Dobrova-Krol and van IJzendoorn2011) to n = 1,854 (Luijk, Roisman, et al., Reference Luijk, Roisman, Haltigan, Tiemeier, Booth-LaForce, van IJzendoorn and Bakermans-Kranenburg2011). Based on the studies that reported gender (k = 21; where k = number of studies), 51.1% of the participants were male and 48.9% of the participants were female. The children within the studies ranged in age from 3 months to 16 years old. Sixteen of the studies across the review observed the G × E impact on attachment in infants aged between 3 and 18 months. Nine of the 25 studies observed children older than 18 months, ranging from 18 months to 8 years old, and 3 of these studies examined children into adolescence up to 16 years old.
Measurement of attachment
Of the studies that measured attachment classification in infants (n = 18), all used the Strange Situation Procedure (SSP) to measure attachment between child and primary caregiver. As described by Ainsworth et al. (Reference Ainsworth, Blehar, Waters and Wall1978), the SSP consists of mildly stressful events including separation from caregiver and the introduction of a stranger, ending in reunion with the caregiver. Attachment was measured in all cases when the infant was between 12 and 18 months old. In one study of children aged between 3 and 6 years (Bakermans-Kranenburg et al., Reference Bakermans-Kranenburg, Dobrova-Krol and van IJzendoorn2011) the SSP was also employed. This was also the case in one study of children aged approximately 42 months (Humpreys et al., Reference Humphreys, Zeanah, Nelson, Fox and Drury2015). As some of the children within the Humphreys et al. (Reference Humphreys, Zeanah, Nelson, Fox and Drury2015) study were raised in institutionalized care, attachment was measured between the child and the caregiver with which they had spent the most time, and appeared to be most attached to. It is not noted whether either of these studies used a modified version of the SSP for children older than 24 months.
A modified version of the SSP was utilized in 2 studies administered to children aged approximately 36 months (Graffi et al., Reference Graffi, Moss, Jolicoeur-Martineau, Moss, Lecompte, Pascuzzo and Wazana2015; Graffi et al., Reference Graffi, Moss, Jolicoeur-Martineau, Moss, Lecompte, Pascuzzo and Wazana2018). The modified SSP as described by Cassidy and Marvin (Reference Cassidy and Marvin1992) consists of four episodes of separation and reunion and is recommended for use with children of preschool age. Two included studies focusing on middle childhood (Hygen et al., Reference Hygen, Guzey, Belsky, Berg-Nielson and Wichstrøm2014; Viddal et al., Reference Viddal, Berg-Neilsen, Belsky and Wichstrøm2017) used the Manchester Child Attachment Story Task. The children within these studies were between the ages of 4 and 6 years old at the time of testing. The Manchester Child Attachment Story Task, as described by Green, Stanley, Smith, and Goldwyn (Reference Green, Stanley, Smith and Goldwyn2000), incorporates age-appropriate aspects of both the SSP and the Adult Attachment Interview, ensuring that the child is able to convey his or her feelings through a simple narrative. In the studies sampling adolescents, a number of resources were used. Borelli et al. (Reference Borelli, Smiley, Rasmussen, Gómez, Seaman and Nurmi2017) used the Security Scale, a 15-item questionnaire that is used to measure self-esteem. Bosmans et al. (Reference Bosmans, Young and Hankin2018) measured anxious and avoidant attachment with the Experiences of Close Relationships—Relationship Structure Questionnaire. This is a 10-item self-report questionnaire designed to measure anxious and avoidant attachment styles (Fraley, Heffernan, Vicary, & Brumbaugh, Reference Fraley, Heffernan, Vicary and Brumbaugh2011). Zimmerman et al. (Reference Zimmerman, Mohr and Spangler2009) used the Late Childhood Attachment Interview (LCAI) in their follow-up of children within the Regensburg Longitudinal Study at 12 years old. The LCAI comprises a semistructured interview in which the children have the opportunity to discuss their feelings of attachment to their caregivers, which can then be attributed to attachment representations (Zimmerman et al., Reference Zimmerman, Mohr and Spangler2009)
Attachment was categorized using a number of validated approaches. A continuous measure of attachment security was employed by 6 studies (Bakermans-Kranenburg et al., Reference Bakermans-Kranenburg, Dobrova-Krol and van IJzendoorn2011; Humphreys et al., Reference Humphreys, Zeanah, Nelson, Fox and Drury2015; Leerkes et al., Reference Leerkes, Gedaly, Zhou, Calkins, Henrich and Smolen2017; Luijk, Tharner, et al., Reference Luijk, Tharner, Bakermans-Kranenburg, van IJzendoorn, Jaddoe, Hofman and Tiemeier2011; Pappa et al., Reference Pappa, Szekely, Mileva-Seitz, Luijk, Bakermans-Kranenburg, van IJzendoorn and Tiemeier2015; and Viddal et al., Reference Viddal, Berg-Neilsen, Belsky and Wichstrøm2017). Zimmerman et al. (Reference Zimmerman, Mohr and Spangler2009) used the “attachment behaviour strategy scale” in line with the LCAI designed for adolescents. The remainder of the studies employed the traditional attachment classification categories (A, B, C, D).
Sampling of genetic markers
All of the genes across the studies were collected from infants and children using validated methods including saliva samples (k = 4), buccal cheek/mouth swabs (k = 19), and cord blood samples (k = 4). Cord blood sampling is known to be associated with contamination from maternal genetic material (Morin et al., Reference Morin, Gatev, McEwen, MacIsaac, Lin, Koen and Jones2017). However, sensitivity checks of this sampling indicated that contamination was present in less that 1% of cases, and where contamination was present, data were excluded from further analyses (Luijk et al., Reference Luijk, Velders, Tharner, van IJzendoorn, Bakermans-Kranenburg, Jaddoe and Tiemeier2010). Gervai et al. (Reference Gervai, Nemoda, Lakatos, Ronai, Toth, Ney and Sasvari-Szekely2005) report collecting genetic information from both the child and the parent, in an attempt to understand generational transmission rates.
A number of genetic markers were identified within studies using a candidate gene approach. Some studies concentrated on one specific gene, whereas other studies broadened their approach and incorporated more than one genotype into their testing. The two main genes examined were located in dopaminergic and serotonergic systems, which were each investigated in 14 studies. Of the “usual suspects” dopaminergic and serotonergic candidate genes, 5HTTLPR was tested for in all 14 studies (Bakermans-Kranenburg et al., Reference Bakermans-Kranenburg, Dobrova-Krol and van IJzendoorn2011; Barry et al., Reference Barry, Kochanska and Philibert2008; Brumariu et al., Reference Brumariu, Bureau, Nemoda, Sasvari-Szekely and Lyons-Ruth2016; Cicchetti et al., Reference Cicchetti, Rogosch and Toth2011; Gervai et al., Reference Gervai, Novak, Lakatos, Toth, Danis, Ronai and Lyons-Ruth2007; Humphreys et al., Reference Humphreys, Zeanah, Nelson, Fox and Drury2015; Kochanska et al., Reference Kochanska, Philibert and Barry2009; Leerkes et al., Reference Leerkes, Gedaly, Zhou, Calkins, Henrich and Smolen2017; Luijk, Roisman, et al., Reference Luijk, Roisman, Haltigan, Tiemeier, Booth-LaForce, van IJzendoorn and Bakermans-Kranenburg2011; Propper, Reference Propper2006; Raby et al., Reference Raby, Chicchetti, Carlson, Cutuli, Englund and Egeland2012; Spangler et al., Reference Spangler, Johann, Ronai and Zimmerman2009; Viddal et al., Reference Viddal, Berg-Neilsen, Belsky and Wichstrøm2017; Zimmerman et al., Reference Zimmerman, Mohr and Spangler2009); DRD4 was tested for in 12 studies (Cicchetti et al., Reference Cicchetti, Rogosch and Toth2011; Gervai et al., Reference Gervai, Nemoda, Lakatos, Ronai, Toth, Ney and Sasvari-Szekely2005, Reference Gervai, Novak, Lakatos, Toth, Danis, Ronai and Lyons-Ruth2007; Graffi et al., Reference Graffi, Moss, Jolicoeur-Martineau, Moss, Lecompte, Pascuzzo and Wazana2015; Graffi et al., Reference Graffi, Moss, Jolicoeur-Martineau, Moss, Lecompte, Pascuzzo and Wazana2018; Lakatos et al., Reference Lakatos, Toth, Nemoda, Ney, Sasvari-Szekely and Gervai2000, Reference Lakatos, Nemoda, Toth., Ronai, Ney, Sasvari-Szekely and Gervai2002; Leerkes et al., Reference Leerkes, Gedaly, Zhou, Calkins, Henrich and Smolen2017; Luijk, Roisman, et al., Reference Luijk, Roisman, Haltigan, Tiemeier, Booth-LaForce, van IJzendoorn and Bakermans-Kranenburg2011; Propper, Reference Propper2006; Spangler et al., Reference Spangler, Johann, Ronai and Zimmerman2009; van IJzendoorn & Bakermans-Kranenburg, Reference van IJzendoorn and Bakermans-Kranenburg2006), 7 of which also investigated the effect of the -521 C/T SNP promoter (Cicchetti et al., Reference Cicchetti, Rogosch and Toth2011; Gervai et al., Reference Gervai, Nemoda, Lakatos, Ronai, Toth, Ney and Sasvari-Szekely2005; Lakatos et al., Reference Lakatos, Nemoda, Toth., Ronai, Ney, Sasvari-Szekely and Gervai2002; Propper, Reference Propper2006; Spangler et al., Reference Spangler, Johann, Ronai and Zimmerman2009; van IJzendoorn & Bakermans-Kranenburg, Reference van IJzendoorn and Bakermans-Kranenburg2006); COMT in 4 studies (Hygen et al., Reference Hygen, Guzey, Belsky, Berg-Nielson and Wichstrøm2014; Leerkes et al., Reference Leerkes, Gedaly, Zhou, Calkins, Henrich and Smolen2017; Li et al., Reference Li, Hygen, Widaman, Berg-Nielson, Wichstrøm and Belsky2016; Luijk, Roisman, et al., Reference Luijk, Roisman, Haltigan, Tiemeier, Booth-LaForce, van IJzendoorn and Bakermans-Kranenburg2011); and DRD2 in 3 studies (Leerkes et al., Reference Leerkes, Gedaly, Zhou, Calkins, Henrich and Smolen2017; Luijk, Roisman, et al., Reference Luijk, Roisman, Haltigan, Tiemeier, Booth-LaForce, van IJzendoorn and Bakermans-Kranenburg2011; Propper, Reference Propper2006). In addition, OXTR was investigated in 2 studies (Leerkes et al., Reference Leerkes, Gedaly, Zhou, Calkins, Henrich and Smolen2017; Luijk, Roisman, et al., Reference Luijk, Roisman, Haltigan, Tiemeier, Booth-LaForce, van IJzendoorn and Bakermans-Kranenburg2011). Further details of the studies, genetic alleles, SNPs, and haplotypes that were identified with each gene are recorded in Table 1.
With regard to de novo candidate genes, polymorphisms BclI rs41423247, TthIIII rs10052957, GR-9b rs6198, N363S rs6195, ER22/23EK rs6189 and rs6190, within the GR receptor gene were discussed in 2 studies (Luijk et al., Reference Luijk, Velders, Tharner, van IJzendoorn, Bakermans-Kranenburg, Jaddoe and Tiemeier2010; Luijk, Tharner, et al., Reference Luijk, Tharner, Bakermans-Kranenburg, van IJzendoorn, Jaddoe, Hofman and Tiemeier2011), and the MR receptor gene was discussed in 1 study (Luijk, Tharner, et al., Reference Luijk, Tharner, Bakermans-Kranenburg, van IJzendoorn, Jaddoe, Hofman and Tiemeier2011). In addition, genetic markers known as FKBP5 (Borelli et al., Reference Borelli, Smiley, Rasmussen, Gómez, Seaman and Nurmi2017; Luijk et al., Reference Luijk, Velders, Tharner, van IJzendoorn, Bakermans-Kranenburg, Jaddoe and Tiemeier2010), NR3C1 (Bosmans et al., Reference Bosmans, Young and Hankin2018), as well as HDAC1, ZNF675, BSCD1, and CACNAZD3 (Pappa et al., Reference Pappa, Szekely, Mileva-Seitz, Luijk, Bakermans-Kranenburg, van IJzendoorn and Tiemeier2015) were all identified as genes of interest across one or more of the studies. Finally, in the only study of its type included in the review Pappa et al. (Reference Pappa, Szekely, Mileva-Seitz, Luijk, Bakermans-Kranenburg, van IJzendoorn and Tiemeier2015) performed a genome-wide association study identifying suggestive loci on chromosomes 3p21, chr12q24, chr5q15, chr3q23, chr7q11, chr2q31, chr3p25, and chr6q12.
Environmental factors identified
A number of candidate environmental factors were identified across the studies and measured for their associations with attachment organization. These factors are considered additional to identifying attachment in itself as an environmental factor. These included parental mental health (k = 2), parenting style (k = 13), physiological responses of the child (k = 2), physical attributes (k = 1), and living situation (k = 3). Furthermore, given the established importance of maternal sensitivity in attachment organization, this was measured in 12 studies. A further 7 studies (Borelli et al., Reference Borelli, Smiley, Rasmussen, Gómez, Seaman and Nurmi2017; Humphreys et al., Reference Humphreys, Zeanah, Nelson, Fox and Drury2015; Hygen et al., Reference Hygen, Guzey, Belsky, Berg-Nielson and Wichstrøm2014; Kochanska et al., Reference Kochanska, Philibert and Barry2009; Li et al., Reference Li, Hygen, Widaman, Berg-Nielson, Wichstrøm and Belsky2016; Viddal et al., Reference Viddal, Berg-Neilsen, Belsky and Wichstrøm2017; Zimmerman et al., Reference Zimmerman, Mohr and Spangler2009) identified the attachment status between child and caregiver as the candidate environmental factor, observing the interplay between genetic marker and attachment to moderate externalized behaviors. Three studies (Gervai et al., Reference Gervai, Nemoda, Lakatos, Ronai, Toth, Ney and Sasvari-Szekely2005; Lakatos et al., Reference Lakatos, Toth, Nemoda, Ney, Sasvari-Szekely and Gervai2000, Reference Lakatos, Nemoda, Toth., Ronai, Ney, Sasvari-Szekely and Gervai2002) included within the review did not identify environmental factors, reporting only on candidate gene associations with attachment. Additional covariates identified in each study are summarized in Table 1.
Significant associations between gene and attachment classification
As the studies within the review observed a number of different genetic markers, and to aid the delineation of patterns and trends, the results are presented subdivided across the candidate genes. Full details of findings, significance, and covariates are displayed in Tables 2 and 3.
Table 2. Results for associations genetic / Gene × Environment and organized attachment (secure, avoidant, or anxious ambivalent)

Note: BIPS, Budapest Infant Parent Study. GEM, Gene Environment Mood Study. RLS, Regensburg Longitudinal Study. SSP, Strange Situation Procedure. SECCYD, Study of Early Child Care and Youth Development. TESS, Trondheim Early Secure Study.
Table 3. Results for associations genetic / Gene × Environment impact and disorganized attachment

Note: BIPS, Budapest Infant Parent Study. MAVAN, Maternal Adversity, Vulnerability and Neurodevelopment Project. RLS, Regensburg Longitudinal Study. SECCYD, Study of Early Child Care and Youth Development. TESS, Trondheim Early Secure Study.
Serotonin markers: 5HTTLPR
With regard to associations between candidate genes and attachment, only 4 of 14 studies found any significant associations between 5HTTLPR and attachment organization. Propper (Reference Propper2006) reported a significant association between 5HTTLPR s/s alleles and lower avoidant attachment behaviors during the SSP, specifically during Episode 8 (reunion with caregiver). In addition, Barry et al. (Reference Barry, Kochanska and Philibert2008) reported an association between 5HTTLPR and attachment security, and Luijk, Roisman, et al. (Reference Luijk, Roisman, Haltigan, Tiemeier, Booth-LaForce, van IJzendoorn and Bakermans-Kranenburg2011) also reported that there is a significant association between the 5HTTLPR short allele (s/s or s/l) and increased attachment security although this was only observed in one of the population samples (Generation R) and was not correlated in their second sample (SECCYD). Conversely, Spangler et al. (Reference Spangler, Johann, Ronai and Zimmerman2009) reported a significant association between the 5HTTLPR short allele and attachment disorganization, but not attachment security.
Dopamine markers: DRD4, DRD2, and COMT
DRD4 was reported to have shown a significant association with attachment status in 5 out of 12 studies. However, as with 5HTTLPR, the results observed across the studies were inconsistent. Lakatos et al. (Reference Lakatos, Toth, Nemoda, Ney, Sasvari-Szekely and Gervai2000) reported that the DRD4 7-repeat allele was found more often in infants who displayed disorganized attachment. In a later study, albeit from the same cohort sample, Lakatos et al. (Reference Lakatos, Nemoda, Toth., Ronai, Ney, Sasvari-Szekely and Gervai2002) also added to their findings that the presence of the -521 T allele paired with the DRD4 gene significantly increased the risk for infants of expressing a disorganized attachment with their primary caregiver. In addition, Gervai et al. (Reference Gervai, Nemoda, Lakatos, Ronai, Toth, Ney and Sasvari-Szekely2005) reported that there was a higher than expected transmission of the DRD4 7-repeat allele from parents to infants who also exhibited a disorganized attachment. Graffi et al. (Reference Graffi, Moss, Jolicoeur-Martineau, Moss, Lecompte, Pascuzzo and Wazana2015) reported a significant association between children without the DRD4 7-repeat allele and disorganized attachment. A later study by Graffi et al. (Reference Graffi, Moss, Jolicoeur-Martineau, Moss, Lecompte, Pascuzzo and Wazana2018) reiterated the findings that children without the DRD4 7-repeat allele were more likely to exhibit disorganized attachment than children with the DRD4 7-repeat allele. A genetic influence was also observed by Propper (Reference Propper2006), which showed a significant association between DRD2 A1/A2 polymorphisms and lower avoidant attachment scores during Episode 8 of the SSP (reunion with caregiver).
Finally, while COMT was tested for in 4 studies, only 1 study reported a significant correlation between the gene and disorganized attachment. Luijk, Roisman, et al. (Reference Luijk, Roisman, Haltigan, Tiemeier, Booth-LaForce, van IJzendoorn and Bakermans-Kranenburg2011) examined the COMT gene in relation to disorganized attachment, reporting a significant correlation in both the Generation R and SECCYD cohorts.
Oxytocin markers: OXTR
A significant association was reported between OXTR heterozygotes and classification of infant disorganized attachment by Leerkes et al. (Reference Leerkes, Gedaly, Zhou, Calkins, Henrich and Smolen2017) in their full sample. However, when these analyses were delineated by racial subgroups (African American or European American), no significant patterns of association were identified. In the second study concentrating on OXTR (Luijk, Roisman, et al., Reference Luijk, Roisman, Haltigan, Tiemeier, Booth-LaForce, van IJzendoorn and Bakermans-Kranenburg2011), no associations were found.
Additional genetic markers
As noted above, an emerging strand of work has identified potential de novo genetic markers. For instance, a number of the novel genetic markers reported by Pappa et al. (Reference Pappa, Szekely, Mileva-Seitz, Luijk, Bakermans-Kranenburg, van IJzendoorn and Tiemeier2015) reported significant associations with attachment status. Presence of the BECN1 gene predicted a significant association for attachment security. The HDAC1, ZNF675, and BSCD1 genes, in conjunction with synaptic transmission pathways and cation transport, showed a significant association with disorganized attachment. Within this study, five suggestive loci on various chromosomes, 3p21, chr12q24, chr5q15, chr3q23, and chr7q11, also suggested significant correlations to disorganization. However, these marker findings are as yet unreplicated.
Significant two-way associations between gene, environment, and attachment classification
Alongside the literature on direct associations between genetic markers and attachment (main effects of genes on attachment), there is also a substantial body of literature incorporating consideration of environmental factors as outlined above. Several studies investigated the impact of environmental factors as moderators or mediators of the reported associations between genetic markers and attachment, with regard to both attachment security (Table 2) and disorganization (Table 3). We consider these studies as G × E studies with an attachment outcome.
Serotonin markers: 5HTTLPR
Parenting styles were measured as an environmental factor in many of the studies, and 5 of 8 studies reported a significant association between 5HTTLPR, parenting, and attachment status. First, Propper (Reference Propper2006) reported that children with the 5HTTLPR long (l/l) allele exhibited a greater degree of avoidant attachment behaviors when exposed to negative parenting compared to children carrying the short (s/s or s/l) allele. Second, Barry et al. (Reference Barry, Kochanska and Philibert2008) reported that children carrying the 5HTTLPR short (s/s or s/l) allele, when exposed to high levels of parental responsiveness, exhibited a greater degree of attachment security compared to children with the 5HTTLPR long (l/l) allele. In contrast, Cicchetti et al. (Reference Cicchetti, Rogosch and Toth2011) reported that nonmaltreated children with the 5HTTLPR long (l/l) allele showed higher levels of secure attachment; however, this was not replicated in children who were maltreated at home.
In addition, there was also some evidence for an association between 5HTTLPR and disorganized attachment status. Spangler et al. (Reference Spangler, Johann, Ronai and Zimmerman2009) reported that children with 5HTTLPR were more likely to have disorganized attachment when exposed to poor maternal responsiveness, and Bakermans-Kranenburg et al. (Reference Bakermans-Kranenburg, Dobrova-Krol and van IJzendoorn2011) reported that children with the 5HTTLPR short allele were significantly more at risk of developing disorganized attachment when placed in an institutionalized home, when compared to those in a family home.
Dopamine markers: DRD4, DRD2, and COMT
With regard to dopamine markers, DRD4 was reported on in 5 studies in interaction with maternal sensitivity and parenting style. However, as noted with regard to direct associations between dopamine and attachment, results across studies are somewhat contradictory.
In the first instance, van IJzendoorn and Bakermans-Kranenburg (Reference van IJzendoorn and Bakermans-Kranenburg2006) reported a significant association between the DRD4 7-repeat allele and increased ratings of disorganized attachment, in the presence of the child being exposed to maternal unresolved loss/trauma. Findings by Gervai et al. (Reference Gervai, Novak, Lakatos, Toth, Danis, Ronai and Lyons-Ruth2007) also support this position, replicating a significant association between DRD4 and disorganized attachment, in this case in the context of the child's exposure to maternal disrupted communication. In addition, Gervai et al. (Reference Gervai, Novak, Lakatos, Toth, Danis, Ronai and Lyons-Ruth2007) also reported an association between the DRD4 short form (without the 7-repeat allele) and increased rates of disorganized attachment, when exposed to disrupted communication between child and mother. Further to this, Luijk, Roisman, et al. (Reference Luijk, Roisman, Haltigan, Tiemeier, Booth-LaForce, van IJzendoorn and Bakermans-Kranenburg2011) reported a significant association between the absence of the DRD4 7-repeat allele and increased attachment security when the child experienced high levels of parental sensitivity. However, these results were only replicated within one half of their composite study (the SECCYD sample), while the opposite trend was reported within the Generation R sample. Similar to the Generation R cohort (Luijk, Roisman, et al., Reference Luijk, Roisman, Haltigan, Tiemeier, Booth-LaForce, van IJzendoorn and Bakermans-Kranenburg2011), significant associations were found between DRD4 and increased attachment security in the context of additional environmental factors. First, associations between absence of the DRD4 risk allele and greater attachment security were observed in the context of positive maternal sensitivity (Leerkes et al., Reference Leerkes, Gedaly, Zhou, Calkins, Henrich and Smolen2017). Second, there was an association between DRD4 risk genotypes on classification as disorganized with respect to attachment at age 2, for children classified as nonmaltreated (Cicchetti et al., Reference Cicchetti, Rogosch and Toth2011). The latter study also reported that an absence of DRD4 in maltreated children would lead to associations with disorganized attachment.
In contrast, in 2 studies (Leerkes et al., Reference Leerkes, Gedaly, Zhou, Calkins, Henrich and Smolen2017; Luijk, Roisman, et al., Reference Luijk, Roisman, Haltigan, Tiemeier, Booth-LaForce, van IJzendoorn and Bakermans-Kranenburg2011) DRD2 showed no significant associations when interacting with environmental factors, and no findings were reported of an effect on attachment status. Finally, an interaction between COMT homozygosity and high parental sensitivity on reduced attachment disorganization was reported by Luijk, Roisman, et al. (Reference Luijk, Roisman, Haltigan, Tiemeier, Booth-LaForce, van IJzendoorn and Bakermans-Kranenburg2011) within their Generation R sample. However, these results were not significantly replicated in the second half of the composite sample (SECCYD).
Oxytocin marker: OXTR
Leerkes et al. (Reference Leerkes, Gedaly, Zhou, Calkins, Henrich and Smolen2017) found a significant association between OXTR and attachment security, among African American infants, when they were exposed to positive maternal sensitivity. Conversely, Luijk, Roisman, et al. (2017) reported no significant associations.
Additional genetic markers
With regard to the possibility of novel genes interacting with maternal sensitivity and responsiveness, Luijk, Tharner, et al. (Reference Luijk, Roisman, Haltigan, Tiemeier, Booth-LaForce, van IJzendoorn and Bakermans-Kranenburg2011) reported that children with the minor MR allele (G) within the HPA axis developed increased attachment security, whereas those children who were exposed to maternal insensitivity and unresponsiveness were more likely to have reduced attachment security. Bosmans et al. (Reference Bosmans, Young and Hankin2018) reported that in children with anxious attachment, the interaction of NRC31 methylation and low maternal support could predict higher anxious attachment in the context of higher stress levels. However, as with de novo findings for candidate gene associations, these novel G × E interactions are still subject to replication.
Gene × Gene interaction
Two studies addressed additive risks from Gene × Gene interactions. First, Propper (Reference Propper2006) reported that a Gene × Gene interaction of the 5-HTTLPR risk alleles and the DRD2 gene allele was associated with increased resistant attachment behavior on the SSP. Second, Cicchetti et al. (Reference Cicchetti, Rogosch and Toth2011) also identified a significant association between combined risk genotyping of DRD4 and 5HTTLPR alleles and disorganized attachment at age 2 in children classified as nonmaltreated. However, this finding was not replicated for children who were classified as maltreated, indicative of an additional environmental interaction.
Studies with no significant associations between genes, attachment, and other environmental factors
In addition, 3 of the studies included within the review reported no significant associations between genetic markers or G × E influences on attachment. In studying the novel gene FKBP5 (Luijk et al., Reference Luijk, Velders, Tharner, van IJzendoorn, Bakermans-Kranenburg, Jaddoe and Tiemeier2010), no associations were reported between the genetic marker and an influence on attachment status. Raby et al. (Reference Raby, Chicchetti, Carlson, Cutuli, Englund and Egeland2012) reported no associations between 5HTTLPR, or 5HTTLPR and maternal responsiveness, and attachment. Likewise, Brumariu et al. (Reference Brumariu, Bureau, Nemoda, Sasvari-Szekely and Lyons-Ruth2016) reported no associations between 5HTTLPR, or 5HTTLPR interacting with maternal behavior, and attachment. However, they did note that 5HTTLPR was significantly associated with the infant's proneness to distress.
G × E interactions of attachment on child outcomes
In addition to studies focusing on G × E interactions on attachment outcomes, 7 studies within the included corpus of studies reported on G × E interactions on outcome where the “E” marker was identified as the attachment classification between the child and caregiver, and the outcome was another child developmental factor (Table 4). Going beyond the testing of associations within the molecular G × E field, this avenue of research specifically reported on interactions between genes and their polymorphisms, with hypothetical moderation of the effects of attachment security on social behavior (Hygen et al., Reference Hygen, Guzey, Belsky, Berg-Nielson and Wichstrøm2014).
Table 4. Results for associations Gene × Environment impact (attachment classification) and externalized behaviors

Note: BEIP, Bucharest Early Intervention Project. RLS, Regensburg Longitudinal Study. SECCYD, Study of Early Child Care and Youth Development. TESS, Trondheim Early Secure Study.
Serotonin markers: 5HTTLPR
With regard to serotonin interactions, 5HTTLPR was examined in 4 papers, each reporting a significant association between the gene and behavior, when moderated by attachment status. In relation to children with disorganized attachment, Zimmerman et al. (Reference Zimmerman, Mohr and Spangler2009) reported that 5HTTLPR and attachment interacted to moderate aggressive behavior in adolescence. Specifically, children with the 5HTTLPR short (s/s or s/l) allele and classified as disorganized attachment exhibited more hostile autonomy and appeared more aggressive. Similar findings by Kochanska et al. (Reference Kochanska, Philibert and Barry2009) reported that children with the 5HTTLPR short (s/s or s/l) allele and disorganized attachment were more likely to develop poor self-regulation skills compared to children with the homozygous long (l/l) allele. Humphreys et al. (Reference Humphreys, Zeanah, Nelson, Fox and Drury2015) added further support to this argument in their paper finding a significant association between the 5HTTLPR short (s/s) allele and disorganized attachment and an increased likelihood of displaying negative externalized behaviors. Finally, Viddal et al. (Reference Viddal, Berg-Neilsen, Belsky and Wichstrøm2017) reported findings for emotion regulation, demonstrating that children with the 5HTTLPR short (s/s) allele and disorganized attachment at 4 to 6 years were more likely to exhibit decreased emotion regulation from 6 to 8 years.
Furthermore, when observing those children within the above 4 studies classified as exhibiting a secure attachment, a similarly consistent pattern emerges. Zimmerman et al. (Reference Zimmerman, Mohr and Spangler2009) reported that children with the 5HTTLPR short (s/s or s/l) allele and secure attachment exhibited more agreeable autonomy and appeared less aggressive. Kochanska et al. (Reference Kochanska, Philibert and Barry2009) reported the same trends, with children with the 5HTTLPR short (s/s or s/l) allele and organized attachment demonstrating a likelihood to develop good self-regulation skills, compared to children with the long (l/l) allele. The results from Humphreys et al. (Reference Humphreys, Zeanah, Nelson, Fox and Drury2015) also showed a significant association between children with the 5HTTLPR short (s/s) allele and secure attachment exhibiting less negative externalized behaviors. Finally, further support for this model comes from Viddal et al. (Reference Viddal, Berg-Neilsen, Belsky and Wichstrøm2017), who reported that children with the 5HTTLPR short (s/s) allele and secure attachment at 4 to 6 years were more likely to exhibit increased emotion regulation from 6 to 8 years.
Dopamine markers: DRD4, DRD2, and COMT
With regard to dopamine, no studies examined the DRD4 or DRD2 genes in relation to G × E interaction on behavior. The COMT gene was highlighted in two studies (Hygen et al., Reference Hygen, Guzey, Belsky, Berg-Nielson and Wichstrøm2014; Li et al., Reference Li, Hygen, Widaman, Berg-Nielson, Wichstrøm and Belsky2016), both showing consistent, significant associations between the interaction of the gene, attachment status, and externalized behaviors.
Hygen et al. (Reference Hygen, Guzey, Belsky, Berg-Nielson and Wichstrøm2014) reported findings that children with the COMT val/val allele and disorganized attachment were more likely to develop aggressive behavior and poor social skills compared to those children with the met allele. These findings were supported by Li et al. (Reference Li, Hygen, Widaman, Berg-Nielson, Wichstrøm and Belsky2016), who reported that children with the homozygous val allele and disorganized attachment exhibited less positive and more negative behaviors than other children aged between 5 and 11. Li et al. (Reference Li, Hygen, Widaman, Berg-Nielson, Wichstrøm and Belsky2016) classify this behavior as the “punitive-controlling” subtype of disorganized attachment. They also reported that children with the met alleles and disorganized attachment exhibited more positive and less negative behaviors than other children aged between 5 and 11 years. This was classified as the “caregiving-controlling” subtype of disorganized attachment.
Additional genetic markers
With regard to novel gene markers, Borrelli et al. (Reference Borelli, Smiley, Rasmussen, Gómez, Seaman and Nurmi2017) reported that FKBP5 and attachment interact to predict externalized behaviors relating to emotion regulation. Their results show that child attachment security is inversely associated with respiratory sinus arrhythmia reactivity, emotional suppression, rumination, and depressive symptoms among children with high risk plasticity (CC allele); however, there was no association found for children with the AA or AC allele. As discussed in previous sections, as these are de novo findings for candidate genes, no replication has yet been reported.
Study quality
Quality ratings were applied using a quantitative summing and a thematic overview of potential methodological sources of bias. With regard to overall score, within a possible score of 0–22, the scores across the studies ranged from 13 to 22. The most frequent issue reported for study methodology was reporting of sample size. The majority of the studies did not report the sample size calculations, making it difficult to ascertain whether power was sufficient. That said, many of the papers acknowledged this issue and addressed power and sample size within their discussion sections. A second issue noted across the papers was a tendency in some papers to inadequately address missing data or dropout over time. Third, several papers did not report whether assessments were carried out blind to outcomes, although over half (n = 16) did report blind testing. All genetic testing was carried out using validated methods, and testing for attachment was undertaken using validated methods, although it should be noted that 2 studies (Bakermans-Kranenburg et al., Reference Bakermans-Kranenburg, Dobrova-Krol and van IJzendoorn2011; Humphreys et al., Reference Humphreys, Zeanah, Nelson, Fox and Drury2015) both used the SSP on older children.
As a number of the papers derive from the same cohorts (Generation R, the Budapest Infant Parent Study, Trondheim Early Secure Study, and the Maternal Adversity, Vulnerability and Neurodevelopment Project) the protocol followed by each paper was similar, if not identical. Summarizing across the included literature, while there are some methodological issues across the studies, with 4 papers receiving the top score of 22 (Gervai et al., Reference Gervai, Novak, Lakatos, Toth, Danis, Ronai and Lyons-Ruth2007; Hygen et al., Reference Hygen, Guzey, Belsky, Berg-Nielson and Wichstrøm2014; Raby et al., Reference Raby, Chicchetti, Carlson, Cutuli, Englund and Egeland2012; Viddal et al., Reference Viddal, Berg-Neilsen, Belsky and Wichstrøm2017), it can be argued that this area of research has robust methodological procedures.
Discussion
To our knowledge, this review is the first to systematically summarize the literature on associations between genetic markers, environmental factors, and attachment. In doing so, we have focused on infant and child attachment status. The key findings of our review can be broken down into three main areas of interest: (a) one-way associations between genetic markers and attachment (gene as main effect), where we find little evidence of consistent patterns of association; (b) two-way associations between genetic markers, environment, and attachment (G × E interactions on attachment outcomes), where we see some associations, albeit again somewhat inconsistently; and (c) two-way associations between genetic markers, attachment, and behavior (G × E interactions with attachment as “E” on child outcomes), where a clearer pattern emerges, particularly with regard to the role of attachment disorganization. A further methodological finding of our review is the as yet underused potential of contemporary genomics to enrich the understanding of these patterns of association and interaction.
When examining the impact of genetic markers upon attachment, we echo previous narrative reviews, indicating little consistency between findings. When considering 5HTTLPR, only 4 of the 14 studies that observed the marker even reported any significant associations, and these reports were not in agreement as to the size of effect of the gene upon attachment. Similarly, with DRD4, only 5 of 12 studies showed any associations, with disparities between findings from different cohorts. Within the results, there was insufficient evidence of association to meaningfully comment on the direct influence of DRD2, OXTR, or COMT on attachment. We therefore support the contemporary position that genetic associations with attachment are most likely to emerge via interaction effects with environmental or behavioral variables (Bakermans-Kranenburg & van IJzendoorn, Reference Bakermans-Kranenburg and van IJzendoorn2007).
Moving to our second set of findings, there seems to be a similar lack of consistency between reports of G × E interactions on attachment outcomes, although some recurring patterns of association do emerge. The existing literature is comparatively consistent on the putative influence of the serotonin gene 5HTTLPR in various environments, with a signal that 5HTTLPR long alleles were potentially implicated in secure attachment, and conversely that the short allele conferred increased risk of attachment disorganization. In contrast, findings for the main dopaminergic marker DRD4 appeared particularly inconsistent, with studies reporting failure to replicate, or contradictory findings; for example, Luijk, Roisman, et al. (Reference Luijk, Roisman, Haltigan, Tiemeier, Booth-LaForce, van IJzendoorn and Bakermans-Kranenburg2011) reported contradictory findings from two samples within the same study. As with the direct associations, there are few significant interactions reported that implicated DRD2, OXTR, and COMT, making it difficult to comment on their effect upon attachment security.
Our final theme, which we propose constitutes a potentially productive pathway for future research in this area, considers the specific case of G × E interactions between genetic markers and attachment in moderating child outcomes, particularly externalizing behaviors. In the included studies (Borrelli et al., Reference Borelli, Smiley, Rasmussen, Gómez, Seaman and Nurmi2017; Humphreys et al., Reference Humphreys, Zeanah, Nelson, Fox and Drury2015; Hygen et al., Reference Hygen, Guzey, Belsky, Berg-Nielson and Wichstrøm2014; Kochanska et al., Reference Kochanska, Philibert and Barry2009; Li et al., Reference Li, Hygen, Widaman, Berg-Nielson, Wichstrøm and Belsky2016; Viddal et al., Reference Viddal, Berg-Neilsen, Belsky and Wichstrøm2017; Zimmerman et al., Reference Zimmerman, Mohr and Spangler2009), there appears to be broad consistency among these results. These studies have so far mainly concentrated on the genes 5HTTLPR and COMT, with one study considering novel genes such as FKBP5. However, there is agreement among the results that would appear to suggest that these genes work in a regulated way to moderate behavior depending on the type of attachment classification that the child represents, and the environment in which the child finds himself or herself. Therefore, for these genetic markers, there is evidence that the presence or absence of a particular allele will influence the child to exhibit more negative externalized behaviors if they also have disorganized attachment, whereas they will exhibit more positive externalized behaviors if they have a secure attachment with their primary caregiver. This is consistent with a differential susceptibility approach (Ellis et al., Reference Ellis, Boyce, Belsky, Bakermans-Kranenburg and van IJzendoorn2011), but also opens up the possibility that multiple gene interactions operate in synchrony to code for vulnerability or resilience in relation to the ontology of caregiver–child behavior. Therefore, future studies will need to increase computational power to disentangle these associations, in tandem with further refinement of the use of biomarkers (e.g., Pappa et al., Reference Pappa, Szekely, Mileva-Seitz, Luijk, Bakermans-Kranenburg, van IJzendoorn and Tiemeier2015).
Methodological considerations in genomics and attachment
There are a number of explanations that we propose could aid in understanding the lack of consistency that exists within the results. The inclusion and exclusion criteria for this review allowed studies that observed any candidate or de novo genetic marker in interaction with the environment. This increased the breadth of studies that we could include within the review. It could be argued from the key findings of our review that results for dopaminergic markers, particularly DRD4 and DRD2, are showing little consistency in their replicability across time, and it is possible to see shifts away from these candidate genes into consideration of other biomarker systems. This is somewhat surprising given the frequent observation in infants of associations between DRD4 genes and the development of arousal and homeostatic regulation in the first 12 months of life (Papageorgiou & Ronald, Reference Papageorgiou, Ronald, Centifani and Williams2017). However, it may be the case that these DRD4 associations are linked to nascent development of the infant's individual capacity to attend and regulate states, rather than the dyadic coregulation of social interaction that we see in attachment behavior. Therefore, it is less that DRD4 is not implicated in attachment per se, but that DRD4 operates as a biological substrate within other regulatory systems, in tandem or overlapping with the attachment system. Our findings for G × E interactions support this notion, particularly for DRD4 and 5HTTLPR.
There is also the possibility that, as with genetic research in psychiatric disorders, we will see a process of research moving on “rapidly and essentially ad infinitum” (Roisman et al., Reference Roisman, Booth-Laforce, Belsky, Burt and Groh2013, p. 385) as results from larger samples fail to replicate previous results. Our synthesis supports this not only with the DRD genes candidates but also with genes in the oxytocin system, such as OXTR. Again, OXTR rapidly emerged as a relatively new candidate gene implicated in attachment, given its association with social interaction (Bakermans-Kranenburg & van IJzendoorn, Reference Bakermans-Kranenburg and van IJzendoorn2014; Leerkes et al., Reference Leerkes, Gedaly, Zhou, Calkins, Henrich and Smolen2017; Luijk, Roisman, et al., Reference Luijk, Roisman, Haltigan, Tiemeier, Booth-LaForce, van IJzendoorn and Bakermans-Kranenburg2011; Roisman et al., Reference Roisman, Booth-Laforce, Belsky, Burt and Groh2013). While there was interest in this gene, as part of a hormonal system linked to human social bonding (Carter, Reference Carter1998), it has already been suggested that other than assisting in neonatal environments, such as childbirth and breastfeeding, there is little evidence to show that it is functional in developing external social behaviors (Bakermans-Kranenburg & van IJzendoorn, Reference Bakermans-Kranenburg and van IJzendoorn2014; Bos, Reference Bos2016). Furthermore, emerging lines of enquiry around both the use of intranasal OXT as a treatment in neurodevelopmental disorders and on endogenous OXT point to little evidence of consistent associations between OXT and social cognition (Keech, Crowe, & Hocking, Reference Keech, Crowe and Hocking2018). This pattern of identification of Candidate Gene × Trait association, followed by lack of replicability, presents something of a dilemma for developmental psychopathology (and the field of G × E interactions as a whole). A consensual approach may be to acknowledge the difficulty in observing genetic biomarkers in isolation and look to identification of multivariate associations as a more fruitful line of enquiry.
A further critique of the literature reviewed here is to suggest that the studies included have such a breadth of heterogeneous variables that between-study comparisons are hampered from the start. For instance, while a number of the studies observe the interaction between genetic marker and maternal sensitivity on attachment, still other studies chose to observe the interaction between the gene and a different variable within the child's environment. The challenge is therefore both a methodological and a theoretical one. From a methodological standpoint, this broad spectrum of covariates, and variation in measures within variables (e.g., sensitivity) introduces error and bias and could inflate the risk of contradictory findings. From a theoretical standpoint, the lack of consistency between studies that evaluate the same environmental variable also points to the presence of additional factors presently unaccounted for in existing models. Successful modeling of these associations therefore requires construction of larger samples, evoking the international consortia assembled for the large-scale analyses in psychiatric genetics (e.g., Milaneschi et al., Reference Milaneschi, Lamers, Peyrot, Baune, Breen, Dehghan and Penninx2017). As noted earlier in our discussion, we also suggest that clearer reporting as to whether G × E studies consider attachment as the outcome, or as the “E” in a G × E interaction would also aid future research in establishing replicable patterns of association.
Considerations for theoretical frameworks involving attachment and genetics
If we take the aforementioned complexity as a given, we can then reformulate the G × E problem in attachment via classic developmental psychopathology concepts of multifinality and equifinality (Cicchetti & Rogosch, Reference Cicchetti and Rogosch1996). Even when children start out with seemingly the same environmental and biological factors, they may, nonetheless, develop along different trajectories; conversely, they may find themselves traveling along the same developmental pathway from very different starting points. This tension can be observed in the contradictory interpretations of the recent meta-analysis of the transmission gap (Barbaro et al., Reference Barbaro, Boutwell, Barnes and Shackelford2017; Verhage et al., Reference Verhage, Schuengel, Madigan, Fearon, Oosterman, Cassibba, Bakermans-Kranenburg and van IJzendoorn2016, Reference Verhage, Pasco Fearon, Schuengel, van IJzendoorn, Bakermans-Kranenburg, Madigan and Brisch2018). Our review thus suggests that gene–environmental interaction could be considered the baseline for enquiry into the role of genetics in attachment, and that we need to attend more clearly to how we parse and delineate the environmental variables in these models.
The findings in the current review give strong support to the importance of differential susceptibility as an explanatory framework within which associations between genes, attachment, and other developmental factors can be understood (Bakermans-Kranenburg & van IJzendoorn, Reference Bakermans-Kranenburg and van IJzendoorn2007; van IJzendoorn & Bakermans-Kranenburg, Reference van IJzendoorn and Bakermans-Kranenburg2019). As previously discussed, differential susceptibility theory argues that a specific gene (or a composite of multiple genes combined into a polygenic susceptibility score; Belsky & Beaver, Reference Belsky and Beaver2011) may serve as a risk factor when exposed to a negative environment, but conversely, may enhance development when placed in optimal conditions. This is most easily discerned within the studies observing the interaction between genetic marker and attachment, moderating externalized behaviors. One potential implication of our review is that this pattern of associations, aligned to concepts of multifinality, equifinality, and differential susceptibility, paves the way for future genetics of attachment studies to take advantage of epigenetics, the dynamic process whereby genes respond to the experiences within the child's environment (Dudley, Li, Kobor, Kippin, & Bredy, Reference Dudley, Li, Kobor, Kippin and Bredy2011). Epigenetic expression is a complex system through which interactions may lead to a number of different phenotypic, and behavioral, outcomes (Bos, Reference Bos2016). Emerging findings suggest that incorporating variance epigenetic processes into standard G × E models of attachment and developmental outcomes may clarify patterns of G × E association (Meaney, Reference Meaney2010). For instance, findings from the Generation R cohort suggest that FKBP5 methylation (an epigenetic binding protein associated with HPA axis function) moderates the associations between the FKBP5 genotype and resistant attachment with cortisol reactivity (Mulder et al., Reference Mulder, Rijlaarsdam, Luijk, Verhulst, Felix, Tiemeier and van IJzendoorn2017). Findings such as these lead us to understand the interaction between gene and environment, not as a simple model (Figure 2), but as a complex modeling in which factors are not only influencing each other in a determined way but are at the same time being impacted upon in a dynamic nature (Figure 3; Champagne, Reference Champagne2016). Future studies may therefore wish to augment consideration of the genetic aspects of G × E interactions on attachment through consideration of genome-wide epigenetics.
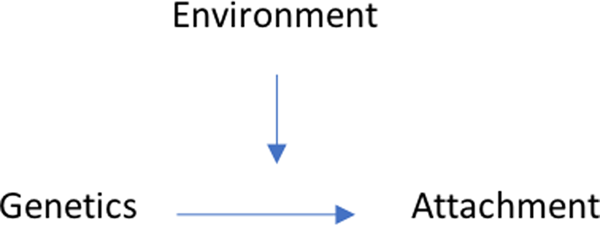
Figure 2. Suggested influences of Gene × Environment on attachment.

Figure 3. Suggested influences of Gene × Environment (attachment) on behavior.
Limitations
These implications notwithstanding, there are a number of limitations of the review that should be highlighted in order to gain a greater understanding of the results contained within. One methodological issue that may need to be addressed in future studies is the measurement of attachment that is used. Verhage et al. (Reference Verhage, Schuengel, Madigan, Fearon, Oosterman, Cassibba, Bakermans-Kranenburg and van IJzendoorn2016) suggest that by using different measures of attachment (i.e., dimensional vs. categorical), this may change the operationalization of the measurement. The authors also question the interreliability of measuring attachment at different ages, using different tools.
While there are 27 studies assessed in the review, these are representative of only 18 cohorts. This leads to an overrepresentation of some of the cohort samples for information, especially the Generation R cohort (which is appraised 4 times within the review). As these cohort studies use the same population samples repeatedly, and are only slightly manipulating the environmental variables, there is likely to be a strong concurrence between their results each time. While this may lead to a results table that appears to lend convincing support for one argument, it is important to bear in mind that each cohort could be considered as only one result, indicating weaker results than are presented currently. In addition, small sample sizes used in a number of the studies could create underpowered work from which it is difficult to extrapolate meaningful results. Leading on from this is the number of genetic markers that were measured against environmental influences. Roisman et al. (Reference Roisman, Booth-Laforce, Belsky, Burt and Groh2013) have argued that with the sheer number of genetic markers that could be nominated as candidate genes, combined with the tendency to use smaller, underpowered, samples, there is more scope for researchers to report significant associations where they may not appear in larger study samples.
Given the heterogeneity of measurement variables and methodologies, we suggest that there is yet insufficient numbers and homogeneity within published studies to justify a meta-analysis. However, it would lend more strength to the presented results to be able to statistically analyze outcomes across studies, and it seems a reasonable proposition that this will become a viable approach in the near future. However, we note that synthesis of these results is compounded by variations in the measurement of genetics (e.g., different variants of dopamine markers including DRD4, DRD2, and COMT), which further reduce comparability of samples. We also note that the small number of studies for each outcome introduces risk of small study effects on any meta-analytical estimates. As it stands, this literature does not seem homogenous enough to sustain a meta-analysis. However, as contemporary G × E studies accumulate, it would be viable to synthesize these results via meta-analysis.
Implications for research
The review's findings generate a number of implications for research, which we propose could influence the next generation of G × E studies involving attachment, and in some instances these are already being enacted. As underpowering and reliance on small studies is a key weakness of the literature, it would be advisable that more cohorts work together to increase the power of their sample sizes, as evidenced by domains such as psychiatric genetics research (Papageorgiou & Ronald, Reference Papageorgiou, Ronald, Centifani and Williams2017). Parallels can be drawn with other areas of research from development, through to behavioral economics, where genomics is increasingly being used to leverage understanding of the links between G × E and behavioral outcomes. This also lends itself to the types of complex modeling that are already widespread in the field of developmental psychopathology.
A further, complementary avenue of research is to explore and model the longitudinal effect of genetic biomarkers and their interaction with dynamic changes in the environment in which the child is developing (Bakermans-Kranenburg & van IJzendoorn, Reference Bakermans-Kranenburg and van IJzendoorn2011). As noted above, epigenetics is not a deterministic factor, but instead operates as a dynamic system that constantly affects the individual from childhood and onward throughout the life course (van IJzendoorn, Caspers, Bakermans-Kranenburg, Beach, & Philibert, Reference van IJzendoorn, Caspers, Bakermans-Kranenburg, Beach and Philibert2010). It may also be suggested that the genotypes within the child's system are influencing behavior at different ages throughout development, which in turn would influence the mechanisms of developing attachment security (Drury et al., Reference Drury, Gleason, Theall, Smyke, Nelson, Fox and Zeanah2012; Papageorgiou & Ronald, Reference Papageorgiou, Ronald, Centifani and Williams2017). A longitudinal study would be more effective in detecting such influences across time. Equally, in addition to methylation, there are other potential epigenetic markers that could be explored in relation to attachment, including chromatin structure and noncoding RNA (Gartstein & Skinner, Reference Gartstein and Skinner2017). In taking this approach, there is the potential to link attachment research to the burgeoning field of developmental origins of health and disease (the DoHaD hypothesis; O'Donnell & Meaney, Reference O'Donnell and Meaney2016; Wadhwa, Buss, Entringer, & Swanson, Reference Wadhwa, Buss, Entringer and Swanson2009). Other options to delineate longitudinal associations would be to incorporate experience sampling methodologies into measurement of behavioral or caregiving variables, as has been successfully demonstrated in research into at-risk psychopathologies in young adults (Myin-Germeys et al., Reference Myin-Germeys, Oorschot, Collip, Lataster, Delespaul and van Os2009)
Leveraging new and emergent technologies for genomic and epigenomic research may also open additional opportunities for developmental psychopathology. While many of the studies included within the current review focused on specific genes, there is evidence to suggest that observing Gene × Gene interactions could produce meaningful findings, as demonstrated by Cicchetti et al. (Reference Cicchetti, Rogosch and Toth2011), who report that the significant interaction between DRD4 and 5HTTLPR influenced attachment organization, rather than one gene candidate or another. Similarly, Pappa et al. (Reference Pappa, Szekely, Mileva-Seitz, Luijk, Bakermans-Kranenburg, van IJzendoorn and Tiemeier2015) also argue that the genetic substrate of the endophenotype of disorganized attachment may be the result of multiple genes of small effect working in concert. The use of genome-wide association study (GWAS) technologies could not only shed light on these gene-to-gene interactions but also elucidate further epigenetic pathways that may impact on attachment downstream from the genetic action. These would include additional factors implicated in synaptic transmission. Rather than specifying a priori genetic markers, GWAS is a bottom-up approach, using computational modeling to survey the whole genome to identify potential genetic markers that associate with the outcome variable. Papageorgiou and Ronald (Reference Papageorgiou, Ronald, Centifani and Williams2017) argue that by using this method of genetic testing, the researcher does not have to hypothesize about the mechanisms of one specific gene, and instead allows a broader view into the effects that multiple genes are enacting upon each other. The application of this methodology in the Pappa et al. (Reference Pappa, Szekely, Mileva-Seitz, Luijk, Bakermans-Kranenburg, van IJzendoorn and Tiemeier2015) study demonstrates proof of concept to identify novel genes, and their pathways associated to both disorganization and attachment security. This type of broad, dynamic study would allow researchers to understand the influence of multiple genetic, neural, and environmental factors working at the same time.
In summary, the current review and evidence synthesis demonstrates the change in the complexity of research in genetic markers for attachment over the last 20 years, from investigation of main effects of genes on attachments, to a contemporary position that situates gene markers and attachment within complex systems models, where attachment may constitute an outcome in interaction with another environmental factor or factors, or may itself constitute an environmental factor in relation to other developmental outcomes. In both cases, these G × E interactions can at present be best understood within a differential susceptibility model of development. Given the heterogeneity of outcomes noted in our review, there is still a need for further research into the field of attachment organization and modeling of the complex systems that are involved in delineating the longitudinal unfolding of the attachment system. This includes modeling of the factors contributing to the transmission gap. Our findings also support the need for more robust approaches as to how we conceptualize and measure gene markers in relation to attachment, which emerges as a key methodological finding of our review. For the field to keep pace with other genomic research endeavors (such as contemporary psychiatric genetics) will require larger samples and new approaches to explore the contribution of novel genes, suggestive chromosomal loci, cation transport, synaptic pathways, GWAS, and SNPs in interaction with a child's environment. We propose that application of these new approaches and the advent of probabilistic epigenetics offer significant opportunities for developmental psychopathology researchers to improve their understanding of how genes and environment interact throughout the life course to influence, and be influenced by, attachment.
Acknowledgments
This review was prepared as part of L.G. and K.d.K’s MSc dissertations. We acknowledge the support of Rowena Stewart, librarian at the University of Edinburgh, in assisting with implementation of the search strategy.
Author contributions
L.G., K.d.K, and A.M. designed the study and the protocol. Search strategy, data extraction, and data checking were conducted by L.G. and K.d.K The initial draft of the manuscript was completed by L.G., and all authors contributed to the final draft.
Author ORCIDs
Angus MacBeth, 0000-0002-0618-044X